Abstract
Multi-kinase inhibitors have been established for the treatment of advanced renal cell cancer, but long-term results are still disappointing and immunotherapeutic approaches remain an interesting experimental option particularly in patients with a low tumor burden. DC are crucial for antigen-specific MHC-restricted T cell immunity. Furthermore, allogeneic HLA-molecules pose a strong immunogenic signal and may help to induce tumor-specific T cell responses. In this phase I/II trial, 7 patients with histologically confirmed progressive metastatic RCC were immunized repetitively with 1 × 107 allogeneic partially HLA-matched DC pulsed with autologous tumor lysate following a schedule of 8 vaccinations over 20 weeks. Patients also received 3 Mio IE IL-2 s.c. once daily starting in week 4. Primary endpoints of the study were feasibility and safety. Secondary endpoints were immunological and clinical responses. Vaccination was feasible and safe with no severe toxicity being observed. No objective response could be documented. However, while all patients had documented progress at study entry, 29% of the patients showed SD throughout the study with a mean TTP of 24.6 weeks (range 5 to 96 weeks). In 3/7 patients, TH1-polarized immune responses against RCC-associated antigens were observed. In one patient showing a minimal clinical response and a TTP of 96 weeks, clonally proliferated T cells against yet undefined antigens were induced by the vaccine. Vaccination with tumor antigen loaded DC remains an interesting experimental approach, but should rather be applied in the situation of minimal residual disease after systemic therapy. Additional depletion of regulatory cells might be a promising strategy.
Introduction
Advanced RCC is among the few malignant diseases that have demonstrated responsiveness to immunotherapeutic intervention and is therefore considered potentially immunogenic.Citation1,Citation2 The long-term outcome of patients with metastatic RCC remains poor, despite novel targeted agents, which have been implemented into therapy. Additionally, more than half of the patients, who have undergone nephrectomy, will relapse locally or with distant metastases.Citation3 Therefore, experimental treatment options are needed.
In RCC, clinical responses with therapeutic vaccinations have so far been limited.Citation4-Citation6 However, the most appropriate setting for immunotherapy approaches might rather be in the clinical situation of minimal residual disease e.g., after successful systemic therapy or surgery.Citation7 In the therapeutic setting different vaccination strategies have been pursued. Tumor cells transfected with co-stimulatory molecules and/or cytokines have been used in order to increase immunogenicity.Citation8 We have previously reported on a phase I study with allogeneic gene-modified tumor cells (RCC26/IL-7/CD80) in RCC patients.Citation9
DC are potent APC Citation10-Citation12 and play a crucial role in the induction of antigen-specific T cell responses. However, DC-based vaccination in RCC patients has been limited by the scarce knowledge about frequently expressed TAA in RCC. Various different strategies have been used to provide appropriate TAA including the use of tumor-associated peptides or tumor RNA. The vaccines have been analyzed in different clinical settings with only modest responses.Citation13-Citation15 Due to the lack of appropriate TAA in RCC, DC-based vaccination has been performed with tumor cells or lysate as a source of TAA. Different phase I/II trials using DC pulsed with tumor lysates (either from autologous tumors or allogeneic tumor cell lines) have demonstrated modest clinical responses.Citation16-Citation19 More recently, DC-tumor cell hybrids were used as a vaccine, but they also showed only modest efficacy.Citation20,Citation21 The use of allogeneic rather than autologous cells has been established since allogeneic HLA molecules pose a potent immunogenic signal and may lead to T cell responses through expression of immunostimulatory cytokines by allo-reactive T cells.Citation19,Citation22
IL-2 is a cytokine which activates cytotoxic T cells, natural killer cells and T helper cells, all of which may contribute to an antitumor immune response.Citation23 Furthermore, IL-2 has been shown to be an effective drug in a particular subgroup of patients with metastatic RCC.Citation24-Citation26
In the current phase I/II study, a DC-based vaccine consisting of allogeneic, partially HLA- matched DC, pulsed with autologous tumor lysate, was evaluated in patients with metastatic RCC. Primary endpoints of the study were feasibility and safety. Secondary endpoint was the induction of an immunological and/or clinical response.
Results
DC production
After 8 d of culture, monocytic precursor cells had acquired a typical DC morphology. Viability was always > 85%, T cell contamination in the cell culture was less than 7% (range 2–7%). FACS analysis after 5 d showed an immature phenotype, after a further 3–4 d of culture in the presence of TNF-α, the immune-phenotype was consistent with mature DC showing lineage- negative cells with high expression of CD80, CD83, CD86 and HLA- DR. In 84 different preparations for clinical application the mean yield of DC per seeded PBMC was 6.3%, with a range of 1.5–14.7%.
Clinical results
According to the Memorial Sloan Kettering Cancer Center (MSKCC) risk score ,Citation27 3 patients belonged to the favorable risk group and 5 patients to the intermediate risk group. All patients had multiple metastatic sites, mostly involving the liver and lymph nodes. Patient characteristics are shown in .
Table 1. Patient characteristics
Patient # 2 withdrew her consent before vaccination was started. All other patients were vaccinated and evaluable for response. Two patients (# 3 and 8) received all 8 scheduled vaccinations through week 20. Five patients (# 1, 4, 5, 6 and 7) were taken off study owing to disease progression in weeks 10, 5, 9, 11 and 14 according to the protocol. 5/7 patients received IL-2 according to the protocol, patient # 6 refused application of IL-2 in week 4 and patient # 8 refused IL-2 application in week 8 due to typical IL-2- related adverse effects (flu- like symptoms grade 1–2).
Vaccination was feasible and safe with no vaccine-related grade 3 or 4 toxicity observed. Mild local skin reactions not fulfilling criteria for a positive DTH-reaction were observed at the reaction site in most patients. Marginal transient Ca2+ elevations were observed in three patients (# 3, 5, 8), the causative relation to vaccination remained unclear. Mild nausea (grade 1–2) was noted in four patients (# 5, 6, 7, 8). Mild grade 1–2 arthralgia was seen in two patients (# 6 and 8). As these latter patients had refused receiving IL-2, this was possibly vaccine-related. In one patient (# 5), clinical deterioration due to disease progression was noted in week 8. He developed fever of unknown origin with signs of septic organ dysfunction. After recovery, he was discharged home, where he died from disease progression a few weeks later.
No objective clinical responses could be observed. However, 2/7 patients showed stable disease throughout the study and the median time to progression (TTP) was 24.6 weeks (range 5–96), with the longest TTP being 96 weeks (patient # 3). This latter patient with a TTP of 96 weeks showed a minimal clinical response of pancreatic lesions and was still alive in December 2012 at a follow-up of 94 mo. Additionally, in this patient, vaccination induced two clonally proliferated T cell populations with productive rearrangement of their TCRβ-genes, which are currently under further investigation ( and data not shown).
Figure 1. TCR repertoire of patient 3 showing a minimal clinical response. PBMC of patient 3 were analyzed before (upper panel) and after vaccination (middle and lower panel) for clonally expanded T cell populations by TCRβ-PCR. Fluorescent fragment analysis profiles of “reaction 1“ show two dominant clonal peaks (*) with identical fragment length, which increase during follow-up. Green peaks indicate amplification of rearrangements involving Jβ1 gene segments; blue peaks indicate amplification of rearrangements involving Jβ2 gene segments; red peak: size marker.
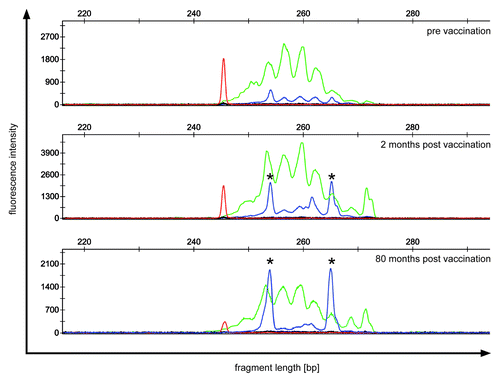
Immunological results
DTH reactions remained negative in 4 patients (# 1, 4, 5, 7), while mild to moderate DTH reactions were observed in 3 patients (# 3, 6, 8). Skin biopsies at the injection site 48h after vaccination revealed scarce cellular lymphoid infiltrates in 4 patients (# 3,6,7,8). These infiltrates predominantly consisted of CD3+CD4+ T cells. Interestingly, in patient # 3, who showed a T cell response and a very long TTP, lymphoid infiltrates together with eosinophilic and plasmacellular infiltration could be observed ().
Peptide-specific T-cell responses
Antigen-specific T-cell responses against several HLA class-I-restricted RCC- associated peptides were determined before and throughout the study by IFN-γ ELISpot and CBA. Before treatment, significant antigen-specific T-cell responses could not be detected in most patients. However, low-level peptide-specific T cells were detectable before treatment in two patients, recognizing Survivin, TYMS, G250, Cyclin D1, Vimentin, ILGF-BP3, ADFP and c-MET (patient # 3) and TYMS, G250, ILGF-BP3 and Vimentin (patient # 7).
Following vaccination, peptide-specific T cells recognizing Survivin/TYMS/Cyclin D1 (patient # 3); TYMS/G250/Vimentin (patient # 7) and TYMS/G250 (patient # 8) were detectable in 3 patients. In all patients, these T cells showed a predominant TH1-cytokine profile ( and ).
Figure 3. IFN- γ ELISpot in patients no. 3 and 7. PBMC from patients before vaccination and at different time points after vaccination were stimulated in vitro with different tumor-associated peptides from survivin, vimentin, ADFP, TYMS, cyclin-D1, c-Met, ILGF and G250. Peptide-specific T cell responses were detected by IFN- γ ELISpot. In Patient no. 7, data from TP2 were not available due to disease progression before this time point. TP, time point.
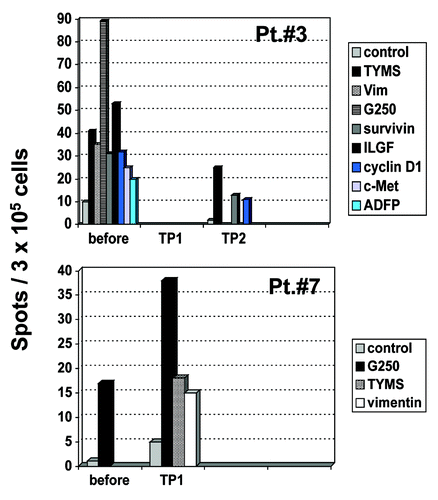
Figure 4. Cytometric bead array in immunized patients before and during vaccination. PBMC from patients before vaccination and at different time points during vaccination were stimulated with different tumor-associated peptides (survivin, vimentin, ADFP, TYMS, cyclin D1, c-Met and G250). Peptide-specific cytokine secretion was measured by CBA. The TH-polarization (TH1 vs. TH2) of the cytokine profile is indicated for each time point and for each peptide. Grey field = TH1 profile, white field = TH2 profile. Borderline = did not meet predefined criteria for a peptide-specific response. NA, not available; TP, time point.
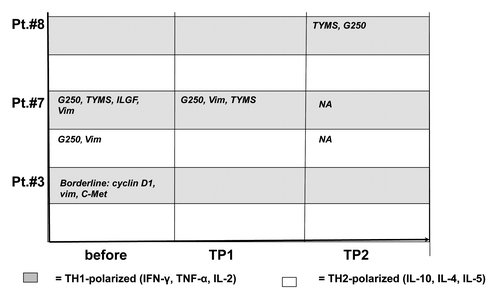
Functional responsiveness of peripheral blood T cells
In order to assess general responsiveness of the patients´ T cells, proliferation assays were performed after stimulation with PHA and IL-2 or allogeneic, partially HLA-matched DC. After stimulation with PHA/IL-2, the patients´ PBMC showed a normal proliferative capacity, without significant changes during vaccination (). Stimulation with allogeneic, partially HLA-matched DC without autologous tumor lysate also revealed adequate proliferative responses of the patients´ T cells, there was a trend toward a higher proliferation during vaccination, however, this was not statistically significant (). Stimulation of PBMC with allogeneic DC loaded with autologous tumor cell lysate did not reveal a significant increase in proliferation during vaccination in most of the patients (). The capacity of patients´ T cells to secrete IFN-γ upon non- specific stimulation with PMA was also assessed. In comparison with healthy donors, no significant differences were observed ().
Figure 5. Proliferative capacity of patients’ PBMC. Proliferative capacity of PBMC from patients before vaccination and at different time points after vaccination in response to (A) PHA/IL-2; (B) irradiated allogeneic, partially HLA-matched unloaded DC; (C) irradiated allogeneic, partially HLA-matched DC which were loaded with autologous tumor lysate. CpM, counts per minute; TP, time point.
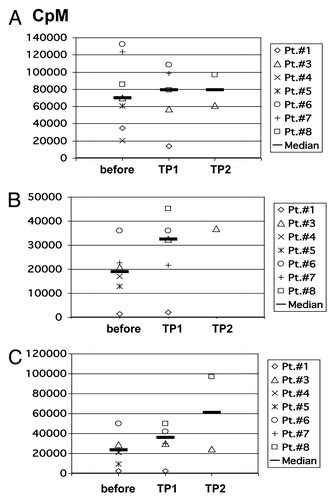
Table 2. IFN-γ secretion of PBMC from patients and healthy donors after stimulation with PMA
Induction of allo- and auto-antibodies
Patients were tested repetitively for the presence of HLA antibodies. One patient (# 7) remained negative throughout the study. One female patient (# 3) already showed HLA class II antibodies when entering the protocol (anti-HLA-DR13 and –DQ6).
One patient (# 4) developed HLA class I antibodies in week 5 detecting HLA-A3, -A9, -B27, -Cw7. At least the specificity for –A3 could be attributed to the DC donor carrying HLA-A3.
Patient # 6 showed HLA class II antibodies in week 10 specific for –DQ4, 5, 6, 7, 8, 9.Patient # 8 developed HLA class I antibodies in week 20 which detected HLA-A2, -A23, -A24, -A25, -A32, -A68, -A69, -B38, -B49, -B51, -B52, -B53, -B57, -B58, -B59, -B63, -B77. Class II antibodies specific for HLA-DQ7, -DQ8 and –DQ9 were also observed. The antibodies specific for –A2 and –B51 could be related to the DC donor carrying these characteristics (). Two patients could not be evaluated continuously (patients # 1 and 5). All patients were tested for anti-nuclear antibodies and rheumatoid factor. No significant changes were seen throughout the study (data not shown).
Table 3. HLA types of patients and healthy donors for DC generation
Analysis of peripheral blood effector cells
Flow cytometric analysis of lymphocyte subpopulations before and during vaccination did not show any significant changes (data not shown). For detection of a clonal expansion of T cells, even T cells with unknown specificity, peripheral blood samples of 7 patients were analyzed by standardized T-cell-receptor (TCR) γ- and β-PCR before and at different time points during vaccination. In 6/7 patients no vaccination-associated T-cell clones were found (data not shown). However, in patient # 3 two dominant TCR rearrangements could be detected in week 10 and were still present in week 20. These dominant rearrangements are currently persisting for over 80 mo, while the patient is still alive showing stable disease under medication with an oral kinase inhibitor (). Sequencing of the two dominant rearrangements detected in the TCRβ-PCR revealed two productive rearrangements, arguing for two distinct T cell clones (data not shown). The specificity of these T cell clones is under further investigation.
The percentage of TSDR+CD4-T cells at baseline was slightly higher than in healthy controls (8.2 ± 3.1% vs. 6.4 ± 1.6% in healthy controls, n.s.). During vaccination, there was a slight increase of TSDR+CD4-T cells in four patients (patients # 1, 3, 7 and 8), however, these changes were not statistically significant (data not shown).
Discussion
In RCC patients, the clinical efficacy of DC-based vaccines has so far been disappointing (reviewed inCitation28). Two major causes for the failure of DC vaccines to induce clinically relevant immune responses are related to tumor-induced immunosuppression in cancer patients and insufficient T cell stimulation by the DC vaccines themselves.Citation29,Citation30 In the past decade, multiple efforts have been made to improve the immunostimulatory capacity of DC vaccines, i.e., by additional immuno-modulating agents, such as recombinant IL-2, diphtheria toxin conjugate DAB(389) IL-2 or low-dose cyclophosphamide.Citation13,Citation31,Citation32 However, all of these strategies only led to very limited progress with respect to clinical efficacy.
Allogeneic DC were introduced into vaccine trials since they may provide additional immunostimulatory signals through allo-reactive T cells secreting high levels of T cell activating cytokines. Improvement of vaccine efficacy by allogeneic DC derived from healthy donors has previously been described in RCC.Citation19,Citation22,Citation33,Citation34
In order to induce MHC-restricted T cell responses against tumor antigens using allogeneic DC, a partial HLA match between patient and DC donor remains a prerequisite. Therefore, in our study protocol, a minimum of two matching HLA-class-I-alleles was chosen as an inclusion criterion. Concomitant IL-2 was given for further activation of antitumor T cell responses.Citation23
In our study, allogeneic DC loaded with autologous tumor lysate were used due to the lack of frequently expressed tumor antigens in RCC and because autologous tumor lysate may serve as a source for a broad spectrum of tumor associated antigens, even yet unknown epitopes.
We showed that vaccination with allogeneic, partially HLA-matched DC is feasible and safe with no severe toxicity being observed.
While all patients showed progressive disease at study entry, disease stabilization for up to 96 weeks was observed and the median TTP was 24.6 weeks (range 5–96 weeks). No objective response could be documented, however, in one patient (no. 3), a minimal clinical response was observed. Interestingly, a T cell response against RCC-associated antigens and increasing numbers of clonally proliferated T cells were observed particularly in this patient.
For the analysis of T cell responses, we used HLA class I restricted peptides from different RCC-associated antigens, which may serve as surrogate markers for immune monitoring.Citation9,Citation35,Citation36 The different peptides from RCC-associated antigens have originally been selected under the aspect of binding to HLA-A 0201 by using motif-based epitope prediction available on the web (http://www.sypeithi.de). However, some of these peptides are also high affinity binders for other HLA molecules explaining detectable immune responses against TYMS, G250 and vimentin in HLA-B08 and HLA-A2402 positive patients. Or results show that immune responses against the selected tumor antigens can be detected before vaccination only in 2/7 patients (# 3 and 7). General immunogenicity of our vaccine is demonstrated by the induction of antibodies against allogeneic HLA alleles in 3/7 patients. The specificity of most “foreign” HLA class I antibodies that were detected (except those expressed on donor DC) could be explained by broad cross-reactivity among different HLA allotypes. With regard to T cell responses against RCC- associated antigens, our data show a heterogeneous pattern: In patients # 7 and 8, vaccination was able to induce TH1- polarized T cells recognizing TYMS and G250 (). In patient # 8, these results were underlined by an increase in T cell proliferation induced by antigen-loaded DC (). In contrast, in patient # 3 T cell responses as measured by ELISpot and CBA rather decreased during vaccination, although it was particularly this patient who achieved a mixed clinical response in combination with a TTP of 96 weeks and an increasing number of clonally proliferated T cells in the peripheral blood (). In 4/7 patients the vaccine failed to induce a detectable immune response, at least against the epitopes chosen as surrogate antigens for immune monitoring. The overall limited ability of the vaccine to induce strong T cell responses may have different possible explanations: it might express the inability of T cell responses measured in peripheral blood to reflect systemic immune responses as has been previously suggested.Citation37 It might also show that the chosen antigens are not the clinically relevant rejection antigens or it might reflect that the vaccine itself is not sufficiently immunogenic. The latter possibility is supported by the fact that proliferation assays (depicted in ) failed to show a statistically significant increase in response to antigen-loaded DC during vaccination in most of the patients. Slightly increasing numbers of Treg observed in 4/7 patients may underline the possibility of insufficient immunogenicity of the vaccine, at least in most of the patients. It remains an open question whether this is primarily due to suboptimal characteristics of the vaccine itself, cancer- induced immunosuppression in the patients, or both.
We have previously reported on vaccination with RCC-26/IL-7/CD80, an allogeneic gene-modified tumor cell line. In this trial, a substantial impairment of IFN-γ secretion of patients´ lymphocytes was observed, the vaccine induced TH2-polarized immune responses, and an increase of Treg could also be observed in some patients.Citation9
In the present trial, this general impairment of IFN-γ secretion in the patients´ T cells was not observed (). It seems likely that this is due to different baseline characteristics of the patients on these small phase- I/II trials, whereas post- vaccination values could have been influenced by concurrent IL-2 administration.
In summary, in this phase I/II trial we could document the feasibility and safety of our vaccine as well as its principal ability to induce TH-1-polarized immune responses against RCC-associated antigens in patients. However, the immunogenicity of the vaccine was low and did not translate into clinically relevant T cell responses in most cases, although a considerably long TTP was observed in some of the patients, particularly in those showing a DTH and a peptide-specific T cell response. Due to the small number of patients in this phase I/II trial, we could not establish a clear correlation between clinical course and immune responses observed (). Nevertheless, it is a very interesting issue whether the clonally expanding T cells detected in patient # 3 show any specificity for RCC-associated antigens, additional experiments are underway. Since this particular patient showed a mixed response and a very long TTP, it is tempting to speculate that the vaccine was in fact able to induce an anti-tumor immune response in this patient.
Table 4. Clinical and immunological results: overview
In the view of the current literature, there is no consensus on the optimal approach for DC-based vaccines. Particular points of concern are the use of immature vs. mature DC (migratory vs. antigen- presenting capacity), autologous vs. allogeneic DC and the source of TAA.
The use of allogeneic DC in RCC vaccine trials has previously been described by several groups.Citation19,Citation22,Citation33,Citation34
The phase I/II- trial published by Märten et al.Citation22 analyzed a vaccine of allogeneic DC fused with either autologous or allogeneic tumor cells in 12 patients with mRCC. Immunological responses were detected in 7/12 patients. Clinical responses were modest with SD being achieved in four patients. In the phase I/II- trial published by Avigan et al.,Citation19 allogeneic DC fused with autologous tumor-derived cells were used. Antitumor immune responses were observed in 10/21 patients and 10/21 patients either showed PR or SD. Zhou et al.Citation33 have reported on allogeneic DC fused with patient-derived tumor cells. 7/10 patients achieved either PR or SD. In contrast, the conclusion from a study by Höltl et al.Citation31 using allogeneic DC loaded with tumor lysate ± cyclophosphamide was that the immunogenicity of their allogeneic DC vaccine is inferior to autologous DC.Citation16
Tumor lysate remains an interesting source of TAA, as it contains multiple and possibly yet unknown peptides. However, the future use of tumor lysate under GMP conditions is hampered by the lack of a clear specification, a high variability, regulatory issues concerning tissue collection and difficulties in monitoring immune responses. Under these aspects, DC transfected with tumor RNA offer significant advantages. With respect to DC maturation, the cytokine cocktail can be improved for optimal generation of TH1-polarized IL-12-producing DC, which are able to induce strong CTL responses.Citation38-Citation41 Since several trials have shown that DC vaccines may also induce Treg, it should be addressed whether the interaction of DC with Treg can be influenced independently from other effector cells.
Meanwhile, the use of autologous tumor material as a source of antigens has become increasingly complicated by regulatory issues. Furthermore, tumor material is a poorly defined and inhomogeneous source of antigens. Therefore, in the future we will prefer well-characterized allogeneic tumor cell lines such as RCC26-IL7/B7.1 and RCC26-IL2/B7.1Citation42,Citation9 as allogeneic vaccines. These cell lines combine the advantages of allo-stimulation with a well-defined and homogenous antigen pattern.
Concomitant inhibition of immunosuppressive mechanisms, such as Treg depletion or combination with anti-inhibitory agents such as PD-1- or CTLA4-antibodies may be a promising strategy to increase vaccine efficacy, particularly in the setting of minimal residual disease after successful systemic therapy or surgery.
Material and Methods
Production of dendritic cells
PBMC from partially HLA-matched healthy donors with at least two matching HLA class I antigens were provided by the Department of Transfusion Medicine. In HLA- A 0201 positive patients, an HLA-A 0201 positive donor was matched, since responses against HLA-A 0201- restricted peptides have been reported.Citation9,Citation35 The patients´ HLA types and the matching HLA types of healthy donors for allogeneic PBMC are depicted in . After the donors´ written informed consent 1–2 × 1010 PBMC were separated in 1–3 leukapheresis sessions. PBMC were enriched by density gradient on Lymphoprep (Nycomed Pharma, Oslo, Norway) and then stored on liquid nitrogen using RPMI 1640 (Bio Whittaker, Verviers, Belgium) with 42.5% human serum albumin (20%) (Octapharma, Langenfeld, Germany) and 10% DMSO (Sigma, Irwine, Scotland). Cultivation of dendritic cells was performed under GMP conditions in our GMP facility at Charité Campus Berlin- Buch as has been previously described.Citation43 Briefly, monocytes were enriched by plastic adherence after thawing.
Monocytes were then cultured for 5 d in RPMI 1640 plus 5–10% autologous plasma. Medium contained 1000 U/ml GM-CSF (Sandoz, Nürnberg/Essex Pharma, München, Germany), 500 U/ml IL-4 (Schering-Plough, Kenilworth, NJ, USA),, and 1% sodium pyruvate. Cell density at the beginning of the cell culture was approximately 5 × 105 cells/ml. On day 3, 2/3 of the medium was exchanged. On day 4, culture medium containing 100 μg/ml tumor cell lysate was added. On day 5, final maturation of DC was induced by the addition of 50 ηg/ml TNF-α (Bender, Wien, Austria) for another 3–4 d. On days 8/9, final quality control including morphologic and immunophenotypic analysis, as well as assays for sterility and viability were performed. After washing twice, DC were frozen on liquid nitrogen in aliquots of 1.5 × 107 cells with 40% HBSS (BioWhittaker), 10% human serum albumin, 42.5% autologous serum and 7.5% DMSO (Sigma). After thawing and control of viability, cells were resuspended in NaCl 0.9%. Before subcutaneous application, cells were irradiated with 30 Gy to prevent a transfusion- related GvHD, which has been reported in immunologically compromised cancer patients. Patients were then injected s.c. in the inguinal region with 1 × 107 tumor lysate-pulsed allogeneic dendritic cells in weeks 1, 3, 5, 8, 11, 14, 17 and 20. Starting in week 4, patients also received 3 Mio IU IL-2 sec.c daily. Tumor lysate for the DC vaccine was prepared from frozen autologous samples. HBSS (BioWhittaker) and gentamycin 50 μg/ml (Ratiopharm, Ulm, Germany) were added before homogenization by a glass homogenizer (Schütt, Göttingen, Germany). After repeated freezing and thawing, particle-containing samples were selected and frozen in aliquots after radiation with 25 kGy. Quality assessment for sterility and endotoxin content was performed before freezing.
Vaccination schedule
After informed consent, 8 patients with AJCC stage IV renal cell cancer were enrolled in this clinical phase I/II trial. The protocol had been approved by the local ethics committee. Inclusion criteria were age 18–80 y, histologically proven renal cell cancer without curative surgical option and at least one measurable marker lesion, Karnofsky performance score > 70, interval between last surgery and enrolment in the trial at least 4 weeks, life expectancy > 3 mo and written informed consent. Additionally, autologous tumor material had to be available. Exclusion criteria were severe impairment of organ function, evidence of psychiatric disease, clinically active autoimmune disease, immunodeficiency or constant treatment with immunosuppressive drugs, organ allografts, history of severe allergy, pregnancy or nursing and evidence of any other malignant disease. Patients with clinically active infections or cerebral metastases were also excluded. Patient characteristics are given in . Vaccination was performed between 2004 and 2006. Primary objectives of the study were feasibility and safety. Secondary endpoint was the induction of an immunological and/or clinical response.
Clinical monitoring
Clinical examination and routine laboratory checks were performed at every visit (weeks 1, 3, 5, 8, 11, 14, 17 and 20). Patients were assessed for clinical signs of autoimmune disease, anti-nuclear antibodies, rheumatoid factor and complement factors. Blood samples for immune monitoring were taken before vaccination and in weeks 10 (TP1) and 20 (TP2). In the case of progressive disease, the last blood sample was taken when the patient was set “off study.” Tumor assessment with CT was performed in weeks 10 and 23. Patients were withdrawn from the study upon evidence of disease progression, according to the response evaluation criteria in solid tumors.Citation44 Further treatment followed current guidelines for treatment.Citation45-Citation47
Immune monitoring
DTH testing
The patients were assessed for a DTH reaction of the skin 48 h after each s.c. injection of the irradiated vaccine. DTH reactions were judged as being positive if an induration or erythema of at least 10 mm in diameter was observed. Furthermore, skin tests were performed in weeks 10 and 20 at the injection site.
T cell responses
ELISpot assays
PBMC were prepared from heparinized whole blood by density gradient centrifugation on Lymphoprep (Progen, Heidelberg, Germany). Cells were harvested and washed four times at low speed to remove platelets. PBMC were then stored in the vapor phase of liquid nitrogen until assays could be performed at the end of the study. For IFN-γ ELISpot assays, PBMC were thawed, washed with CTL WashTM supplement medium (Cellular Technology Ltd., Cleveland, OH, USA) plus 1% L-glutamine and benzonase nuclease (50 U/ml; Novagen Merck Biosciences, Darmstadt, Germany), rested 2–5 h in serum-free culture medium (CTL test medium) plus 1% L-glutamine and seeded at a density of 1 × 105 cells/well in triplicates on PVDF plates, which had been coated with IFN-γ antibody (MabTech AB, Nacka, Sweden). PBMC were stimulated directly with selected peptides (10 μg/ml) (see below) in serum-free culture medium (CTL test medium), supplemented with 1 μg/ml anti-CD28 antibody (BD Biosciences, San Jose, CA, USA and recombinant IL-2 2 U/ml (Proleukin Chiron, Emeryville, CA, USA) as has been previously described.Citation42,Citation48 Incubation was performed overnight at 37C°, ELISpot plates were developed according to the manufacturer’s instructions. Spots were evaluated using the AID ELISpot reader system with the software version 3.1 (AID Autoimmun Diagnostika GmbH, Strassberg, Germany) and controlled by human audit. Results were expressed as spots per 3 × 105 PBMC. Peptide-specific responses were defined as having (1) a ratio of specific peptide: irrelevant control peptide > 2 and (2) an absolute number of spots > 10.
Peptides for immune monitoring were selected from TAA which are overexpressed in renal cell cancer tumor cells lines such as RCC 26Citation49 using HLA motif-based epitope predictions available on the web (http://www.syfpeithi.de) or as published in the literature.Citation50 The following peptides were used:
Survivin (ELTLGEFLKL 95–104, TLPPAWQPFL 5–14)Citation51; heteroclitic surviving-related peptide (LMLGEFLKL 69–104,Citation52 and EKVRRAIEQL 129–138); cyclin D1 (LLGATCMFV 101–109, RLTRFLSRV 228–236)Citation53; adipophilin (SVASTITGV 129–137, TLLSNIQGV 327–335)Citation54; c-Met proto-oncogene (YVDPVITSI 654–662, VLAPGILVL 6–14, GLIAGVVSI 31–39)Citation54; TYMS (VLEELLWFI, RILRKVEKI, YMIAHITGL); ILGF (AALTLLVLL, LLDGRGLCV)Citation9; vimentin (DLERKVESL 218–226, ILLAELEQL)Citation9 and G250 (HLSTAFARV 217–225; GLLFAVTSV). Peptide synthesis was performed as described previously.Citation48 The peptides were kindly provided by R. Frank, Department of Chemical Biology, Helmholtz Center for Infection Research, Braunschweig, Germany.
Originally, these peptides were only used for immune-monitoring in HLA-A 0201- positive patients, however, using the same motif-based epitope prediction algorithm, some of the peptides were also high-affinity binders to other HLA molecules such as HLA-B 08 and HLA-A 2402 (TYMS peptide RILRKVEKI; (SYFPEITHI scores: 21, 17) and HLA-B 08 (vimentin peptides (DLERKVESL 218–226, ILLAELEQL); (SYFPEITHI score: 27, 17). An irrelevant peptide from the CML fusion protein bcr3/abl2 (SSKALQRPV 926–934)Citation55 was used as a negative control. PMA (500 ηg/ml) was used as a positive control for IFN-γ release.
Cytometric bead array (CBA)
CBA assays permit simultaneous quantification of multiple cytokines in solution by capturing these to spectrally distinct beads. The cytokine pattern measured by the CBA in this study (CBA, Becton-Dickinson) consists of IFN-γ, IL-2, TNF-α, IL-4, IL-10 and IL-5. 1.0 × 105 cells were stimulated directly with selected peptides (10 μg/ml of each peptide) in serum-free culture medium (CTL test medium), supplemented with 1 μg/ml anti-CD28- antibody (BD Biosciences) and 2 U/ml recombinant IL-2 (Proleukin Chiron). Incubation was performed in duplicates overnight in a 96-well tissue culture plate (Falcon, Becton-Dickinson). Culture supernatant was frozen at -20C° until cytokine analysis was performed according to the manufacturer’s instructions. Duplicates were pooled for cytokine analysis. A peptide-specific response was defined as having a ratio “cytokine secretion of specific peptide: cytokine secretion of control peptide “ ≥ 2 and “mean fluorescence intensity of specific peptide: mean fluorescence intensity of control peptide” ≥ 2.
Mixed lymphocyte reaction and proliferation assays
For proliferation assays, triplicates of 1 × 105 PBMC per well were used as responder cells in RPMI 1640 medium supplemented with 4 mM L-glutamine, 25 mM HEPES, 1 mM Na-pyruvate (Lonza, Verviers, Belgium) and 50 μg/ml gentamycin (Ratiopharm), and 10% heat-inactivated fetal calf serum (HyClone, Thermo Fisher Scientific, Logan, UT, USA), further referred to as complete medium. PBMC from healthy donors were used as a positive control. Viability of thawed PBMC was > 70% in all samples, as measured by trypane blue or propidium iodide staining. One × 105 irradiated (30 Gy) allogeneic, partially HLA-matched DC +/− tumor lysate cultured in autologous plasma were used as stimulator cells. Proliferative capacity of the responder cells was measured in a standard 5-d 3H-thymidine proliferation assay. During the last 16 h of the 5-d culture, 1 μCi/ml 3H-thymidine (Amersham Life Science, Buckingham, UK) was added. Cells were harvested and radioactivity was measured in a scintillation counter. Maximum proliferation rate was determined by cultivation of 1 × 105 PBMC in the presence of PHA and IL-2. Minimum proliferation rate was determined by cultivation of PBMC alone. Median values were determined from the triplicates.
Analysis of lymphoid subpopulations in the peripheral blood
EDTA blood was analyzed by immunofluorescence staining using Pharm Lyse solution (Becton-Dickinson) according to the manufacturer’s instructions. Direct immunofluorescence staining was performed in whole blood using the following fluoroisothiocyanat- and phycoerythrin-conjugated monoclonal antibodies: CD3, CD4, CD8, CD19, CD56, CD25, HLA-DR and isotype control (Becton- Dickinson). Three × 103 cells were analyzed on a FACS Calibur (Becton-Dickinson). Analysis was performed on gated lymphocytes.
HLA typing
HLA typing was performed initially at a low-resolution level by PCR using sequence-specific oligonucleotide probes (Dynal RELI_SSO HLA Typing Kits from Dynal, Dynal Biotech, Oslo, Norway). HLA high resolution subtyping was subsequently performed by polymerase chain reaction (PCR), using sequence-specific primers (Olerup, SSP-kits, Genovison ;Qiagen, Wien, Austria).
HLA antibody screening
Screening for HLA-class-I and –class-II antibodies was performed by means of an enzyme-linked immunosorbent assay-based HLA screening assay (Lambda Antigen Tray LATM, OneLambda, Canoga Park, CA, USA), and determination of the HLA antibody specificity was accomplished by the HLA specification assay LAT1288 (OneLambda).
Immunohistological analysis
Sections from paraffin-embedded cutaneous biopsies were deparaffinized and subjected to an antigen-retrieval pretreatment using pronase or high-pressure cooking with citrate buffer. After pretreatment, sections were incubated with primary antibody. The following antibodies were used in this study: CD3 (clone LN10, Leica Biosystems, concentration 1:100), CD4 (clone 4B12, Leica Biosystems, Newcastle, UK, concentration 1:25), CD8 (clone C8/144B, Dako, concentration 1:100), CD56 (clone 123C3, Monosan, concentration 1:10), CD68 (clone PGM1, Dako, concentration 1:50) and CD20 (clone L26, Dako, Glostrup, Denmark, concentration 1:50). Bound antibodies were visualized using the alkaline phosphatase-anti alkaline phosphatase method, employing FastRed as chromogen (reagents obtained also from Dako).
T cell receptor (TCR) analysis by PCR
PBMC were prepared from 10 ml of citrate blood by density gradient centrifugation through Ficoll-Paque-Plus (GE Healthcare, Freiburg, Germany) and genomic DNA was prepared by QIAamp DNA Mini Kit (Qiagen, Hilden, Germany) according to the manufacturer’s recommendations. Samples were then analyzed for the presence of a clonally expanded T cell population by multiplex TCRβ- and TCRγ-rearrangement PCR using primers and protocols developed within the BIOMED-2 BMHA-CT98–3936 Concerted Action initiative.Citation56 Subsequent analysis of the PCR products was done by fluorescence fragment analysis on an ABI PRISM 310 Genetic Analyzer using Gene Mapper 3.7 software (Applied Biosystems, Weiterstadt, Germany).
Dominant peaks in the TCRβ-PCR were verified by sequencing. Therefore, the multiplex PCR reaction was divided into multiple single reactions with different subsets of the Vβ and Jβ primers, until the dominant peaks could be separated to different reaction tubes. Amplification products were purified and sequenced in both directions using the respective set of Vβ and Jβ primers. Sequencing was done by using the BigDye Terminator V1.1 cycle sequencing kit (Applied Biosystems) and subsequent analysis by high resolution electrophoresis on an ABI PRISM 310 Genetic Analyzer. TCR sequences were further analyzed by submission to the International Immunogenetics Information System V-QUEST tool (http://imgt.cines.fr/IMGT_vquest/share/textes).Citation57,Citation58
FOXP3 Treg-specific demethylated region (TSDR) quantification
Genomic DNA was isolated from PBMC samples using the QIAamp R DNA Blood Mini kit (Qiagen). In all, 500 ηg eluted genomic DNA was used in a subsequent bisulfite treatment (EpiTectR, Qiagen). A minimum of 60 ηg bisulfite-treated genomic DNA was sued in a Realtime-PCR to quantify the FOXP3 TSDR as previously described.Citation59 Realtime-PCR was performed in a final reaction volume of 20 μl containing 10 μl FastStart Universal Probe Master (ROX) (Roche Diagnostics, Mannheim, Germany), 50 ηg/μl Lamda DNA (New England Biolabs, Frankfurt, Germany), 5 ρmol/μl methylation or non-methylation specific probe, 30 ρmol/μl methylation or non-methylation specific primers and 60 ηg bisulfite-treated DNA or respective amounts of plasmid standard. Primers and standards were provided by Epiontis GmbH, Berlin, Germany. The samples were analyzed in triplicates on an ABI 7500 cycler, using the following cycling conditions: 1 cycle of 10 min 95C° and 45 cycles of 15s 95C° followed by 1 min 61C°. The % FOXP3 TSDR content was then calculated by dividing the non-methylated copy number by the total genomic FOXP3 copy number. As PBMC samples were used, the obtained results were adjusted to the individual percentage of CD4+ T cells determined by flow cytometry.Citation59 The classification of changes in the percentage of TSDR+ CD4-T cells (increase vs. decrease vs. unchanged) is based on a comparison of the respective values pre- and post-vaccination as these parameters reflect the outcome of the whole vaccination period.
Statistical analysis
Statistical analysis was done descriptively. The differences in parameters between two groups were calculated and the student’s t test was applied in order to determine statistical significance. All statistical analyses were two-sided and a p value of < 0,05 was considered to be statistically significant. Analyses were done by means of SPSS software (SPSS, version 20.0, IBM).
Abbreviations: | ||
AJCC | = | American Joint Committee on Cancer |
APC | = | antigen presenting cell |
CBA | = | cytometric bead array |
CML | = | chronic myeloid leukemia |
CT | = | computed tomography |
CTL | = | cytotoxic T lymphocyte |
CTLA-4 | = | cytotoxic T lymphocyte antigen 4 |
DAB | = | diphtheria toxin conjugate |
DMSO | = | dimethylsulfoxide DNA, deoxyribonucleic acid |
DC | = | dendritic cell |
DTH | = | delayed type hypersensitivity EDTA, ethylenediaminetetraacetic acid |
ELISpot | = | enzyme linked immunospot |
FCS | = | fetal calf serum |
FFA | = | fluorescence fragment analysis |
GM-CSF | = | granulocyte macrophage colony stimulating factor |
GMP | = | good manufacturing practice |
GvHD | = | graft versus host disease |
Gy | = | gray |
HBSS | = | Hanks buffered saline solution |
HLA | = | human leucocyte antigen |
IU | = | international unit |
IL-2 | = | interleukin-2 |
IL-4 | = | interleukin-4 |
IL-5 | = | interleukin-5 |
IL-10 | = | interleukin-10 |
IFN-γ | = | interferon-γ |
KLH | = | keyhole limpet hemocyanin |
NaCl | = | sodium chloride |
ηg | = | nanogram |
mM | = | millimol |
ml | = | milliliter |
μg | = | microgram |
μl | = | microliter |
MSKCC | = | Memorial Sloan Kettering Cancer Center |
PBMC | = | peripheral blood mononuclear cell |
PD-1 | = | programmed cell death protein 1 |
PHA | = | phytohemagglutinine |
PCR | = | polymerase chain reaction |
PMA | = | phorbol myristate acetate |
ρmol | = | picomol |
PVDF | = | polyvinyledene fluoride |
RCC | = | renal cell cancer RPMI, Roswell Park Memorial Institute culture medium |
s.c. | = | subcutaneous |
TAA | = | tumor associated antigen |
TCR | = | T cell receptor |
TH1 | = | T helper cell group 1 |
TNF-α | = | tumor necrosis factor α |
Treg | = | regulatory T cell |
TSDR | = | Treg specific demethylated region |
TTP | = | time to progression |
Disclosure of Potential Conflicts of Interest
No potential conflicts of interest were disclosed.
References
- Rosenberg SA. Progress in human tumour immunology and immunotherapy. Nature 2001; 411:380 - 4; http://dx.doi.org/10.1038/35077246; PMID: 11357146
- Rosenberg SA, Yang JC, Restifo NP. Cancer immunotherapy: moving beyond current vaccines. Nat Med 2004; 10:909 - 15; http://dx.doi.org/10.1038/nm1100; PMID: 15340416
- Janzen NK, Kim HL, Figlin RA, Belldegrun AS. Surveillance after radical or partial nephrectomy for localized renal cell carcinoma and management of recurrent disease. Urol Clin North Am 2003; 30:843 - 52; http://dx.doi.org/10.1016/S0094-0143(03)00056-9; PMID: 14680319
- Fournier P, Schirrmacher V. Randomized clinical studies of anti-tumor vaccination: state of the art in 2008. Expert Rev Vaccines 2009; 8:51 - 66; http://dx.doi.org/10.1586/14760584.8.1.51; PMID: 19093773
- Mocellin S, Mandruzzato S, Bronte V, Lise M, Nitti D. Part I: Vaccines for solid tumours. Lancet Oncol 2004; 5:681 - 9; http://dx.doi.org/10.1016/S1470-2045(04)01610-9; PMID: 15522656
- de Gruijl TD, van den Eertwegh AJM, Pinedo HM, Scheper RJ. Whole-cell cancer vaccination: from autologous to allogeneic tumor- and dendritic cell-based vaccines. Cancer Immunol Immunother 2008; 57:1569 - 77; http://dx.doi.org/10.1007/s00262-008-0536-z; PMID: 18523771
- Sobol RE. The rationale for prophylactic cancer vaccines and need for a paradigm shift. Cancer Gene Ther 2006; 13:725 - 31; http://dx.doi.org/10.1038/sj.cgt.7700950; PMID: 16543919
- Pardoll DM. Cancer vaccines. Nat Med 1998; 4:Suppl 525 - 31; http://dx.doi.org/10.1038/nm0598supp-525; PMID: 9585204
- Westermann J, Flörcken A, Willimsky G, van Lessen A, Kopp J, Takvorian A, et al. Allogeneic gene-modified tumor cells (RCC-26/IL-7/CD80) as a vaccine in patients with metastatic renal cell cancer: a clinical phase-I study. Gene Ther 2011; 18:354 - 63; http://dx.doi.org/10.1038/gt.2010.143; PMID: 21068778
- Steinman RM, Cohn ZA. Identification of a novel cell type in peripheral lymphoid organs of mice. I. Morphology, quantitation, tissue distribution. J Exp Med 1973; 137:1142 - 62; http://dx.doi.org/10.1084/jem.137.5.1142; PMID: 4573839
- Banchereau J, Steinman RM. Dendritic cells and the control of immunity. Nature 1998; 392:245 - 52; http://dx.doi.org/10.1038/32588; PMID: 9521319
- Banchereau J, Schuler-Thurner B, Palucka AK, Schuler G. Dendritic cells as vectors for therapy. Cell 2001; 106:271 - 4; http://dx.doi.org/10.1016/S0092-8674(01)00448-2; PMID: 11509176
- Dannull J, Su Z, Rizzieri D, Yang BK, Coleman D, Yancey D, et al. Enhancement of vaccine-mediated antitumor immunity in cancer patients after depletion of regulatory T cells. J Clin Invest 2005; 115:3623 - 33; http://dx.doi.org/10.1172/JCI25947; PMID: 16308572
- Wierecky J, Müller MR, Wirths S, Halder-Oehler E, Dörfel D, Schmidt SM, et al. Immunologic and clinical responses after vaccinations with peptide-pulsed dendritic cells in metastatic renal cancer patients. Cancer Res 2006; 66:5910 - 8; http://dx.doi.org/10.1158/0008-5472.CAN-05-3905; PMID: 16740731
- Bleumer I, Tiemessen DM, Oosterwijk-Wakka JC, Völler MC, De Weijer K, Mulders PF, et al. Preliminary analysis of patients with progressive renal cell carcinoma vaccinated with CA9-peptide-pulsed mature dendritic cells. J Immunother 2007; 30:116 - 22; http://dx.doi.org/10.1097/01.cji.0000211318.22902.ec; PMID: 17198090
- Höltl L, Zelle-Rieser C, Gander H, Papesh C, Ramoner R, Bartsch G, et al. Immunotherapy of metastatic renal cell carcinoma with tumor lysate-pulsed autologous dendritic cells. Clin Cancer Res 2002; 8:3369 - 76; PMID: 12429623
- Märten A, Flieger D, Renoth S, Weineck S, Albers P, Compes M, et al. Therapeutic vaccination against metastatic renal cell carcinoma by autologous dendritic cells: preclinical results and outcome of a first clinical phase I/II trial. Cancer Immunol Immunother 2002; 51:637 - 44; http://dx.doi.org/10.1007/s00262-002-0324-0; PMID: 12439609
- Kim JH, Lee Y, Bae YS, Kim WS, Kim K, Im HY, et al. Phase I/II study of immunotherapy using autologous tumor lysate-pulsed dendritic cells in patients with metastatic renal cell carcinoma. Clin Immunol 2007; 125:257 - 67; http://dx.doi.org/10.1016/j.clim.2007.07.014; PMID: 17916447
- Avigan DE, Vasir B, George DJ, Oh WK, Atkins MB, McDermott DF, et al. Phase I/II study of vaccination with electrofused allogeneic dendritic cells/autologous tumor-derived cells in patients with stage IV renal cell carcinoma. J Immunother 2007; 30:749 - 61; http://dx.doi.org/10.1097/CJI.0b013e3180de4ce8; PMID: 17893567
- Barbuto JA, Ensina LF, Neves AR, Bergami-Santos P, Leite KR, Marques R, et al. Dendritic cell-tumor cell hybrid vaccination for metastatic cancer. Cancer Immunol Immunother 2004; 53:1111 - 8; http://dx.doi.org/10.1007/s00262-004-0551-7; PMID: 15185011
- Avigan D, Vasir B, Gong J, Borges V, Wu Z, Uhl L, et al. Fusion cell vaccination of patients with metastatic breast and renal cancer induces immunological and clinical responses. Clin Cancer Res 2004; 10:4699 - 708; http://dx.doi.org/10.1158/1078-0432.CCR-04-0347; PMID: 15269142
- Märten A, Renoth S, Heinicke T, Albers P, Pauli A, Mey U, et al. Allogeneic dendritic cells fused with tumor cells: preclinical results and outcome of a clinical phase I/II trial in patients with metastatic renal cell carcinoma. Hum Gene Ther 2003; 14:483 - 94; http://dx.doi.org/10.1089/104303403321467243; PMID: 12691613
- Gansbacher B, Zier K, Daniels B, Cronin K, Bannerji R, Gilboa E. Interleukin 2 gene transfer into tumor cells abrogates tumorigenicity and induces protective immunity. J Exp Med 1990; 172:1217 - 24; http://dx.doi.org/10.1084/jem.172.4.1217; PMID: 2212951
- Fyfe G, Fisher RI, Rosenberg SA, Sznol M, Parkinson DR, Louie AC. Results of treatment of 255 patients with metastatic renal cell carcinoma who received high-dose recombinant interleukin-2 therapy. J Clin Oncol 1995; 13:688 - 96; PMID: 7884429
- Fyfe GA, Fisher RI, Rosenberg SA, Sznol M, Parkinson DR, Louie AC. Long-term response data for 255 patients with metastatic renal cell carcinoma treated with high-dose recombinant interleukin-2 therapy. J Clin Oncol 1996; 14:2410 - 1; PMID: 8708739
- Negrier S, Escudier B, Lasset C, Douillard JY, Savary J, Chevreau C, et al. Recombinant human interleukin-2, recombinant human interferon alfa-2a, or both in metastatic renal-cell carcinoma. Groupe Français d’Immunothérapie. N Engl J Med 1998; 338:1272 - 8; http://dx.doi.org/10.1056/NEJM199804303381805; PMID: 9562581
- Motzer RJ, Bacik J, Murphy BA, Russo P, Mazumdar M. Interferon-alfa as a comparative treatment for clinical trials of new therapies against advanced renal cell carcinoma. J Clin Oncol 2002; 20:289 - 96; http://dx.doi.org/10.1200/JCO.20.1.289; PMID: 11773181
- Nencioni A, Grünebach F, Schmidt SM, Müller MR, Boy D, Patrone F, et al. The use of dendritic cells in cancer immunotherapy. Crit Rev Oncol Hematol 2008; 65:191 - 9; http://dx.doi.org/10.1016/j.critrevonc.2007.10.002; PMID: 18055210
- Hanahan D, Weinberg RA. Hallmarks of cancer: the next generation. Cell 2011; 144:646 - 74; http://dx.doi.org/10.1016/j.cell.2011.02.013; PMID: 21376230
- Draube A, Klein-González N, Mattheus S, Brillant C, Hellmich M, Engert A, et al. Dendritic cell based tumor vaccination in prostate and renal cell cancer: a systematic review and meta-analysis. PLoS One 2011; 6:e18801; http://dx.doi.org/10.1371/journal.pone.0018801; PMID: 21533099
- Höltl L, Ramoner R, Zelle-Rieser C, Gander H, Putz T, Papesh C, et al. Allogeneic dendritic cell vaccination against metastatic renal cell carcinoma with or without cyclophosphamide. Cancer Immunol Immunother 2005; 54:663 - 70; http://dx.doi.org/10.1007/s00262-004-0629-2; PMID: 15918076
- Oosterwijk-Wakka JC, Tiemessen DM, Bleumer I, de Vries IJ, Jongmans W, Adema GJ, et al. Vaccination of patients with metastatic renal cell carcinoma with autologous dendritic cells pulsed with autologous tumor antigens in combination with interleukin-2: a phase 1 study. J Immunother 2002; 25:500 - 8; http://dx.doi.org/10.1097/00002371-200211000-00006; PMID: 12439347
- Zhou J, Weng D, Zhou F, Pan K, Song H, Wang Q, et al. Patient-derived renal cell carcinoma cells fused with allogeneic dendritic cells elicit anti-tumor activity: in vitro results and clinical responses. Cancer Immunol Immunother 2009; 58:1587 - 97; http://dx.doi.org/10.1007/s00262-009-0668-9; PMID: 19221746
- Ranieri E, Gigante M, Storkus WJ, Gesualdo L. Translational mini-review series on vaccines: Dendritic cell-based vaccines in renal cancer. Clin Exp Immunol 2007; 147:395 - 400; http://dx.doi.org/10.1111/j.1365-2249.2006.03305.x; PMID: 17302887
- Schendel DJ, Gansbacher B, Oberneder R, Kriegmair M, Hofstetter A, Riethmüller G, et al. Tumor-specific lysis of human renal cell carcinomas by tumor-infiltrating lymphocytes. I. HLA-A2-restricted recognition of autologous and allogeneic tumor lines. J Immunol 1993; 151:4209 - 20; PMID: 8409397
- Frankenberger B, Pohla H, Noessner E, Willimsky G, Papier B, Pezzutto A, et al. Influence of CD80, interleukin-2, and interleukin-7 expression in human renal cell carcinoma on the expansion, function, and survival of tumor-specific CTLs. Clin Cancer Res 2005; 11:1733 - 42; http://dx.doi.org/10.1158/1078-0432.CCR-04-1883; PMID: 15755994
- Malyguine AM, Strobl SL, Shurin MR. Immunological monitoring of the tumor immunoenvironment for clinical trials. Cancer Immunol Immunother 2012; 61:239 - 47; http://dx.doi.org/10.1007/s00262-011-1148-6; PMID: 22080408
- Kalinski P, Edington H, Zeh HJ, Okada H, Butterfield LH, Kirkwood JM, et al. Dendritic cells in cancer immunotherapy: vaccines or autologous transplants?. Immunol Res 2011; 50:235 - 47; http://dx.doi.org/10.1007/s12026-011-8224-z; PMID: 21717071
- Okada H, Kalinski P, Ueda R, Hoji A, Kohanbash G, Donegan TE, et al. Induction of CD8+ T-cell responses against novel glioma-associated antigen peptides and clinical activity by vaccinations with alpha-type 1 polarized dendritic cells and polyinosinic-polycytidylic acid stabilized by lysine and carboxymethylcellulose in patients with recurrent malignant glioma. J Clin Oncol 2011; 29:330 - 6; http://dx.doi.org/10.1200/JCO.2010.30.7744; PMID: 21149657
- Spranger S, Javorovic M, Bürdek M, Wilde S, Mosetter B, Tippmer S, et al. Generation of Th1-polarizing dendritic cells using the TLR7/8 agonist CL075. J Immunol 2010; 185:738 - 47; http://dx.doi.org/10.4049/jimmunol.1000060; PMID: 20511554
- Zobywalski A, Javorovic M, Frankenberger B, Pohla H, Kremmer E, Bigalke I, et al. Generation of clinical grade dendritic cells with capacity to produce biologically active IL-12p70. J Transl Med 2007; 5:18; http://dx.doi.org/10.1186/1479-5876-5-18; PMID: 17430585
- Buchner A, Pohla H, Willimsky G, Frankenberger B, Frank R, Baur-Melnyk A, et al. Phase 1 trial of allogeneic gene-modified tumor cell vaccine RCC-26/CD80/IL-2 in patients with metastatic renal cell carcinoma. Hum Gene Ther 2010; 21:285 - 97; http://dx.doi.org/10.1089/hum.2008.192; PMID: 19788391
- Westermann J, Kopp J, van Lessen A, Hecker AC, Baskaynak G, le Coutre P, et al. Vaccination with autologous non-irradiated dendritic cells in patients with bcr/abl+ chronic myeloid leukaemia. Br J Haematol 2007; 137:297 - 306; http://dx.doi.org/10.1111/j.1365-2141.2007.06547.x; PMID: 17408402
- Therasse P, Arbuck SG, Eisenhauer EA, Wanders J, Kaplan RS, Rubinstein L, et al. New guidelines to evaluate the response to treatment in solid tumors. European Organization for Research and Treatment of Cancer, National Cancer Institute of the United States, National Cancer Institute of Canada. J Natl Cancer Inst 2000; 92:205 - 16; http://dx.doi.org/10.1093/jnci/92.3.205; PMID: 10655437
- Ljungberg B, Hanbury DC, Kuczyk MA, Merseburger AS, Mulders PF, Patard JJ, et al, European Association of Urology Guideline Group for renal cell carcinoma. Renal cell carcinoma guideline. Eur Urol 2007; 51:1502 - 10; http://dx.doi.org/10.1016/j.eururo.2007.03.035; PMID: 17408850
- Ljungberg B, Cowan NC, Hanbury DC, Hora M, Kuczyk MA, Merseburger AS, et al, European Association of Urology Guideline Group. EAU guidelines on renal cell carcinoma: the 2010 update. Eur Urol 2010; 58:398 - 406; http://dx.doi.org/10.1016/j.eururo.2010.06.032; PMID: 20633979
- Escudier B, Kataja V, ESMO Guidelines Working Group. Renal cell carcinoma: ESMO Clinical Practice Guidelines for diagnosis, treatment and follow-up. Ann Oncol 2010; 21:Suppl 5 v137 - 9; http://dx.doi.org/10.1093/annonc/mdq206; PMID: 20555064
- Brill TH, Kübler HR, von Randenborgh H, Fend F, Pohla H, Breul J, et al. Allogeneic retrovirally transduced, IL-2- and IFN-gamma-secreting cancer cell vaccine in patients with hormone refractory prostate cancer--a phase I clinical trial. J Gene Med 2007; 9:547 - 60; http://dx.doi.org/10.1002/jgm.1051; PMID: 17514769
- Pohla H, Frankenberger B, Stadlbauer B, Oberneder R, Hofstetter A, Willimsky G, et al. Allogeneic vaccination for renal cell carcinoma: development and monitoring. Bone Marrow Transplant 2000; 25:Suppl 2 S83 - 7; http://dx.doi.org/10.1038/sj.bmt.1702362; PMID: 10933197
- Rammensee H, Bachmann J, Emmerich NP, Bachor OA, Stevanović S. SYFPEITHI: database for MHC ligands and peptide motifs. Immunogenetics 1999; 50:213 - 9; http://dx.doi.org/10.1007/s002510050595; PMID: 10602881
- Schmitz M, Diestelkoetter P, Weigle B, Schmachtenberg F, Stevanovic S, Ockert D, et al. Generation of survivin-specific CD8+ T effector cells by dendritic cells pulsed with protein or selected peptides. Cancer Res 2000; 60:4845 - 9; PMID: 10987296
- Otto K, Andersen MH, Eggert A, Keikavoussi P, Pedersen LO, Rath JC, et al. Lack of toxicity of therapy-induced T cell responses against the universal tumour antigen survivin. Vaccine 2005; 23:884 - 9; http://dx.doi.org/10.1016/j.vaccine.2004.08.007; PMID: 15603888
- Sadovnikova E, Jopling LA, Soo KS, Stauss HJ. Generation of human tumor-reactive cytotoxic T cells against peptides presented by non-self HLA class I molecules. Eur J Immunol 1998; 28:193 - 200; http://dx.doi.org/10.1002/(SICI)1521-4141(199801)28:01<193::AID-IMMU193>3.0.CO;2-K; PMID: 9485199
- Weinschenk T, Gouttefangeas C, Schirle M, Obermayr F, Walter S, Schoor O, et al. Integrated functional genomics approach for the design of patient-individual antitumor vaccines. Cancer Res 2002; 62:5818 - 27; PMID: 12384544
- Yotnda P, Firat H, Garcia-Pons F, Garcia Z, Gourru G, Vernant JP, et al. Cytotoxic T cell response against the chimeric p210 BCR-ABL protein in patients with chronic myelogenous leukemia. J Clin Invest 1998; 101:2290 - 6; http://dx.doi.org/10.1172/JCI488; PMID: 9593785
- van Dongen JJ, Langerak AW, Brüggemann M, Evans PA, Hummel M, Lavender FL, et al. Design and standardization of PCR primers and protocols for detection of clonal immunoglobulin and T-cell receptor gene recombinations in suspect lymphoproliferations: report of the BIOMED-2 Concerted Action BMH4-CT98-3936. Leukemia 2003; 17:2257 - 317; http://dx.doi.org/10.1038/sj.leu.2403202; PMID: 14671650
- Brochet X, Lefranc MP, Giudicelli V. IMGT/V-QUEST: the highly customized and integrated system for IG and TR standardized V-J and V-D-J sequence analysis. Nucleic Acids Res 2008; 36:Web Server issue W503-8; http://dx.doi.org/10.1093/nar/gkn316; PMID: 18503082
- Lefranc MP, Giudicelli V, Ginestoux C, Jabado-Michaloud J, Folch G, Bellahcene F, et al. IMGT, the international ImMunoGeneTics information system. Nucleic Acids Res 2009; 37:Database issue D1006 - 12; http://dx.doi.org/10.1093/nar/gkn838; PMID: 18978023
- Wieczorek G, Asemissen A, Model F, Turbachova I, Floess S, Liebenberg V, et al. Quantitative DNA methylation analysis of FOXP3 as a new method for counting regulatory T cells in peripheral blood and solid tissue. Cancer Res 2009; 69:599 - 608; http://dx.doi.org/10.1158/0008-5472.CAN-08-2361; PMID: 19147574