Abstract
Increasing the potency and supply of seasonal and pandemic influenza vaccines remains an important unmet medical need which may be effectively accomplished with adjuvanted egg- or cell culture-derived vaccines. Vaxfectin®, a cationic lipid-based adjuvant with a favorable safety profile in phase 1 plasmid DNA vaccines trials, was tested in combination with seasonal split, trivalent and pandemic whole virus, monovalent influenza vaccines produced in Vero cell cultures. Comparison of hemagglutination inhibition (HI) antibody titers in Vaxfectin®-adjuvanted to nonadjuvanted vaccinated mice and guinea pigs revealed 3- to 20-fold increases in antibody titers against each of the trivalent influenza virus vaccine strains and 2- to 8-fold increases in antibody titers against the monovalent H5N1 influenza virus vaccine strain. With the vaccine doses tested, comparable antibody responses were induced with formulations that were freshly prepared or refrigerated at conventional 2–8°C storage conditions for up to 6 mo. Comparison of T-cell frequencies measured by interferon-gamma ELISPOT assay between groups revealed increases of between 2- to 10-fold for each of the adjuvanted trivalent strains and up to 22-fold higher with monovalent H5N1 strain. Both trivalent and monovalent vaccines were easy to formulate with Vaxfectin® by simple mixing. These preclinical data support further testing of Vaxfectin®-adjuvanted Vero cell culture vaccines toward clinical studies designed to assess safety and immunogenicity of these vaccines in humans.
Introduction
Influenza viruses are a leading cause of acute respiratory infections, with worldwide disease burden estimates of 1 billion cases, 3–5 million severe illnesses, and 300,000–500,000 deaths annually.Citation1 In the US, the estimated annual mortality rate attributed to seasonal influenza between 1976 and 2007 ranged from 3,349 to 48,614, with approximately 90% of deaths in individuals ≥ 65 years old.Citation2 Overall, seasonal influenza infections inflict substantial disease burden resulting in tremendous economic loss.Citation3 In addition to seasonal influenza virus infections, pandemics occasionally arise when a novel influenza A virus subtype infects humans with little to no preexisting immunity, as occurred in 1918, 1957 and 1968, when avian or swine origin influenza viruses reassorted with circulating human strains and developed efficient human-human transmission. Potential pandemics have loomed during the past 15 years from highly pathogenic avian influenza viruses such as H5N1 that incur very high (60%) case fatality rates in humans (www.who.int/influenza/human_animal_interface/EN_GIP_20120810CumulativeNumberH5N1cases.pdf). Although limited sustained human-human transmission has occurred following H5N1 infections to date, recent studies using experimental reassortants found H5N1 transmissibility among ferrets.Citation4 Collectively, seasonal and pandemic influenza viruses represent significant public health concerns.
Vaccines are recognized as the most efficient means of controlling the morbidity and mortality associated with seasonal and pandemic influenza viruses. Unique among all licensed vaccines, seasonal influenza vaccines require annual updating based on predictions of the three prevailing circulating strains for A/H1N1, A/H3N2, and B lineages viruses. Trivalent inactivated influenza vaccines (TIV) are designed to elicit serum antibodies that exhibit hemagglutination inhibition (HI) activity against the hemagglutinin (HA) head of each of these three viruses, a serological correlate of protection.Citation5 The vast majority of licensed influenza vaccines consumed today are: produced in embryonated chicken eggs using egg-adapted influenza A reassortant strains, chemically inactivated and subsequently processed as split or subunit components, standardized for dose based on 15 μg of each HA, and formulated without an adjuvant. The mean vaccine efficacy of egg-derived TIV at preventing confirmed influenza infections has recently been reanalyzed and reported to be 59% for 18–65 y olds by meta-analysis of ten randomized controlled trials.Citation6 A recent case-controlled study during a season when all three vaccine strains were antigenically similar to the circulating strains revealed an overall vaccine effectiveness of 60% among all age groups but only 38% in those ≥ 65 y old.Citation7 Although a systematic review has been undertaken to define egg-derived TIV efficacy and effectiveness in the elderlyCitation8 there is currently only limited evidence from randomized controlled trials for protection by TIV in this high-risk age group.Citation6 Furthermore, although TIV coverage in the elderly increased to 65% during the past few decades, the estimated mortality rates attributed to influenza also increased over the same time.Citation9 The demonstration of influenza vaccine efficacy in the elderly and other high risk groups is complicated by the non-feasibility of conducting placebo-controlled studies in populations for which seasonal influenza vaccination is recommended. The potentially suboptimal protection of TIV in elderly individuals ≥ 65 y old and moderate protection in those < 65 y old indicate that improved influenza vaccines are needed for all age groups.
Strategies to enhance egg-derived TIV immunogenicity, especially in the elderly, have included increased vaccine dosage, intradermal delivery, and formulation with an adjuvant. Randomized controlled trials in ≥ 65 y old subjects have demonstrated superior or noninferior (depending upon the strain tested) serum HI immune responses with high dose TIV (60 μg; 4-fold higher HA/strain) or intradermal injection of standard dose TIV using a microinjection system.Citation10,Citation11 Adjuvants are designed to stimulate innate immune responses which consequently increase adaptive immune responses such as antibody and T-cell responses; they may also facilitate antigen and/or dose sparing.Citation12-Citation15 Recent randomized controlled trials testing squalene-based oil-in-water adjuvant formulations such as MF-59 or AS03 revealed significantly enhanced efficacy in children or significantly enhanced immunogenicity in children and in adults, including those ≥ 65 y old.Citation16-Citation18 Adjuvanted influenza vaccines may be critical to overcoming the limitations of immunosenescence in the elderlyCitation19,Citation20 as well as augmenting immune responses to poorly-immunogenic hemagglutinins such as H5Citation21 in all age groups; adjuvants are required for H5N1 vaccines to achieve robust HI responses.Citation22 Adjuvants may also be critical for expanding the doses available during a pandemic when vaccine demand is high but supplies are low; several adjuvant formulations were experimentally tested in the US and used as part of licensed vaccines in Europe during the 2009 H1N1 pandemic, when there were concerns that vaccine supplies could be limited.Citation23
In this report, a cationic lipid-based adjuvant, Vaxfectin®, was formulated with seasonal and pandemic vaccines produced by Vero cell cultureCitation24-Citation26 and tested for adjuvanting immune responses. Vaxfectin® is being developed for adjuvanting plasmid DNA vaccinesCitation27,Citation28 and has shown a promising safety profile in phase 1 plasmid DNA vaccine trials.Citation29 Initial preclinical studies of Vaxfectin® with an egg-derived TIV revealed significant enhancements of HI antibodies.Citation30 Cell culture-derived influenza vaccines may be important for increasing influenza vaccine supply, particularly during pandemics when the short notice and limited availability of chicken eggs may hinder vaccine productionCitation31; furthermore, they offer several advantages in addition to reducing the dependency on eggs for vaccine production, including mitigating the risk of egg and antibiotic allergies and compressing the manufacturing time line.Citation32 Vero cell culture-derived vaccines have provided significant efficacy of 78.5% in adults 18–49 y old against culture-confirmed, antigenically-matched influenza virus infection in randomized controlled trials of seasonal, split TIVCitation33-Citation35 and have been found to be immunogenic as a pandemic, whole virus vaccine.Citation36 The aims of the preclinical studies in this report were to evaluate the potential of Vaxfectin® to increase the humoral and cell-mediated immune responses to seasonal, split trivalent and pandemic, whole virus monovalent influenza vaccines produced by Vero cell culture as well as to assess the adjuvanted vaccine stability up to 6 mo at 2–8°C.
Results
Formulation of TIV with Vaxfectin®
A seasonal, split trivalent vaccine was produced using Vero cell cultures; monovalent bulks were created from each of the seed viruses antigenically matching A/Solomon Islands/3/06 (H1N1), A/Wisconsin/67/05 (H3N2), and B/Malaysia/2506/04, the three strains selected by the WHO for the Northern hemisphere 2007–2008 influenza season. All experiments with TIV were conducted with these three vaccine strains. TIV doses containing either 15 μg or 7.5 μg of each of the three HA antigens per 0.5 mL injection were formulated as described in the Materials and Methods section with 900 μg of Vaxfectin®. These TIV doses correspond to 1× and ½× of the human vaccine dose, 30 μg/mL and 15 μg/mL of each HA, respectively, or 90 μg/mL and 45 μg/mL of total HA, respectively. The 900 μg Vaxfectin® adjuvant dose was previously found to elicit high levels of antibodies to an egg-derived TIVCitation30; the Vaxfectin®:HA weight:weight ratios tested here were 60 and 120 per each HA (or 20 and 40 for total HA), for the 15 μg and 7.5 μg doses, respectively. All formulations were uniform suspensions with no visible aggregates. Samples were tested for immunogenicity shortly after formulation (Time 0) as well as after 6 mo of storage at 2–8°C, the standard storage temperature for TIV. Sufficient amounts of formulated samples were prepared at Time 0 in order to boost this group at 3 weeks, to prime a separate group of naïve animals at 6 mo, and to boost this 6-mo-group 3 weeks later.
Immunogenicity of 15 μg and 7.5 μg doses of each HA in TIV +/− Vaxfectin® in mice
Two doses of TIV with and without Vaxfectin® were tested for immunogenicity after formulation at Time 0. Female CD-1 mice (n = 10/group) received subcutaneous injections (0.5 mL) at 0 and 3 weeks, and HI titers were assessed from individual serum samples collected at 6 weeks. All mice were negative (HI titer < 10) for all strains prior to first injection. The geometric mean titers (GMT) and 95% confidence intervals (CI) are shown (A–C, open and solid black bars) for each of the three virus strains from each of the 4 vaccine groups (15 μg +/− Vaxfectin® and 7.5 μg +/− Vaxfectin®). Pairwise comparisons of nonadjuvanted to adjuvanted GMT within each dose and strain revealed highly significant p-values (by Mann Whitney U-test). The p-values for HI GMT from both H1N1 dose comparisons and the 7.5 μg dose comparison of H3N2 groups were p < 0.001 while all remaining comparisons were p ≤ 0.01. Vaxfectin® enhanced the HI GMT between 2.6–5.3-fold (GMT ratio, , Time 0 column). In the only instance when HI titers were assessed from pooled serum samples within groups, sera collected at 3 weeks after dose 1 were pooled from mice in each group and tested by HI assay to assess the titers following a single vaccine dose. H1N1 HI titers were 640 for each of the adjuvanted dose groups vs. 40 for each of the nonadjuvanted dose groups; H3N2 HI titers were 1280 and 2560 for the 15 μg and 7.5 μg adjuvanted groups vs. 320 and 160 for nonadjuvanted doses, respectively; and B HI titers were 640 for each of the adjuvanted doses vs. 80 and 40 for the 15 μg and 7.5 μg nonadjuvanted dose groups. Collectively, these results indicate that Vaxfectin® provided an appreciable adjuvant effect using a Vero cell culture-derived vaccine at these TIV doses.
Figure 1. HI titers to TIV strains in mice and guinea pigs. CD-1 mice (A–C) or Dunkan-Hartley guinea pigs (D–F) received two injections at a 3 week interval with the indicated TIV doses with (solid bars) or without (open bars) 900 μg Vaxfectin® (“Vax”) prepared fresh at Time 0 (open and solid black bars) or after 6 mo of storage at 2–8°C (open and solid gray bars, also denoted as “-6” on x-axis labels). The y-axis depicts the GMT (+/− 95% confidence intervals for A–C) of HI antibodies measured from serum collected at week 6 (3 weeks after the second dose). Each of the three rows represents one of the three vaccine strains used to measure HI titers, indicated above each graph.

Table 1. Geometric mean titer (GMT) ratios of hemagglutination inhibition antibodies
Immunogenicity of 15 μg and 7.5 μg doses of each HA in TIV +/− Vaxfectin® in mice after 6 mo storage
Aseptically-prepared stoppered vials containing 15 μg or 7.5 μg of TIV with and without Vaxfectin® were stored for 6 mo at 2–8°C and then tested for immunogenicity. Female CD-1 mice (n = 5/group) received injections as described above for the Time 0 experiment and HI titers were measured from week 6 serum samples. The GMT and 95% CI are shown for each group that received vaccine stored for 6 mo (A–C, open and solid gray bars). Comparisons of HI GMT within vaccine treatment groups at Time 0 and 6 mo revealed no significant differences (p > 0.05). Formulations stored at 2–8°C for up to 6 mo were also periodically evaluated for stability by single radial immunodiffusion (SRD) assay. Interpretation of the SRD data was complicated by the fact that the staining pattern for Vaxfectin® formulations yielded two concentric rings whereas the pattern for TIV alone revealed a single ring (data not shown). For Vaxfectin® preparations, only the inner ring, which corresponded to the single ring observed in the nonadjuvanted formulations, was measured, thereby potentially underestimating the HA-content. shows the percent recovery of each HA at 0, 1, 3 and 6 mo of storage with Vaxfectin® formulations compared with TIV alone. The % HA recovery appeared to gradually decrease with time, with up to nearly 50% lower recovery at 6 mo depending upon strain and dose. However, HPLC analysis of the same samples suggested only a modest decrease in stability; recovery of all HAs varied between 82% to 94% in formulations stored for 6 mo (data not shown). Furthermore, in contrast to the decreasing HA content measured by the SRD assay (), in vivo immunogenicity results showed that Vaxfectin® formulations stored for 6 mo elicited comparable or higher GMT ratios of HI titers compared with freshly prepared formulations, except for the Vaxfectin®-adjuvanted 7.5 μg H3N2 group which had a lower ratio at 6 mo (). GMT ratios of Vaxfectin®-adjuvanted/nonadjuvanted TIV doses are shown to account for any decrease in HI responses with nonadjuvanted TIV over time.
Table 2. Time course of single radial immunodiffusion recoveries of HA in TIV
Immunogenicity of 15 μg and 7.5 μg doses of TIV +/− Vaxfectin® in guinea pigs
An exploratory guinea pig immunogenicity study was conducted to determine if the adjuvant effect of Vaxfectin® on TIV was evident in another species. Female Dunkan-Hartley guinea pigs (n = 3/group) were vaccinated with the same vaccines and schedules used for the CD-1 mouse studies, including TIV formulations at Time 0 and stored for 6 mo. The HI GMT against each of the three influenza virus strains measured from week 6 serum samples are shown in , (D–F). The open and solid black bars represent Time 0 formulations compared with the open and solid gray bars representing formulations stored for 6 mo prior to injection. Statistical analyses were not performed due to the small group sizes. The GMT ratios of HI titers observed in guinea pigs for each of the three strains () indicate an adjuvant effect (range, 2- to 6-fold enhancement for Time 0) that also remained consistent over time, effects similar to that observed in mice.
Dose ranging study of TIV +/− Vaxfectin® in mice
A dose ranging study was conducted to test the antigen sparing capability of Vaxfectin® formulation with TIV doses of 3 μg of each HA through 5-fold decreasing amounts down to 0.0048 μg while maintaining a constant dose of Vaxfectin® (900 μg). Female CD-1 mice (n = 5/gp) received injections at 0 and 3 weeks and HI titers were assessed from serum collected at 6 weeks. The dose response curves indicate a prominent antigen sparing effect that was evident as the dose of each HA decreased relative to a constant Vaxfectin® dose (). Significantly higher HI GMT in adjuvanted vs. nonadjuvanted vaccines were observed for 3–4 of the doses tested for each strain (p < 0.05 by Mann Whitney test for all three strains at the three highest doses; the B strain for the 0.024 μg dose, and the H3N2 strain for the 0.0048 μg dose). Comparisons of HI GMT from animals injected with adjuvanted low doses to nonadjuvanted high doses revealed significant differences (p < 0.05) and allowed an estimate of antigen sparing effect of 5-fold to 25-fold depending upon strain (based on pairwise comparisons and p-values for H1N1, H3N2, and B strains). Negative HI titers (< 10) were observed following injection of Vaxfectin® alone (not shown).
Figure 2. HI titers to different doses of TIV in mice. CD-1 mice were injected as described in legend with the indicated doses of TIV (0.0048–3 μg of each HA) adjuvanted with (black lines) or without (gray lines) 900 μg Vaxfectin®. Significantly higher responses (p < 0.05) were observed for the three highest dose groups.
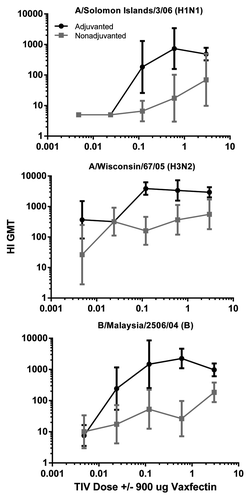
HA-specific IgG subclass typing in mice
The qualitative characteristics of the antibody response induced by different doses of TIV formulated with or without Vaxfectin® were assessed by measuring the concentrations of IgG1, IgG2a, and IgG2b isotypes capable of binding recombinant HA by ELISA. BALB/c mice were injected at 0 and 3 weeks and serum collected at 6 weeks. shows the HA-specific IgG isotype concentrations achieved at TIV doses of 0.015 μg, 0.15 μg and 1.5 μg of each HA alone or in combination with 900 μg of Vaxfectin®. As expected, nonadjuvanted TIV at all three dose levels induced predominantly (50–99%) IgG1 against all three strains. Vaxfectin® provided substantial fold increases in the concentrations of all three isotypes which were most pronounced at the two lowest dose levels; in several cases the fold increases for IgG2 isotypes surpassed those of IgG1 but the absolute IgG2 concentration did not in any instance. The overall predominance of IgG1 was therefore still maintained with Vaxfectin®-adjuvanted TIV but higher levels of IgG2 isotypes were induced relative to TIV alone. HI titers were also measured in this experiment () and the results are consistent with earlier results showing enhanced functional antibody levels with Vaxfectin®-adjuvanted TIV.
Table 3. Concentrations of serum IgG isotypes elicited by TIV +/− Vaxfectin®
Immunogenicity of whole virus, monovalent H5N1 vaccine in mice and guinea pigs
Several doses of monovalent A/Vietnam/1203/04 (H5N1) were tested with and without Vaxfectin® formulation (900 μg) in the same manner described above for TIV testing with vaccine prepared at Time 0 or stored at 2–8°C for 6 mo. CD-1 mice (n = 10/gp for Time 0 study or n = 5/gp for 6 mo study) or Dunkan-Hartley guinea pigs (n = 3/gp for both studies) received vaccine doses at 0 and 3 weeks followed by testing of HI titers from sera collected at 6 weeks. Even with relatively small group sample sizes, significant increases in HI titers were observed in mice at every dose [p < 0.05 by Mann Whitney test for each pairwise comparison within dose at both Time 0 (black bars) and 6 mo (gray bars) time points; ]. A comparison of HI GMT from the 7.5 μg nonadjuvanted H5N1 vaccine group to 1.88 μg Vaxfectin®-adjuvanted group revealed a highly significant p-value (0.0003, Mann Whitney test), suggesting that there is at least a 4-fold dose sparing effect based on this experiment. Due to the small group sizes in the guinea pig studies statistical analyses were not performed but the data in suggested a similar adjuvant effect for these H5N1 doses in this species. The GMT ratio for every dose and time point was 3.2 except for the 7.5 μg dose group at 6 mo (1.2), suggesting that the H5N1-Vaxfectin® formulations maintained stability across these doses over 6 mo. An SRD assay was used to measure the H5 HA stability over this period. The % recoveries of H5 HA in Vaxfectin® formulations for the 15, 7.5, and 3.75 μg doses at Time 0 were 87%, 96%, and 104%, respectively and the % recoveries at these respective doses were 81%, 99%, and 82% at 6 mo. The diminution of H5 HA content as measured by SRD assay was not as much as for the HAs in TIV () but the double rings were also not observed for the whole virus H5N1 vaccine formulated with Vaxfectin® compared with TIV formulated with Vaxfectin® (data not shown). Similar to the HI results for TIV after 6 mo of storage, the HI titers against H5N1 also remained relatively steady (based on GMT ratios above and in ).
Figure 3. HI titers to monovalent H5N1 vaccine in mice and guinea pigs. CD-1 mice (A) or Dunkan-Hartley guinea pigs (B) received two injections at a 3 week interval with the indicated doses of a whole virus A/Vietnam/1203/04 (H5N1) vaccine with (solid bars) or without (open bars) 900 μg Vaxfectin® freshly prepared (Time 0, open and filled black bars) or after 6 mo of storage (open and filled gray bars).
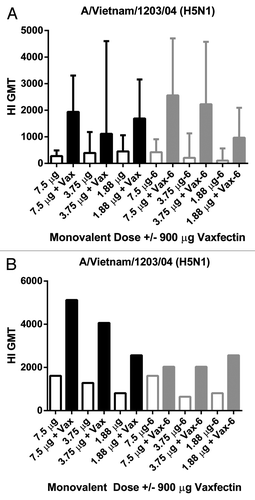
T-cell responses to TIV and monovalent H5N1 +/− Vaxfectin® in mice
Interferon gamma (IFN-γ)- and interleukin-4 (IL-4)-producing T-cell frequencies were measured by ELISPOT assays from mice vaccinated with different amounts of influenza vaccines with or without Vaxfectin®. BALB/c mice were injected at 0 and 3 weeks with TIV containing 0.015 μg, 0.15 μg, or 1.5 μg/HA +/− a 100 μg dose of Vaxfectin® and the T-cell responses were measured from splenocytes harvested at four time points (8, 28, 98 and 196 d after first injection). A 100 μg Vaxfectin® dose provided the highest IFN-γ frequencies to all antigens at all times although the responses were generally similar to the 30 μg Vaxfectin® dose; in contrast, the third Vaxfectin® dose tested, 900 μg, provided the lowest increase in IFN-γ producing T cells at the two lowest TIV doses but reasonable responses for the 1.5 μg/HA dose (data not shown). The IFN-γ responses to each individual monovalent vaccine antigen as well as the trivalent vaccine antigens are shown in (A–C). For each of the three antigens in TIV (H1N1, H3N2, B), robust increases in the IFN-γ spot forming units/106 splenocytes harvested at various time points were noted when TIV was formulated with Vaxfectin® (black bars) compared with nonadjuvanted TIV (white bars stacked beneath black bars). The 1.5 μg/HA TIV group provided the highest overall frequencies over all time points; the enhancement for this TIV dose formulated with Vaxfectin® ranged from 3–20 fold. Of note for all dosages, the longevity of the enhanced responses with Vaxfectin®-formulation persisted out to day 196, the last time point studied. In contrast to the large increases in the frequency of IFN-γ-producing T cells, modest increases in IL-4 frequencies (< 2-fold) were noted for all antigens (data not shown).
Figure 4. Frequency of vaccine-specific IFN-γ-producing T cells. BALB/c mice received two injections at a 3 week interval of the indicated TIV doses with (solid black bars) or without (open bars) Vaxfectin® and an ex vivo IFN-γ ELISPOT assay was used to measure the number of spot forming units (SFU) of IFN-γ T cells per 106 splenocytes harvested at 8, 28, 98, and 196 d after first injection. The TIV doses included 0.015 μg (A), 0.15 μg (B), and 1.5 μg (C) per each HA or the former two for the H5N1 vaccine (D). The rare instances of open bars only (e.g., upper left panel, Day 8 TIV and Day 196 TIV) indicate the adjuvanted response did not exceed the nonadjuvanted response. The sources of antigen for recalling H5-specific responses included H5N1 and recombinant H5 HA (“rH5”). Due to the large number of treatment groups tested, each bar represents the pooled splenocytes of each group; therefore no statistical analyses were possible. The TIV data were derived from two separate studies (0.015 μg and 0.15 μg doses in one study and 1.5 μg dose in a second study).

The IFN-γ-producing T-cell frequencies in mice receiving two different doses of H5N1, 0.015 μg or 0.15 μg, at 0 and 3 weeks were also found to be substantially increased by Vaxfectin®-formulation (D). A dose of 30 μg of Vaxfectin® provided the highest increases among the three Vaxfectin® doses tested (data not shown). D displays the T-cell frequencies recalled after stimulation with H5N1 antigen as well as a recombinant H5 hemagglutinin (“rH5”), with the latter antigen generally lower than the whole H5N1 antigen. Because this vaccine was a whole virus vaccine, it is possible that antigen specificities beyond those directed only to H5 HA may have been generated and are contributing to this response. With the exception of the T cells measured at day 8 after injection of the 0.015 μg dose, all Vaxfectin®-formulated dosages at each time point were 4- to 22-fold higher than the nonadjuvanted H5N1 vaccine at the same dose (compare black to white stacked bars within each column). The longevity of the enhanced responses with Vaxfectin®-formulation persisted out to day 196 for both H5N1 doses.
Discussion
Increasing the potency and supply of inactivated influenza vaccines remain important objectives for decreasing the morbidity and mortality associated with seasonal and pandemic influenza viruses. Adjuvant formulations are recognized as a key strategy for achieving these objectives. A few adjuvanted influenza vaccines are licensed in Europe and a growing number of other candidates are undergoing preclinical and clinical development.Citation13,Citation15 In addition, the use of cell culture-derived vaccines could reduce the dependency on cumbersome egg-based manufacturing processes and potentially offer other advantages such as shortened production time.Citation32 The aims of the studies in this report were to evaluate the potential of a cationic liposome-based adjuvant, Vaxfectin®, to increase the humoral and cell-mediated immune responses to seasonal, split trivalent and pandemic, whole virus monovalent influenza vaccines produced by Vero cell culture.
The most relevant endpoint for preclinical studies evaluating adjuvanted influenza vaccines, including the studies in this report, is the magnitude of enhanced antibodies possessing functional significance, specifically those with hemagglutination inhibition (HI) activity. HI titers have been considered a correlate of protection for influenza vaccines in humansCitation5 and are ideally the best measure for establishing proof of concept in preclinical studies. Furthermore, for trivalent, seasonal vaccines, it is important to demonstrate an adjuvant effect for HI titers against each of the three vaccine strains. Because adjuvant effects can vary with vaccine dose as well as animal model tested, the current studies explored a series of different TIV doses in mice to provide statistical powering and also in pilot guinea pig studies. Significant enhancements of HI titers (p < 0.05) were observed in mice to all three antigens from all TIV doses tested above 0.024 μg/HA ( and ). These results clearly indicate the adjuvant effect of Vaxfectin® for significantly enhancing HI titers to all three strains over a broad dose range and also demonstrate the antigen sparing effect (), which was 5–25-fold depending upon the strain. Although currently the standard TIV doses are 15 μg for each HA (45 μg total HA), adjuvantation of TIV could permit reductions for trivalent antigens of future licensed vaccines, a step that could increase the overall vaccine manufacturing capacity. In addition to CD-1 and BALB/c mice, guinea pigs also responded to Vaxfectin®-formulated TIV with augmented HI titers (), confirming that the Vaxfectin® adjuvant effect is not unique to mice and is likely to be generalizable for protein-based vaccines, similar to its adjuvant effects for a variety of plasmid DNA vaccines in numerous animal models.Citation28
Complementing the findings of enhanced HI titers afforded by Vaxfectin® when freshly (Time 0) formulated with TIV was the findings of the adjuvant effect after 6 mo of storage at 2–8°C for the TIV doses tested, 1× and 1/2× the human doses (). Other adjuvant formulations previously tested with TIV have not necessarily been compatible for storage at this temperature despite evidence of enhanced immunogenicity of freshly-formulated vaccine (Kistner, unpublished). When TIV was tested in both mice and guinea pigs after 6 mo of refrigerated storage, the GMT ratios remained constant for nearly all strains and doses (); this data provides initial feasibility that TIV and Vaxfectin® are compatible in a single vial formulation over time and that the adjuvant effect appears to be stable, which also suggests that there may be minimal degradation of the Vaxfectin® lipids over this time. There appeared to be discordancy between these results from in vivo immunogenicity testing and the in vitro SRD assay testing suggestive of a diminished recovery of TIV HA antigens over 6 mo (), but formulation optimization studies were not performed to either optimize shelf life or extraction method of HA recovery from adjuvant for more accurate measurement. Future efforts will be required to optimize the performance of the SRD assay for detection of HA when formulated with Vaxfectin®, for instance using detergents to solubilize the antigens and prevent diffusion artifacts (e.g., double rings) and, if needed, to identify an excipient to further enhance HA stability. If these measures prove unsatisfactory, then the option remains for extemporaneous (“bedside”) mixing of vaccine and adjuvant just prior to administration. While not ideal, this approach using extemporaneous formulation is feasible, at least in a pandemic setting, as demonstrated by clinical studies showing successful adjuvantation of an H1N1 vaccine.Citation17 Although the SRD assay is currently the reference release assay for influenza vaccines, alternative, assays such as HPLC or others are in development and may ultimately be preferable to the SRD assay.Citation37,Citation38
Influenza viruses with pandemic potential including H5N1, H7N7, H9N2 and other novel subtypes pose continuous threats to humans due to lack of preexisting immunity and the attendant specter of high fatality rates, as exemplified by the continuous infections of humans by H5N1 subtypes since 1997 (WHO). The vast array of different influenza virus subtypes (as many as 170) circulating in and between avian and other natural reservoirs with ample opportunity for reassortment clearly indicates the continuous need for both surveillance as well as proactive development and testing of pandemic vaccine candidates. The most recent H1N1 pandemic in 2009 illustrated numerous measures for improving pandemic preparedness.Citation38 Such preparedness is vital to an understanding of vaccine potency among different viral hemagglutinins; for instance, H5N1 vaccines have inherently poor-to-moderate immunogenicity in humans, requiring high doses for modest seroconversionCitation21 and substantially benefit from adjuvantation (reviewed inCitation39). In the current report, a whole virus A/Vietnam/1203/04 (H5N1) monovalent vaccine adjuvanted with Vaxfectin® provided significant improvements of HI titers in mice () and numerically higher titers were also achieved in guinea pigs (). Immunogenicity testing of Vaxfectin®-formulated H5N1 vaccine after 6 mo of storage indicated stable HI GMT ratios (), results consistent with similar testing performed with TIV (). While a variety of different adjuvant formulations have been clinically tested with H5N1, predominantly squalene-based oil-in-water adjuvants including MF-59, AS03 and AF01,Citation40-Citation42 additional adjuvant formulations such as Vaxfectin® should be developed because it is conceivable that even adjuvant supplies may be limited during a pandemic. In addition, potential safety issues observed for some adjuvant formulations in pandemic settings, most recently with the reported association of AS03-adjuvanted H1N1 vaccine and narcolepsy in children,Citation43 could restrict their annual usage with seasonal vaccines in normal healthy subjects. In addition to squalene-based adjuvants, other cationic liposome-based adjuvants with a variety of different cationic and neutral lipid components have been explored with seasonal and pandemic strains and have demonstrated enhanced immunogenicity,Citation44-Citation46 supporting consideration of cationic liposomes as a discrete adjuvant class. Because the current studies focused on characterization of Vaxfectin®, direct comparisons to other adjuvants were not conducted. Any cationic liposome-based or other adjuvant formulation used in combination with influenza vaccines will require appropriate clinical testing for safety and tolerability to support licensure. Furthermore, continued post-licensure surveillance, as implemented for any vaccine, will be in place through the Vaccine Adverse Events Reporting System, a data collection system cosponsored by the US CDC and FDA, for detecting data on adverse events.
Seasonal TIV formulated with the MF-59 adjuvant has been licensed in numerous European countries since 1997 and has been administered to over 50 million people.Citation15 Recent preclinical mouse studies with a cell culture-derived TIV adjuvanted with MF-59, alumininum, CpG, and other formulations revealed the highest HI titer increases were achieved with MF-59, with up to approximately 15-fold increases in HI titer following two injections of 0.1 μg antigen doses with MF-59 compared with nonadjuvanted vaccine.Citation47 In the studies presented here, two injections of 0.12 μg antigen doses formulated with Vaxfectin® revealed > 20-fold increases in HI titers compared with nonadjuvanted vaccine (). Within each of these preclinical studies, appreciable increases in HI titers to cell culture-derived TIV formulated with the respective adjuvants could be achieved after a two dose injection schedule in influenza-naïve animals. The ability of Vaxfectin® to increase titers in animals previously-exposed to influenza virus infection has not yet been tested but could be addressed in future preclinical studies.
The evaluation of T-cell responses to Vaxfectin®-adjuvanted TIV and H5N1 vaccine strains revealed pronounced enhancements in the frequency of IFN-γ-producing T cells as measured by ELISPOT assays () while IL-4-producing T-cell frequencies were only slightly increased for all doses tested (< 2-fold; data not shown). These enhancements along with the higher levels of serum IgG2a and IgG2b subclass antibody responses observed for the adjuvanted formulations () both indicate that Vaxfectin® has a propensity to promote Th1 responses in mice. An apparent conundrum exists for the development of Vaxfectin® as an adjuvant that simultaneously enhances both HI antibody levels and IFN-γ T cell frequencies because high Vaxfectin® doses (900 μg) provided optimal antibody enhancements while lower Vaxfectin® doses (30 to 100 μg) provided optimal IFN-γ T-cell-producing frequencies. However, this appears to be dose-dependent because appreciable increases in T-cell responses were noted at 28 d and later after first injection for the 1.5 μg TIV dose group formulated with 900 μg of Vaxfectin® but not for lower TIV doses with this Vaxfectin® dose (data not shown). This could mean that discrete antigen presenting cell populations may be stimulated by different adjuvant:antigen ratios and these in turn may influence CD4+ cell differentiation toward those providing help to B cells vs. those producing IFN-γ and other effector cytokines by CD4+ T cells. Recent evidence supports the importance of the CD4+ T-cell response to influenza, including in the elderly, where T-cell responses may be better correlates of protection than HI antibody levels,Citation48-Citation51 so increasing vaccine potency may benefit from not only enhanced HI titers but also enhanced effector T-cell producing IFN-γ, additional antiviral cytokines, or even CD4+ T-cell cytolytic activity. Further preclinical studies can be designed to more thoroughly titrate the doses of both antigen and adjuvant to inform a more narrow testing range for clinical studies to define the optimum conditions for enhancing both humoral and cellular responses in humans.
Vaxfectin® was initially tested as an adjuvant for enhancing humoral immune responses to plasmid DNA vaccinesCitation27 and has subsequently been reported to enhance immune responses and/or protection from pathogen challenge in a wide variety of animal models testing different plasmid DNA vaccines (reviewed inCitation28). Both lipids comprising Vaxfectin® can be synthesized by straightforward, well-defined processes according to good manufacturing practices and Vaxfectin® liposomes are easy to formulate with various forms of antigensCitation28 The results of recent repeat-dose safety and toxicity studies with influenza and dengue DNA vaccines indicate the safety of 2 mg doses administered three times up to 4 mg doses administered four times.Citation52,Citation53 Vaxfectin®-formulated plasmid DNA vaccines have been tested in phase 1 trials at doses up to 1 mg each of DNA and Vaxfectin® administered twice over a three week interval and have demonstrated favorable safety and tolerability profiles as well as evidence of humoral and T-cell mediated immune responses.Citation29 Higher doses of plasmid DNA:Vaxfectin® (2 mg of each) given three times are currently undergoing phase 1 testing by the US. Navy with a dengue vaccine (T. Kochel, personal communication). Although not yet tested clinically with protein-based vaccines, Vaxfectin® tested alone or with plasmid DNA vaccines provides an acceptable safety profile with no tolerability issues in GLP-compliant animal studies as well as Vaxfectin®-adjuvanted plasmid DNA vaccines in phase 1 clinical trials cited above. No tolerability issues were noted for any of the mice or guinea pigs tested in the current studies. A formal GLP-compliant safety-toxicity study, including evaluation of local reactogenicity, to thoroughly assess the safety of multidosing of Vaxfectin®-adjuvanted TIV was beyond the scope of the current studies but would be completed and provided as part of an investigational new product application to regulatory agencies prior to clinical testing.
The adjuvant activity of Vaxfectin® with plasmid DNA vaccines prompted its testing for activity with protein-based vaccines and initial results with commercially-available egg-based TIV revealed enhanced HI titers to influenza A H1N1 and H3N2 strains.Citation30 The studies reported here elaborate on these initial results to include more comprehensive testing of humoral and T-cell mediated immunogenicity for all three TIV strains as well as a whole virus H5N1 produced by Vero cell culture. The mechanisms of Vaxfectin®‘s adjuvant effect for influenza vaccines remain to be fully elucidated but the particle size of these formulations (median diameter ~20 μm) suggests a depot effect could play a role; in addition, the induction of a proinflammatory cytokine profile suggests this formulation may effectively stimulate innate immune responses which in turn augment adaptive immune responses; toll-like receptor pathways dependent upon MyD88 signaling, however, are not required for Vaxfectin®‘s adjuvant effect with TIV.Citation28,Citation54 Additional mechanistic studies would be valuable in understanding and potentially further enhancing the adjuvant activity of Vaxfectin®.
There are several limitations in the current studies. Neutralizing titers were not measured in the current studies and may have provided further information about the adjuvant effect of Vaxfectin® in addition to HI titers. Extensive influenza challenge studies were not conducted although a pilot challenge study was conducted with A/Vietnam/1203/04 (H5N1) using small group sizes (n = 5/group) and 10-fold differences in HA dose from 1.5 μg to 0.000015 μg with and without Vaxfectin®. Survival advantages were observed for Vaxfectin®-adjuvanted groups receiving 0.015 and 0.0015 μg (4/5 and 1/5 mice respectively) compared with nonadjuvanted groups at these doses (3/5 and 0/5 mice, respectively) but this did not reach significance (data not shown). Future studies to demonstrate in vivo protection in challenge models could be designed to build upon the in vivo immunogenicity results reported here. Finally, further in vivo immunogenicity studies employing even lower HA doses may provide more information on the in vivo stability at limiting antigen doses. The current in vivo studies evaluating the immunogenicity of formulations stored for 6 mo tested relatively high doses which may have masked effects evident at lower doses. Further refinements in the SRD or other stability-indicating assays can be addressed in future development work.
In summary, these studies provide evidence of adjuvant effects of Vaxfectin® as determined by increased HI antibody levels and increased IFN-γ-producing T cells to all three influenza strains in a trivalent seasonal vaccine and a monovalent pandemic H5N1 vaccine. All vaccines were produced using Vero cell culture in lieu of eggs by manufacturing processes licensed in Europe for seasonal and pandemic vaccines. The combination of vaccine manufacture in cell culture with an adjuvant formulation such as Vaxfectin® may address some of the shortcomings of current influenza vaccine such as the need for improved efficacy, not only in the elderly but in all age groups, and the need to enhance vaccine supplies, particularly during pandemics. The encouraging performance of Vaxfectin® in the preclinical studies reported here and the favorable clinical safety profile of Vaxfectin® in conjunction with plasmid DNA vaccines support the continued development of Vaxfectin® as an adjuvant for influenza vaccines ultimately for clinical determination of the magnitude of increased immune responses in humans.
Materials and Methods
Vaccines and formulations
The following influenza viruses were kindly provided by the Centers for Disease Control and Prevention (CDC, Atlanta) or the National Institute for Biological Standards and Control (NIBSC, UK): A/Vietnam/1203/04 (H5N1; CDC#2004706280), A/Solomon Island/3/06 (A/H1N1; NIBSC 06/234), A/Wisconsin/67/05 (A/H3N2; NIBSC 05/230) and B/Malaysia/2506/04 (B; NIBSC 05/144). The monovalent bulks for the seasonal split, trivalent inactivated influenza strains were amplified in Baxter’s serum protein free Vero cells for production of seed viruses. Growth of influenza virus strains in Vero cells in 100 L or 6,000 L fermenters containing microcarrier cultures was described previously.Citation25,Citation55 Supernatant virus was harvested, double-inactivated with formalin and by UV irradiation and purified by sucrose gradient ultracentrifugation followed by ultrafiltration/diafiltration.Citation56 Influenza HA antigen content was determined by single radial immunodiffusion (SRD) assays.Citation57 The trivalent strains corresponded to those selected for the Northern hemisphere 2007–2008 influenza season including A/Solomon Islands/3/06 (H1N1), A/Wisconsin/67/05 (H3N2), and B/Malaysia/2506/04. The concentration of each of HA was 30 μg/mL or a total for all three of 90 μg/mL. The H5N1 whole virus vaccine corresponded to the A/Vietnam/1203/04 influenza strain and was prepared as previously described.Citation55
Vaxfectin® consists of a 1:1 molar mixture of cationic lipid VC1052 and neutral co-lipid DPyPE. VC1052 [(±)-N-(3-aminopropyl)-N,N-dimethyl-2,3-bis(cis-9-tetradecenyloxy)-1-propanaminium bromide] was synthesized as previously described.Citation27 DPyPE (1,2-diphytanoyl-sn-glycero-3 phosphoethanolamine) was purchased from Avanti Polar Lipids, Inc. Vaxfectin® was prepared as a dried lipid film from an equimolar chloroform solution of VC1052 and DPyPE in glass vials containing FluroTec® stoppers. The chloroform was evaporated under a stream of nitrogen and Vaxfectin®-containing vials were placed under vacuum overnight.
Specific Vaxfectin® doses tested for each influenza vaccine dose were obtained by creating dried lipid film with the target adjuvant dose. For instance, to achieve the 900 μg Vaxfectin® adjuvant doses, 1.2 mL of trivalent vaccine was added to 2.18 mg of Vaxfectin® as a dried lipid film and incubated overnight at 2–8°C followed by 10 sec of vortexing. Formulations were acceptable if the visual appearance was a uniform suspension with no visible aggregates. After Vaxfectin® formulation, the pH of the trivalent seasonal and monovalent pandemic vaccines was 7.38 ± 0.03 and the median diameter of particle sizes was < 25 μm and < 20 μm, respectively. Additional characterization of Vaxfectin® formulation of egg-based TIV has been previously reported.Citation54
Influenza viruses were titered using a hemagglutination assay. This assay is based on the ability of influenza viruses to bind to and agglutinate red blood cells (RBC). A fixed amount of RBC was mixed with an equal volume of 2-fold dilutions of influenza virus preparations in V-shaped microtiter plate and incubated for 30 min at room temperature. The titer was defined as the last dilution showing complete hemagglutination and was expressed as the reciprocal value of this dilution.
Animals
Female mice were obtained from Charles River Laboratories, Germany, and held in quarantine for one week prior to use. BALB/c mice (8–10 weeks of age) or CD-1 mice (6–7 weeks of age) weighed approximately 20 g at the time of first injection. Female Dunkan-Hartley guinea pigs were obtained from Charles River Laboratories, Germany, and held in quarantine for one week prior to use. The guinea pigs (5–6 weeks of age) weighed 350–400 g at the time of first injection.
Mice and guinea pigs were immunized subcutaneously in a 500 μL volume with the indicated dose of vaccine at day 0 and then an equivalent homologous booster dose was administered three weeks after primary injection. Three weeks after the booster injection (week 6), serum was collected for determining the hemagglutination inhibition titers.
Animal experiments were approved by the Institutional Animal Care and Use Committee and Austrian regulatory authorities and were conducted in accordance with Austrian laws on animal experimentation and guidelines set out by the Association for Assessment and Accreditation of Laboratory Animal Care International (AAALAC) and the Office of Laboratory Animal Welfare (OLAW). Animals were housed according to OLAW and AAALAC guidelines, in housing facilities accredited by the AAALAC.
Immunological assays
Hemagglutination assays for each of the seasonal virus strains in TIV and the pandemic H5N1 strain were conducted as described below. For seasonal strains, sera were treated with a receptor-destroying enzyme (RDE) to remove potentially interfering reactivity, inactivated at 56°C and serially diluted (2-fold dilution series starting at a 1:10 dilution). The pre-treated sera were pre-incubated at a ratio of 1:1 with an egg-grown influenza virus antigen adjusted to a HA titer of 8 HA units (HAU) per 50 µL. Following addition of 0.4% chicken RBCs, the suspensions were carefully mixed, incubated at room temperature and the HI titer was determined. Sera giving a negative signal in the first dilution (1:10) were assigned a nominal HI titer of 5. Titers were expressed as reciprocal of serum dilution.
For measurement of HI titers to the pandemic H5N1 strain, HI assays were performed according to established procedures but using horse RBCs (World Health Organization, WHO manual on animal influenza diagnosis and surveillance 2002, WHO/CDS/CSR/NCS/2002.5;Citation58). Sera were treated with receptor destroying enzyme (Vibrio Cholera neuraminidase, SERVA Electrophoresis AG, Heidelberg) to remove potentially interfering reactivity, inactivated at 56°C and serially diluted (2-fold dilution series starting at a 1:10 dilution). Prior to addition of erythrocytes, the pre-treated sera were mixed at a ratio of 1:1 with H5N1 virus antigen preparations adjusted to a HA titer of about 3 and pre-incubated for 30–40 min at room temperature. Following addition of erythrocytes, the suspensions were carefully mixed, incubated for 45–60 min at room temperature and the HI titer was determined. Sera giving a negative signal in the first dilution (1:10) were assigned a nominal HI titer of 5.
HA-specific IgG isotypes were measured by ELISA from serum collected at week 6 following TIV doses injected at 0 and 3 weeks. Briefly, ELISA plates were coated overnight at 4°C with either baculovirus-derived recombinant rHA (Protein Sciences, UK) or polyclonal anti-mouse F(ab’)2 IgG (Sigma) for sample and standard wells, respectively. After blocking of nonspecific binding and washing of the plates, appropriately diluted test sera were added to the wells containing rHA, and serial dilutions of purified murine IgG1, IgG2a or IgG2b (Sigma) were added to the standard wells. Following incubation for 1 h at room temperature, the plates were washed again and IgG-subclass specific peroxidase-conjugated goat-anti-mouse IgG antibodies were added to all wells for 1 h. The binding of IgG subclass antibodies was measured after color development with TMB substrate. The IgG subclass concentrations were read off a standard curve and results were expressed as µg/mL.
The frequency of IFN-γ or IL-4 -secreting cells was analyzed using mouse IFN- γ and IL-4 ELISPOT kits (Mabtech AB, Nacka, Sweden) following the instructions of the manufacturer. Polyvinylidene difluoride-coated 96-well plates (Millipore Corp. Bedford, MA) were coated with anti-IFN-γ or anti-IL-4 monoclonal antibodies overnight at 4°C. Splenocytes were distributed in the plates at a concentration of 2 × 105 cells/well and stimulated with monovalent bulks from inter-pandemic influenza strains A/H1N1/Solomon Islands, A/H3N2/ Wisconsin, A/H5N1/Vietnam/1203/04, B/Malaysia and TIV at a concentration of 1 µg HA/mL. Wells containing no antigen or 0.1 µg/mL of pokeweed mitogen (Sigma, St. Louis) were used as negative and positive controls, respectively. The plates were incubated overnight at 37°C and the cells were discarded. After extensive washing with PBS, IFN-γ or IL-4 spots were detected by biotinylated IFN-γ- or IL-4-specific antibody followed by addition of streptavidin-alkaline phosphatase and development of spots with BCIP/NBT substrate solution. Spots were counted by an automated ELISPOT reader (AID, Strassberg, Germany). The number of spots observed in wells containing only medium without antigen was subtracted from the number of spots observed in wells containing specific antigen and the results were expressed as the number of spot forming units (SFU)/106 spleen cells.
SRD assay
Quantification of specific HA was performed using calibrated standard reference antigens and specific corresponding anti-HA serum for each vaccine strain obtained from NIBSC or the Center for Biologics Evaluation and Research of the US. Food and Drug Administration. The SRD assay was performed in an agarose gel system to measure the size of precipitin rings formed by the interaction of HA antigens and specific antiserum; the size was directly proportional to the HA antigen quantity. The HA content of each influenza vaccine preparation was calculated by slope ratio analysis plotting the values of the diameters of the precipitation zones against the dilution of reference antigen and vaccine preparations. The result for each HA was expressed in μg of HA per mL.
Statistical analyses
All graphical displays and statistical comparisons were produced using GraphPad Prism version 6 (GraphPad Software, Inc.). The levels of HI titers for each treatment group were expressed as geometric mean titers (GMT) +/− 95% confidence intervals. HI negative samples were assigned a value of 5 for purposes of GMT calculation. For pairwise comparisons of Vaxfectin®-adjuvanted to nonadjuvanted influenza vaccine treatment groups, statistical significance was determined using Mann-Whitney U-test and all p < 0.05 were considered statistically significant. No adjustments were made for multiple comparisons.
Abbreviations: | ||
CI | = | confidence interval |
ELISA | = | enzyme-linked immunosorbent assay |
ELISPOT | = | enzyme-linked immunospot |
GMT | = | geometric mean titer |
HA | = | hemagglutinin |
HI | = | hemagglutination inhibition |
IFN | = | interferon |
SRD | = | single radial immunodiffusion |
SFU | = | spot forming units |
TIV | = | trivalent inactivated influenza vaccine |
Author Disclosure and Conflict of Interest
All authors are employees of either Vical Inc. or Baxter and may own company’s stock or stock options.
Acknowledgments
The authors thank Claudia Stanislaw, Franz Ferdinand Roch and Isabella Strini for technical assistance with the immunogenicity and stability studies and Lynne “Condor 3” Goldacker for editorial assistance with this manuscript.
References
- Girard MP, Cherian T, Pervikov Y, Kieny MP. A review of vaccine research and development: human acute respiratory infections. Vaccine 2005; 23:5708 - 24; http://dx.doi.org/10.1016/j.vaccine.2005.07.046; PMID: 16154667
- Thompson MG. Estimates of Deaths Associated with Seasonal Influenza-United States, 1976-2007. Morb Mortal Wkly Rep 2010; 59:1057 - 62
- Molinari NA, Ortega-Sanchez IR, Messonnier ML, Thompson WW, Wortley PM, Weintraub E, et al. The annual impact of seasonal influenza in the US: measuring disease burden and costs. Vaccine 2007; 25:5086 - 96; http://dx.doi.org/10.1016/j.vaccine.2007.03.046; PMID: 17544181
- Imai M, Watanabe T, Hatta M, Das SC, Ozawa M, Shinya K, et al. Experimental adaptation of an influenza H5 HA confers respiratory droplet transmission to a reassortant H5 HA/H1N1 virus in ferrets. Nature 2012; 486:420 - 8; PMID: 22722205
- Ohmit SE, Petrie JG, Cross RT, Johnson E, Monto AS. Influenza hemagglutination-inhibition antibody titer as a correlate of vaccine-induced protection. J Infect Dis 2011; 204:1879 - 85; http://dx.doi.org/10.1093/infdis/jir661; PMID: 21998477
- Osterholm MT, Kelley NS, Sommer A, Belongia EA. Efficacy and effectiveness of influenza vaccines: a systematic review and meta-analysis. Lancet Infect Dis 2012; 12:36 - 44; http://dx.doi.org/10.1016/S1473-3099(11)70295-X; PMID: 22032844
- Treanor JJ, Talbot HK, Ohmit SE, Coleman LA, Thompson MG, Cheng PY, et al, US Flu-VE Network. Effectiveness of seasonal influenza vaccines in the United States during a season with circulation of all three vaccine strains. Clin Infect Dis 2012; 55:951 - 9; http://dx.doi.org/10.1093/cid/cis574; PMID: 22843783
- Jefferson T, Rivetti D, Rivetti A, Rudin M, Di Pietrantonj C, Demicheli V. Efficacy and effectiveness of influenza vaccines in elderly people: a systematic review. Lancet 2005; 366:1165 - 74; http://dx.doi.org/10.1016/S0140-6736(05)67339-4; PMID: 16198765
- Simonsen L, Reichert TA, Viboud C, Blackwelder WC, Taylor RJ, Miller MA. Impact of influenza vaccination on seasonal mortality in the US elderly population. Arch Intern Med 2005; 165:265 - 72; http://dx.doi.org/10.1001/archinte.165.3.265; PMID: 15710788
- Falsey AR, Treanor JJ, Tornieporth N, Capellan J, Gorse GJ. Randomized, double-blind controlled phase 3 trial comparing the immunogenicity of high-dose and standard-dose influenza vaccine in adults 65 years of age and older. J Infect Dis 2009; 200:172 - 80; http://dx.doi.org/10.1086/599790; PMID: 19508159
- Holland D, Booy R, De Looze F, Eizenberg P, McDonald J, Karrasch J, et al. Intradermal influenza vaccine administered using a new microinjection system produces superior immunogenicity in elderly adults: a randomized controlled trial. J Infect Dis 2008; 198:650 - 8; http://dx.doi.org/10.1086/590434; PMID: 18652550
- Guy B. The perfect mix: recent progress in adjuvant research. Nat Rev Microbiol 2007; 5:505 - 17; http://dx.doi.org/10.1038/nrmicro1681; PMID: 17558426
- Dormitzer PR, Tsai TF, Del Giudice G. New technologies for influenza vaccines. Hum Vaccin Immunother 2012; 8:45 - 58; PMID: 22251994
- Coffman RL, Sher A, Seder RA. Vaccine adjuvants: putting innate immunity to work. Immunity 2010; 33:492 - 503; http://dx.doi.org/10.1016/j.immuni.2010.10.002; PMID: 21029960
- O’Hagan DT, De Gregorio E. The path to a successful vaccine adjuvant--‘the long and winding road’. Drug Discov Today 2009; 14:541 - 51; http://dx.doi.org/10.1016/j.drudis.2009.02.009; PMID: 19508916
- Ferguson M, Risi G, Davis M, Sheldon E, Baron M, Li P, et al. Safety and long-term humoral immune response in adults after vaccination with an H1N1 2009 pandemic influenza vaccine with or without AS03 adjuvant. J Infect Dis 2012; 205:733 - 44; http://dx.doi.org/10.1093/infdis/jir641; PMID: 22315336
- Jackson LA, Chen WH, Stapleton JT, Dekker CL, Wald A, Brady RC, et al. Immunogenicity and safety of varying dosages of a monovalent 2009 H1N1 influenza vaccine given with and without AS03 adjuvant system in healthy adults and older persons. J Infect Dis 2012; 206:811 - 20; http://dx.doi.org/10.1093/infdis/jis427; PMID: 22782949
- Vesikari T, Knuf M, Wutzler P, Karvonen A, Kieninger-Baum D, Schmitt HJ, et al. Oil-in-water emulsion adjuvant with influenza vaccine in young children. N Engl J Med 2011; 365:1406 - 16; http://dx.doi.org/10.1056/NEJMoa1010331; PMID: 21995388
- McElhaney JE. The unmet need in the elderly: designing new influenza vaccines for older adults. Vaccine 2005; 23:Suppl 1 S10 - 25; http://dx.doi.org/10.1016/j.vaccine.2005.04.019; PMID: 15908062
- Weinberger B, Herndler-Brandstetter D, Schwanninger A, Weiskopf D, Grubeck-Loebenstein B. Biology of immune responses to vaccines in elderly persons. Clin Infect Dis 2008; 46:1078 - 84; http://dx.doi.org/10.1086/529197; PMID: 18444828
- Treanor JJ, Campbell JD, Zangwill KM, Rowe T, Wolff M. Safety and immunogenicity of an inactivated subvirion influenza A (H5N1) vaccine. N Engl J Med 2006; 354:1343 - 51; http://dx.doi.org/10.1056/NEJMoa055778; PMID: 16571878
- Leroux-Roels G. Unmet needs in modern vaccinology: adjuvants to improve the immune response. Vaccine 2010; 28:Suppl 3 C25 - 36; http://dx.doi.org/10.1016/j.vaccine.2010.07.021; PMID: 20713254
- Lambert LC, Fauci AS. Influenza vaccines for the future. N Engl J Med 2010; 363:2036 - 44; http://dx.doi.org/10.1056/NEJMra1002842; PMID: 21083388
- Barrett PN, Mundt W, Kistner O, Howard MK. Vero cell platform in vaccine production: moving towards cell culture-based viral vaccines. Expert Rev Vaccines 2009; 8:607 - 18; http://dx.doi.org/10.1586/erv.09.19; PMID: 19397417
- Kistner O, Barrett PN, Mundt W, Reiter M, Schober-Bendixen S, Dorner F. Development of a mammalian cell (Vero) derived candidate influenza virus vaccine. Vaccine 1998; 16:960 - 8; http://dx.doi.org/10.1016/S0264-410X(97)00301-0; PMID: 9682344
- Kistner O, Barrett PN, Mundt W, Reiter M, Schober-Bendixen S, Eder G, et al. Development of a Vero cell-derived influenza whole virus vaccine. Dev Biol Stand 1999; 98:101 - 10, discussion 111; PMID: 10494963
- Hartikka J, Bozoukova V, Ferrari M, Sukhu L, Enas J, Sawdey M, et al. Vaxfectin enhances the humoral immune response to plasmid DNA-encoded antigens. Vaccine 2001; 19:1911 - 23; http://dx.doi.org/10.1016/S0264-410X(00)00445-X; PMID: 11228361
- Sullivan SM, Doukas J, Hartikka J, Smith L, Rolland A. Vaxfectin: a versatile adjuvant for plasmid DNA- and protein-based vaccines. Expert Opin Drug Deliv 2010; 7:1433 - 46; http://dx.doi.org/10.1517/17425247.2010.538047; PMID: 21118032
- Smith LR, Wloch MK, Ye M, Reyes LR, Boutsaboualoy S, Dunne CE, et al. Phase 1 clinical trials of the safety and immunogenicity of adjuvanted plasmid DNA vaccines encoding influenza A virus H5 hemagglutinin. Vaccine 2010; 28:2565 - 72; http://dx.doi.org/10.1016/j.vaccine.2010.01.029; PMID: 20117262
- Hartikka J, Bozoukova V, Yang CK, Ye M, Rusalov D, Shlapobersky M, et al. Vaxfectin, a cationic lipid-based adjuvant for protein-based influenza vaccines. Vaccine 2009; 27:6399 - 403; http://dx.doi.org/10.1016/j.vaccine.2009.06.014; PMID: 19552895
- Wright PF. Vaccine preparedness--are we ready for the next influenza pandemic?. N Engl J Med 2008; 358:2540 - 3; http://dx.doi.org/10.1056/NEJMp0803650; PMID: 18550873
- Barrett PN, Portsmouth D, Ehrlich HJ. Developing cell culture-derived pandemic vaccines. Curr Opin Mol Ther 2010; 12:21 - 30; PMID: 20140813
- Barrett PN, Berezuk G, Fritsch S, Aichinger G, Hart MK, El-Amin W, et al. Efficacy, safety, and immunogenicity of a Vero-cell-culture-derived trivalent influenza vaccine: a multicentre, double-blind, randomised, placebo-controlled trial. Lancet 2011; 377:751 - 9; http://dx.doi.org/10.1016/S0140-6736(10)62228-3; PMID: 21329971
- Ehrlich HJ, Berezuk G, Fritsch S, Aichinger G, Singer J, Portsmouth D, et al. Clinical development of a Vero cell culture-derived seasonal influenza vaccine. Vaccine 2012; 30:4377 - 86; http://dx.doi.org/10.1016/j.vaccine.2011.11.114; PMID: 22172502
- Ehrlich HJ, Singer J, Berezuk G, Fritsch S, Aichinger G, Hart MK, et al. A cell culture-derived influenza vaccine provides consistent protection against infection and reduces the duration and severity of disease in infected individuals. Clin Infect Dis 2012; 54:946 - 54; http://dx.doi.org/10.1093/cid/cir959; PMID: 22267715
- Ehrlich HJ, Müller M, Oh HM, Tambyah PA, Joukhadar C, Montomoli E, et al, Baxter H5N1 Pandemic Influenza Vaccine Clinical Study Team. A clinical trial of a whole-virus H5N1 vaccine derived from cell culture. N Engl J Med 2008; 358:2573 - 84; http://dx.doi.org/10.1056/NEJMoa073121; PMID: 18550874
- Lorbetskie B, Wang J, Gravel C, Allen C, Walsh M, Rinfret A, et al. Optimization and qualification of a quantitative reversed-phase HPLC method for hemagglutinin in influenza preparations and its comparative evaluation with biochemical assays. Vaccine 2011; 29:3377 - 89; http://dx.doi.org/10.1016/j.vaccine.2011.02.090; PMID: 21397719
- Rappuoli R, Dormitzer PR. Influenza: options to improve pandemic preparation. Science 2012; 336:1531 - 3; http://dx.doi.org/10.1126/science.1221466; PMID: 22723412
- Keitel WA, Atmar RL. Preparing for a possible pandemic: influenza A/H5N1 vaccine development. Curr Opin Pharmacol 2007; 7:484 - 90; http://dx.doi.org/10.1016/j.coph.2007.06.004; PMID: 17644429
- Garçon N, Vaughn DW, Didierlaurent AM. Development and evaluation of AS03, an Adjuvant System containing α-tocopherol and squalene in an oil-in-water emulsion. Expert Rev Vaccines 2012; 11:349 - 66; http://dx.doi.org/10.1586/erv.11.192; PMID: 22380826
- Levie K, Leroux-Roels I, Hoppenbrouwers K, Kervyn AD, Vandermeulen C, Forgus S, et al. An adjuvanted, low-dose, pandemic influenza A (H5N1) vaccine candidate is safe, immunogenic, and induces cross-reactive immune responses in healthy adults. J Infect Dis 2008; 198:642 - 9; http://dx.doi.org/10.1086/590913; PMID: 18576945
- O’Hagan DT. MF59 is a safe and potent vaccine adjuvant that enhances protection against influenza virus infection. Expert Rev Vaccines 2007; 6:699 - 710; http://dx.doi.org/10.1586/14760584.6.5.699; PMID: 17931151
- Nohynek H, Jokinen J, Partinen M, Vaarala O, Kirjavainen T, Sundman J, et al. AS03 adjuvanted AH1N1 vaccine associated with an abrupt increase in the incidence of childhood narcolepsy in Finland. PLoS One 2012; 7:e33536; http://dx.doi.org/10.1371/journal.pone.0033536; PMID: 22470453
- Barnier Quer C, Elsharkawy A, Romeijn S, Kros A, Jiskoot W. Cationic liposomes as adjuvants for influenza hemagglutinin: more than charge alone. Eur J Pharm Biopharm 2012; 81:294 - 302; http://dx.doi.org/10.1016/j.ejpb.2012.03.013; PMID: 22487055
- Even-Or O, Samira S, Rochlin E, Balasingam S, Mann AJ, Lambkin-Williams R, et al. Immunogenicity, protective efficacy and mechanism of novel CCS adjuvanted influenza vaccine. Vaccine 2010; 28:6527 - 41; http://dx.doi.org/10.1016/j.vaccine.2010.04.011; PMID: 20412874
- Rosenkrands I, Vingsbo-Lundberg C, Bundgaard TJ, Lindenstrøm T, Enouf V, van der Werf S, et al. Enhanced humoral and cell-mediated immune responses after immunization with trivalent influenza vaccine adjuvanted with cationic liposomes. Vaccine 2011; 29:6283 - 91; http://dx.doi.org/10.1016/j.vaccine.2011.06.040; PMID: 21722683
- Wack A, Baudner BC, Hilbert AK, Manini I, Nuti S, Tavarini S, et al. Combination adjuvants for the induction of potent, long-lasting antibody and T-cell responses to influenza vaccine in mice. Vaccine 2008; 26:552 - 61; http://dx.doi.org/10.1016/j.vaccine.2007.11.054; PMID: 18162266
- Mahnke YD, Saqr A, Hazenfeld S, Brady RC, Roederer M, Subbramanian RA. Age-related changes in durability and function of vaccine-elicited influenza-specific CD4(+) T-cell responses. Vaccine 2011; 29:8606 - 14; http://dx.doi.org/10.1016/j.vaccine.2011.09.019; PMID: 21939709
- McElhaney JE, Xie D, Hager WD, Barry MB, Wang Y, Kleppinger A, et al. T cell responses are better correlates of vaccine protection in the elderly. J Immunol 2006; 176:6333 - 9; PMID: 16670345
- Pedersen GK, Madhun AS, Breakwell L, Hoschler K, Sjursen H, Pathirana RD, et al. T-helper 1 cells elicited by H5N1 vaccination predict seroprotection. J Infect Dis 2012; 206:158 - 66; http://dx.doi.org/10.1093/infdis/jis330; PMID: 22551811
- Wilkinson TM, Li CK, Chui CS, Huang AK, Perkins M, Liebner JC, et al. Preexisting influenza-specific CD4+ T cells correlate with disease protection against influenza challenge in humans. Nat Med 2012; 18:274 - 80; http://dx.doi.org/10.1038/nm.2612; PMID: 22286307
- Doukas J, Morrow J, Bellinger D, Hilgert T, Martin T, Jones D, et al. Nonclinical biodistribution, integration, and toxicology evaluations of an H5N1 pandemic influenza plasmid DNA vaccine formulated with Vaxfectin®. Vaccine 2011; 29:5443 - 52; http://dx.doi.org/10.1016/j.vaccine.2011.05.060; PMID: 21641955
- Raviprakash K, Luke TC, Doukas J, Danko J, Porter K, Burgess T, et al. A dengue DNA vaccine formulated with Vaxfectin (®) is well tolerated, and elicits strong neutralizing antibody responses to all four dengue serotypes in New Zealand white rabbits. Hum Vaccin Immunother 2012; 8:8; PMID: 23032166
- Shlapobersky M, Wei Q, Sullivan S, Vilalta A. Vaxfectin-adjuvanted seasonal influenza protein vaccine: correlation of systemic and local immunological markers with formulation parameters. Vaccine 2009; 27:6404 - 10; http://dx.doi.org/10.1016/j.vaccine.2009.06.087; PMID: 19607952
- Kistner O, Howard MK, Spruth M, Wodal W, Brühl P, Gerencer M, et al. Cell culture (Vero) derived whole virus (H5N1) vaccine based on wild-type virus strain induces cross-protective immune responses. Vaccine 2007; 25:6028 - 36; http://dx.doi.org/10.1016/j.vaccine.2007.05.013; PMID: 17614165
- Howard MK, Kistner O, Barrett PN. Pre-clinical development of cell culture (Vero)-derived H5N1 pandemic vaccines. Biol Chem 2008; 389:569 - 77; http://dx.doi.org/10.1515/BC.2008.060; PMID: 18953724
- Wood JM, Schild GC, Newman RW, Seagroatt V. An improved single-radial-immunodiffusion technique for the assay of influenza haemagglutinin antigen: application for potency determinations of inactivated whole virus and subunit vaccines. J Biol Stand 1977; 5:237 - 47; http://dx.doi.org/10.1016/S0092-1157(77)80008-5; PMID: 408355
- Stephenson I, Wood JM, Nicholson KG, Charlett A, Zambon MC. Detection of anti-H5 responses in human sera by HI using horse erythrocytes following MF59-adjuvanted influenza A/Duck/Singapore/97 vaccine. Virus Res 2004; 103:91 - 5; http://dx.doi.org/10.1016/j.virusres.2004.02.019; PMID: 15163495