Abstract
Immunization with synthetic, preaggregated β-amyloid (Aβ) was the first treatment approach able to dramatically reduce brain Aβ pathology in Alzheimer’s disease (AD) animal models. For the development of a safe vaccine, we investigated whether 4Aβ1-15 (four tandem repeats of GPGPG-linked Aβ1-15 sequences) had therapeutic effects in the APP/PS1 transgenic mice model of AD. We described the production of anti-Aβ antibodies in APP/PS1 mice immunized with 4Aβ1-15 mixed with MF59 adjuvant. The anti-Aβ antibody concentrations were increased which bound to AD plaques, markedly reduced Aβ pathology in transgenic AD mice and levels of intracerebral Aβ (soluble and insoluble), whereas increased serum Aβ levels. Immunization via 4Aβ1-15 (mainly of the IgG1 Class) may induce a non-inflammatory Th2 reaction. Immunohistochemistry analysis of MHC Class II and CD45 revealed that microglial cells were in a less activated state. Of note, 4Aβ1-15-immunized mice showed improved acquisition of memory compared with controls in a reference-memory Morris water-maze behavior test. The data identify the novel immunogen 4Aβ1-15 as a promising new tool for AD immunotherapy.
Introduction
Amyloid plaques, deposits of the Aβ peptide, are defining lesions in Alzheimer’s disease brain. Many factors might cause the disease, but converging evidence indicates a central role of aggregated forms of Aβ peptide.Citation1,Citation2 The main species of senile plaques are Aβ40 and Aβ42. Aβ42 is more fibrillogenic than shorter Aβ peptides, and elevated Aβ42 concentrations are thought to drive the formation of the insoluble fibrils that compose amyloid plaques.Citation3 Although the exact mechanism of Aβ toxicity is unknown, interventions that block Aβ aggregation and deposition are thought to be promising therapeutic options. Immunotherapy accomplished by vaccination with Aβ peptide has proved efficacious in AD mouse models. Antibodies against Aβ are able to reduce amyloid load and improve cognition.Citation4 These results have raised the hope that Alzheimer’s disease could be treated by immunotherapy and prevented by vaccination. The AN1792 clinical trial was discontinued because meningoencephalitis occurred in 6% of the patient as a side effect. Adjuvant QS21 strongly induces Th1 lymphocytes, this vaccine design aimed to induce a strong cell-mediated immune response.Citation5 Thus, there is a continuing need for a therapy producing a mild antigen–antibody reaction, but do not have profound disease-modifying effects.Citation6 Several trials of active human immunization are underway. The antigenic profile of Aβ peptide modifications may to favor a humoral response reducing also the potential for a Th1-mediated response. This approach has been termed altered peptide ligands. The Aβ1-42 has one major antibody-binding site located on its N-terminus and two major T-cell epitopes located at the central and C-terminal hydrophobic regions encompassing residues 17-21 and 29-42, respectively.Citation7 There was also expression of the major histocompatibility complex (MHC) Class II molecule on some of the microglial cells after intravitreal injection of A42 that overproduction of Aβ induced neuro-degeneration.Citation8 Hence, elimination or modification of these sites provides a double gain by eliminating toxicity and the potential for T-cell stimulation.
Current strategies for disease modifications in AD include therapies that interfere with Aβ production, enhance its degradation or cause clearance from the central nervous system. Several active immunization strategies have been shown to dramatically prevent or reduce Aβ accumulation in the brains of APP transgenic miceCitation9,Citation10 and to protect transgenic mice from cognitive impairment. Subsequent studies have proved that both B- and T-cell epitopes are located in aggregated Aβ42. Aβ1-15 or other shorter N-terminal fragments have been identified as the B-cell epitope-bearing fragments.Citation11 In order to reduce the risk of an adverse T cell-mediated immune response and enhance the humoral immune response, we develop a vaccine composed of tandem repeats of Aβ1-15. The 4Aβ1-15 also has the advantage of increasing the number of epitopes to enhance its immunogenicity and the molecular weight as well as reducing degradation of the short single Aβ1-15 peptides. Modified 4Aβ1-15 plus MF59 (the adjuvant approved for human useCitation12 that promoted Aβ-specific antibody production). If communization begins early, Aβ-lowering might prevent formation of neurofibrillary tangles (NFTs), which seems to be a result of Aβ-related toxicity,Citation13 and thus vaccination could provide better cognitive benefits than it has in trials to date. In transgenic mice, antibodies cleared both Aβ and early, but not late, forms of hyperphosphorylated tau aggregations.Citation14 Therefore, Aβ immunotherapy could prevent formation of new tangles without affecting numbers or morphology of those already formed. Aβ antibodies were produced when the vaccine were administered to APP/PS1 mice, successfully reducing Aβ accumulation in their brains.
In this study, to determine whether 4Aβ1-15 has therapeutic effects in AD, APP/PS1 mice were immunized subcutaneously. Amounts of Aβ in the APP/PS1 mice were measured by sandwich enzyme-linked immunosorbent assay, and the effects were assessed by improvement in memory using a Morris water maze test. In this report immunization with 4Aβ1-15 resulted in significant decreases in cerebral Aβ40 and Aβ42 levels coincident with increased circulating levels of Aβ40 and Aβ42, suggesting brain-to-blood efflux of Aβ associated with Aβ plaque clearance and a decrease in the level of microglial activation in the brain.
Results
Anti-Aβ antibody concentration in APP/PS1 mice
To determine whether 4Aβ1-15 vaccination was effective in a mouse model of AD, we immunized APP/PS1 mice subcutaneously. Mice were immunized biweekly over 16 weeks. Blood samples were taken at weeks 0, 5, 9, 13 and 17. Serum Aβ antibody concentrations were measured by ELISA. Aβ antibodies were first detected at week 5 in all immunized mice and dramatically increased thereafter (*#P < 0.001) in 4Aβ1-15 and Aβ42 treated mice. Thus, the 4Aβ1-15 epitope vaccine was at least as effective as Aβ42 at initiating the antibody immune responses, (). Of note, transgenic mice immunized with PBS plus MF59 adjuvant alone in parallel did not induce anti-Aβ antibodies in these animals, confirming the specificity of our titer assay.
Figure 1. Generation of immune responses in APP/PS1 mice immunized with 4Aβ1-15 peptide plus MF59. (A) Aβ antibody titers were measured by ELISA. Data are presented as mean ± SD of Aβ antibodies (μg/ml). One-way ANOVA followed by post hoc comparison revealed significant differences in anti-Aβ titers of 4Aβ1-15-immunized group when comparing week 9 to weeks 13 or 17 (n = 9, *P <0.01). The same trend was observed in human Aβ42-immunized group (n = 9, #P < 0.01). (B) Detection of IgG1, IgG2a and IgG2b subclasses of anti-Aβ antibodies in mice immunized with the vaccines. Isotyping in sera from immunized mice after the final immunization. (C) The results revealed significant differences of the ratio of IgG1 and IgG2a between 4Aβ1-15 vs. Aβ42-immunized group at each week shown (n = 9, *P <0.01). (D) Lymphocytes from APP/PS1 mice immunized with Aβ42 or 4Aβ1-15 were individually isolated and cultured then stimulated with ConA (5 μg/ml), 4Aβ1-15 or Aβ42 (20 μg/ml) for 36 h. The number of IL-4 and IFN-γ positive T cells in immunized mice detected by ELISPOT. Data were presented as mean ± SD of each cytokine. There was no significant difference between vaccine-treated groups for levels of each cytokines after in vitro ConA challenge. However, there was a significant difference between groups in cytokine levels of IL-4 and IFN-γ after 4Aβ1-15 or Aβ42 challenge.
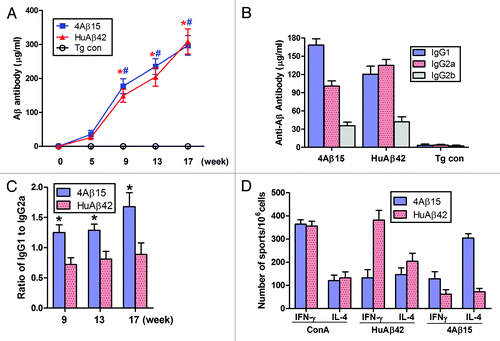
We next characterized the types of humoral immune responses from each vaccination group, anti-Aβ antibody isotypes IgG1 for Th2 reactions and IgG2a for Th1 reactions were measured by ELISA. Immunoglobulin isotype-specific ELISA suggested that immunization with 4Aβ1-15 and Aβ42 induced a broad spectrum of anti-Aβ antibody isotypes (IgG1, IgG2a and IgG2b), (). The pattern of antibody isotypes induced upon 4Aβ1-15 immunization showed that IgG1 was the predominant isotype with relatively lower titers of IgG2a and IgG2b. Different results were found in mice immunized with Aβ42; IgG2a was the predominant Ig isotype, lower levels of IgG1 and IgG2b were detected. The IgG1/IgG2a ratio for the 4Aβ1-15 group (1.68 ± 0.23) was approximately two times greater than that for the Aβ42 group (0.89 ± 0.19) at 17th week, (). In addition, low amounts of IgG3, IgM and IgA were detected in both groups (data not shown).
Aβ-specific immune responses in lymphocytes
To further investigate T-cell responses to vaccination, we analyzed Th1 (IFNγ) and Th2 (IL-4) cytokines production by lymphocytes from immunized APP/PS1 Tg mice using ELISPOT assay (). PBS treated mice exhibited background levels of IL-4 and IFN-γ in the presence or absence of 4Aβ1-15 and Aβ42 peptide in the cultures of lymphocytes. Nonspecific mitogenic stimulation of cultured lymphocytes with ConA resulted in high levels of IFN- and IL-4 production. No statistically significant difference was noted between 4Aβ1-15 and human Aβ42 groups for each cytokine after restimulated with ConA (P > 0.05). Recall stimulation of lymphocytes from 4Aβ1-15 immunized mice resulted in significantly increased production of IL-4 compared with lymphocytes cultured from mice immunized with Aβ42, whereas less IFN- γ production was observed. Groups of mice immunized with Aβ42 exhibited significantly higher IFN-γ responses in splenocytes stimulated with Aβ42. Higher levels of IL-4 and IFN-γ were only observed in splenocytes that were restimulated with the corresponding immunogens. Obviously, mice injected with the 4Aβ1-15 showed a Th2 (IL-4) response on the basis of the number of lymphocytes producing this lymphokine, whereas mice immunized with Aβ42 showed a Th1 (IFN-γ) response.
Immunization with 4Aβ1-15 changed the circulating and intracerebral Aβ levels
Immunization of the transgenic APP/PS1 mice were started at 4 month of age in this study, which overproduced human Aβ and develop significant amyloid deposits by 8 month of age. We quantified Aβ levels in the sera by ELISA and found significantly increased circulating Aβ40 and Aβ42 in APP/PS1 mice immunized with 4Aβ1-15 as early as 5 weeks after immunization, (). Importantly, serum Aβ40 level increased rapidly to the highest values of 927 ± 65 pg/ml by week 9, and serum Aβ42 level reached the highest values of 164 ± 25 pg/ml by week 17. Instead, there were no significant differences between serum Aβ levels of 4Aβ1-15 and human Aβ42 vaccinated groups at each time point. More specifically, detergent-soluble Aβ40 and Aβ42 levels were reduced by 54% and 50%, respectively, (). Insoluble Aβ40 and Aβ42 (prepared by detergent-insoluble material in 5 M guanidine) levels were reduced by 51% and 47%, respectively, in 4Aβ1-15-immunized APP/PS1 mice, (). The same results were observed in brains of mice immunized with human Aβ42, significantly less Aβ42 and Aβ40 levels were detected in Aβ42-immunized animals.
Figure 2. Aβ levels in APP/PS1 mice. For Aβ analysis, blood samples were individually collected from 4Aβ1-15, Aβ42 or PBS immunized APP/PS1 mice at the time points indicated. (A) Serum Aβ40 (B) Aβ42 peptides were measured separately by Aβ ELISA. Data were presented as mean ± SD of Aβ40 or Aβ42 (pg/ml). The Aβ levels of both 4Aβ15 (n = 9, *P< 0.01) and Aβ42-treated (n = 9, #P <0.01) group were increased compared with the control group. Detergent-soluble Aβ40, Aβ42 peptides (C) and insoluble Aβ40, Aβ42 prepared from 5M guanidine extraction (D) were separately measured in brain homogenates by ELISA. Data were presented as mean ± SD of Aβ40 or Aβ42 (pg/mg protein), and reductions for each group are indicated.
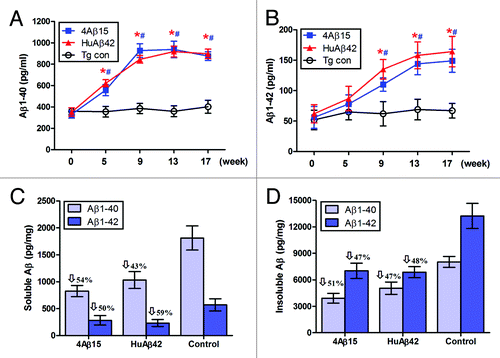
In order to understand the relationships between anti-Aβ antibody concentration and intracerebral soluble and Tris-insoluble Aβ42 levels, we evaluated them on a mouse-by-mouse basis, (). Although there was a significant inverse correlation between anti-Aβ antibody concentration and level of soluble Aβ42 (r = −0.832, p < 0.0001), there was no significant correlation between anti-Aβ antibody concentration and level of insoluble Aβ42 (r = −0.472, p = 0.0478). To determine whether the serum increase in Aβ40 and Aβ42 was associated with a reduction in cerebral Aβ levels, we evaluated the relationship between serum Aβ level and intracerebral soluble and Tris-insoluble Aβ42 levels, (). Serum Aβ level was significantly correlated with intracerebral soluble (r = 0.649, p = 0.0035) and Tris-insoluble (r = 0.868, p < 0.0001) Aβ42 levels.
Figure 3. Correlation of Aβ levels between sera and brains. (A) A significant inverse correlation between Anti-Aβ antibody concentration and brain-soluble Aβ levels was revealed (r = −0.832, p < 0.0001). (B) Anti-Aβ antibody concentration tended to correlate with the Tris-insoluble Aβ42 level (r = −0.472, p = 0.0478). (C) Serum Aβ42 level correlates with the brain soluble Aβ42 level (r = 0.649, p = 0.0035). (D) Serum Aβ42 level also correlates with the brain Tris-insoluble Aβ42 level (r = 0.868, p < 0.0001).
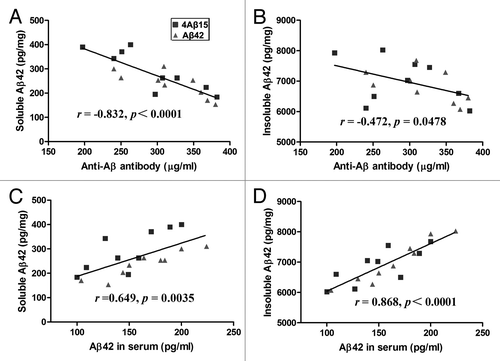
4Aβ1-15 immunization significantly improved memory deficits in AD model mice
The Morris water maze was used to determine the effect of 4Aβ15 on spatial memory starting at the age of 8.5 month after the mice received 4 mo vaccination. Meanwhile nine 8.5-month-old wild-type C57BL mice (five male and four female) as positive control (WT) were also be tested. In the visible platform tests, 4Aβ1-15, human Aβ42-treated, APP/PS1 Tg and WT mice had similar escape latency (p > 0.05; ) and path length (p > 0.05; ), which indicated that 4Aβ1-15 or Aβ42-treatment did not affect mouse motility or vision. In the hidden platform-swimming test, APP/PS1 mice injected with 4Aβ1-15 and Aβ42 showed significant improvements compared with the PBS-treated controls. The escape latency on the third and fourth day of the hidden platform test was shorter than non-treated APP/PS1 mice (*#p < 0.01; ). The epitope vaccine treated mice were able to swim significantly shorter distances to reach the platform compared with control mice on the third and fourth day (*#p < 0.01; ). In the probe trial on the last day of testing, the platform was removed. 4Aβ15 vaccination significantly improved the AD mouse’s spatial memory. The number of times the mice traveled into the third quadrant, where the hidden platform was previously placed, was significantly greater with 4Aβ1-15 treatment compared with control (*p < 0.01; ). These results strongly support that 4Aβ1-15 vaccination significantly improves the memory deficits seen in APP/PS1 mice. There were no significant differences in the escape latency and path length in the hidden platform trial of the Morris water maze test between 4Aβ1-15 and Aβ42 treatment groups (p > 0.05).
Figure 4. 4Aβ15 improves memory deficits in AD transgenic mice. A Morris water maze test consists of 1 d of visible platform tests and 4 d of hidden platform tests, plus a probe trial 24 h after the last hidden platform test. Animal movement was tracked and recorded. The 8.5-month APP/PS1 group mice and the same age wildtype group mice (WT) were tested. (A) During the first day of visible platform tests, both the 4Aβ1-15-treated, control APP/PS1 mice (Tg con) and WT mice exhibited a similar latency to escape onto the visible platform (n = 9, p > 0.05 by Student’s t-test). (B) Four group of treated and control mice had similar swimming distances before escaping onto the visible platform in the visible platform test (n = 9, p > 0.05 by Student’s t-test). (C) In hidden platform tests, mice were trained with 6 trials per day for 4 d. 4Aβ1-15-treated APP/PS1 mice showed a shorter latency to escape onto the hidden platform on the third and fourth day (n = 9, *#p < 0.01 by ANOVA). (D) The 4Aβ1-15-treated APP/PS1 mice had a shorter swimming length before escaping onto the hidden platform on the third and fourth day (n = 9, *#p < 0.01 by ANOVA). (E) In the probe trial on the sixth day, the 4Aβ15-treated mice traveled into the third quadrant, where the hidden platform was previously placed, significantly more times than controls (n = 9, *p < 0.01 by Student’s t-test).
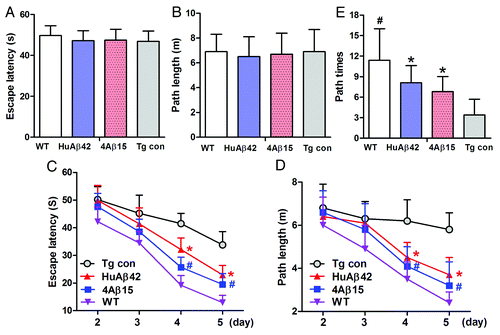
Immunized with 4Aβ1-15 reduced cerebral amyloidosis
The neuropathological changes analysis of cerebral amyloidosis provided further evidence of the therapeutic efficacy of anti-Aβ antibodies generated in response to the peptide epitope vaccine. To demonstrate, we further analyzed Aβ plaques in brains of experimental and control APP/PS1 mice by 4G8 immnunohistochemistry and thioflavin T histochemistry (after 8 injections). A significant decrease in cortical plaque burden in APP/PS1 mice immunized with the epitope vaccine () compared with the control adjuvant-only injected group (). Additionally, we demonstrated significant reduction of ThT-positive Aβ deposits in the brains of experimental mice () vs. controls ().
Figure 5. Reduction of cerebral Aβ pathology in APP/PS1 mice immunized with 4Aβ1-15. (A, B, E and F) The hippocampus. (C, D, G and H) The parietal cortex. (left) PBS-immunized APP/PS1 mice. (right) 4Aβ1-15-immunized APP/PS1 mice. (A–D) Mouse brain coronal sections were stained with monoclonal anti-human Aβ antibody 4G8. (I) Percentages (plaque burden, area plaque/total area) of Aβ antibody-immunoreactive Aβ plaques were calculated by quantitative image analysis and reductions for each mouse brain area analyzed are indicated. (E–H) Mouse brain sections from the indicated regions were stained with thioflavin T. (J) Percentages of thioflavin T-stained plaques were quantified by image analysis, and reductions for each brain region are indicated (n = 9, #p < 0.01). (Scale bar: 200 μm.)
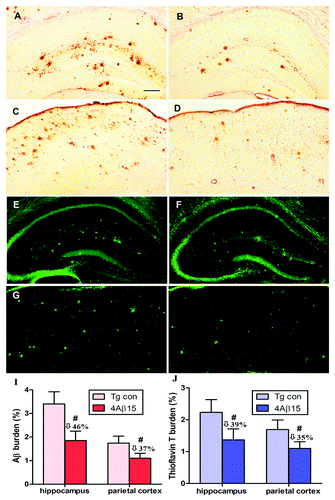
Quantitative image analysis of anti-Aβ mono-antibody immunoreactive Aβ deposits revealed that there was 46% reduction in hippocampus region and 37% reduction in parietal cortex brain region in 4Aβ-15-immunized vs. control mice, (). We examined 39% and 35% reductions of ThT-positive Aβ deposits, respectively, across hippocampal and parietal cortex brain regions (). Together, these results provide further evidence that that immunization with 4Aβ-15 epitope vaccine is effective in reducing cerebral amyloidosis in APP/PS1 mice.
Effect of vaccination on microglial activation
The same brain regions used for Aβ burden studies were evaluated for microglial activation. Representative immunoreactivity of MHC Class II (IA/IE) positive cells (red) demonstrated significantly decreased microglial activation in hippocampal brain regions and the cortical region of APP/PS1 mice vaccinated with the 4Aβ1-15 epitope vaccine () compared with that in control mice (). These data are similar to the results obtained after immunostaining with anti-CD45 antibody (green) (). The quantitative image analyses indicated that vaccinated mice had a lesser degree of microglial activation compared with the control group and this decrease is statistically significant, ().
Figure 6. 4Aβ1-15 epitope vaccine reduces microglial activation. (A, B, E and F) The hippocampus. (C, D, G and H) The parietal cortex. (left) PBS-immunized APP/PS1 mice. (right) 4Aβ1-15-immunized APP/PS1 mice. Image analysis of cortex areas from vaccinated or control mice were performed after staining with anti-I-A/I-E antibody (red) or anti-CD45 antibody (green). Vaccination with the epitope vaccine decreased microglial activation in hippocampal brain regions of immune mice compared with that in control mice. Representative pictures of brain sections stained with each antibody showing a decreased immunoreactivity also in the cortical region in mice immunized with the epitope vaccine compared with control animals. (I and J) The quantitative image analyses indicated that vaccinated mice had a lesser degree of microglia activation compared with the control group (n = 9, #p < 0.01). (Scale bar: 200 μm.)
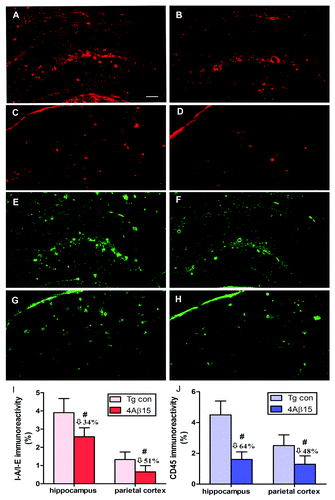
Discussion
Recent advances in our understanding of the physiopathological mechanisms of AD have led to the development of putative disease-modifying treatments.Citation15 During the last decade, most of the efforts of the pharmaceutical industry were directed against the production and accumulation of Aβ. The most innovative of the pharmacological approaches was the stimulation of Aβ clearance from the brain of AD patients via the administration of Aβ antigens (active vaccination) or anti-Aβ antibodies (passive vaccination).Citation14 It has been suggested that active vaccination could be more effective is started before the development of clinically significant AD-related pathology. Compared with passive immunotherapy, active immunotherapy offers long-term advantages owing to the continuous production and affinity maturation of therapeutic antibodies over time and to the need for less frequent administration.Citation16 Many novel therapeutic strategies are being developed, with active immunization being among the most advanced approaches.Citation17 The type of immune response generated can be characterized by determining the isotype of the generated antibodies. The subclass of IgG induced is an indirect indication of the cytokines generated by the stimulated T helper cells. Production of IgG1 is in mice induced by IL-4 and IL-5 secreted by activated Th2 cells while IgG2a is induced by IL-2 and IFN-γ secreted by Th1 cellsCitation18 and the Th2 response is characterized as non-inflammatory. Aβ immunization appears to modulate immune responses based on three major criteria:Citation19 tissue route of delivery, antigen epitope used for immunization and properties of the co-administered adjuvant. Recently, subcutaneous injections with a novel active Aβ immunotherapy, CAD106 (Aβ1-6 coupled to a carrier) were done to investigate the safety, tolerability and Aβ-specific antibody response in patients with mild to moderate Alzheimer’s disease.Citation20 The subcutaneous immunization delivery route was safe and suitable to the patients.
Here we describe our newly developed Aβ epitope vaccine for AD. The majority of anti-Aβ antibodies generated in animals and humans recognize an epitope located within the N-terminus of Aβ protein (Aβ-15), whereas the T-cell epitope has been mapped to within Aβ15-42. These observations have been used to design alternative immunogens. Using Aβ1-15 as the primary immunogen for intranasal immunization did not initiate strong antibody production.Citation21 Therefore duplicate, triplicate even quadruplicate tandem Aβ1-15 repeat peptides were chosen be the candidate vaccines. The repeated copies of the antigen may overcome immunological tolerance, therefore in the present study, 4Aβ1-15 might relatively overcome the immune hyporesponsiveness to Aβ in Tg mice. The immune hyporesponsiveness could be overcome if Aβ B cell epitope coupled to other T-cell epitopes. Furthermore, the new T-cell epitopes produced among four Aβ1-15 fragments might replace the T-cell epitopes of Aβ42.
Among various possible spacers, GPGPG was selected for two reasons. First, GPGPG extensions around the core binding region greatly decrease binding affinity, thus suggesting that GPGPG-containing epitopes would bind efficiently. Second, the GPGPG spacer was selected because regions rich in G and P are known to be associated with β turns. The presence of this spacer at 15–20 residue intervals might help create some secondary and possibly tertiary structure, thereby facilitating Ag expression and potential purification.Citation22
In this study, we used MF59 as the adjuvant and found that it was an effective adjuvant that effectively induced an immunological reaction against Aβ. MF59 is a safe and effective vaccine adjuvant, which was originally approved to be included in a licensed influenza vaccine. We used human Aβ42 as a positive control vaccine compared with 4Aβ1-15 immunogen, Aβ42 active vaccination has previously been shown to produce anti-Aβ antibody titers and reduce amyloid deposition in transgenic mice.Citation19 It has also been shown to attenuate memory loss in APP/PS1 transgenic mice. We have also shown that there appears to be a dependence on microglial activation for the removal amyloid deposits following active vaccination. To confirm the potential therapeutic efficacy of 4Aβ1-15 immunization, 4-mo-old transgenic APP/PS1 mice were immunized against 4Aβ1-15, human Aβ42 or MF59 alone for 16 weeks. Results showed the serum anti-Aβ antibody titer was significantly elevated in mice administered the Aβ vaccines compared with controls throughout the 16 weeks immunization period. We chose APPswe/PS1-dE9 double transgenic animals, which display characteristic compact, dense-cored, deposits throughout the cortex and hippocampus, which could be stained with ThT. The use of ThT is widespread in structural and mechanistic studies of amyloid formation. Changes in its intensity are used not only as a qualitative indicator of amyloid but also as a quantitative measure of amyloid formation.Citation23,Citation24 Previously it was demonstrated that Aβ neurotoxicity requires insoluble fibril formation. The senile plaques are the result of misprocessing of the amyloid precursor protein, a type-1 transmembrane protein, by β- and γ-secretases to form a toxic β-amyloid peptide of 40-42 amino acids.Citation25 A striking problem with active immunization aimed at just the removal or prevention of parenchymal amyloid deposition is the autopsy data from the human trial.Citation26 Here, we investigated the potential of Aβ immunization for the treatment of AD-like cerebral amyloidosis in transgenic mice immunization. Aβ deposition could simply represent a host response to an upstream pathophysiologic process or serving a protective functionCitation27 likely as a metal chelator.Citation28 Our immunization methods successfully decreased the burden of intracerebral Aβ42 and senile plaques as measured via immunological staining. CD45 immunohistochemistry reveals microglial cells in an activated state and microglial CD45 abundance is increased in brains of AD patients and in mouse models of the disease. It is known that Aβ is able to cause microglial chemotaxis that is mediated by the release of soluble factors from the activated astrocytes, such as transforming growth factor β. Overexpression of human APP in mice and subsequent amyloid deposition causes proliferation of resident microglial cells. A proportion of these activated microglia expressed the MHC II molecule and are able to process and present antigens. The amyloid clearance in most cases was in association with microglia that showed Aβ immunoreactivity, suggesting phagocytosis. Given the presence of these Aβ antibodies in the brain, it is possible that additional Aβ clearance mechanisms are operating, such as mediated by the Fc receptor on phagocytic microglia.Citation29
Cytokine dysregulation in AD could explain HLA DR overexpression, since MHC Class II expression can be induced on neutrophils by combined stimulation with interferon-γ and IL-3.Citation26,Citation30 Furthermore, amyloid reduction was accompanied by increased expression of the subunits of the immunoproteasome in microglial and inflammatory cells surrounding collapsed plaques. Release of antigenic peptides derived from -amyloid processing may enhance T-cell inflammatory responses accounting for the meningoencephalitis following a peptide immunization.Citation31
Together, these results indicate that vaccination of 4Aβ1-15 is not only effective at inducing novel anti-Aβ antibody production, but also reduces intracerebral Aβ. Furthermore, in support of peripheral sink hypothesis,Citation32 we found a significant correlation between the anti-Aβ antibody titer and the level of soluble, intracerebral Aβ42. To determine the safety of vaccination, we investigated the IgG isotypes, markers of inflammation, of the anti-Aβ antibodies found in the serum of vaccinated mice. Antibody isotype characterization demonstrated a predominantly IgG1 (IgG1 > IgG2a > IgG2b) response in Tg mice compared with controls. To confirm specific systemic vs. local immune cell activation, we analyzed primary cultures of isolated lymphocytes from immunized mice and found that 4Aβ1-15 immunization conferred Aβ-specific T-cell response as measured by secretion of cytokines IFN- and IL-4 upon aggregated Aβ42 peptide recall challenge. Importantly, there was a marked increase in IL-4 secretion compared with IFN-γ, further suggesting Th2 immune responses after 4Aβ1-15 immunization. It has been suggested that Aβ immunotherapy may either prevent further amyloid accumulation or remove existing deposits. The current study is inconclusive with regard to this issue.
These side effects were seen in vaccinated AD patients and not in the transgenic AD mice immunized with the vaccine candidate AN1792. This shows that results obtained in animal models are not easily translated to humans. New active vaccines are being engineered to drive Th2 or Th3 responses or stimulate innate immunity. Furthermore, a balance must be achieved between effective prevention and clearance of amyloid deposits and the induction of autoimmunity.
Conclusion
In summary, we have shown here an amyloid-lowering therapy administered with 4Aβ1-15 and MF59 adjuvant together does improve APP/PS1 Tg mice cognitive ability. The 4Aβ1-15 vaccination preferentially induced a Th2 response. Our results together with reports from other researchers, demonstrate that Aβ1-15 immunogens with spacer GPGPG is promising candidate for active immunization vaccine. These data established here should increase concern toward the development of an epitope vaccine leading to an effective immunotherapy for AD.
Materials and Methods
Animals
All APP/PS1 mice we used were obtained from a 5th generation cross between heterozygous APP K670N/M671L and heterozygous PS1 transgenic mice (line 6.2 bearing the M146L mutation).Citation16 The backgrounds of all offspring were an identical mix of C57/B6/SJL/Swiss Webster. Mice were genotyped and singly housed prior to the beginning of the study. Throughout the study-period, animals were housed in a controlled environment (temperature 22 °C, humidity 50-60%, 12-h light/12-h dark schedules), with free access to rodent chow and water, and their experiments were performed in accordance with our institutional guidelines.
Peptide synthesis and immunization protocol
The peptides used in our studies, human Aβ42, 4Aβ1-15 were synthesized by GenScript Co., Ltd., China. The purity (greater than 95%) of the peptides was substantiated by mass spectrometry and composition analysis. 4Aβ1-15 peptide is a 4-fold repeat of the Aβ1-15 peptide bearing an interposed five-amino-acid linker GPGPG. The peptides were diluted in distilled water at 4 mg/ml. The APP/PS1 mice were randomly assigned to three groups (n = 9 for each group, five male and four female), and vaccination was begun at 4.1 mo of age. MF59 (Novartis) was used as adjuvant. The three groups are 4Aβ1-15-immunized group, human Aβ42-immunized group and the transgenic mice vaccinated with PBS plus MF59 served as negative control (Tg con) group. The mice were immunized with human Aβ42 or 4Aβ1-15 subcutaneously. The peptides were mixed with MF59 and administered at 50 μg/100 L/body. Immunization was performed eight times at 2-week intervals. One week after each vaccination, blood was regularly drawn and was utilized for hematological and biochemical examinations.
Detection of anti-Aβ42 antibodies
Aβ antibodies in mouse serum were measured as described.Citation17 Plates were coated with Aβ42 dissolved in 0.15 M ammonium hydroxide solution and then washed five times with wash buffer. Next, the plates were blocked with 1% bovine serum albumin 60 min at room temperature and washed four times. Blood serum samples were diluted 10–105-fold. The 6E10 monoclonal anti-Aβ antibody was diluted 10,000–160,000-fold and used as a positive control. Each sample as applied to a well and incubated at 37 °C for 1 h. After washing six times, each well was blocked at room temperature for 30 min with 1% bovine serum albumin and then washed five times. Wells were incubated with an HRP-conjugated secondary antibody at 37 °C for 1 h and washed 10 times. They were next incubated with tetramethylbenzidine (TMB) substrate at room temperature in the dark. After sufficient color development had occurred, 2M phosphate buffer was added to stop the reaction. The absorbance of each well at 450 nm was measured with a spectrophotometer. Aβ antibodies were represented as ng per ml of serum. Sera from the vaccination were utilized for isotype determination. We measured the absorbance at 450 nm with a spectrophotometer and calculated antibody titers using the statistics software, PRISM version 5. The ratios of IgG1 to IgG2a were calculated for each time point from each mouse individually by using optical density values and then the average ratio for each group.
Production of cytokines by immune lymphocytes
Presence and type of Aβ specific T cells was analyzed by ELISPOT assay. Production of proinflammatory lymphokine IFN-γ (Th1) or anti-inflammatory IL-4 (Th2) was evaluated by restimulation of lymphocytes from experimental mice. Briefly, 96-well ELISPOT plates (BD PharMingen) were coated with capture IFN- or IL-4 specific antibodies. Lymphocytes from individual animals were added in tetraplicate wells (2 × 106 cells/well) and were restimulated with ConA (5 μg/ml), 4Aβ1-15 or Aβ42 (20 μg/ml), or left without restimulation in a culture medium only. After incubation for 36 h (37 °C, 5% CO2), cytokines were detected with biotinylated detection antibodies, followed by avidin-HRP. Substrate AEC (Sigma-Aldrich) was added to develop the reaction. Spots representing cytokine-producing cells were counted using dissecting microscope (Olympus) by three independent investigators. Averaged data were presented as number of cytokine secreting cells per 1 × 106 lymphocytes after restimulation with ConA, 4Aβ1-15 or Aβ42 peptides.
Quantification analysis of Aβ levels in APP/PS1 mouse brains
Biochemical analysis of the brain tissue was processed as described previously.Citation18 Briefly, frozen cortices were thawed, minced and then homogenized in 50 mM TRIS-HCl buffer containing 2% SDS, pH 8.0 and a mixture of protease inhibitors (Sigma). Homogenates were centrifuged (100,000 × g, 1 h, 4 °C) and supernatants were stored at −70°C for additional analysis of soluble Aβ. After we sonicated the pellets in 5 M guanidine–Tris buffer, the samples were incubated for 30 min at room temperature and then centrifuged (100,000 × g, 1 h, 4 °C) and supernatants were stored for analysis of insoluble Aβ. Concentrations of insoluble and soluble Aβ40 and Aβ42 were analyzed using Aβ ELISA kits (Invitrogen) according to the manufacturer’s recommendations. Plates were analyzed spectrophotometrically at 450 nm via a microplate reader, and the concentrations of Aβ40 and Aβ42 were calculated using standard curves by comparing the sample’s absorbance with the absorbance of known concentrations of a standard. Graph-Pad Prism4 curve fitting software was used to generate the standard curve and calculate Aβ concentration in the samples. Using the wet weight of hippocampus or cortex region in the original homogenate, the final values of Aβ were expressed as micrograms per gram wet weight of each region.
Immunohistochemistry and immunofluorescence staining
To analyze the effect of active immunization, with the epitope vaccine on neuropathological changes in APP/PS1mice, the brains were processed for immunohistochemistry by previously published methods.Citation14 Two weeks after the final vaccination, treated and control mice were sacrificed under deep anesthesia. To ensure proper fixation and immunostaining of brain tissues, mice were exsanguinated by transcardial perfusion with normal saline. Then brains were removed and bisected in midsagittal plane. The right hemisphere was snap frozen for biochemical analysis, whereas the left hemisphere was fixed in 4% paraformaldehyde for immunohistochemical analysis. Forty-micrometer-thick free-floating coronal sections of fixed hemibrains were collected using a vibratome. To assess the extent of neuropathology that occurs in the brains of mice, the following primary antibody was used. Aβ deposits were detected with anti-Aβ42 (dilution, 1:2000; Invitrogen). Sections were then washed and incubated with appropriate biotinylated secondary antibodies (1 h at room temperature). After multiple washes, the tissues were incubated in ABC for 1 h, and color development was performed using DAB (3, 3′-diaminobenzidine) substrate. Sections were mounted on slides. Activated microglia was detected with the anti-CD45 (1:300; Serotec) antibody and anti-I-A/I-E antibody [marker of major histocompatibility complex (MHC) II alloantigens; 1:200; BD PharMingen]. The tissues from all animals within a given experimental group were processed in parallel. Hydrogen peroxide-quenched and blocked sections were incubated with primary antibody overnight at 4 °C, followed by appropriate secondary antibody conjugated with Alexa-Fluor488 (1:400) for 1 h. Sections were then washed three times in PBS and mounted with fluorescence mounting media. Aβ deposits were visualized using Thioflavin T (ThT). Briefly, mouse brain sections were washed with Tris buffer and stained for 10 min with a solution of 0.5% ThT in 50% ethanol. Finally, sections were washed in 50% ethanol and Tris buffer, then dried and covered using Vectashield (Vector Laboratories). All experiments were performed in triplicate.
Quantitative image analysis
For quantitative image analysisCitation33 of Aβ plaque and glial reactivity, six 8-μm coronal sections at 40-μm intervals each mouse were immunostained to assess plaque load. Dorsal hippocampus and cerebral cortex (parietal association cortex) were chosen for quantitative analysis, and Aβ burden was assessed using Leica image analysis software interfaced with a Leica microscope and a CCD video camera. The brain area (dorsal hippocampus and cerebral cortex) was outlined using the edit plane function, and the area of the plaques and the brain region outlined were recorded. Hippocampal measurements were performed by circumscribing the entire hippocampus. The cortical area analyzed was dorsomedial from the cingulate cortex and extended ventrolaterally to the rhinal fissure within the right hemisphere. The quantitative analysis was performed at 5× magnification. Data were pooled for all six sections. The percentage of brain region covered by Aβ immunoreaction was used to measure Aβ burden. ThT-positive plaques were counted by visual inspection of cortical region of all stained sections although blind with respect to treatment condition; a mean semiquantitative score was independently determined for each slide by two observers.
Morris water maze test
Behavioral testing was performed during the light period, by a researcher blind to animal or treatment. The water maze test was performed in a 1.0-m-diameter pool. The mice trained to find the submerged platform in a 1.0-m-diameter pool of white, non-toxic colored water (temperature: 23 °C). The procedureCitation34 consisted of 1 d of visible platform tests and 4 d of hidden platform tests, plus a probe trial 24 h after the last hidden platform test. In the visible platform test, mice were tested for 5 contiguous trials, with an intertrial interval of 30 min. In the hidden platform tests, mice were trained for 6 trials, with an intertrial interval of 1 h. The movements of the animal in the maze were recorded by a video camera suspended 2.5 min above the center of the maze and connected to a video tracking system (Academia Sinica) and analyzed with a computer. The data were analyzed by two-way ANOVA.
Acknowledgments
This study was supported by the National Natural Science Foundation of China (No. 81000545, No. 81071033); Technology Project of Guangdong Province (No. 0911220600567); Technology Project of Guangzhou City (No. 10C32060117, No. 1100567); Fundamental Research Funds for the Central Universities. We thank technician Qunfang Yuan for her helpful suggestions.
Disclosure of Potential Conflicts of Interest
No potential conflicts of interest were disclosed.
References
- Jin M, Shepardson N, Yang T, Chen G, Walsh D, Selkoe DJ. Soluble amyloid beta-protein dimers isolated from Alzheimer cortex directly induce Tau hyperphosphorylation and neuritic degeneration. Proc Natl Acad Sci U S A 2011; 108:5819 - 24; http://dx.doi.org/10.1073/pnas.1017033108; PMID: 21421841
- Sakono M, Zako T. Amyloid oligomers: formation and toxicity of Abeta oligomers. FEBS J 2010; 277:1348 - 58; http://dx.doi.org/10.1111/j.1742-4658.2010.07568.x; PMID: 20148964
- Qu BX, Xiang Q, Li L, Johnston SA, Hynan LS, Rosenberg RN. Abeta42 gene vaccine prevents Abeta42 deposition in brain of double transgenic mice. J Neurol Sci 2007; 260:204 - 13; http://dx.doi.org/10.1016/j.jns.2007.05.012; PMID: 17574274
- Weksler ME, Gouras G, Relkin NR, Szabo P. The immune system, amyloid-beta peptide, and Alzheimer’s disease. Immunol Rev 2005; 205:244 - 56; http://dx.doi.org/10.1111/j.0105-2896.2005.00264.x; PMID: 15882358
- Wisniewski T, Frangione B. Immunological and anti-chaperone therapeutic approaches for Alzheimer disease. Brain Pathol 2005; 15:72 - 7; http://dx.doi.org/10.1111/j.1750-3639.2005.tb00102.x; PMID: 15779239
- Citron M. Strategies for disease modification in Alzheimer’s disease. Nat Rev Neurosci 2004; 5:677 - 85; http://dx.doi.org/10.1038/nrn1495; PMID: 15322526
- Singh H, Raghava GP. ProPred1: prediction of promiscuous MHC Class-I binding sites. Bioinformatics 2003; 19:1009 - 14; http://dx.doi.org/10.1093/bioinformatics/btg108; PMID: 12761064
- Walsh DT, Bresciani L, Saunders D, Manca MF, Jen A, Gentleman SM, et al. Amyloid beta peptide causes chronic glial cell activation and neuro-degeneration after intravitreal injection. Neuropathol Appl Neurobiol 2005; 31:491 - 502; http://dx.doi.org/10.1111/j.1365-2990.2005.00666.x; PMID: 16150120
- Wiessner C, Wiederhold KH, Tissot AC, Frey P, Danner S, Jacobson LH, et al. The second-generation active Aβ immunotherapy CAD106 reduces amyloid accumulation in APP transgenic mice while minimizing potential side effects. J Neurosci 2011; 31:9323 - 31; http://dx.doi.org/10.1523/JNEUROSCI.0293-11.2011; PMID: 21697382
- Maier M, Seabrook TJ, Lemere CA. Modulation of the humoral and cellular immune response in Abeta immunotherapy by the adjuvants monophosphoryl lipid A (MPL), cholera toxin B subunit (CTB) and E. coli enterotoxin LT(R192G). Vaccine 2005; 23:5149 - 59; http://dx.doi.org/10.1016/j.vaccine.2005.06.018; PMID: 16054274
- Cribbs DH, Ghochikyan A, Vasilevko V, Tran M, Petrushina I, Sadzikava N, et al. Adjuvant-dependent modulation of Th1 and Th2 responses to immunization with beta-amyloid. Int Immunol 2003; 15:505 - 14; http://dx.doi.org/10.1093/intimm/dxg049; PMID: 12663680
- O’Hagan DT, Ott GS, De Gregorio E, Seubert A. The mechanism of action of MF59 - an innately attractive adjuvant formulation. Vaccine 2012; 30:4341 - 8; http://dx.doi.org/10.1016/j.vaccine.2011.09.061; PMID: 22682289
- Knopman DS, Parisi JE, Salviati A, Floriach-Robert M, Boeve BF, Ivnik RJ, et al. Neuropathology of cognitively normal elderly. J Neuropathol Exp Neurol 2003; 62:1087 - 95; PMID: 14656067
- Panza F, Frisardi V, Solfrizzi V, Imbimbo BP, Logroscino G, Santamato A, et al. Immunotherapy for Alzheimer’s disease: from anti-β-amyloid to tau-based immunization strategies. Immunotherapy 2012; 4:213 - 38; http://dx.doi.org/10.2217/imt.11.170; PMID: 22339463
- Frisardi V, Solfrizzi V, Imbimbo PB, Capurso C, D’Introno A, Colacicco AM, et al. Towards disease-modifying treatment of Alzheimer’s disease: drugs targeting beta-amyloid. Curr Alzheimer Res 2010; 7:40 - 55; http://dx.doi.org/10.2174/156720510790274400; PMID: 19939231
- Chackerian B, Rangel M, Hunter Z, Peabody DS. Virus and virus-like particle-based immunogens for Alzheimer’s disease induce antibody responses against amyloid-beta without concomitant T cell responses. Vaccine 2006; 24:6321 - 31; http://dx.doi.org/10.1016/j.vaccine.2006.05.059; PMID: 16806604
- Wisniewski T. Active immunotherapy for Alzheimer’s disease. Lancet Neurol 2012; 11:571 - 2; http://dx.doi.org/10.1016/S1474-4422(12)70136-9; PMID: 22677257
- Mosmann TR, Cherwinski H, Bond MW, Giedlin MA, Coffman RL. Two types of murine helper T cell clone. I. Definition according to profiles of lymphokine activities and secreted proteins. 1986. J Immunol 2005; 175:5 - 14; PMID: 15972624
- Yamada K, Yabuki C, Seubert P, Schenk D, Hori Y, Ohtsuki S, et al. Abeta immunotherapy: intracerebral sequestration of Abeta by an anti-Abeta monoclonal antibody 266 with high affinity to soluble Abeta. J Neurosci 2009; 29:11393 - 8; http://dx.doi.org/10.1523/JNEUROSCI.2021-09.2009; PMID: 19741145
- Winblad B, Andreasen N, Minthon L, Floesser A, Imbert G, Dumortier T, et al. Safety, tolerability, and antibody response of active Aβ immunotherapy with CAD106 in patients with Alzheimer’s disease: randomised, double-blind, placebo-controlled, first-in-human study. Lancet Neurol 2012; 11:597 - 604; http://dx.doi.org/10.1016/S1474-4422(12)70140-0; PMID: 22677258
- Leverone JF, Spooner ET, Lehman HK, Clements JD, Lemere CA. Abeta1-15 is less immunogenic than Abeta1-40/42 for intranasal immunization of wild-type mice but may be effective for “boosting”. Vaccine 2003; 21:2197 - 206; http://dx.doi.org/10.1016/S0264-410X(02)00754-5; PMID: 12706711
- Guruprasad K, Rajkumar S. Beta-and gamma-turns in proteins revisited: a new set of amino acid turn-type dependent positional preferences and potentials. J Biosci 2000; 25:143 - 56; PMID: 10878855
- Oakley H, Cole SL, Logan S, Maus E, Shao P, Craft J, et al. Intraneuronal beta-amyloid aggregates, neurodegeneration, and neuron loss in transgenic mice with five familial Alzheimer’s disease mutations: potential factors in amyloid plaque formation. J Neurosci 2006; 26:10129 - 40; http://dx.doi.org/10.1523/JNEUROSCI.1202-06.2006; PMID: 17021169
- Biancalana M, Koide S. Molecular mechanism of Thioflavin-T binding to amyloid fibrils. Biochim Biophys Acta 2010; 1804:1405 - 12; http://dx.doi.org/10.1016/j.bbapap.2010.04.001; PMID: 20399286
- Walter J, Kaether C, Steiner H, Haass C. The cell biology of Alzheimer’s disease: uncovering the secrets of secretases. Curr Opin Neurobiol 2001; 11:585 - 90; http://dx.doi.org/10.1016/S0959-4388(00)00253-1; PMID: 11595492
- Smith WB, Guida L, Sun Q, Korpelainen EI, van den Heuvel C, Gillis D, et al. Neutrophils activated by granulocyte-macrophage colony-stimulating factor express receptors for interleukin-3 which mediate class II expression. Blood 1995; 86:3938 - 44; PMID: 7579364
- Lee HG, Zhu X, Nunomura A, Perry G, Smith MA. Amyloid beta: the alternate hypothesis. Curr Alzheimer Res 2006; 3:75 - 80; http://dx.doi.org/10.2174/156720506775697124; PMID: 16472207
- Rottkamp CA, Raina AK, Zhu X, Gaier E, Bush AI, Atwood CS, et al. Redox-active iron mediates amyloid-beta toxicity. Free Radic Biol Med 2001; 30:447 - 50; http://dx.doi.org/10.1016/S0891-5849(00)00494-9; PMID: 11182300
- Lynch MA, Mills KH. Immunology meets neuroscience--opportunities for immune intervention in neurodegenerative diseases. Brain Behav Immun 2012; 26:1 - 10; http://dx.doi.org/10.1016/j.bbi.2011.05.013; PMID: 21664452
- Gosselin EJ, Wardwell K, Rigby WF, Guyre PM. Induction of MHC class II on human polymorphonuclear neutrophils by granulocyte/macrophage colony-stimulating factor, IFN-gamma, and IL-3. J Immunol 1993; 151:1482 - 90; PMID: 8335942
- Ferrer I, Boada Rovira M, Sánchez Guerra ML, Rey MJ, Costa-Jussá F. Neuropathology and pathogenesis of encephalitis following amyloid-beta immunization in Alzheimer’s disease. Brain Pathol 2004; 14:11 - 20; http://dx.doi.org/10.1111/j.1750-3639.2004.tb00493.x; PMID: 14997933
- Zhang Y, Lee DH. Sink hypothesis and therapeutic strategies for attenuating Abeta levels. Neuroscientist 2011; 17:163 - 73; http://dx.doi.org/10.1177/1073858410381532; PMID: 21330304
- Head E, Garzon-Rodriguez W, Johnson JK, Lott IT, Cotman CW, Glabe C. Oxidation of Abeta and plaque biogenesis in Alzheimer’s disease and Down syndrome. Neurobiol Dis 2001; 8:792 - 806; http://dx.doi.org/10.1006/nbdi.2001.0431; PMID: 11592849
- Qing H, He G, Ly PT, Fox CJ, Staufenbiel M, Cai F, et al. Valproic acid inhibits Abeta production, neuritic plaque formation, and behavioral deficits in Alzheimer’s disease mouse models. J Exp Med 2008; 205:2781 - 9; http://dx.doi.org/10.1084/jem.20081588; PMID: 18955571