Abstract
Rotavirus (RV) is the most important cause of severe gastroenteritis in children worldwide. Current live RV vaccines are efficacious but show lower efficacy in developing countries, as well as a low risk of intussusception. This has led to the development of parenteral non-live candidate vaccines against RV. RV capsid VP6 protein is highly conserved and the most abundant RV protein forming highly immunogenic oligomeric structures with multivalent antigen expression. Both recombinant VP6 (rVP6) or double-layered (dl) 2/6-virus-like particles (VLPs), might be considered as the simplest RV subunit vaccine candidates. Human rVP6 protein and dl2/6-VLPs were produced in Sf9 insect cells by baculovirus expression system. Formation of rVP6 tubules and VLPs were confirmed by electron microscopy. BALB/c mice were immunized intramuscularly, and immune responses were analyzed. Both rVP6 and dl2/6-VLPs induced a balanced Th1-type and Th2-type response and high levels of serum IgG antibodies with cross-reactivity against different RV strains (Wa, SC2, BrB, 69M, L26, WC3, and RRV). In addition, mucosal VP6-specific IgG and IgA antibodies were detected in feces and vaginal washes (VW) of immunized animals. Importantly, VWs of immunized mice inhibited RV Wa and RRV infection in vitro. Immunization with either protein preparation induced a similar level of VP6-specific, interferon-γ secreting CD4+ T cells in response to different RVs or the 18-mer peptide (AA242–259), a VP6-specific CD4+ T cell epitope. RV rVP6 and dl2/6-VLPs induced equally strong humoral and cellular responses against RV in mice and therefore, may be considered as non-live vaccine candidates against RV.
Keywords: :
Introduction
Rotavirus (RV) is the major cause of severe gastroenteritis in children worldwide with high rates of morbidity and mortality.Citation1 RVs are classified into seven distinct serogroups (A-G) with human associated infections predominantly caused by group A.Citation2 The capsid of RV consists of three protein layers: the core formed by 60 dimers of VP2, the intermediate layer consisting of 260 trimers of VP6 covered by the external layer of VP7 glycoprotein with 60 hemagglutinin spikes of VP4.Citation3,Citation4 The outer capsid proteins VP7 and VP4 define 19 G (glycoprotein) and 27 P (protease sensitive) RV typesCitation5 and induce protective immunity based on type-specific neutralizing antibodies (N-Abs).Citation6 The major capsid protein VP6 contains viral group- and subgroup-specific antigenic determinants and is highly conserved,Citation7 immunogenic,Citation2,Citation8-Citation10 and the most abundant RV protein.Citation6 When produced by recombinant baculovirus (rBV) expression system VP6 can self-assemble into different oligomeric structures, including tubules, spheres and sheets.Citation11 Moreover, co-expression of VP2 and VP6 results in the formation of double-layered (dl) virus-like particles (VLP)Citation3 while co-expression of VP2, VP6, and VP7 (with or without VP4) leads to triple-layered (tl) VLPs resembling native infectious RV particles.Citation12
Two recently licensed live oral RV vaccines (pentavalent RotaTeq® and monovalent Rotarix®) are efficacious and largely safe, but they may be associated with a low risk of intussusceptionCitation13 and other issues related to live vaccines including the risk of introduction of vaccine strains into the environment, genetic reassortment between the vaccine and a wild-type strain, and reversion of the vaccine strain toward virulence.Citation1 Non-live vaccines against RV have therefore been considered, including inactivated RV particles,Citation14,Citation15 RV VLPs of different composition,Citation16-Citation18 recombinant VP6 (rVP6) protein,Citation8,Citation19,Citation20 and DNA plasmids expressing VP6.Citation21,Citation22 The evidence that immune responses to the neutralizing proteins VP4 and VP7 are not necessarily required for protection against RV infection in animals is considerable.Citation16-Citation18 Inactivated dl RV particles lacking VP4 and VP7 induced protection in mouse model against virus shedding.Citation14,Citation15 When rVP6 was expressed in Escherichia coli as a fusion chimeric protein and administered to mice, intestinal RV antigen production was suppressed by >93% after murine RV challenge.Citation19,Citation20 Furthermore, immunization with DNA encoding VP6 induced protection in mice.Citation21,Citation22 These studies suggest that VP6 alone plays an important role in RV protective immunity. Although VP6 does not induce serum N-Abs it induces heterologous cross-protective RV immunity in mice.Citation14,Citation15,Citation18,Citation23 The mechanisms of protection are not clearly defined but it has been suggested that protection is dependent on VP6-specific CD4+ T-helper (Th) cells,Citation18,Citation23 which have been shown to mediate protection either by direct cytotoxic mechanism or by antiviral cytokine interferon–γ (IFN-γ) production.Citation24-Citation26 In addition, mucosal VP6-specific IgG and, even more, IgA antibodies were shown to correlate with protection in a mouse RV challenge model.Citation22,Citation27-Citation29
Therefore, the ability of VP6 to form highly immunogenic oligomeric structures (i.e., tubules and VLPs) with repetitive multivalent antigen expressionCitation11 in vitro and to elicit protective immune response makes it the simplest non-live, subunit RV vaccine candidate relatively easy to produce. We hypothesized that both rVP6 and dl2/6-VLPs are able to induce similar VP6-specific immune responses although having different assembly conformation. However, the immunogenicity of these RV VP6 derived oligomeric subviral structures has not been compared simultaneously in animal models. Our results show that both structures are equally immunogenic in mice, supporting the use of either one as a non-live vaccine candidate against RV gastroenteritis.
Results
Expression and characterization of rVP6 and dl2/6-VLPs
Production conditions for the recombinant human rVP6 protein and dl2/6-VLPs were optimized. The best rVP6 yield (~5 mg/l) was achieved at 7 days post infection (dpi) with rBV-VP6 of 5 pfu/cell. Production of dl2/6-VLPs with 10 pfu/cell of the rBV-VP2 and 1 pfu/cell of rBV-VP6 yielded ~3–4 mg/l of the total protein concentration with the 42% proportion of VP6.
shows sodium dodecyl sulphate polyacrylamide gel electrophoresis (SDS-PAGE) gel with the identified RV VP6 (45 kDa) and VP2 (102 kDa) bands of purified products. In concordance with the work of othersCitation30 we also detected an additional cleavage product of VP2 with an apparent molecular weight of ~90 kDa. Oligomeric structures including rVP6 tubules (~0.2–1.5 μm in length) with hexagonal subunit pattern and dl2/6-VLPs (~65 nm in diameter) were confirmed under electron microscopy (EM) (). At pH 7.2 most of the rVP6 trimers formed tubular structures with occasional spheres and sheets, as shown by others as well.Citation11 No VP6 tubules were detected in the dl2/6-VLPs preparations.
Figure 1. Characterization of the purified rVP6 and dl2/6-VLPs. (A) Purity and integrity analysis of RV rVP6 (lane 1) and dl2/6-VLPs (lane 2) with SDS-PAGE followed by PageBlue staining. Lane M illustrates molecular weight marker. Corresponding molecular weights are indicated with arrows on the right of the gel image. (B) Electron microscopy images of morphological structures assembled by RV rVP6 (panel 1) and dl2/6-VLPs (panel 2) corresponding to the SDS-PAGE lanes 1 and 2 (A) respectively. Protein structures were examined after negative staining with 3% uranyl acetate pH 4.6. (C) Evaluation of antigenicity of the purified rVP6 and dl2/6-VLPs at different concentrations with ELISA for total IgG antibodies using human polyclonal anti-rotavirus serum. Mean OD values with standard errors of duplicate wells are shown.
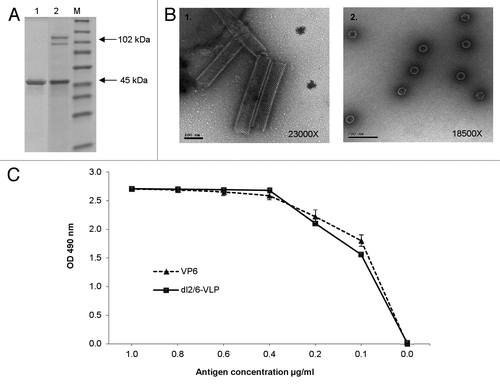
The antigenicity of the rVP6 and dl2/6-VLPs was compared with an enzyme-linked immunosorbent assay (ELISA) assay. When equal amount of VP6 protein in both preparations was coated in the wells rVP6 and dl2/6-VLPs reacted equally with human RV antiserum (). In addition, recombinant VP6 and dl2/6-VLP contained endotoxin at a very low level (0.57 EU and 0.21 EU per 20 μg of protein respectively).
Serum IgG and IgA antibody responses
Mice were immunized intramuscularly (IM) twice with 3 μg or 10 μg of rVP6 or dl2/6-VLPs (the doses of dl2/6-VLPs calculated according to the VP6 content, respectively). The single immunization with both doses of the two RV immunogens induced detectable VP6-specific IgG levels in the sera collected at week 3 (). After the first immunization IgG antibodies were boosted with the second dose () resulting in similar levels of total IgG in each experimental group at the time of termination (p ≥ 0.05). Although low IgG responses (optical density, OD ≤ 0.18) of pre-immune sera at the study week 0 were seen (), the responses were below the cut-off value (OD 0.15) in the termination sera of control mice at the study week 5 ().
Figure 2. Humoral immune response in BALB/c mice immunized with RV VP6 proteins. (A) Kinetics of RV VP6–specific total IgG antibodies in sera of BALB/c mice (3–5 mice/group) immunized intramuscularly with 3 μg or 10 μg doses of rVP6 or dl2/6-VLPs at weeks 0 and 3. Group means with standard errors of tail blood samples collected at study weeks 0 (pre-immune sera) and 3 and termination sera at week 5 are shown. (B) End point serum titrations of RV VP6-specific IgG antibody responses of different experimental groups of mice. Control mice receiving carrier only (PBS) were used as controls. Mean titers with standard errors of termination sera at week 5 are shown. A dashed line indicates the lower cut off value (OD 0.15) calculated as follows: mean OD (termination sera of control mice) + 3 × SD (C) VP6-specific IgG1 and IgG2a subtype antibody responses of groups of mice immunized with rVP6 or dl2/6-VLPs. Data are expressed as the geometric mean titers (log10) with standard errors of the reciprocal dilutions of specific IgG1 and IgG2a antibodies in termination sera at week 5.
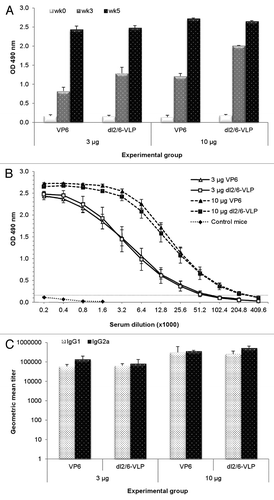
High titers of VP6 IgG antibodies were elicited with both doses of rVP6 or dl2/6-VLPs (p ≥ 0.5) with end point titer > 5log10 in each experimental group (). IgG1 and IgG2a serum antibodies specific for VP6 () were measured as indicators of Th2- and Th1-type immune responses.Citation31 Doses of 10 μg rVP6 or dl2/6-VLPs induced 5- and 4-fold increases in IgG1 titers compared with 3 μg doses. Similarly, compared with 3 μg doses, immunization with 10 μg of rVP6 or dl2/6-VLPs caused 3- and 6-fold greater IgG2a titers. However, both doses of rVP6 and dl2/6-VLPs induced a balanced Th1-type and Th2-type response with comparable IgG2a and IgG1 titers (p ≥ 0.2).
IgA antibody levels were measured in groupwise pooled sera of the immunized and control mice. We did not detect any VP6-specific IgA (OD ≤ 0.07) in the termination sera of any group even at a very low dilution (1:2, respectively).
Cross reactive serum IgG antibody responses
We further evaluated whether rVP6 or dl2/6-VLPs could induce heterotypic IgG antibodies to different RV strains using different RV cell cultures as antigens in ELISA (). Serum antibodies of mice immunized with 10 μg of antigenic formulations were cross-reactive with different RV strains including bovine WC3 and rhesus RV (RRV) as well as several human strains indicating broad reactivity as well as conserved nature of the VP6 protein. The 3 μg doses also elicited high levels of cross-reactive IgG antibodies toward each of the RV strains tested (data not shown). Significant differences were observed between all experimental groups and the control group (p < 0.05).
Mucosal antibodies
We also determined mucosal VP6-specific IgG antibodies in the groupwise pooled 10% fecal suspensions and vaginal washes (VW) of the experimental groups. Low levels of fecal (final dilution 1:50) IgG antibodies prior to termination at week 5 were detected in the groups immunized with 3 μg doses of rVP6 (OD 0.18) and dl2/6-VLP (OD 0.24). However, considerable levels of IgG antibodies in feces were induced in the groups immunized with 10 μg doses of rVP6 (OD 0.61) and dl2/6-VLP (OD 1.08) at a 1:50 dilution. Similar quantities of VP6-specific IgG antibodies (OD 0.61 for rVP6; OD 1.13 for dl2/6-VLP) were detected in 1:5 diluted VWs of groups immunized with 10 μg doses. No anti-VP6 IgG was detected in feces or VWs of control mice (OD ≤ 0.08).
After two immunization doses low to moderate levels of mucosal VP6-specific IgA (OD 0.13–0.24) were detected in feces at a dilution of 1:20 and VWs at a dilution of 1:2 of groups immunized with 10 μg doses of both immunogens. Mucosal samples of control group remained negative for IgA (OD 0.01).
Cellular immune responses
The T cell immune responses from immune and naïve cells were analyzed by enzyme-linked immunosorbent spot (ELISPOT) IFN-γ assay. Splenocytes were stimulated ex vivo with different RV cell culture antigens or VP6-specific 18-mer R6–2 peptide. Immunization of mice with either rVP6 or dl2/6-VLPs revealed significant quantities of VP6-specific, IFN-γ secreting cells in response to stimulation with Wa, BrB or WC3 RV cell cultures (). Comparisons of T cell responses indicated that levels were substantially higher in every immunized group compared with controls but were not significantly different between the experimental groups (p > 0.05 for all). All immunized mice developed significant T cell responses to the R6–2 peptide (), an earlier identified VP6-specific T cell epitope.Citation24 MA104 mock cell culture or negative control peptide stimulated no IFN-γ production.
Figure 4. Rotavirus VP6-specific T-cell response detection by ELISPOT. (A) Different RV cell cultures and a synthetic RV R6–2 peptide were used to stimulate interferon-γ (IFN-γ) production from the splenocytes of mice immunized twice with 3 μg rVP6 or dl2/6-VLPs. Control mice received carrier only (PBS). Results are expressed as mean IFN-γ spot forming cells (SFC)/106 cells of duplicate wells with standard errors. (B) CD4+ T cells are responsible for IFN-γ production of mice immunized twice with 10 μg dl2/6-VLPs. Splenocytes were stimulated with different RV cell cultures and a synthetic R6–2 peptide in the presence or absence of CD4 and CD8 specific antibodies or control antibodies to block the T cell activation. Results are expressed as the mean % inhibition of duplicate wells.
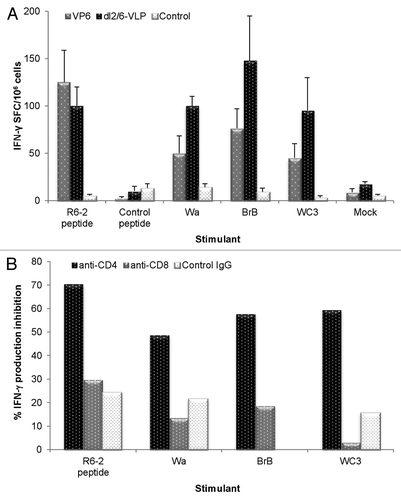
In order to determine the T cell type responsible for production of IFN-γ we employed functional antibodies specific for mouse CD4 and CD8 molecules to block the interaction of antigen and T cell receptor and thus T cell activation. An ELISPOT-IFN-γ assay was performed with splenocytes of mice immunized with 10 μg of dl2/6-VLPs stimulated with the R6–2 peptide and RV cell cultures in the presence or absence of anti-CD4, anti-CD8 or control antibodies (). The results showed that only CD4 specific antibodies blocked IFN-γ production by the immune cells (50–70%, respectively) in response to the peptide epitope and the RVs indicating that CD4+ T cells were the main effectors producing IFN-γ.
Inhibition of RV infection in vitro
Inhibition of RV Wa (homologous to VP6 used for immunization) and RRV infection by mucosal samples and sera of immunized and control mice was tested by ELISA-based antigen reduction neutralization assay. No neutralizing effect was detected with the serum () samples of immunized or control groups. Several attempts to test the neutralizing activity of fecal samples failed each time as the samples from all mice including control even at a high dilutions induced toxicity for all cell cultures, an observation seen by others as well.Citation32 Thus, VWs containing similar levels of mucosal antibodies were used for neutralization experiments. VWs of mice immunized with 10 μg doses of rVP6 or dl2/6-VLPs inhibited RV infection with both strains indicating homotypic and heterotypic neutralizing activity ( and ). Inhibition of infectivity of RV Wa () and RRV () was detected in VWs of rVP6 immunized mice with the maximum neutralization titers of 160 and 320, respectively. The neutralizing titer of dl2/6-VLPs immunized group was 160 against Wa () and RRV (). The low neutralization (titer of ≤10) was observed in VWs of control mice ( and ). All the samples were analyzed simultaneously except that the control #2 in has been run later, hence a weakness in the study. However in all VWs (including two separate pooled controls) were run simultaneously.
Figure 5. Inhibition of rotavirus infection in vitro. Inhibition activity of sera (A) and vaginal washes (B and C) of mice immunized with 10 μg of rVP6 or dl2/6-VLPs against homologous rotavirus Wa (A and B) and heterologous RRV (C) was analyzed by Neutralizing ELISA (NELISA). Sera and vaginal washes of mice receiving carrier only (PBS) were used as controls. Control #1 and #2 shown in panels B and C represent two separate pooled controls. In neutralization experiments the immunized and control samples from the same time point (week 5) were run simultaneously. Only neutralization of control #2 against Wa in panel B has been run later. Results are shown as the mean % inhibition of rotavirus infectivity of at least two independent experiments, each done in duplicate, with standard errors. A dashed line indicates 60% reduction in virus infectivity.
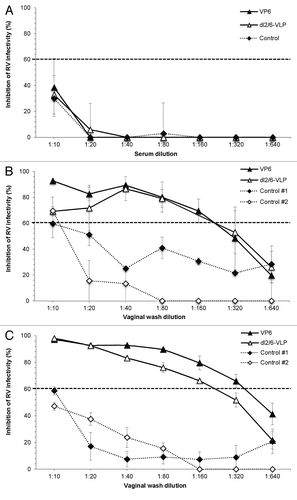
Discussion
We have recently proposed a combined RV VP6 – norovirus VLP vaccine to combat acute gastroenteritis in children.Citation33 For the RV VP6 component of the proposed vaccine candidate either rVP6 tubular structures or dl2/6-VLPs might be considered. To prove this hypothesis, RV proteins rVP6 and dl2/6-VLPs were produced by the rBV expression system and immunogenicity of these proteins was compared in a mouse model.
This comprehensive study of humoral and cellular immune responses induced by the two VP6 assembly formulations, showed that either one can be used as a simplest subunit vaccine candidate against RV. Although this study does not extend to determine in vivo RV protection with challenge experiments, it determines functionality of anti-VP6 antibodies by inhibition of RV infection in vitro.
Immunization of mice and rabbits with RV dl2/6-VLPs,Citation16-Citation18 chimeric VP6 proteinCitation19,Citation20 or DNA encoding VP6Citation21,Citation22 have been shown to efficiently protect against RV infection, suggesting that VP6 plays a notable role in RV protective immunity, and may be sufficient for protection without the surface neutralizing proteins VP4 or VP7. An indirect proof comes from the experience of live attenuated RV vaccines which are currently in use. The monovalent (G1P1A[8]) Rotarix® vaccine is as effective as the pentavalent RotaTeq® vaccine against severe RV gastroenteritis caused by different RV genotypesCitation34,Citation35 indicating the importance of other types of protective mechanisms but the type-specific N-Abs to external VP4 or VP7 proteins.
We found that the repeating multivalent antigenic structures of rVP6 trimers packed into tubules or in the form of dl2/6-VLPs are both very strong immunogens in mice without the need for external adjuvants. In contrast to soluble proteins which often require an adjuvant to potentiate their function,Citation19,Citation20,Citation26 antigens displayed in a highly dense, multivalent format such as the oligomeric rVP6 structures described in this study, efficiently activate B cells leading to cross-linking of B cell receptors on the cell surface.Citation36 In addition, the particulate nature and the size range of around 40 nm of VLPs is optimal for the uptake of nanoparticles by dendritic cells (DC),Citation37 which play a key role in activating innate and adaptive immune responses.Citation38 Likewise, bluetongue virus nonstructural protein NS1 forms tubules similar to VP6 in morphology and size, and have been shown to be extremely immunogenic and excellent heterologous antigen carriers.Citation39 These tubules, internalized by macrophages and DC, are efficiently presenting T cell and B epitopes to the immune system.
In addition to the enhancing immunological features of the rVP6 protein discussed above there are other characteristics of VP6 protein which make it a promising vaccine candidate. VP6 among group A RVs is highly conserved with ~90% homology at the amino acid level.Citation7 Our results show that when the mice were immunized with rVP6 tubules or dl2/6-VLPs, RV-specific serum IgG antibodies and T cells were reactive to RV with VP6 type 1 (Wa), homologous to the rVP6 used for immunizations, as well as cross-reactive to heterologous RVs having VP6 type 2 (e.g., 69M, L26, WC3, and RRV) indicating broad reactivity because of the well conserved nature of VP6 among different RVs. In addition, these findings indicate the conservation of the VP6-specific B and T cell epitopes across different RV strains. When the splenocytes of mice were stimulated with a synthetic 18-mer VP6-specific peptide, the peptide previously reported to stimulate memory CD4+ T cells,Citation24 we observed that both rVP6 and dl2/6-VLPs recipients developed significant IFN-γ producing T cells. The cells responsible for IFN-γ production in response to the RV cell culture antigens as well as to the R6–2 peptide were identified as CD4+ T cells in blocking antibody experiments. VP6 specific CD4+ Th cells, as the ones we detected, were shown to suffice for protection against RV infection in mice either by direct cytotoxic mechanism in mucosa or by antiviral cytokine, IFN-γ, production.Citation24-Citation26 In addition, VP6-specific Th cells in mice have been shown to provide cognate help to B cells specific for neutralizing epitopes on the heterotypic VP4 or VP7 molecules of RV,Citation18,Citation23 making these T cells essential for the induction of a cross-protective immune response against RV. Recently, CD4+ T cells responding to conserved influenza internal proteins were shown to correlate with disease protection against influenza challenge in humans.Citation40 Altogether, immunization with rVP6 alone could be expected to protect against RV gastroenteritis caused by any group A RVs.
Mucosal VP6-specific IgG and even more IgA antibodies have also been implicated to account for protection against RV challenge in a mouse modelCitation22,Citation27 and non-neutralizing mucosal IgA against VP6 has been shown to inhibit RV replication intracellularly.Citation29,Citation41 We also detected considerable levels of IgG and low levels of IgA in the intestinal lumen and VWs of mice immunized with rVP6 or dl2/6-VLPs. It may be that mucosal delivery or/and use of external adjuvants are needed to obtain higher levels of IgA antibodies. However, relatively low levels of fecal VP6-specific IgA, comparable to the levels we have detected, were induced by mucosal immunization with VP6 DNA vaccineCitation22 or adjuvanted dl2/6-VLPsCitation27 and were shown to correlate to protection against RV challenge in mice. Although it was thought that antibodies directed against VP6 have no neutralizing ability in vitro, evidence to the contrary has been mounting.Citation42-Citation45 Our attempts to neutralize RV with fecal suspensions failed most likely because of toxicity of the samples to the MA104 cells.Citation32 Instead, in the present work we show for the first time that VWs of mice immunized with both of the VP6 preparations inhibited homologous Wa RV and heterologous RRV infection in vitro. Low neutralization background seen in VWs of control mice may be due to non-specific factors in VWs such as the estrous cycle. These results indicate the importance of VP6-specific IgA antibodies especially as the serum samples, which completely lacked IgA, also lacked RV neutralizing activity.
Although IgA antibodies of sera were not detected, the presence of serum VP6-specific IgA cannot be definitively excluded as existence of serum IgG antibodies at high titers may interfere with the detection of IgA and consequentially neutralizing activity.Citation46,Citation47 Moreover, the timing of serum sample collection at the termination of mice at week 5 might be suboptimal for IgA detection. In any event, other investigators have also reported RV-specificCitation48 as well as VP6-specificCitation28 mucosal IgA antibodies without detection of serum IgA following parentalCitation48 as well as mucosal immunization.Citation28
The mechanisms by which VP6-specific antibodies exert the neutralizing effect are not yet understood but it is possible that these antibodies interfere with the binding of VP6 to the cellular receptor as has been suggested by Gualtero et al.Citation43 or induce conformational changes in the viral particle interfering with virus entry to the cell. Alternatively, it has been also shown that VP6-specific mucosal (secretory) IgA can impair RV infection in vitro by intracellular neutralization.Citation49 It is also possible that neutralizing anti-VP6 IgA we detected could be introduced to the cells on the virus and inhibit RV replication intracellularly as it was shown that VP6-specific IgA antibodies bind to intact RV particles.Citation49
Recombinant VP6 and dl2/6-VLPs have been generally administered mucosally to mice in combination with different adjuvants.Citation17,Citation18,Citation20,Citation26,Citation27,Citation29,Citation50 In this study we have administered rVP6 tubuli or dl2/6-VLPs parenterally without an adjuvant. Only one study described immunogenicity of oligomeric VP6 delivered IM without the adjuvant but lacking detailed analysis of the mucosal or cellular immune responses.Citation8 Importantly, humoral and cellular immune responses induced by the two VP6 oligomeric structures have never been compared simultaneously in an animal model. Furthermore, we showed for the first time inhibition of RV replication by VP6-specific mucosal antibodies in vitro whereas others have shown correlation of mucosal antibodies with the protection in vivo.Citation22,Citation27-Citation29
Compared with tlVLPs containing RV external neutralizing proteins VP4 and VP7, both rVP6 and dl2/6-VLPs are simple and straightforward to produce and purify for use as a candidate vaccine. These non-replicating subunit protein vaccines are also considered safe as they are deprived of any live attenuated or inactivated viruses or their genetic material. Both VP6 derived oligomeric subviral protein structures were equally immunogenic and able to elicit strong cross-reactive B and T cell immune responses in mice, specifically IFN-γ producing CD4+ T cells and mucosal IgG and IgA antibodies, which are implicated as mediators in protection against RV infection in mice.Citation22,Citation24-Citation27 We therefore propose that RV rVP6 protein either in tubular form or dl2/6-VLPs can be used to possibly induce protective RV immunity in humans. In young children, a VP6 vaccine could be used after primary immunization with live RV vaccines as protective immunity induced by live RV vaccines may wane over time, particularly in developing countries, and booster immunization may be desirable. Live RV vaccines currently in use could hardly be used for such boosting because of the risk of intussusception. Studies of VP6 containing candidate vaccines in humans are warranted and should be conducted for primary and booster immunization.
Materials and Methods
Construction of recombinant VP6 and VP2 expression systems
To obtain the complete nucleotide sequences of VP6 and VP2 gene segments extracted dsRNA of RV from the stool of 3-y-old RV G1P1A[8] positive patientCitation33 was subjected to RT-PCR reactions with specific primer pairs of VP6 and VP2Citation51 followed by sequencing as previously described.Citation33 The DNAs encoding sequences of RV VP6 (Database accession number GQ477131) and VP2 (Database accession number HQ609556) were optimized for expression in Spodoptera frugiperda (Geneart AG, Regensburg, Germany). Nucleotide differences resulted in no amino acid changes. The VP6 and VP2 nucleotide sequences were inserted into the pFastBac1 expression vector (Invitrogen) and the pFastBacDual expression vector (Invitrogen) respectively. The generation of recombinant bacmid DNAs by a Bac-to-Bac Baculovirus expression system (Invitrogen, Cat. 10359–016), followed by the amplification of P1 and P2 rBV stocks, was performed as described earlier.Citation33 rBV titer of P2 stocks expressed as the multiplicity of infection (MOI) was determined by the BacPak™ Baculovirus Rapid titer kit (Clontech laboratories, Cat. 631406).
Rotavirus rVP6 and dl2/6-VLP production
To produce rVP6 and dl2/6-VLPs cell number, MOI/cell, and dpi were tested for the optimal conditions. The recombinant proteins were produced and purified essentially as previously described for rVP6.Citation33 rVP6 was produced in S. frugiperda (Sf9) insect cells (Gibco, Cat.12659–017) at a density of 1 × 106 cells/ml and MOI of 5 pfu/cell. For dl2/6-VLPs production Sf9 cells were co-infected with the rBV-VP2 P2 stock and rBV-VP6 P2 stock at a MOI of 10 and 1 pfu/cell. Cell cultures at 3–8 dpi were analyzed for recombinant protein contents by SDS-PAGE. The 7 dpi conditions were chosen as optimal for producing significant amount of the proteins for further purification steps.
Rotavirus rVP6 and dl2/6-VLP purification
Cultures were clarified at 1,000 rpm for 20 min at +4°C. Cell lysates were used for purification of rVP6 while dl2/6-VLPs were purified from the supernatant. Both proteins were concentrated by ultracentrifugation at 100,000 × g for 1.5 h at +4°C (L8–60M ultracentrifuge, Beckman SW-32.1 Ti rotor) and purified on continuous sucrose gradients (10–60%). Fractions containing rVP6 or dl2/6VLPs were pooled, dialyzed against phosphate buffered saline (PBS) by Slide-A-Lyzer® Dialysis Cassette G2 of 20 000 MWCO (Thermo Scientific, Cat. 87738) and concentrated by ultrafiltration in Amicon Ultra-30 or -100 centrifugal filter units (Millipore Carrigtwohill, Cat. UFC903024, Cat. UFC910024). The schematic diagram of the production and purification processes resulting in the best yield of the VP6 proteins is shown in Figure S1. Total protein concentration of the products was quantified by Pierce® BCA Protein Assay (Thermo Fisher Scientific Inc., Cat. 23227).
Determination of purity, integrity, morphology and antigenicity of the recombinant proteins
The purity, integrity, and morphology of rVP6 and dl2/6VLPs were verified by SDS-PAGE followed by densitometric analysis with AlphaEase® FC Software (Alpha Innotech, San Leandro, CA) and EM with FEI Tecnai F12 (Philips Electron Optics, Holland) after negative staining with 3% uranyl acetate pH 4.6. The proportion of VP6 protein in dl2/6-VLPs was determined by densitometric analysis and the VP6 concentration calculated from the total protein content determined by BCA Protein Assay.
Endotoxin levels were quantified with Limulus Amebocyte Lysate assay (Lonza, Cat. N184–25) according to the manufacturer’s instructions.
The antigenicity of the rVP6 and dl2/6-VLPs was evaluated by ELISA, where equal quantities of VP6 antigen (0 to 1 μg/ml PBS) in both protein preparations were coated to Costar High Binding 96-well half area polystyrene plates (Corning Inc., Cat. 3690). Human polyclonal anti RV serum originating from a patient with RV infection was added to the wells (1:200 dilution) and binding of the antibodies was detected with 1:4000 diluted horseradish peroxidase (HRP)-conjugated anti-human IgG (Invitrogen, Cat. 627120) and SIGMA FAST o-phenylenediamine dihydrochloride (OPD) substrate (Sigma-Aldrich, Cat. P9187–50SET). Absorbance (OD) at 490 nm was measured by Victor2 1420 microplate reader (Perkin Elmer, Waltham, MA).
Preparation of RV cell culture antigens
In order to use RVs as antigens in ELISA and IFN-γ ELISPOT assay, MA104 cells were infected with the human RV strains Wa, SC2, BrB, 69M, L26, bovine WC3, and rhesus RV as described elsewhere.Citation50 shows the classification of the RV strains used. After observing maximum cytopathic effect (3–4 d respectively), the VP6 amount of the virus cultures was determined by Ridascreen® Rotavirus kit (R-Biopharm AG, Cat. C0901) using the internal insect cell derived rVP6 standard and expressed in ng/ml. RV cell culture antigens were diluted in PBS to contain equal quantities of VP6 protein per each culture prior to using in the ELISA or ELISPOT assays.
Table 1. Classification of human and animal rotaviruses according to the different genome segments
Immunization of mice and sample collection
Female 7–9-week-old BALB/c OlaHsd mice (3–5 mice/experimental group) obtained from Harlan Laboratories (Horst, Netherlands) were immunized IM with 3 μg or 10 μg of the VP6 or dl2/6-VLPs, at weeks 0 and 3. The doses of dl2/6-VLPs were calculated based on the amount of VP6 protein in the preparation and not the total protein concentration. Naïve mice receiving carrier only (sterile PBS) were used as controls. No external adjuvants were used at any immunization point.
Tail blood samples (diluted 1:200 at the time of collection) and feces were collected at weeks 0 (pre-immune sample) and 3. At week 5 mice were euthanized and feces, whole blood, VWs, and lymphoid tissues were collected. Fresh feces of mice were pooled groupwise and 10% fecal suspension in 10 mM Tris (Cat. T1378) buffer containing 100 mM NaCl (Cat. 31434N), 1mM CaCl2 (Cat. C5670), 0.02% Tween (Cat. P1379), 1% aprotinin (Cat. A6279), and 10 μM leupeptine (Cat. L2884) (all from Sigma-Aldrich) were prepared as previously described.Citation33 Blood samples and a single-cell suspension from the spleen of each mouse were prepared according to Tamminen et al.Citation52 Vaginal lavages were collected by washing twice with 125 μl of cold PBS followed by a centrifugation at 12,000 × g for 10 min at +4°C. The VWs were pooled groupwise and stored at –20°C. All procedures were performed according to the regulations and guidelines of the Finnish Animal Experiment Board.
RV specific IgG and IgA detection from serum
Sera of each mouse at 1:200 dilution or 2-fold dilution series were tested for total IgG and IgG subtype antibodies by ELISA assays as previously describedCitation33 with minor modifications. Briefly, Costar High Binding 96-well half area polystyrene plates were coated with 40 ng/well of rVP6 protein in PBS. The bound VP6-specific IgGs were detected with HRP-conjugated goat anti-mouse IgG (Sigma-Aldrich, Cat. A4416), IgG1 (Invitrogen, Cat. A10551) or IgG2a (Invitrogen, Cat. A10685). A sample was considered positive if the OD was above the set cut-off value calculated as follows: mean OD (termination sera of control mice) + 3 × standard deviation (SD).Citation33 The cut-off value never exceeded OD 0.15. End-point serum titers were expressed as log10 of the reciprocal of the highest serum dilution giving a positive reading.
Sandwich ELISACitation33 was used for the detection of serum VP6-specific IgA antibodies. The plates were coated with polyclonal rabbit anti-rotavirus antibody at 1 μg/ml (DakoCytomation, Cat. B0218) followed by the addition of rVP6 (50 ng/well). Antibodies in sera (at dilutions of 1:2 and 1:5) were detected with goat anti-mouse IgA-HRP (Sigma-Aldrich, Cat. A4589).
Detection of serum cross-reactive RV-specific IgG antibodies
Cross-reactive RV VP6-specific IgG serum antibodies were detected with the sandwich ELISA as described above but the plates were coated with RV cell culture antigens (homologous, Wa; and heterologous, SC2, BrB, 69M, L26, WC3, and RRV) at the VP6 antigen concentration of 100 ng/ml and serum dilution of 1:200 was utilized.
RV specific IgG and IgA detection from mucosal samples
For the detection of mucosal VP6-specific antibodies the sandwich ELISA was used as described above. Stool suspensions and VWs were used at dilutions of 1:2 and 1:5.
Cell-mediated immune response detection
VP6-specific T cell responses were measured with ELISPOT assay by quantification of IFN-γ production from splenocytes.Citation52 Multiscreen 96-well HTS-IP filter plates (Millipore, Cat. MSIPN4W50) were coated with anti-mouse IFN-γ monoclonal antibody AN18 (Mabtech AB, Cat. 3321-3-1000) at 2.5 μg/ml. Splenocytes (0.1 × 106 cells/well) from the immunized or control mice were stimulated in duplicates with Wa, BrB or WC3 RV cultures (500 ng VP6 antigen/ml) or 5 μg/ml of a synthetic VP6 derived 18-mer peptide (Proimmune Ltd., Oxford, United Kingdom), previously identified as an VP6-specific BALB/c mouse CD4+ T cell epitope (DGATTWYFNPVILRPNNV, AA242–259),Citation24 named R6–2. MA104 mock cell culture and synthetic norovirus capsid-derived peptide (CLLPQEWIQHLYQES) were used as negative controls. Cells incubated in culture media alone and cells stimulated with 10 μg/ml of concanavalin A (ConA, Sigma-Aldrich, Cat. C5275), a T cell mitogen, served as background and viability controls. After overnight incubation at 37°C cytokine secretion was detected with biotinylated anti-mouse IFN-γ monoclonal antibody R4–6A2 (Mabtech AB, Cat. 3321–6-1000) at 2 μg/ml and streptavidin-ALP (Mabtech AB, Cat. 3310–10) at 1:500. The spots developed with BCIP/NBT substrate (Mabtech, Cat. 3650–10) were counted by ImmunoSpot® automatic CTL analyzer (CTL-Europe GmbH, Bonn, Germany). The results were expressed as mean spot forming cells (SFC)/106 splenocytes of duplicate wells.
In order to determine cell type responsible for the IFN-γ production, splenocytes were preincubated for 1 h at 37°C with the functional blocking antibodies (rat anti mouse-CD4 (Cat. 16-0041) and rat anti-mouse CD8a (Cat. 16-0081) from eBioscience) or rat IgG isotype control antibody (eBioscience, Cat. 16-4321) at 30 μg/ml concentration prior to stimulation with the peptide or RV cell culture antigens.
Inhibition of RV infection in vitro
Neutralizing activity of VP6-specific sera, fecal samples, and VWs was determined by reduction in RV antigen production using an ELISA-based antigen reduction neutralization assay (NELISA) as described previously by others.Citation53,Citation54 In each assay the samples of immunized and control mice from the same time point (week 5) were always run simultaneously. 2-fold dilutions (from 1:10 to 1:640) of each sample were equally mixed with Wa RV and RRV containing 250 focus-forming units and incubated at 37°C for 60 min. The mixture was overlaid to confluent MA104 cell monolayers in 96-well plates and the plates were spun (1000 × g) for 60 min. The virus inoculum was replaced with medium containing trypsin (Sigma-Aldrich, Cat. T4799) at 4 µg/ml followed by incubation at 37°C for 15h. The cells were lysed by one cycle of freeze and thaw and the plates were stored at –80°C. RV VP6 antigen production in each sample performed in duplicates was measured by a Ridascreen® Rotavirus test as described above. A reduction in 450 nm OD value greater than 60% compared with the positive control wells (trypsin activated RV Wa or RRV without the test sample) was considered to indicate neutralization. Neutralizing titers were expressed as the reciprocal of the highest sample dilution yielding neutralization.
Statistical analyses
To compare the intergroup differences in the VP6 responses Mann-Whitney U-test was used. Statistical analyses were conducted using IBM SPSS Statistics (SPSS Inc., Chicago, IL) version 19.0, where p < 0.05 was considered to be statistically significant.
Abbreviations: | ||
RV | = | rotavirus |
N-Abs | = | neutralizing antibodies |
BV | = | baculovirus |
dl | = | double-layered |
VLP | = | virus-like particle |
tl | = | triple-layered |
Th | = | T-helper |
IFN-γ | = | interferon–γ |
dpi | = | days post infection |
SDS-PAGE | = | sodium dodecyl sulphate polyacrylamide gel electrophoresis |
EM | = | electron microscopy |
ELISA | = | enzyme-linked immunosorbent assay |
IM | = | intramuscular |
OD | = | optical density |
VW | = | vaginal wash |
ELISPOT | = | enzyme-linked immunosorbent spot assay |
DC | = | dendritic cell |
MOI | = | multiplicity of infection |
HRP | = | horseradish peroxidase |
SFC | = | spot forming cells |
Additional material
Download Zip (119.1 KB)Acknowledgments
We gratefully acknowledge the technical assistance given by the laboratory personnel of the Vaccine Research Center of University of Tampere Medical School. Special thanks are due to Eeva Jokela and Marianne Karlsberg for their contribution to the laboratory methods. We are also grateful to Mervi Lindman and Helena Vihinen of the Institute of Biotechnology (University of Helsinki) for their guidance and technical help in transmission electron microscopy. This work has not received any funding.
Disclosure of Potential Conflicts of Interest
No potential conflicts of interest were disclosed.
Supplemental Materials
Supplemental materials may be found here: www.landesbioscience.com/journals/vaccines/article/25249
References
- Parez N. Rotavirus gastroenteritis: why to back up the development of new vaccines?. Comp Immunol Microbiol Infect Dis 2008; 31:253 - 69; http://dx.doi.org/10.1016/j.cimid.2007.07.005; PMID: 17698192
- Franco MA, Angel J, Greenberg HB. Immunity and correlates of protection for rotavirus vaccines. Vaccine 2006; 24:2718 - 31; http://dx.doi.org/10.1016/j.vaccine.2005.12.048; PMID: 16446014
- Labbé M, Charpilienne A, Crawford SE, Estes MK, Cohen J. Expression of rotavirus VP2 produces empty corelike particles. J Virol 1991; 65:2946 - 52; PMID: 1851866
- Prasad BV, Wang GJ, Clerx JP, Chiu W. Three-dimensional structure of rotavirus. J Mol Biol 1988; 199:269 - 75; http://dx.doi.org/10.1016/0022-2836(88)90313-0; PMID: 2832610
- Matthijnssens J, Ciarlet M, Heiman E, Arijs I, Delbeke T, McDonald SM, et al. Full genome-based classification of rotaviruses reveals a common origin between human Wa-Like and porcine rotavirus strains and human DS-1-like and bovine rotavirus strains. J Virol 2008; 82:3204 - 19; http://dx.doi.org/10.1128/JVI.02257-07; PMID: 18216098
- Kapikian AZ, Hoshino Y, Chanock RM. Rotaviruses. In: Knipe DM, Howley PM, eds. Fields Virology. 4th Edn. Philadelphia: Lippincott Williams & Wilkins, 2001:1787-833.
- Tang B, Gilbert JM, Matsui SM, Greenberg HB. Comparison of the rotavirus gene 6 from different species by sequence analysis and localization of subgroup-specific epitopes using site-directed mutagenesis. Virology 1997; 237:89 - 96; http://dx.doi.org/10.1006/viro.1997.8762; PMID: 9344910
- Estes MK, Crawford SE, Penaranda ME, Petrie BL, Burns JW, Chan WK, et al. Synthesis and immunogenicity of the rotavirus major capsid antigen using a baculovirus expression system. J Virol 1987; 61:1488 - 94; PMID: 3033276
- Ishida S, Feng N, Tang B, Gilbert JM, Greenberg HB. Quantification of systemic and local immune responses to individual rotavirus proteins during rotavirus infection in mice. J Clin Microbiol 1996; 34:1694 - 700; PMID: 8784572
- Angel J, Franco MA, Greenberg HB. Rotavirus vaccines: recent developments and future considerations. Nat Rev Microbiol 2007; 5:529 - 39; http://dx.doi.org/10.1038/nrmicro1692; PMID: 17571094
- Lepault J, Petitpas I, Erk I, Navaza J, Bigot D, Dona M, et al. Structural polymorphism of the major capsid protein of rotavirus. EMBO J 2001; 20:1498 - 507; http://dx.doi.org/10.1093/emboj/20.7.1498; PMID: 11285214
- Crawford SE, Labbé M, Cohen J, Burroughs MH, Zhou YJ, Estes MK. Characterization of virus-like particles produced by the expression of rotavirus capsid proteins in insect cells. J Virol 1994; 68:5945 - 52; PMID: 8057471
- Centers for Disease Control and Prevention. Statement regarding Rotarix® and RotaTeq® rotavirus vaccines and intussusception. Available from: http://www.cdc.gov/vaccines/vpd-vac/rotavirus/intussusception-studies-acip.htm [accessed 20.8.2012].
- McNeal MM, Rae MN, Conner ME, Ward RL. Stimulation of local immunity and protection in mice by intramuscular immunization with triple- or double-layered rotavirus particles and QS-21. Virology 1998; 243:158 - 66; http://dx.doi.org/10.1006/viro.1998.9060; PMID: 9527925
- McNeal MM, Rae MN, Bean JA, Ward RL. Antibody-dependent and -independent protection following intranasal immunization of mice with rotavirus particles. J Virol 1999; 73:7565 - 73; PMID: 10438846
- Ciarlet M, Crawford SE, Barone C, Bertolotti-Ciarlet A, Ramig RF, Estes MK, et al. Subunit rotavirus vaccine administered parenterally to rabbits induces active protective immunity. J Virol 1998; 72:9233 - 46; PMID: 9765471
- O’Neal CM, Crawford SE, Estes MK, Conner ME. Rotavirus virus-like particles administered mucosally induce protective immunity. J Virol 1997; 71:8707 - 17; PMID: 9343229
- Bertolotti-Ciarlet A, Ciarlet M, Crawford SE, Conner ME, Estes MK. Immunogenicity and protective efficacy of rotavirus 2/6-virus-like particles produced by a dual baculovirus expression vector and administered intramuscularly, intranasally, or orally to mice. Vaccine 2003; 21:3885 - 900; http://dx.doi.org/10.1016/S0264-410X(03)00308-6; PMID: 12922123
- Choi AH, Basu M, McNeal MM, Clements JD, Ward RL. Antibody-independent protection against rotavirus infection of mice stimulated by intranasal immunization with chimeric VP4 or VP6 protein. J Virol 1999; 73:7574 - 81; PMID: 10438847
- Choi AH, McNeal MM, Basu M, Flint JA, Stone SC, Clements JD, et al. Intranasal or oral immunization of inbred and outbred mice with murine or human rotavirus VP6 proteins protects against viral shedding after challenge with murine rotaviruses. Vaccine 2002; 20:3310 - 21; http://dx.doi.org/10.1016/S0264-410X(02)00315-8; PMID: 12213401
- Herrmann JE, Chen SC, Fynan EF, Santoro JC, Greenberg HB, Wang S, et al. Protection against rotavirus infections by DNA vaccination. J Infect Dis 1996; 174:Suppl 1 S93 - 7; http://dx.doi.org/10.1093/infdis/174.Supplement_1.S93; PMID: 8752297
- Chen SC, Jones DH, Fynan EF, Farrar GH, Clegg JC, Greenberg HB, et al. Protective immunity induced by oral immunization with a rotavirus DNA vaccine encapsulated in microparticles. J Virol 1998; 72:5757 - 61; PMID: 9621034
- Esquivel FR, Lopez S, Guitierrez-X L, Arias C. The internal rotavirus protein VP6 primes for an enhanced neutralizing antibody response. Arch Virol 2000; 145:813 - 25; http://dx.doi.org/10.1007/s007050050674; PMID: 10893159
- McNeal MM, Basu M, Bean JA, Clements JD, Choi AH, Ward RL. Identification of an immunodominant CD4+ T cell epitope in the VP6 protein of rotavirus following intranasal immunization of BALB/c mice. Virology 2007; 363:410 - 8; http://dx.doi.org/10.1016/j.virol.2007.01.041; PMID: 17337285
- Choi AH, Basu M, McNeal MM, Flint J, VanCott JL, Clements JD, et al. Functional mapping of protective domains and epitopes in the rotavirus VP6 protein. J Virol 2000; 74:11574 - 80; http://dx.doi.org/10.1128/JVI.74.24.11574-11580.2000; PMID: 11090155
- McNeal MM, VanCott JL, Choi AH, Basu M, Flint JA, Stone SC, et al. CD4 T cells are the only lymphocytes needed to protect mice against rotavirus shedding after intranasal immunization with a chimeric VP6 protein and the adjuvant LT(R192G). J Virol 2002; 76:560 - 8; http://dx.doi.org/10.1128/JVI.76.2.560-568.2002; PMID: 11752147
- Zhou H, Guo L, Wang M, Qu J, Zhao Z, Wang J, et al. Prime immunization with rotavirus VLP 2/6 followed by boosting with an adenovirus expressing VP6 induces protective immunization against rotavirus in mice. Virol J 2011; 8:3; http://dx.doi.org/10.1186/1743-422X-8-3; PMID: 21205330
- Lee S, Belitsky BR, Brinker JP, Kerstein KO, Brown DW, Clements JD, et al. Development of a Bacillus subtilis-based rotavirus vaccine. Clin Vaccine Immunol 2010; 17:1647 - 55; http://dx.doi.org/10.1128/CVI.00135-10; PMID: 20810679
- Schwartz-Cornil I, Benureau Y, Greenberg H, Hendrickson BA, Cohen J. Heterologous protection induced by the inner capsid proteins of rotavirus requires transcytosis of mucosal immunoglobulins. J Virol 2002; 76:8110 - 7; http://dx.doi.org/10.1128/JVI.76.16.8110-8117.2002; PMID: 12134016
- González SA, Affranchino JL. Assembly of double-layered virus-like particles in mammalian cells by coexpression of human rotavirus VP2 and VP6. J Gen Virol 1995; 76:2357 - 60; http://dx.doi.org/10.1099/0022-1317-76-9-2357; PMID: 7561777
- Finkelman FD, Holmes J, Katona IM, Urban JF Jr., Beckmann MP, Park LS, et al. Lymphokine control of in vivo immunoglobulin isotype selection. Annu Rev Immunol 1990; 8:303 - 33; http://dx.doi.org/10.1146/annurev.iy.08.040190.001511; PMID: 1693082
- Ruggeri FM, Johansen K, Basile G, Kraehenbuhl JP, Svensson L. Antirotavirus immunoglobulin A neutralizes virus in vitro after transcytosis through epithelial cells and protects infant mice from diarrhea. J Virol 1998; 72:2708 - 14; PMID: 9525588
- Blazevic V, Lappalainen S, Nurminen K, Huhti L, Vesikari T. Norovirus VLPs and rotavirus VP6 protein as combined vaccine for childhood gastroenteritis. Vaccine 2011; 29:8126 - 33; http://dx.doi.org/10.1016/j.vaccine.2011.08.026; PMID: 21854823
- Ruiz-Palacios GM, Pérez-Schael I, Velázquez FR, Abate H, Breuer T, Clemens SC, et al, Human Rotavirus Vaccine Study Group. Safety and efficacy of an attenuated vaccine against severe rotavirus gastroenteritis. N Engl J Med 2006; 354:11 - 22; http://dx.doi.org/10.1056/NEJMoa052434; PMID: 16394298
- Vesikari T, Matson DO, Dennehy P, Van Damme P, Santosham M, Rodriguez Z, et al, Rotavirus Efficacy and Safety Trial (REST) Study Team. Safety and efficacy of a pentavalent human-bovine (WC3) reassortant rotavirus vaccine. N Engl J Med 2006; 354:23 - 33; http://dx.doi.org/10.1056/NEJMoa052664; PMID: 16394299
- Bachmann MF, Rohrer UH, Kündig TM, Bürki K, Hengartner H, Zinkernagel RM. The influence of antigen organization on B cell responsiveness. Science 1993; 262:1448 - 51; http://dx.doi.org/10.1126/science.8248784; PMID: 8248784
- Fifis T, Gamvrellis A, Crimeen-Irwin B, Pietersz GA, Li J, Mottram PL, et al. Size-dependent immunogenicity: therapeutic and protective properties of nano-vaccines against tumors. J Immunol 2004; 173:3148 - 54; PMID: 15322175
- Keller SA, Bauer M, Manolova V, Muntwiler S, Saudan P, Bachmann MF. Cutting edge: limited specialization of dendritic cell subsets for MHC class II-associated presentation of viral particles. J Immunol 2010; 184:26 - 9; http://dx.doi.org/10.4049/jimmunol.0901540; PMID: 19949081
- Ghosh MK, Dériaud E, Saron MF, Lo-Man R, Henry T, Jiao X, et al. Induction of protective antiviral cytotoxic T cells by a tubular structure capable of carrying large foreign sequences. Vaccine 2002; 20:1369 - 77; http://dx.doi.org/10.1016/S0264-410X(01)00467-4; PMID: 11818155
- Wilkinson TM, Li CK, Chui CS, Huang AK, Perkins M, Liebner JC, et al. Preexisting influenza-specific CD4+ T cells correlate with disease protection against influenza challenge in humans. Nat Med 2012; 18:274 - 80; http://dx.doi.org/10.1038/nm.2612; PMID: 22286307
- Burns JW, Siadat-Pajouh M, Krishnaney AA, Greenberg HB. Protective effect of rotavirus VP6-specific IgA monoclonal antibodies that lack neutralizing activity. Science 1996; 272:104 - 7; http://dx.doi.org/10.1126/science.272.5258.104; PMID: 8600516
- Sabara M, Gilchrist JE, Hudson GR, Babiuk LA. Preliminary characterization of an epitope involved in neutralization and cell attachment that is located on the major bovine rotavirus glycoprotein. J Virol 1985; 53:58 - 66; PMID: 2578197
- Gualtero DF, Guzmán F, Acosta O, Guerrero CA. Amino acid domains 280-297 of VP6 and 531-554 of VP4 are implicated in heat shock cognate protein hsc70-mediated rotavirus infection. Arch Virol 2007; 152:2183 - 96; http://dx.doi.org/10.1007/s00705-007-1055-5; PMID: 17876681
- Garaicoechea L, Olichon A, Marcoppido G, Wigdorovitz A, Mozgovoj M, Saif L, et al. Llama-derived single-chain antibody fragments directed to rotavirus VP6 protein possess broad neutralizing activity in vitro and confer protection against diarrhea in mice. J Virol 2008; 82:9753 - 64; http://dx.doi.org/10.1128/JVI.00436-08; PMID: 18632867
- Aladin F, Einerhand AW, Bouma J, Bezemer S, Hermans P, Wolvers D, et al. In vitro neutralisation of rotavirus infection by two broadly specific recombinant monovalent llama-derived antibody fragments. PLoS One 2012; 7:e32949; http://dx.doi.org/10.1371/journal.pone.0032949; PMID: 22403728
- Butler JE, Heo Y, Adams P, Richerson HB. The antigen-limited nature of microtiter ELISAs requires partial depletion of IgG to permit reliable determination of rabbit serum IgA antibody activity. Mol Immunol 1990; 27:319 - 25; http://dx.doi.org/10.1016/0161-5890(90)90044-Z; PMID: 2359410
- Jauhiainen T, Tuomi T, Leinonen M, Kark JD, Saikku P. Interference of immunoglobulin G (IgG) antibodies in IgA antibody determinations of Chlamydia pneumoniae by microimmunofluorescence test. J Clin Microbiol 1994; 32:839 - 40; PMID: 8195403
- Coffin SE, Clark SL, Bos NA, Brubaker JO, Offit PA. Migration of antigen-presenting B cells from peripheral to mucosal lymphoid tissues may induce intestinal antigen-specific IgA following parenteral immunization. J Immunol 1999; 163:3064 - 70; PMID: 10477570
- Corthésy B, Benureau Y, Perrier C, Fourgeux C, Parez N, Greenberg H, et al. Rotavirus anti-VP6 secretory immunoglobulin A contributes to protection via intracellular neutralization but not via immune exclusion. J Virol 2006; 80:10692 - 9; http://dx.doi.org/10.1128/JVI.00927-06; PMID: 16956954
- Fromantin C, Jamot B, Cohen J, Piroth L, Pothier P, Kohli E. Rotavirus 2/6 virus-like particles administered intranasally in mice, with or without the mucosal adjuvants cholera toxin and Escherichia coli heat-labile toxin, induce a Th1/Th2-like immune response. J Virol 2001; 75:11010 - 6; http://dx.doi.org/10.1128/JVI.75.22.11010-11016.2001; PMID: 11602741
- Matthijnssens J, Rahman M, Martella V, Xuelei Y, De Vos S, De Leener K, et al. Full genomic analysis of human rotavirus strain B4106 and lapine rotavirus strain 30/96 provides evidence for interspecies transmission. J Virol 2006; 80:3801 - 10; http://dx.doi.org/10.1128/JVI.80.8.3801-3810.2006; PMID: 16571797
- Tamminen K, Huhti L, Koho T, Lappalainen S, Hytönen VP, Vesikari T, et al. A comparison of immunogenicity of norovirus GII-4 virus-like particles and P-particles. Immunology 2012; 135:89 - 99; http://dx.doi.org/10.1111/j.1365-2567.2011.03516.x; PMID: 22044070
- Knowlton DR, Spector DM, Ward RL. Development of an improved method for measuring neutralizing antibody to rotavirus. J Virol Methods 1991; 33:127 - 34; http://dx.doi.org/10.1016/0166-0934(91)90013-P; PMID: 1658027
- Ward RL, Kapikian AZ, Goldberg KM, Knowlton DR, Watson MW, Rappaport R. Serum rotavirus neutralizing-antibody titers compared by plaque reduction and enzyme-linked immunosorbent assay-based neutralization assays. J Clin Microbiol 1996; 34:983 - 5; PMID: 8815124