Abstract
There has long been interest and demand for the development of a vaccine to prevent infections caused by the Gram-positive organism group A streptococcus. Despite numerous efforts utilizing advanced approaches such as genomics, proteomics and bio-informatics, there is currently no vaccine. Here we review various strategies employed to achieve this goal. We also discuss the approach that we have pursued, a non-host reactive, conformationally constrained minimal B cell epitope from within the C-repeat region of M-protein, and the potential limitations in moving forward.
Introduction
The history of the development of vaccines to prevent group A streptococcus (GAS) infections is one with many promises and many disappointments. It is one where, nevertheless, proponents have passionately progressed the needs for such a vaccine and where many novel approaches and strategies have been tested. Today, however, there is no vaccine.
Industry has flirted with the idea of a vaccine as they have weighed the plusses, from a commercial perspective, of a vaccine to prevent streptococcal tonsillitis, with the risks that any vaccine may actually cause disease. Rheumatic fever (RF) and rheumatic heart disease (RHD) are autoimmune conditions that can follow infections with GAS (see below) and immunizing children with streptococcal antigens and adjuvants may thus be a bridge too far for industry. Still, the need exists. A conservative estimate of the deaths due to GAS infections and the autoimmune complications that can follow, principally RHD, is 500 000 per year.Citation1 However, in many countries, RF is poorly diagnosed or ignored as a possible cause of illness. In many African countries, for example, a child presenting with fever and lethargy is considered to have malaria and is usually treated as such since blood parasitemia is highly prevalent and often not considered informative to the diagnosis. RF can thus be missed and the consequences can be dire. Thus, the true incidence of GAS pathology may be much higher.
GAS causes death principally by deep tissue sepsis and rheumatic heart disease. However, it is estimated that there are over 600 million cases of non-life threatening pharyngitis annually and over 100 million cases of pyoderma annually. If untreated, pharyngitis and possibly also pyoderma,Citation2 can progress to RF and RHD-conditions where GAS cannot be found in the affected tissues and where pathology occurs as a result of mis-directed immune responses that originated from the preceding GAS infection but which now are recognizing human tissue, such as heart valves. It is thus critical that any vaccine approach minimizes the possibility of inducing auto-reactive B and T cell responses. This is an obvious impediment to vaccine research, particularly since in an early study using a crude antigen prepared from GAS organisms, three “at-risk” individuals developed RHD.Citation3 However, this is in contrast to other studies where GAS was administered with no reported serious adverse events.Citation4,Citation5
In 1979, the FDA regulated the exclusion of GAS from vaccine products after reviewing and considering the findings of the independent advisory Panel on “Review of Bacterial Vaccines and Bacterial Antigens.”Citation6 This exclusion was lifted in 2006.Citation7
A further significant impediment to vaccine development is the fact that there is significant antigenic strain variation with GAS. Immunity to any particular strain is thought to be due to the development of antibodies to the N-terminal segment of the surface M protein, a virulence factor for the organism.Citation8 These strain-specific antibodies can neutralize the pathogenic effects of the M protein, but they can only act against the specific strain that induced them. Unfortunately, there are over 180 different emm types, based on nucleotide sequences of the M protein-encoding gene.Citation9,Citation10 This enormous sequence diversity has led to two separate strategies—the current leading strategy of combining multiple common serotypic determinants into recombinant polypeptidesCitation11-Citation13 and other strategies of using either conserved regions of the M protein or conserved non M proteins or fragments thereof as vaccine candidates.Citation14-Citation17
While the M protein, being a major virulence factor, is an obvious target for vaccine consideration, non-M protein vaccine candidates have also been identified (such as virulence factors including proteasesCitation18,Citation19 or adhesinsCitation20) or by ‘reverse vaccinology’ approaches of using genomics, proteomics and immunomics to identify conserved, surface expressed molecules recognized by serum from infected individuals.Citation21-Citation23
Discovery of a Cryptic Vaccine Epitope
Our approach has been to identify a concealed epitope on the M-protein that is poorly recognized in the human host but which can nevertheless lead to the induction of antibodies that can recognize and destroy GAS. We believe that an advantage of our approach is that hidden or ‘cryptic’ epitopes are highly conserved, as they are not under immune pressure. The surprising factor is, though, that although cryptic epitopes may not be recognized as a result of infection, antibodies induced against cryptic epitopes in isolation may recognize the organism. The cryptic epitope that has most interested us is contained within a 28-amino acid peptide referred to as “J8” as described later. The central 12 amino acids of J8 are GAS-derived (from the “C3” repeat region of the M protein—a region almost buried in the capsule of the organism). The flanking sequences are designed to enable the 12 amino acid critical GAS sequence to maintain its α-helical folding, which is required for its antigenicity. The strategy to identify this cryptic epitope may be applicable to vaccine development for other organisms.Citation24,Citation25
Our specific approach was to focus on the carboxyl-terminal region of the M protein, a major virulence factor for the organism. There was evidence that this relatively large but highly conserved carboxyl-terminal segment of the protein was capable of inducing protection in a mucosal challenge model.Citation26 It was curious, however, that antibodies to the region were shown by others to be non-opsonic,Citation27,Citation28 in contrast to antibodies to the serotypic N-terminal segment. Our initial goal was to ask whether antibodies to much smaller peptide fragments within this region might be opsonic. We thus produced a panel of nine overlapping 20-mer synthetic peptides spanning the C3 repeat region and asked whether antibodies produced in mice to these individual peptides would kill GAS in vitro in the presence of human neutrophils and complement. This was a standard “Lancefield” indirect opsonophagocytosis assayCitation29 with one important exception, that being that we used ‘stationary’ phase organisms with a pre-incubation step and not ‘log’ phase organisms.Citation30 One peptide in particular, referred to as P145, was capable of inducing antibodies that could opsonize stationary phase organisms. When log phase organisms were used we observed significantly less opsonic activity possibly because of enhanced expression of hyaluronic acid (HA) capsules in log phase organism.Citation31 Moreover, we observed that antibodies to P145 could opsonize multiple serotypic strains of GAS in stationary phase,Citation17,Citation32 in keeping with the conserved nature of this region. This peptide then formed the basis of our on-going approach to vaccine development.
We observed that the peptide was cryptic. Thus only 30% of Aboriginal children living in a highly streptococcal-endemic area of northern Australia had P145-specific antibodies, although this rose to 90% by adulthood.Citation33 It thus seemed that many years of streptococcal exposure were required for the development of this immune response. However, once developed, these human antibodies were capable of killing the organisms similarly to the murine antibodies induced by peptide vaccination. More recently we have shown in the mouse model that sequential exposure to multiple GAS strains does not induce these antibodies (M Pandey et al., unpublished). Given the very poor immunogenicity of P145 in its native state, it was not surprising to us that P145 is highly conserved. At the time of our initial studies the data suggested that it was completely conserved within different strains although recent examination of hundreds of different strains has shown that there are minor variants of P145,Citation34 which are cross-reactive.Citation35
Vaccine Safety
As mentioned, GAS infection, if untreated, can lead to the serious autoimmune disease, rheumatic fever (RF), in a small subset of the population (about 3%).Citation36 Evidences suggest that streptococcal specific antibodies and T cells may contribute to this pathology.Citation37-Citation40 In the case of RHD it has been suggested that antibodies to the streptococcal sugars damage the endothelial surface of the heart valves enabling the ingress of streptococcal-specific immune cells, including CD4 and CD8 T cellsCitation41-Citation43 which are activated by cross-reactive cardiac antigens leading to inflammation and scarring.Citation40,Citation44 T cells taken from the excised heart valves of acute RF patients have been analyzed for specificity and some have been shown to respond to epitopes from the M protein,Citation45,Citation46 raising potential concerns that vaccines containing those epitopes may induce pathology. However, there is no reason to believe that any streptococcal antigen could not be involved in pathogenesis of RHD assuming it was cross-reactive with heart epitopes. Thus, safety concerns span all streptococcal vaccine candidates. However, while heart cross-reactive T cell clones have been generated from the peripheral blood of RHD patients, clones have also been generated from the blood of individuals with no evidence of heart pathology.Citation47 The functionality of the T cells is likely to be as important as their specificity and so far only limited analyses have been performed on T cells isolated from RHD valve specimens.Citation48
The above observations raise concerns regarding the safety of any streptococcal vaccine. The approach that we undertook to minimize the risk of a vaccine based on P145 inducing pathology was to define a minimal B cell epitope (capable of inducing protective antibodies) while not stimulating T cells. We defined a 12-mer epitope within P145, referred to as J8i, that was a B cell epitope but did not stimulate T cells in the different mouse strains that we examined.Citation17 Conversely, other minimal epitopes within P145, principally J2i, stimulated T cells but were not recognized by monoclonal antibodies generated against P145. J8i thus became the focus of vaccine design. However, unfortunately J8i was too small to maintain its helical structure which was required for its antigenicity and as such a technology was developed to fold J8i as a helixCitation49 resulting in J8, a 28-mer synthetic peptide of which only the central 12 amino acids (J8i) are derived from GAS sequences, with the flanking sequences derived from a non-streptococcal peptide. The sequence and structure of J8 is illustrated in .
Figure 1. Overlapping peptides synthesized from the parent peptide P145. Sequences derived from the M protein of group A streptococcus are underlined. Flanking sequences (not underlined) are derived from the yeast DNA binding protein GCN4 (A). A graphic representation of vaccine candidate J8-DT with J8 peptide conjugated to diphtheria toxoid (DT) molecule. A ratio of DT: J8 (1:8–14 molecules) (B).
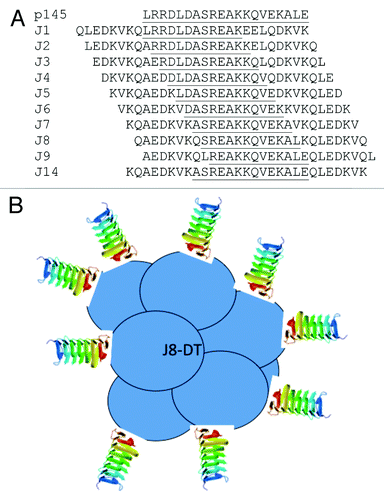
To render J8 immunogenic in an outbred population, it was then conjugated to diphtheria toxoid, giving the vaccine the name, “J8-DT”. This vaccine generates specific memory B cells (MBC) and long-lasting Ab responsesCitation50 which prevent streptococcal sepsis in mice.Citation51 T cell help is required for activation of MBC but can be provided by naive T cells responding directly to GAS at the time of infection (). Thus individuals whose T cells do not recognize the short synthetic peptide in the vaccine will be able to generate a protective and rapid memory Ab response at the time of infection. The vaccine can also prevent GAS pyoderma in an animal model that we developed to closely mimic human GAS pyoderma (M Pandey, unpublished). J8, when embedded in protein vesicles (without DT)Citation52 or presented as lipopeptidesCitation53 can also induce salivary IgA and protection from intranasal GAS challenge. Using the rat valvulitis model referred to above, J8 has been shown not to induce pathology (M Batzloff et al., unpublished).
Figure 2. Adoptive transfer and assessment of protective efficacy of J8-DT memory and naïve B and/or T cells: To investigate synergistic effect of memory and naïve B and T cells in protection against GAS, SCID mice (n = 5 per group) were transfused with combinations of B and T cells from J8-DT-immunized or naïve BALB/c mice. Cohorts of mice were transfused with J8-DT B cells/naive T cells, naïve B/J8-DT T cells, J8-DT B and T cells or naive B and T cells, J8-DT or naïve splenocytes or J8-DT B/T cells. Immunized mice received the vaccine 14–16 weeks before their cells were transferred. Twenty-four h post-adoptive transfer, the mice received an IV infection with 50 000 CFU of GAS M1 strain. The bacterial bio-burden in the blood was monitored and bacteremia on day 12 post-infection is shown. Results are shown as means ± standard errors of the means for at least 5 mice in each group. Significance determined by two-way analysis of variance (ANOVA) where **p < 0.01, ***p < 0.001. (Fig. adapted with permission from Pandey et al.; 2013Citation50)
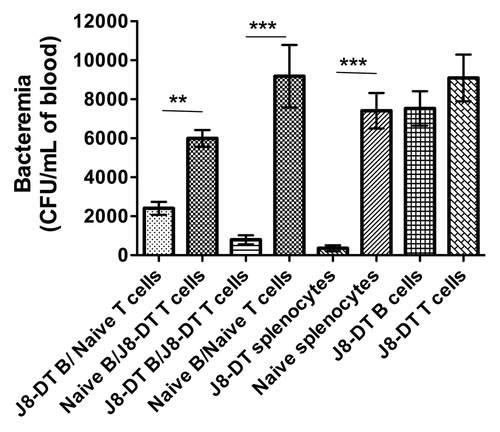
Way Forward and Summary
As mentioned, there has not been consistent industry support for the development of GAS vaccines. Thus development will depend on funding from Government and NGOs. It is thus pleasing to note that the New Zealand and Australian Governments have pledged significant funding to complete pre-clinical evaluation of three leading candidates (M protein based and non-M protein based), with the goal of taking the most promising candidates through to clinical trials. A major motivating force for these Governments is the very high incidence of streptococcal-related pathology within the indigenous populations of these two countries.
In summary, attempts to develop a vaccine to prevent GAS infections are not new, but as more is learnt about strain diversity and as novel concepts are developed to identify new antigens and present them to the immune system in a safe manner it is likely that an efficacious vaccine (or vaccines) will be developed in the near future.
Acknowledgments
This work was supported by the National Heart Foundation of Australia, National Health and Medical Research Council (Australia), the Prince Charles Hospital foundation, the Cooperative Research Centre for vaccine Technology, the National Institute of Health (USA) and the Cooperative Research Centre for Aboriginal Health.
Disclosure of Potential Conflicts of Interest
No potential conflicts of interest were disclosed.
References
- Carapetis JR, Steer AC, Mulholland EK, Weber M. The global burden of group A streptococcal diseases. Lancet Infect Dis 2005; 5:685 - 94; http://dx.doi.org/10.1016/S1473-3099(05)70267-X; PMID: 16253886
- McDonald M, Currie BJ, Carapetis JR. Acute rheumatic fever: a chink in the chain that links the heart to the throat?. Lancet Infect Dis 2004; 4:240 - 5; http://dx.doi.org/10.1016/S1473-3099(04)00975-2; PMID: 15050943
- Massell BF, Honikman LH, Amezcua J. Rheumatic fever following streptococcal vaccination. Report of three cases. JAMA 1969; 207:1115 - 9; http://dx.doi.org/10.1001/jama.1969.03150190037007; PMID: 5818242
- D’Alessandri R, Plotkin G, Kluge RM, Wittner MK, Fox EN, Dorfman A, et al. Protective studies with group A streptococcal M protein vaccine. III. Challenge of volunteers after systemic or intranasal immunization with Type 3 or Type 12 group A Streptococcus. J Infect Dis 1978; 138:712 - 8; http://dx.doi.org/10.1093/infdis/138.6.712; PMID: 368261
- Fox EN, Waldman RH, Wittner MK, Mauceri AA, Dorfman A. Protective study with a group A streptococcal M protein vaccine. Infectivity challenge of human volunteers. J Clin Invest 1973; 52:1885 - 92; http://dx.doi.org/10.1172/JCI107372; PMID: 4719668
- Food and Drug Administration, HHS. Revocation of status of specific products; Group A streptococcus. Direct final rule. Fed Regist 2005; 70:72197 - 9; PMID: 16323338
- Food and Drug Administration, HHS. Revocation of status of specific products; Group A streptococcus. Direct final rule. Fed Regist 2005; 70:72197 - 9; PMID: 16323338
- Fischetti VA. Streptococcal M protein: molecular design and biological behavior. Clin Microbiol Rev 1989; 2:285 - 314; PMID: 2670192
- Steer AC, Danchin MH, Carapetis JR. Group A streptococcal infections in children. J Paediatr Child Health 2007; 43:203 - 13; http://dx.doi.org/10.1111/j.1440-1754.2007.01051.x; PMID: 17444820
- Smeesters PR, Mardulyn P, Vergison A, Leplae R, Van Melderen L. Genetic diversity of Group A Streptococcus M protein: implications for typing and vaccine development. Vaccine 2008; 26:5835 - 42; http://dx.doi.org/10.1016/j.vaccine.2008.08.037; PMID: 18789365
- Dale JB, Penfound TA, Chiang EY, Walton WJ. New 30-valent M protein-based vaccine evokes cross-opsonic antibodies against non-vaccine serotypes of group A streptococci. Vaccine 2011; 29:8175 - 8; http://dx.doi.org/10.1016/j.vaccine.2011.09.005; PMID: 21920403
- Gordon DM, McGovern TW, Krzych U, Cohen JC, Schneider I, LaChance R, et al. Safety, immunogenicity, and efficacy of a recombinantly produced Plasmodium falciparum circumsporozoite protein-hepatitis B surface antigen subunit vaccine. J Infect Dis 1995; 171:1576 - 85; http://dx.doi.org/10.1093/infdis/171.6.1576; PMID: 7769295
- Dale JB, Penfound T, Chiang EY, Long V, Shulman ST, Beall B. Multivalent group A streptococcal vaccine elicits bactericidal antibodies against variant M subtypes. Clin Diagn Lab Immunol 2005; 12:833 - 6; PMID: 16002631
- Brandt ER, Hayman WA, Currie B, Pruksakorn S, Good MF. Human antibodies to the conserved region of the M protein: opsonization of heterologous strains of group A streptococci. Vaccine 1997; 15:1805 - 12; http://dx.doi.org/10.1016/S0264-410X(97)00178-3; PMID: 9364687
- Ji YD, Carlson B, Kondagunta A, Cleary PP. Intranasal immunization with C5a peptidase prevents nasopharyngeal colonization of mice by the group A Streptococcus. Infect Immun 1997; 65:2080 - 7; PMID: 9169735
- Zabriskie JB, Poon-King T, Blake MS, Michon F, Yoshinaga M. Phagocytic, serological, and protective properties of streptococcal group A carbohydrate antibodies. Adv Exp Med Biol 1997; 418:917 - 9; PMID: 9331798
- Hayman WA, Brandt ER, Relf WA, Cooper J, Saul A, Good MF. Mapping the minimal murine T cell and B cell epitopes within a peptide vaccine candidate from the conserved region of the M protein of group A streptococcus. Int Immunol 1997; 9:1723 - 33; http://dx.doi.org/10.1093/intimm/9.11.1723; PMID: 9418133
- Kapur V, Maffei JT, Greer RS, Li LL, Adams GJ, Musser JM. Vaccination with streptococcal extracellular cysteine protease (interleukin-1 beta convertase) protects mice against challenge with heterologous group A streptococci. Microb Pathog 1994; 16:443 - 50; http://dx.doi.org/10.1006/mpat.1994.1044; PMID: 7830531
- Shet A, Kaplan EL, Johnson DR, Cleary PP. Immune response to group A streptococcal C5a peptidase in children: implications for vaccine development. J Infect Dis 2003; 188:809 - 17; http://dx.doi.org/10.1086/377700; PMID: 12964111
- Valentin-Weigand P, Talay SR, Kaufhold A, Timmis KN, Chhatwal GS. The fibronectin binding domain of the Sfb protein adhesin of Streptococcus pyogenes occurs in many group A streptococci and does not cross-react with heart myosin. Microb Pathog 1994; 17:111 - 20; http://dx.doi.org/10.1006/mpat.1994.1057; PMID: 7861955
- Rodríguez-Ortega MJ, Norais N, Bensi G, Liberatori S, Capo S, Mora M, et al. Characterization and identification of vaccine candidate proteins through analysis of the group A Streptococcus surface proteome. Nat Biotechnol 2006; 24:191 - 7; http://dx.doi.org/10.1038/nbt1179; PMID: 16415855
- Zhang S, Green NM, Sitkiewicz I, Lefebvre RB, Musser JM. Identification and characterization of an antigen I/II family protein produced by group A Streptococcus. Infect Immun 2006; 74:4200 - 13; http://dx.doi.org/10.1128/IAI.00493-06; PMID: 16790795
- Henningham A, Chiarot E, Gillen CM, Cole JN, Rohde M, Fulde M, et al. Conserved anchorless surface proteins as group A streptococcal vaccine candidates. J Mol Med (Berl) 2012; 90:1197 - 207; http://dx.doi.org/10.1007/s00109-012-0897-9; PMID: 22527883
- Chan AH, Tan HC, Chow AY, Lim AP, Lok SM, Moreland NJ, et al. A human PrM antibody that recognizes a novel cryptic epitope on dengue E glycoprotein. PLoS One 2012; 7:e33451; http://dx.doi.org/10.1371/journal.pone.0033451; PMID: 22509258
- Kelker HC, Itri VR, Valentine FT. A strategy for eliciting antibodies against cryptic, conserved, conformationally dependent epitopes of HIV envelope glycoprotein. PLoS One 2010; 5:e8555; http://dx.doi.org/10.1371/journal.pone.0008555; PMID: 20052405
- Bessen D, Fischetti VA. Synthetic peptide vaccine against mucosal colonization by group A streptococci. I. Protection against a heterologous M serotype with shared C repeat region epitopes. J Immunol 1990; 145:1251 - 6; PMID: 1696296
- Jones KF, Khan SA, Erickson BW, Hollingshead SK, Scott JR, Fischetti VA. Immunochemical localization and amino acid sequences of crossreactive epitopes within the group A streptococcal M6 protein. J Exp Med 1986; 164:1226 - 38; http://dx.doi.org/10.1084/jem.164.4.1226; PMID: 2428914
- Miller L, Burdett V, Poirier TP, Gray LD, Beachey EH, Kehoe MA. Conservation of protective and nonprotective epitopes in M proteins of group A streptococci. Infect Immun 1988; 56:2198 - 204; PMID: 2456272
- Lancefield RC. Differentiation of group A streptococci with a common R antigen into three serological types, with special reference to the bactericidal test. J Exp Med 1957; 106:525 - 44; http://dx.doi.org/10.1084/jem.106.4.525; PMID: 13475611
- Pruksakorn S, Galbraith A, Houghten RA, Good MF. Conserved T and B cell epitopes on the M protein of group A streptococci. Induction of bactericidal antibodies. J Immunol 1992; 149:2729 - 35; PMID: 1383324
- Crater DL, van de Rijn I. Hyaluronic acid synthesis operon (has) expression in group A streptococci. J Biol Chem 1995; 270:18452 - 8; http://dx.doi.org/10.1074/jbc.270.31.18452; PMID: 7629171
- Pruksakorn S, Currie B, Brandt E, Martin D, Galbraith A, Phornphutkul C, et al. Towards a vaccine for rheumatic fever: identification of a conserved target epitope on M protein of group A streptococci. Lancet 1994; 344:639 - 42; http://dx.doi.org/10.1016/S0140-6736(94)92083-4; PMID: 7520963
- Brandt ER, Hayman WA, Currie B, Carapetis J, Wood Y, Jackson DC, et al. Opsonic human antibodies from an endemic population specific for a conserved epitope on the M protein of group A streptococci. Immunology 1996; 89:331 - 7; http://dx.doi.org/10.1046/j.1365-2567.1996.d01-754.x; PMID: 8958044
- Steer AC, Magor G, Jenney AWJ, Kado J, Good MF, McMillan D, et al. emm and C-repeat region molecular typing of beta-hemolytic Streptococci in a tropical country: implications for vaccine development. J Clin Microbiol 2009; 47:2502 - 9; http://dx.doi.org/10.1128/JCM.00312-09; PMID: 19515838
- Bauer MJ, Georgousakis MM, Vu T, Henningham A, Hofmann A, Rettel M, et al. Evaluation of novel Streptococcus pyogenes vaccine candidates incorporating multiple conserved sequences from the C-repeat region of the M-protein. Vaccine 2012; 30:2197 - 205; http://dx.doi.org/10.1016/j.vaccine.2011.12.115; PMID: 22265945
- Martin DR, Sriprakash, K. S. Epidemiology of group A streptococcal disease in Australia and NewZealand. Recent advances in Microbiology 1996; 4:1-40.
- Dale JB, Beachey EH. Multiple, heart-cross-reactive epitopes of streptococcal M proteins. J Exp Med 1985; 161:113 - 22; http://dx.doi.org/10.1084/jem.161.1.113; PMID: 2578539
- Froude J, Gibofsky A, Buskirk DR, Khanna A, Zabriskie JB. Cross-reactivity between streptococcus and human tissue: a model of molecular mimicry and autoimmunity. Curr Top Microbiol Immunol 1989; 145:5 - 26; http://dx.doi.org/10.1007/978-3-642-74594-2_2; PMID: 2680297
- Guilherme L, Oshiro SE, Faé KC, Cunha-Neto E, Renesto G, Goldberg AC, et al. T-cell reactivity against streptococcal antigens in the periphery mirrors reactivity of heart-infiltrating T lymphocytes in rheumatic heart disease patients. Infect Immun 2001; 69:5345 - 51; http://dx.doi.org/10.1128/IAI.69.9.5345-5351.2001; PMID: 11500404
- Cunningham MW. T cell mimicry in inflammatory heart disease. Mol Immunol 2004; 40:1121 - 7; http://dx.doi.org/10.1016/j.molimm.2003.11.023; PMID: 15036918
- Guilherme L, Cunha-Neto E, Tanaka AC, Dulphy N, Toubert A, Kalil J. Heart-directed autoimmunity: the case of rheumatic fever. J Autoimmun 2001; 16:363 - 7; http://dx.doi.org/10.1006/jaut.2000.0487; PMID: 11334505
- Martins TB, Hoffman JL, Augustine NH, Phansalkar AR, Fischetti VA, Zabriskie JB, et al. Comprehensive analysis of antibody responses to streptococcal and tissue antigens in patients with acute rheumatic fever. Int Immunol 2008; 20:445 - 52; http://dx.doi.org/10.1093/intimm/dxn004; PMID: 18245783
- Goldstein I, Halpern B, Robert L. Immunological Relationship between Streptococcus a Polysaccharide and Structural Glycoproteins of Heart Valve. Nature 1967; 213:44; http://dx.doi.org/10.1038/213044a0
- Guilherme L, Dulphy N, Douay C, Coelho V, Cunha-Neto E, Oshiro SE, et al. Molecular evidence for antigen-driven immune responses in cardiac lesions of rheumatic heart disease patients. Int Immunol 2000; 12:1063 - 74; http://dx.doi.org/10.1093/intimm/12.7.1063; PMID: 10882418
- Guilherme L, Cunha-Neto E, Coelho V, Snitcowsky R, Pomerantzeff PM, Assis RV, et al. Human heart-infiltrating T-cell clones from rheumatic heart disease patients recognize both streptococcal and cardiac proteins. Circulation 1995; 92:415 - 20; http://dx.doi.org/10.1161/01.CIR.92.3.415; PMID: 7634457
- Sampaio RO, Fae KC, Demarchi LM, Pomerantzeff PM, Aiello VD, Spina GS, et al. Rheumatic heart disease: 15 years of clinical and immunological follow-up. Vasc Health Risk Manag 2007; 3:1007 - 17; PMID: 18200819
- Pruksakorn S, Currie B, Brandt E, Phornphutkul C, Hunsakunachai S, Manmontri A, et al. Identification of T cell autoepitopes that cross-react with the C-terminal segment of the M protein of group A streptococci. Int Immunol 1994; 6:1235 - 44; http://dx.doi.org/10.1093/intimm/6.8.1235; PMID: 7981150
- Guilherme L, Cury P, Demarchi LMF, Coelho V, Abel L, Lopez AP, et al. Rheumatic heart disease: proinflammatory cytokines play a role in the progression and maintenance of valvular lesions. Am J Pathol 2004; 165:1583 - 91; http://dx.doi.org/10.1016/S0002-9440(10)63415-3; PMID: 15509528
- Relf WA, Cooper J, Brandt ER, Hayman WA, Anders RF, Pruksakorn S, et al. Mapping a conserved conformational epitope from the M protein of group A streptococci. Pept Res 1996; 9:12 - 20; PMID: 8727479
- Pandey M, Wykes MN, Hartas J, Good MF, Batzloff MR. Long-term antibody memory induced by synthetic peptide vaccination is protective against Streptococcus pyogenes infection and is independent of memory T cell help. J Immunol 2013; 190:2692 - 701; http://dx.doi.org/10.4049/jimmunol.1202333; PMID: 23401589
- Batzloff MR, Hayman WA, Davies MR, Zeng M, Pruksakorn S, Brandt ER, et al. Protection against group A streptococcus by immunization with J8-diphtheria toxoid: contribution of J8- and diphtheria toxoid-specific antibodies to protection. J Infect Dis 2003; 187:1598 - 608; http://dx.doi.org/10.1086/374800; PMID: 12721940
- Batzloff MR, Yan H, Davies MR, Hartas J, Lowell GH, White G, et al. Toward the development of an antidisease, transmission-blocking intranasal vaccine for group a streptococcus. J Infect Dis 2005; 192:1450 - 5; http://dx.doi.org/10.1086/466528; PMID: 16170764
- Batzloff MR, Hartas J, Zeng WG, Jackson DC, Good MF. Intranasal vaccination with a lipopeptide containing a conformationally constrained conserved minimal peptide, a universal T cell epitope, and a self-adjuvanting lipid protects mice from group A streptococcus challenge and reduces throat colonization. J Infect Dis 2006; 194:325 - 30; http://dx.doi.org/10.1086/505146; PMID: 16826480