Abstract
The recent H7N9 influenza outbreak in China highlights the need for influenza vaccine production systems that are robust and can quickly generate substantial quantities of vaccines that target new strains for pandemic and seasonal immunization. Although the influenza vaccine system, a public-private partnership, has been effective in providing vaccines, there are areas for improvement. Technological advances such as mammalian cell culture production and synthetic vaccine seeds provide a means to increase the speed and accuracy of targeting new influenza strains with mass-produced vaccines by dispensing with the need for egg isolation, adaptation, and reassortment of vaccine viruses. New influenza potency assays that no longer require the time-consuming step of generating sheep antisera could further speed vaccine release. Adjuvants that increase the breadth of the elicited immune response and allow dose sparing provide an additional means to increase the number of available vaccine doses. Together these technologies can improve the influenza vaccination system in the near term. In the longer term, disruptive technologies, such as RNA-based flu vaccines and ‘universal’ flu vaccines, offer a promise of a dramatically improved influenza vaccine system.
Introduction
Influenza virus infections are responsible for significant morbidity and mortality in a broad age range, with particularly severe outcomes in the elderly and young children. Seasonal epidemics are responsible for about 250 000 to 500 000 deaths and between three and five million cases worldwide. Concern that a new pandemic could arise like the 1918 pandemic that claimed ~50 million lives highlights the need for both constant surveillance and improved preparedness.Citation1
Vaccines against influenza are unique because they require nearly annual reformulation due to continuous viral evolution through antigenic drift (changes in hemagglutinin [HA] surface residues), antigenic shift (new viruses resulting from genome segment swaps), and zoonotic transmission (introduction of non-human animal influenza viruses into the human population).Citation2 The solution to this important problem has been the implementation of a worldwide influenza vaccine production system that includes both public and private components and carries out four major activities: influenza surveillance, vaccine virus generation, vaccine manufacturing, and strain-specific release assay development (). The system must be sufficiently robust to reliably produce seasonal vaccines and bring on line surge capacity for pandemic vaccine production when pandemics arise.
Figure 1. Broad overview of the (A) current influenza vaccine global system from clinical isolate to final commercial vaccine and (B) anticipated system. Advancements at every step streamline the process, generating a new global system in which information on genetic sequences, antigenicity testing, and release assays rather than materials are the primary items shared, resulting in accelerated and increased vaccine supply. Images courtesy of Debora Cartagena and Brian Judd/James Gathany.
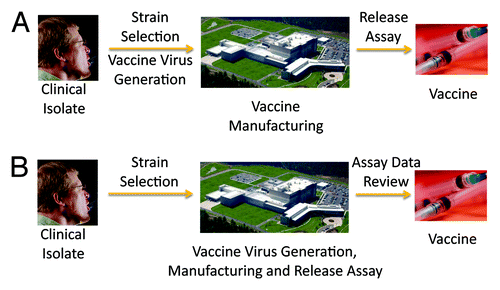
Currently, most licensed influenza vaccines (inactivated whole virus, detergent or solvent split vaccines, live attenuated vaccines, and some subunit vaccines) require a viral vaccine seed to produce vaccine, and the virus or viral genes for this seed typically are provided by a WHO Collaborating Center. Generating an influenza vaccine seed begins when an influenza virus is isolated from an infected individual’s respiratory secretions at a WHO-linked National Influenza Center. The virus is then adapted for growth in eggs, generally resulting in egg-specific adaptations that change the virus binding specificity from the receptors dominant in human respiratory epithelium (α2,6-linked sialosides) to those dominant in the egg allantoic cavity (α2,3-linked sialosides).Citation3 Egg adaptation can generate mutations in the important neutralizing epitopes that surround the sialoside binding site.Citation4
The HA generated from this process can be a genetic mismatch to the clinical isolate and is sometimes an antigenic mismatch.Citation5 Historically, vaccine antigenic mismatch has been particularly prominent during seasons in which there has been high influenza-like illness leading to particular stresses on the worldwide vaccine production system (). Human immunization with vaccines produced from the egg adapted vaccine seed viruses for both A/H3N2 in the 2010–11 season and influenza B in the 2009–10 and 2010–11 seasons elicited low neutralizing and hemagglutination inhibition (HI) titers to circulating viruses and to the isolates of these viruses on mammalian (Madin-Darby canine kidney [MDCK]) cells, despite high titers to the egg-adapted vaccine strain.Citation6 As recently as the 2012–13 season, egg-adaptations in the A/H3N2 vaccine virus may have contributed to lower vaccine effectiveness.Citation7A WHO report from after the 2012-13 vaccination campaign began showed that the egg-adapted reference strain appeared to be a mismatch from the circulating strains.Citation8 An equivalent strain isolated in MDCK cells was a match to circulating strains, but even vaccine manufacturers that can produce vaccine in mammalian cells were required to produce the season’s influenza vaccine using the mismatched egg-adapted vaccine seed virus.
Figure 2. Percentage of patient visits for influenza-like illness in the US for selected seasons and years of mismatch. Chart on patient visits are from the US. Outpatient Influenza-like Illness Surveillance Network, Centers for Disease Control and Prevention (http://www.cdc.gov/flu/weekly/). Mismatch data are from the World Health Organization vaccine formulation recommendation from the relevant years.Citation8,Citation35–Citation37
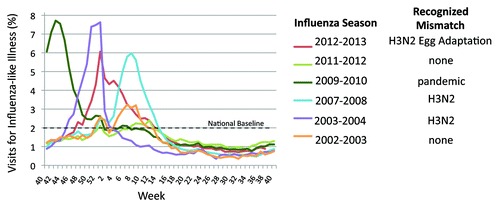
Two newer approaches can address the limitations of egg isolation: MDCK cells as an alternate substrate for virus isolation and the use of synthetic seed viruses.Citation9,Citation10 Data on virus isolation rates in 2009 demonstrated that for the H3N2 subtype, a much higher proportion of circulating viruses could be isolated in MDCK cells than in chicken eggs.Citation11 The large difference in isolation rates and declining egg isolation rates over time raised the concern that, if trends continued, a suitable virus match to circulating H3N2 strains would not be available. MDCK cells have both α2,3 and α2,6 sialosides on their surfaces, decreasing the degree of adaptation needed for growth in the laboratory and resulting in higher virus isolation rates.Citation12,Citation13 A collaboration was formed between the World Health Organization (WHO) and International Federation of Pharmaceutical Manufacturers and Associations (IFPMA) to further explore the use of a mammalian cell lines in vaccine virus isolation. For the 2013–14 influenza season, for the first time, the WHO has recommended that the viruses used to produce influenza vaccines may be antigenically “like” a MDCK-isolated H3N2 reference virus that is well matched to circulating influenza strains.Citation8 In the past, only vaccine viruses that match egg-adapted reference strains were allowed. Once regulatory hurdles to the use of purely MDCK-cultured seasonal vaccine viruses are overcome, manufacturers who produce vaccine antigens on mammalian cells will be able to produce better matched vaccines, no longer constrained to match viruses with egg-adaptive mutations. While these efforts are ongoing, a recently published approach shows how the generation of vaccine viruses using synthetic DNA also alleviates the issues of egg-adaptation while providing a number of additional advantages.
Synthetic Seed and Cell Culture Technology
Synthetic vaccine viruses are influenza viruses that have been generated using reverse genetics techniques with synthetic genes for the purpose of preparing vaccine seeds. These viruses are rescued (generated from nucleic acids) in a vaccine-approved MDCK cell line, and the process has robustly rescued viruses (>33) from multiple relevant subtypes while maintaining the original viral HA and neuraminidase (NA) sequences.Citation10 From the time HA and NA sequences are obtained, synthetic vaccine viruses with high growth backbones can be rescued in <5 d, eliminating the additional time required in traditional processes to adapt viruses to growth in eggs and “mate” them with donor strains to improve their growth and antigen productivity for vaccine manufacturing.Citation14 The process decreases the risk of adventitious agent contamination because the key strain-specific starting material is an electronically transmitted gene sequence, not a sick patient’s respiratory secretions. When this becomes the method for routine preparation of vaccine viruses, the WHO’s global influenza surveillance system will likely retain its primarily role in information gathering and sharing and in making recommendations, but the production of strain-specific starting materials used in vaccine manufacturing is likely to shift to organizations with established track records for timely, well-controlled, and highly compliant production of pharmaceutical products.
The benefits of improved vaccine seed virus production can be increased through the use of newer cell-culture based production technologies. Cell culture production systems, such as those using MDCK cells or Vero cells, allow for faster scale up in case of pandemics and enable greater safety of supply, because exhaustively tested cell banks are far better characterized than the 100s of millions of chicken eggs used for influenza vaccine manufacturing each year. Unlike the eggs laid by large commercial bird flocks, the mammalian cells are propagated in carefully controlled environments, using well-defined media and have been tested for a number of qualities, including absence of adventitious agents, purity, and genetic stability, to ensure homogeneity and safety. Overall, completely egg-free, mammalian cell culture-based vaccine manufacturing can be expected to introduce fewer mutations away from circulating influenza antigens, increase a timely vaccine supply, and meet modern standards of pharmaceutical production.
Alternative Potency Assays
Although the most rapid vaccine response in history was executed during the 2009 H1N1 pandemic, substantial vaccine quantities were available only after the second pandemic wave had peaked.Citation15 The time required to generate calibrated reagents for the antiquated potency assay (the assay used to measure the amount of vaccine antigen put in each dose), serial radial immunodiffusion (SRID), was rate limiting.Citation16 Reagent generation for this assay requires the time-consuming steps of immunizing sheep with antigen from the new strain, waiting for the sheep to seroconvert, and then calibrating the strain-specific sera and antigen standards. The timing of SRID reagent availability determines the speed of vaccine release because blending, filling, finishing, and distributing the vaccine only occur after vaccine potency is determined. Public health agencies and vaccine manufacturers worldwide have recognized that replacing the current influenza potency assay, which involves a complicated international system to generate and calibrate reagents, with alternative approaches can benefit public health by accelerating vaccine availability.
Alternative influenza vaccine potency assay approaches can be placed in two main categories: antibody-independent and antibody-dependent. To date, antibody-independent assays, such as reversed phase high pressure liquid chromatography (RP-HPLC),Citation17,Citation18 isotope dilution mass spectrometryCitation19 and SDS-PAGE,Citation20 can determine the amount of HA present but denature the HA before measurement. Therefore, they are non-conformational and do not distinguish HA that can elicit protective antibodies (immunologically active HA) from misfolded or denatured HA that is unlikely to elicit potently protective immune responses. Antibody-dependent assays, such as ELISACitation21,Citation22 and surface plasmon resonance,Citation23 can distinguish immunologically active HA if conformationally specific antibodies to certain regions are used. However, when a strain change occurs, new antibodies would often need to be generated and characterized, slowing vaccine release as occurs today.
Thus, although these alternative approaches for measuring potency have promising aspects, none robustly and accurately measure immunologically active HA quickly. An optimal influenza vaccine antigen potency assay must combine conformational selectivity with speed and use only non-strain-specific reagents. This should be possible. For example, if a biochemical pre-step can separate immunologically active HA from damaged or misfolded HA and then an accurate, reproducible, and rapid but denaturing measurement technique measures only the HA in the immunologically active fraction, then speed, conformational selectivity, and accuracy can be combined. For use in testing seasonal vaccine stability during storage, it would be desirable for the separation and measurement procedure to determine accurately and simultaneously the amount of immunologically active HA of each strain present in a trivalent or quadrivalent mixture. This is an area of active exploration. As other manufacturing advances, such as the use of synthetic seeds, speed vaccine production it will become increasingly important to have such an alternative potency assay to speed vaccine release.
Adjuvants
Adjuvants have been an exciting area of exploration for vaccines and have been reviewed extensively elsewhere.Citation24 Only the oil-in-water emulsion adjuvants MF59 and ASO3 are used in contemporary influenza vaccines. Influenza vaccines using these adjuvants elicit increased quantities of neutralizing antibodies, increase the breadth of the immune response for coverage of mismatched and drifted influenza strains, and enable antigen dose sparing.Citation25 A particularly striking example of how adjuvants can improve vaccine efficacy in the pediatric population has been shown in a Phase III clinical trial for Fluad® (MF59-adjuvanted trivalent inactivated vaccine [TIV], Novartis) compared with un-adjuvanted TIV and a control non-influenza vaccine. Over 4500 vaccine-naïve 6–72 mo-old children were immunized with the respective vaccines and were monitored for PCR-confirmed influenza-like illness over two influenza seasons.Citation26 Fluad® had an 86% absolute efficacy against all influenza strains, and TIV had a 43% absolute efficacy. These data demonstrate the ability of adjuvants to improve the efficacy of influenza vaccination.
Disruptive Technologies
Two disruptive technologies recently described in the literature could bring about more dramatic changes in the current system: RNA-based influenza vaccines and ‘universal’ influenza vaccines. RNA technologies promise quick, strain-independent production with high yields from a small manufacturing footprint. Recently, Hekele et al. (2013) demonstrated that an H7N9 SAM® influenza vaccine candidate based on self-amplifying mRNA delivered by synthetic lipid nanoparticles could be produced within 8 d of the posting of the first H7N9 HA sequence on a web-based data sharing platform (GISAID) by the Chinese CDC. This vaccine candidate was immunogenic in mice, raising an HI geometric mean titer (GMT) of 238 three wk after the second immunization when there was a three week interval between the first and second immunization.Citation27 If the interval between immunizations was 8 wk, an HI GMT of 807 was elicited. If this vaccine is similarly immunogenic, safe and well-tolerated in humans, rapidly generated SAM pandemic influenza vaccines could be new tools to slow an influenza pandemic.
Universal influenza vaccine technologies seek to generate robust, durable memory responses that are broadly protective against current and future influenza viruses. Different groups are developing vaccines that target T cell- and/or B cell-mediated immunity.Citation28 T cell-based vaccines rely on the generation of a response to conserved T cell epitopes on both internal and surface exposed proteins. T cell responses against conserved buried epitopes generally have greater breadth than antibody responses against more variable surface-exposed epitopes. However, the protective efficacy of vaccine-elicited T cell responses upon natural challenge is uncertain, and demonstrating their value in human subjects may require expensive efficacy trials because, unlike for antibody-mediated protection, there is no established laboratory test of cell-mediated immunity that is accepted as a correlate of protection for the purpose of vaccine licensure.
In 2009, efforts to generate a B cell-mediated universal influenza vaccine were bolstered by the structural elucidation of a broadly neutralizing antibody, CR6261, bound to the HA stem region.Citation29 The structure provided evidence that, despite HA antigenic variability, a broadly neutralizing epitope exists, and it provided a target epitope for structure-guided antigen design efforts. Initial efforts to generate a stem-only modified HA antigen have proven challenging with limited in vivo success.Citation30
An alternative approach for directing the immune response was supported by data from the 2009 H1N1 pandemic, during which the B cell antibody repertoire of patients exposed to the 2009 H1N1 pandemic virus contained a higher proportion of cells expressing broadly neutralizing antibodies than similar samples from subjects who had not yet been exposed to the pandemic virus.Citation31 This observation led to the hypothesis that a vaccination strategy of sequential immunization with immunogens that only share broadly neutralizing epitopes could have the effect of boosting a pre-existing B cell response to these conserved regions.Citation32 Recently, this approach has been tested through the generation of HA immunogens that contain a stem region from a single virus strain but head regions from exotic HA subtypes.Citation33 Mice immunized in succession with these antigens have shown both an increase in anti-stem antibody titers and expanded breadth of protection in challenge models. On the other hand, an efficacy study in a pig model of influenza infection provides a cautionary example.Citation34 In pigs, immunization with an inactivated influenza virus that shared HA stem epitopes but not HA head epitopes or NA epitopes with a live influenza challenge strain elicited cross-reactive HA stem antibodies but enhanced disease upon challenge.
Conclusions
The current influenza system provides a valuable public health service through the large-scale production of influenza vaccines. Recent technological advances can modernize several aspects of vaccine virus generation and vaccine production and have the potential to accelerate the speed of vaccine production and release. Adjuvants increase the neutralizing immune response, increase vaccine breadth, and allow dose sparing. While disruptive technologies show promise for how the system could evolve in the future, there is a real opportunity to improve the influenza vaccine system to address vaccine needs using technologies that are ready today.
Disclosure of Potential Conflicts of Interest
The authors are Novartis stockholders and employees of Novartis Vaccines and Diagnostics.
References
- Taubenberger JK, Morens DM. 1918 Influenza: the mother of all pandemics. Emerg Infect Dis 2006; 12:15 - 22; PMID: 16494711
- Wright PF, Neumann G, Kawaoka Y. Orthomyxoviruses. In: Knipe DM, Howley PM, editors. Field's Virology. Philadeplphia: Lippincott, Williams and Wilkins; pages 1691–740.
- Shinya K, Ebina M, Yamada S, Ono M, Kasai N, Kawaoka Y. Avian flu: influenza virus receptors in the human airway. Nature 2006; 440:435 - 6; http://dx.doi.org/10.1038/440435a; PMID: 16554799
- Schild GC, Oxford JS, de Jong JC, Webster RG. Evidence for host-cell selection of influenza virus antigenic variants. Nature 1983; 303:706 - 9; http://dx.doi.org/10.1038/303706a0; PMID: 6190093
- Robertson JS, Naeve CW, Webster RG, Bootman JS, Newman R, Schild GC. Alterations in the hemagglutinin associated with adaptation of influenza B virus to growth in eggs. Virology 1985; 143:166 - 74; http://dx.doi.org/10.1016/0042-6822(85)90105-9; PMID: 4060580
- Kishida N, Fujisaki S, Yokoyama M, Sato H, Saito R, Ikematsu H, Xu H, Takashita E, Tashiro M, Takao S, et al. Evaluation of influenza virus A/H3N2 and B vaccines on the basis of cross-reactivity of postvaccination human serum antibodies against influenza viruses A/H3N2 and B isolated in MDCK cells and embryonated hen eggs. Clin Vaccine Immunol 2012; 19:897 - 908; http://dx.doi.org/10.1128/CVI.05726-11; PMID: 22492743
- Skowronski DM, Janjua NZ, De Serres G, Dickinson JA, Winter A-L, Mahmud SM, Sabaiduc S, Gubbay JB, Charest H, Petric M, et al. Interim estimates of influenza vaccine effectiveness in 2012/13 from Canada’s sentinel surveillance network, January 2013. Euro Surveill 2013; 18:18; PMID: 23399422
- World Health Organization. Recommended composition of influenza virus vaccines for use in the 2013-2014 northern hemisphere influenza season [Internet]. 2013. Available from: http://www.who.int/influenza/vaccines/virus/recommendations/201302_recommendation.pdf
- Dormitzer PR. Cell culture-derived influenza vaccines. In: Del Giudice G, Rappuoli R, editors. Influenza vaccines for the future. Basel: Birkhauser Inc; 2010. pages 293–312.
- Dormitzer PR, Suphaphiphat P, Gibson DG, Wentworth DE, Stockwell TB, Algire MA, Alperovich N, Barro M, Brown DM, Craig S, et al. Synthetic generation of influenza vaccine viruses for rapid response to pandemics. Sci Transl Med 2013; 5:85ra68; http://dx.doi.org/10.1126/scitranslmed.3006368; PMID: 23677594
- Minor PD, Engelhardt OG, Wood JM, Robertson JS, Blayer S, Colegate T, Fabry L, Heldens JGM, Kino Y, Kistner O, et al. Current challenges in implementing cell-derived influenza vaccines: implications for production and regulation, July 2007, NIBSC, Potters Bar, UK. Vaccine 2009; 27:2907 - 13; http://dx.doi.org/10.1016/j.vaccine.2009.02.064; PMID: 19366582
- Katz JM, Wang M, Webster RG. Direct sequencing of the HA gene of influenza (H3N2) virus in original clinical samples reveals sequence identity with mammalian cell-grown virus. J Virol 1990; 64:1808 - 11; PMID: 2319652
- Ito T, Suzuki Y, Takada A, Kawamoto A, Otsuki K, Masuda H, Yamada M, Suzuki T, Kida H, Kawaoka Y. Differences in sialic acid-galactose linkages in the chicken egg amnion and allantois influence human influenza virus receptor specificity and variant selection. J Virol 1997; 71:3357 - 62; PMID: 9060710
- Ramanunninair M, Le J, Onodera S, Fulvini AA, Pokorny BA, Silverman J, Devis R, Arroyo JM, He Y, Boyne A, et al. Molecular signature of high yield (growth) influenza a virus reassortants prepared as candidate vaccine seeds. PLoS One 2013; 8:e65955; http://dx.doi.org/10.1371/journal.pone.0065955; PMID: 23776579
- Rappuoli R, Dormitzer PR. Influenza: options to improve pandemic preparation. Science 2012; 336:1531 - 3; http://dx.doi.org/10.1126/science.1221466; PMID: 22723412
- Schild GC, Wood JM, Newman RW. A single-radial-immunodiffusion technique for the assay of influenza haemagglutinin antigen. Proposals for an assay method for the haemagglutinin content of influenza vaccines. Bull World Health Organ 1975; 52:223 - 31; PMID: 816480
- Kapteyn JC, Porre AM, de Rond EJP, Hessels WB, Tijms MA, Kessen H, Slotboom AME, Oerlemans MA, Smit D, van der Linden J, et al. HPLC-based quantification of haemagglutinin in the production of egg- and MDCK cell-derived influenza virus seasonal and pandemic vaccines. Vaccine 2009; 27:1468 - 77; http://dx.doi.org/10.1016/j.vaccine.2008.11.113; PMID: 19110022
- Lorbetskie B, Wang J, Gravel C, Allen C, Walsh M, Rinfret A, Li X, Girard M. Optimization and qualification of a quantitative reversed-phase HPLC method for hemagglutinin in influenza preparations and its comparative evaluation with biochemical assays. Vaccine 2011; 29:3377 - 89; http://dx.doi.org/10.1016/j.vaccine.2011.02.090; PMID: 21397719
- Williams TL, Luna L, Guo Z, Cox NJ, Pirkle JL, Donis RO, Barr JR. Quantification of influenza virus hemagglutinins in complex mixtures using isotope dilution tandem mass spectrometry. Vaccine 2008; 26:2510 - 20; http://dx.doi.org/10.1016/j.vaccine.2008.03.014; PMID: 18440105
- Harvey R, Hamill M, Robertson JS, Minor PD, Vodeiko GM, Weir JP, Takahashi H, Harada Y, Itamura S, Bamford P, et al. Application of deglycosylation to SDS PAGE analysis improves calibration of influenza antigen standards. Biologicals 2012; 40:96 - 9; http://dx.doi.org/10.1016/j.biologicals.2011.12.009; PMID: 22244521
- Hashem AM, Gravel C, Farnsworth A, Zou W, Lemieux M, Xu K, Li C, Wang J, Goneau M-F, Merziotis M, et al. A novel synthetic receptor-based immunoassay for influenza vaccine quantification. PLoS One 2013; 8:e55428; http://dx.doi.org/10.1371/journal.pone.0055428; PMID: 23424631
- Bodle J, Verity EE, Ong C, Vandenberg K, Shaw R, Barr IG, Rockman S. Development of an enzyme-linked immunoassay for the quantitation of influenza haemagglutinin: an alternative method to single radial immunodiffusion. Influenza Other Respir Viruses 2013; 7:191 - 200; http://dx.doi.org/10.1111/j.1750-2659.2012.00375.x; PMID: 22583601
- Nilsson CE, Abbas S, Bennemo M, Larsson A, Hämäläinen MD, Frostell-Karlsson A. A novel assay for influenza virus quantification using surface plasmon resonance. Vaccine 2010; 28:759 - 66; http://dx.doi.org/10.1016/j.vaccine.2009.10.070; PMID: 19857452
- Mount A, Koernig S, Silva A, Drane D, Maraskovsky E, Morelli AB. Combination of adjuvants: the future of vaccine design. Expert Rev Vaccines 2013; 12:733 - 46; http://dx.doi.org/10.1586/14760584.2013.811185; PMID: 23885819
- O’Hagan DT, Rappuoli R, De Gregorio E, Tsai T, Del Giudice G. MF59 adjuvant: the best insurance against influenza strain diversity. Expert Rev Vaccines 2011; 10:447 - 62; http://dx.doi.org/10.1586/erv.11.23; PMID: 21506643
- Vesikari T, Knuf M, Wutzler P, Karvonen A, Kieninger-Baum D, Schmitt H-J, Baehner F, Borkowski A, Tsai TF, Clemens R. Oil-in-water emulsion adjuvant with influenza vaccine in young children. N Engl J Med 2011; 365:1406 - 16; http://dx.doi.org/10.1056/NEJMoa1010331; PMID: 21995388
- Hekele A, Bertholet S, Archer J, Gibson DG, Palladino G, Brito LA, Otten GR, Brazzoli M, Buccato S, Bonci A, et al. Rapidly produced SAM® vaccine against H7N9 influenza is immunogenic in mice. Emerg Microbes Infect 2013; 2:e52; http://dx.doi.org/10.1038/emi.2013.54
- Gilbert SC. Advances in the development of universal influenza vaccines. Influenza Other Respir Viruses 2013; 7:750 - 8; http://dx.doi.org/10.1111/irv.12013; PMID: 22998622
- Ekiert DC, Bhabha G, Elsliger M-A, Friesen RHE, Jongeneelen M, Throsby M, Goudsmit J, Wilson IA. Antibody recognition of a highly conserved influenza virus epitope. Science 2009; 324:246 - 51; http://dx.doi.org/10.1126/science.1171491; PMID: 19251591
- Steel J, Lowen AC, Wang TT, Yondola M, Gao Q, Haye K, García-Sastre A, Palese P. Influenza virus vaccine based on the conserved hemagglutinin stalk domain. MBio 2010; 1:1; http://dx.doi.org/10.1128/mBio.00018-10; PMID: 20689752
- Wrammert J, Koutsonanos D, Li G-M, Edupuganti S, Sui J, Morrissey M, McCausland M, Skountzou I, Hornig M, Lipkin WI, et al. Broadly cross-reactive antibodies dominate the human B cell response against 2009 pandemic H1N1 influenza virus infection. J Exp Med 2011; 208:181 - 93; http://dx.doi.org/10.1084/jem.20101352; PMID: 21220454
- Settembre EC, Dormitzer PR, Rappuoli R. Learning from the 2009 H1N1 pandemic: prospects for more broadly effective influenza vaccines. J Mol Cell Biol 2011; 3:144 - 6; http://dx.doi.org/10.1093/jmcb/mjq046; PMID: 21430014
- Krammer F, Pica N, Hai R, Margine I, Palese P. Chimeric hemagglutinin influenza virus vaccine constructs elicit broadly protective stalk-specific antibodies. J Virol 2013; 87:6542 - 50; http://dx.doi.org/10.1128/JVI.00641-13; PMID: 23576508
- Khurana S, Loving CL, Manischewitz J, King LR, Gauger PC, Henningson J, Vincent AL, Golding H. Vaccine-induced anti-HA2 antibodies promote virus fusion and enhance influenza virus respiratory disease. Sci Transl Med 2013; 5:ra114; http://dx.doi.org/10.1126/scitranslmed.3006366; PMID: 23986398
- World Health Organization. Recommended composition of influenza virus vaccines for use in the 2010-2011 northern hemisphere influenza season [Internet]. 2010. Available from: http://www.who.int/influenza/vaccines/virus/recommended_compositionFeb08FullReport.pdf
- World Health Organization. Recommended composition of influenza virus vaccines for use in the 2008-2009 northern hemisphere influenza season [Internet]. 2008. Available from: http://www.who.int/influenza/vaccines/ recommended_compositionFeb08FullReport.pdf.
- World Health Organization. Recommended composition of influenza virus vaccines for use in the 2004-2005 northern hemisphere influenza season [Internet]. 2004. Available from: http://www.who.int/influenza/vaccines/virus/recommended_compositionFeb04FullReport.pdf