Abstract
Both ricin toxin (RT) and abrin toxin (AT) are 2 important toxin agents as potantial bioweapons. A dual subunit vaccine against RT and AT exposure is a promising option for developing prophylactic vaccination. In this study, we constructed a dual vaccine with RT B chain and AT B chain named RTB-ATB. The RTB-ATB chimeric protein was expressed in Escherichia coli (E. coli), and the purified protein was used to evaluate the immune response by a 2 × 2 × 2 × 2 factorial design. The main effects included dose of RTB-ATB, route of immunization injection, immunization time interval, and dose of native toxins challenge. For 2 × LD50 challenge of RT or AT, 100% of the RTB-ATB immunized mice survived and regained or exceeded their initial weights within 10 days. For 4 × LD50 challenge, different routes of immunization injection caused significant difference (P < 0.05), intraperitoneal (i.p.) administration of immunogen protected mice better than the subcutaneous (s.c.) administration. In conclusion, when administered i.p. to mice with 25 μg per mouse and immunization time interval Π in the absence of adjuvant, the chimeric protein elicited a stronger immune response and protected the animals from a dose of native toxins which was 4 times higher than their LD50 in unvaccinated mice. Besides, the RTB-ATB chimeric protein could induce specific neutralizing antibodies against these 2 toxins. We anticipate that this study will open new possibilities in the preparation of RTB-ATB dual subunit vaccine against the exposure to deadly RT and AT.
Introduction
Ricin toxin (RT) and abrin toxin (AT), deadly plant toxins, with the greatest potential use as biological weapons and possible bioterrorist agents, have caused growing awareness on their possible applications as vaccines and related diagnosis in recent years. The 2 toxins, derived from castor beans (Ricinus communis seeds) and jequirity beans (Abrus precatorius seeds) respectively, belong to the type II ribosome-inactivating proteins.Citation1 There are similar structures with 2 chains linked by a disulfide bond for RT and AT. A chain (RTA or ATA) confers cellular toxicity while B chain (RTB or ATB) is essential for cell binding. Molecular weight of 2 toxins is approximately 60~65 kDa. The estimated LD50 of RT in mice is 3 μg/kg of body weight when injected,Citation2 while LD50 of AT is 0.04 μg/kg of body weight.Citation3 Therefore, AT is 75 times more toxic than RT. Because of their easy availability, stability, and extreme toxicity, the use of these agents as bioterrorist weapons is of great concern. For instance, RT was used in the highly publicized assassination of a Bulgarian defector in the 1970s, and is now classified by the Centers for Disease Control (CDC), Atlanta, GA as a level B biothreat.Citation4
RT or AT intoxication can be achieved by vaccination with toxoid,Citation5,Citation6 or by passive administration with antibodies in experimental animals.Citation1,Citation7-Citation9 Both procedures are effective in the prophylaxis or toxin intoxication in mice. Several preparations of RT have been used for vaccination including formalinized RT (toxoid),Citation5 deglycosylated RTA (dgRTA),Citation10 and attenuated RTA subunit.Citation2,Citation4,Citation11,Citation12 All preparations confer complete protection against RT. As to AT, there are few effective vaccines for AT exposure except that a toxoid attenuated with formalin was reported by GriffithsCitation6 and ATA mutant (mABRA) was produced in our lab.Citation3 Until now no vaccine study against simultaneously RT and AT has been described.
It is known that multivalent antigen vaccines are a promising option for protection against the different native antigen. Although composite vaccines developed with several antigens are useful in reducing the number of vaccination, there are some drawbacks, such as problems with preparation each recombinant proteins. Compared with mixed vaccines, the chimeric protein vaccine has many advantages, such as good safety and definite component.Citation13 Therefore, we tried to construct a chimeric protein which can confer complete protection against native RT and AT intoxication.
RTA was used to developing a vaccine candidate against RT, it has been studied for years as a potential immunotoxin.Citation2-Citation4,Citation10-Citation12 However, evidences suggest that B chain may be useful as a toxin subunit vaccine.Citation14,Citation15 First, B chain, in the absence of A chain, is non-toxic. Second, anti-RTB antibodies administered intravenously (i.v.) protected mice against a lethal dose of challenge. Third, B subunit-based vaccines have found to be effective in other members of the A–B family of toxins, such as cholera and tetanus. Finally, B chain of toxins themselves also has adjuvant activity because it specifically binds to the cell surfaces of M-cells.
In this study, we developed a dual subunit vaccine retained both RTB and ATB with high immunogenicity and a good protective immunity. This is the first report on development of dual subunit vaccine based on RTB and ATB as potentially novel and effective vaccine candidate against simultaneously AT and RT exposure threat.
Results
Construction of chimeric protein RTB-ATB expression vector
According to E. coli codon usage, there were 272 rare codons of the natural RTB and ATB gene were replaced with synonymous codons of high-frequency in a synthetic gene. The synthetic fragment was cloned into pQE-80L vector and identified by PCR and restriction analysis, which showed that the synthetic gene sequence was assembled correctly ().
Expression and purification of RTB-ATB
The chimeric protein with a 6 × His tag was expressed in the E. coli strain M15 and produced in insoluble form at 30 °C after induction with 1 mM IPTG. RTB-ATB (approximately 60 kDa) was purified using a Ni2+-chelating affinity chromatography resin column and eluted with elution buffer containing 300 mM imidazole. After induction, extraction, purification, and renaturation, the purified protein was analyzed using SDS-PAGE and 98.9% purity was achieved according to Total Lab 2.01 software ().
Figure 2. Coomassie-stained SDS-PAGE analysis of the RTB-ATB expression and purification (A) and affinity chromatographic profile (B). (A) Lane M, low molecular weight protein marker. Lanes 1 and 2, total cellular lysate of M15/pQE80L-RTB/ATB induced without IPTG and with IPTG, respectively. Lane 3 and 4, cell supernatants and cell debris after centrifugation at 12 000 rpm for 30 min. Lane 5, RTB-ATB eluted with elution buffer containing 300 mM imidazole. (B) The RTB-ATB protein was eluted with elution buffer containing 300 mM imidazole.
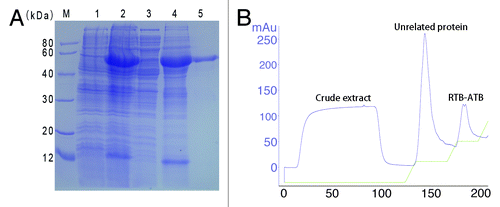
Immunoblotting
A western blotting showed that RTB-ATB was recognized by the rabbit pAb against native RT or AT, respectively (). These results indicated that both RTB component and ATB component are present in the expressed chimeric protein.
Vaccination and native toxins challenge
The results are shown in and . 100% of the RTB-ATB immunized mice (groups 1, 2, 5, 6, 9, 10, 13, and 14) survived challenge with RT or AT with a weight loss which was <10% during the first 2~4 d following challenge with 2 × LD50 of native toxins. For 4 × LD50 challenge, comparison between immunization time interval І and time interval Π showed that there was no significant difference. Also no difference was found between different doses of chimeric protein. Only different route of immunization injection caused significant difference (RT: P < 0.05, AT: P < 0.05). No significant difference was observed for the weight of survival of the mice among subgroups challenged with 2 and 4 × LD50 of native toxins (RT: P = 0.901 > 0.05, AT: P = 0.296 > 0.05). In contrast, 100% of the PBS-vaccinated mice died within 2 d following challenge with 2 × LD50 of native toxins (data not shown).
Table 1. Survival data and mean values of mice weight challenged with different doses of native RT
Table 2. Survival data and mean values of mice weight challenged with different doses of native AT
Antibodies responses in immunized mice
The anti-RTB-ATB antibody titers of sera, presented in , showed the antibodies responses following booster immunizations and 7 d after being challenged i.p. with native toxins by the ELISA. The data revealed that the antibody titers increased after each immunization, and the sera of immunized mice had no significant differences following the last vaccination. The mean IgG titers ranged from 1:106 to 1:107 after the fourth immunization and a strong secondary response was induced in vaccinated mice when challenged with RT or AT. In addition, the ELISA absorbance of sera from the PBS-vaccinated mice was less than 1:10, which is significantly different from those of the the chimeric protein-vaccinated groups (P < 0.05, data not shown).
Table 3. The anti-RTB-ATB IgG antibody titers of sera after each immunization and challenge trial
Performance of passive protection
The sera identified by the ELISA were used in an RTB-ATB neutralization assay ( and ). The survival results of the mice, which were treated with the mixture of immunized sera and RT in vitro, showed that the anti-RTB-ATB antibodies could completely neutralize 4 × LD50 dose of RT. In contrast, 90% mice in the control group, which were co-injected with the mixture of non-immunized sera and 2 or 4 × LD50 of RT, died within 2 d after administration, except that one mouse was alive with approximately 20% of body weight loss. Also, the same results were observed when mice in sera neutralization and passive protection challenged with native AT. These results suggested that the anti-RTB-ATB antiserum could neutralize 4 × LD50 of native toxins.
Table 4. Survival data and mean values of mice weight challenged with native RT
Table 5. Survival data and mean values of mice weight challenged with native AT
Discussion
RT and AT, which inhibit protein synthesis in eukaryotes, are extremely toxic plant proteins and very similar in structure and function. Although not infectious, the 2 toxins are classified as potential agents for use as biological warfare and bioterrorism because of their toxicity, stability, and the relatively easy purification procedure. The development of effective vaccine has received considerable attention. The US Army Medical Research Institute of Infectious Diseases (USAMRIID) has been conducting research and development of a RT vaccine since the late 1980s in an effort to provide prophylactic treatment for warfighters and other selected populations.Citation16 However, no clinical vaccines or therapeutics currently exist to protect against the exposure to deadly 2 toxins. Thus, it is important for a dual subunit vaccine to provide simultaneously effective protection from RT and AT exposure.
Although RTA has been studied for years as a subunit vaccine, previous studies also have demonstrated that B chain of toxins can exhibit both antigenic and adjuvant activity.Citation14,Citation15,Citation17 And compared with the prophylactic or therapeutic vaccine against RT or AT published previously,Citation1-Citation10 the chimeric protein vaccine exhibits several advantages as described above. Therefore, we developed a chimeric protein vaccine and investigated the immunogenicity and protective immunity generated by vaccination of RTB-ATB without adjuvant.
In this study, an optimizing gene encoding RTB and ATB was designed (), expressed and the gene product purified from E. coli using Ni2+-chelating affinity chromatography resin column (). And RTB-ATB was confirmed by western blot analysis (), which demonstrated the target protein could highly react with the rabbit pAb against native toxins, respectively.
After confirming that RTB-ATB could express in E. coli successfully, the novel vaccine was used to immunize mice by the different immunization schedules with a 2 × 2 × 2 × 2 factorial arrangement before challenge with toxins. The anti-RTB-ATB antibody titers of sera reached 1:106 or 1:107 after 4 continuous immunizations (). Our results indicated that all of the RTB-ATB-vaccinated mice survived after be challenged i.p. with 2 × LD50 of native toxins. As to 4 × LD50 challenge, only different routes of immunization injection caused significant difference (P < 0.05), i.p. administration of immunogen protected mice better than s.c. administration. Although there was no statistically significant difference was found in the different immunization time intervals and different doses of chimeric protein, 25 μg per mouse immunogen with immunization time interval Π seemed to protect mice better than the other immunization schedules according to the data. Therefore, i.p. administration of 25 μg per mouse immunogen with immunization time interval Π was believed the best immunization schedules and used to assess native toxins neutralization assay. No significant difference was observed among subgroups for the weight of survival mice challenged with 2 and 4 × LD50 of native toxins (P > 0.05) ( and ). In contrast, all of the PBS-vaccinated mice died within 2 d following challenge with 2 × LD50 of native toxins.
Furthermore, the results of sera neutralization assay revealed that the immunized sera (the same manner as group 8) could provide protective efficacy in naive mice which were treated with a mixture of immunized sera and 4 × LD50 of native toxins ( and ).
Therefore, we conclude that a normal immunological response can be induced by the chimeric protein in mice. And RTB-ATB is sufficient to prevent and protect in vaccinated mice when challenged with 4 × LD50 of RT or AT. The 6 × His tag, which we used in the chimeric protein in this study, has several advantages over other tags such as ease of purification, lack of immunogenicity, making it acceptable for use in laboratory research and even Phase 1 clinical trials. Even if the vaccine candidate requires further process, we can remove the tag by digestion easily.
Currently, RT or AT vaccine focused on toxoid or attenuated A chain subunit. Although the toxoids ultimately protected mice from exposure to lethal doses of native toxins respectively, they had residual toxicity, which were poisonous to animals and people. As to attenuated A chain subunit, RTA vaccine (such as Rivax)Citation2,Citation4,Citation11,Citation12 and ATA vaccine (such as mABRA)Citation3 both can confer complete protection after being challenged with 10 × LD50 of native RT or AT. Although our results showed that RTB-ATB elicited a protective immune response which can provide protective efficacy following challenge with a low 4 × LD50 of RT or AT, the B chain of 2 toxins was first showed to triger a good immune response and protective efficacy without adjuvant. More importantly, chimeric protein is non-toxic, which means it is more safety to be used in both animals and humans.
In summary, we have designed and constructed a chimeric vaccine RTB-ATB with good immunogenicity and immunoreactivity, which could elicit specific neutralizing antibodies against native toxins at a dose 4 times higher than the LD50 of RT or AT, when administered i.p. in the absence of adjuvant. Altogether, RTB-ATB is a promising vaccine candidate and should be investigated deeply.
Materials and Methods
Construction of the RTB-ATB expression plasmid
According to the inclination for codon usage in E. coli, an optimum design of 1647bp gene, encoding RTB gene fragment (GenBank accession number X52908) and ATB amino acid sequence (GenBank accession number 1908235A), was performed by replacing rare codons with synonymous codons of high-frequency. A flexible linker (G4S)3 was introduced between the 2 fragments of the construction chimeric protein. Then the full-length gene was synthesized (Invitrogen) and cloned into the BamH І and Hind Ш restriction sites of E. coli expression plasmid pQE-80L. This new plasmid pQE80L-RTB/ATB was analyzed by PCR and restriction digest after it was transformed into E. coli M15 (Qiagen).
Expression and purification of the chimeric protein
The E. coli strain M15 cells containing pQE80L-RTB/ATB was grown in Luria Bertani (LB) medium supplemented with 100 µg/ml ampicillin at 37 °C in a shaker until an optical density at 600 nm was 0.6~0.8. When the temperature was decreased to 30 °C, 1M IPTG was added to a final concentration of 0.1 mM for an additional 5 h. After induction, the cells were harvested by centrifugation and washed with cold phosphate-buffered saline solution (PBS). The pellets were resuspended by the addition of a 0.2-fold volume of PBS and lysed by sonication. Then the bacterial lysates were centrifuged at 12 000 rpm for 30 min, and the supernatant and precipitates were analyzed by 15% sodium dodecyl sulfate PAGE (SDS-PAGE) to identify RTB-ATB expression products.
One liter of LB broth was used to prepare the large scale of the chimeric protein. The bacteria cells were collected, lysed, and centrifuged for extraction of insoluble RTB-ATB formed in the inclusion bodies. Inclusion bodies were washed 4 times with wash buffer (2% Triton X-100, 2% Tween-20, 1 M NaCl, 2 M urea) and dissolved in 100 ml buffer A (20 mM NaH2PO4, 500 mM NaCl, 10 mM imidazole, 8 M urea, pH 8.0). After the precipitate dissolved completely, the solution was filtered 3 times (3.0 μm, 0.8 μm, then 0.45 μm). The recombinant product then was purified through a HiTrap Chelating high performance 5 mL column pre-packed with a precharged Ni2+ column (Amersham) and eluted with buffer B containing 300 mM imidazole. After the fractions were pooled, the solution was transferred to the renaturation buffer (PBS containing 4 M, 2 M, 1 M, 0.5 M, and 0 M urea) and stirred gently at 4 °C for not less than 24 h. The chimeric protein RTB-ATB was confirmed using SDS-PAGE followed by coomassie blue staining and the BCA assay kit was applied to measure the concentration.
Immunoblotting
Western blotting was employed to further identify the immunogenicity of RTB-ATB. Using a semi-dry electrophoretic transfer device (Amersham Biosciences), purified protein was transferred from a 15% SDS-PAGE to an electro-transferred nitrocellulose membrane. Nonspecific binding sites were blocked overnight at 4 °C in blocking buffer (3% BSA in PBS, pH 7.4, with 0.5% Tween-20). The membrane was incubated in a 1:1000 dilution of rabbit anti-RT polyclonal antibody (pAb) or rabbit anti-AT pAb (previously prepared in our laboratory) for 1h at room temperature, respectively. Then the membrane was washed 3 times with PBST (PBS containing 0.5% Tween-20). HRP-conjugated goat anti-rabbit IgG (Sigma) (1:50 000) was subsequently used at room temperature for 1 h. Finally, the membrane was incubated with SuperSignal Substrate Working Solution (Thermo) for 5 min, and exposed in AE-1000 cool CCD image analyzer (Beijing BGI-GBI Biotech Co., Ltd).
Vaccination of mice and challenge with native toxins
As B chain of the A–B family of toxins contains adjuvant activity, the chimeric protein was administered without other adjuvants in our experiments. Eighty specific pathogen-free (SPF) female Balb/c mice (purchased from Laboratory Animal Center, The Academy of Military Medical Sciences) 6 wk of age were randomly distributed to 16 experimental groups. A 2 × 2 × 2 × 2 factorial arrangement in a completely randomized design was used; dose of RTB-ATB (15 or 25 μg per mouse), route of immunization (i.p. or s.c.) injection, immunization time interval [time interval І (days 0, 7, 14, and 21) or time interval Π (days 0, 14, 28, and 42)], and dose of RT challenge (2, 4 × LD50) were the main effects (). Another 80 mice were treated in the same way by AT challenge. One week after the last injection, all mice were challenged with different doses of RT or AT. As negative control groups, another 20 mice, named PBS-vaccinated mice and vaccinated only with PBS, were assigned randomly to 4 groups and received time interval І or time interval Π respectively, then were challenged with 2 × LD50 of RT or AT. The weights and deaths of animals were recorded daily for 10 d, a time sufficient for all mice to gain or lose any weight after the challenge. Animal studies were conducted in compliance with the Guideline for the Care and Use of Laboratory Animals and the Association for Assessment and Accreditation of Laboratory Animal Care International.
Table 6. Immunization schedules challenged with different doses of RT or AT
Measurement of antibody titers
Serum, which were sampled from the caudal vein of every individual mouse one week after each vaccination and one week after challenge with native toxins, were screened for anti-RTB-ATB antibodies by an enzyme-linked immunosorbent assay (ELISA). A 96-well plate was coated with 100 μl purified RTB-ATB (5 μg/mL) overnight at 4 °C, and then washed with PBST and blocked with 3% BSA in 0.01 mM PBS. Serum samples were serially diluted 1:10 and 100 μL was added to each well at 37 °C. After incubation for 1 h and washing with PBST, 100 μL of a 1:5000 dilution of HRP-coupled goat anti-mouse IgG antibodies (Sigma) was added for 1h at 37 °C. After washing with PBST, anti-RTB-ATB reactivity was visualized by adding the substrate solution for 10 min at room temperature. 2M H2SO4 was then used to stop the reaction and the absorbance was read at 450 nm using a microplate reader (Molecular Device). The results were considered as positive when the values of OD450 were greater than 2.1-fold negative control.
Neutralization assay
New 60 mice were used for passive protection. The collected sera samples containing anti-RTB-ATB antibody from 20 mice vaccinated in the same immunization schedule as group 8 were detected for ability to protect mice against toxins challenge. Mixtures of RT or AT in 50 mM PBS containing an equal volume of sera from mice vaccinated were incubated at 37 °C for 30 min before injection. Then, 20 naive BALB/c mice were randomly divided into 4 groups and injected i.p. with the mixtures of 2 or 4 × LD50 of native toxins and immunized sera using a volume of 500 μL/mouse, respectively. As control, another 20 naive mice were treated with the mixtures of 2 or 4 × LD50 of native toxins containing non-vaccinated sera. The mice were monitored for 10 d, and the weights and survival were recorded.
Statistical analysis
A randomized complete design with a 2 × 2 × 2 × 2 factorial arrangement of treatment was used to evaluate RTB-ATB; dose of RTB-ATB, route of injection, immunization time interval, and dose of native toxins challenge were the main effects. Data were expressed as mean ± standard deviation (S.D.) and subjected to ANOVA and t test procedures. Comparisons among groups were made using the Duncan multiple-range test in which groups were more than 2, and t test was used in comparing 2 major groupings. A 2 × 2 × 2 multi-layered Chi-Square analysis, using Cochran-Mantel-Haenszel test was conducted to determine statistical differences in survival between the treatment groups. For all tests only data resulting in P values of P < 0.05 were considered as statistically significant.
Abbreviations: | ||
RT | = | ricin toxin |
RTB | = | ricin toxin B chain |
RTA | = | ricin toxin A chain |
AT | = | abrin toxin |
ATB | = | abrin toxin B chain |
E. coli | = | Escherichia coli |
LD50 | = | 50% lethal dose |
i.p. | = | intraperitoneal or intraperitoneally |
s.c. | = | subcutaneous or subcutaneously |
PBS | = | phosphate-buffered saline solution |
SDS-PAGE | = | sodium dodecyl sulfate polyacrylamide gel electrophoresis |
ELISA | = | enzyme-linked immunosorbent assay |
pAb | = | polyclonal antibody |
TMB | = | tetramethyl benzidine |
Disclosure of Potential Conflicts of Interest
No potential conflicts of interest were disclosed.
Acknowledgments
We thank Dr Jinwen Chen (School of Vet Medicine, University of Pennsylvania) for his critical reading of the manuscript.
References
- Surendranath K, Karande AA. A neutralizing antibody to the a chain of abrin inhibits abrin toxicity both in vitro and in vivo. Clin Vaccine Immunol 2008; 15:737 - 43; http://dx.doi.org/10.1128/CVI.00254-07; PMID: 18353919
- Smallshaw JE, Richardson JA, Vitetta ES. RiVax, a recombinant ricin subunit vaccine, protects mice against ricin delivered by gavage or aerosol. Vaccine 2007; 25:7459 - 69; http://dx.doi.org/10.1016/j.vaccine.2007.08.018; PMID: 17875350
- Han YH, Gao S, Xin WW, Kang L, Wang JL. A recombinant mutant abrin A chain expressed in Escherichia coli can be used as an effective vaccine candidate. Hum Vaccin 2011; 7:838 - 44; http://dx.doi.org/10.4161/hv.7.8.16258; PMID: 21817853
- Smallshaw JE, Richardson JA, Pincus S, Schindler J, Vitetta ES. Preclinical toxicity and efficacy testing of RiVax, a recombinant protein vaccine against ricin. Vaccine 2005; 23:4775 - 84; http://dx.doi.org/10.1016/j.vaccine.2005.04.037; PMID: 15961194
- Kende M, Yan C, Hewetson J, Frick MA, Rill WL, Tammariello R. Oral immunization of mice with ricin toxoid vaccine encapsulated in polymeric microspheres against aerosol challenge. Vaccine 2002; 20:1681 - 91; http://dx.doi.org/10.1016/S0264-410X(01)00484-4; PMID: 11858879
- Griffiths GD, Lindsay CD, Allenby AC, Bailey SC, Scawin JW, Rice P, Upshall DG. Protection against inhalation toxicity of ricin and abrin by immunisation. Hum Exp Toxicol 1995; 14:155 - 64; http://dx.doi.org/10.1177/096032719501400201; PMID: 7779439
- Hu WG, Yin J, Chau D, Negrych LM, Cherwonogrodzky JW. Humanization and characterization of an anti-ricin neutralization monoclonal antibody. PLoS One 2012; 7:e45595; http://dx.doi.org/10.1371/journal.pone.0045595; PMID: 23049820
- Hu WG, Yin J, Chau D, Hu CC, Lillico D, Yu J, Negrych LM, Cherwonogrodzky JW. Conformation-dependent high-affinity potent ricin-neutralizing monoclonal antibodies. Biomed Res Int 2013; 2013:471346; http://dx.doi.org/10.1155/2013/471346; PMID: 23484120
- Yermakova A, Mantis NJ. Protective immunity to ricin toxin conferred by antibodies against the toxin’s binding subunit (RTB). Vaccine 2011; 29:7925 - 35; http://dx.doi.org/10.1016/j.vaccine.2011.08.075; PMID: 21872634
- Vallera DA, Burns LJ, Frankel AE, Sicheneder AR, Gunther R, Gajl-Peczalska K, Pennell CA, Kersey JH. Laboratory preparation of a deglycosylated ricin toxin A chain containing immunotoxin directed against a CD7 T lineage differentiation antigen for phase I human clinical studies involving T cell malignancies. J Immunol Methods 1996; 197:69 - 83; http://dx.doi.org/10.1016/0022-1759(96)00127-5; PMID: 8890895
- Smallshaw JE, Vitetta ES. A lyophilized formulation of RiVax, a recombinant ricin subunit vaccine, retains immunogenicity. Vaccine 2010; 28:2428 - 35; http://dx.doi.org/10.1016/j.vaccine.2009.12.081; PMID: 20074685
- Smallshaw JE, Firan A, Fulmer JR, Ruback SL, Ghetie V, Vitetta ES. A novel recombinant vaccine which protects mice against ricin intoxication. Vaccine 2002; 20:3422 - 7; http://dx.doi.org/10.1016/S0264-410X(02)00312-2; PMID: 12213413
- Wang L, Liu XF, Yun S, Yuan XP, Mao XH, Wu C, Zhang WJ, Liu KY, Guo G, Lu DS, et al. Protection against Helicobacter pylori infection by a trivalent fusion vaccine based on a fragment of urease B-UreB414. J Microbiol 2010; 48:223 - 8; http://dx.doi.org/10.1007/s12275-009-0233-4; PMID: 20437155
- Mantis NJ. Vaccines against the category B toxins: Staphylococcal enterotoxin B, epsilon toxin and ricin. Adv Drug Deliv Rev 2005; 57:1424 - 39; http://dx.doi.org/10.1016/j.addr.2005.01.017; PMID: 15935880
- Liu W, Xu N, Yuan H, Li S, Liu L, Pu Z, Wan J, Wang H, Chang Y, Li R. Immunomodulatory activity of recombinant ricin toxin binding subunit B (RTB). Int J Mol Sci 2013; 14:12401 - 10; http://dx.doi.org/10.3390/ijms140612401; PMID: 23765218
- McLain DE, Lewis BS, Chapman JL, Wannemacher RW, Lindsey CY, Smith LA. Protective effect of two recombinant ricin subunit vaccines in the New Zealand white rabbit subjected to a lethal aerosolized ricin challenge: survival, immunological response, and histopathological findings. Toxicol Sci 2012; 126:72 - 83; http://dx.doi.org/10.1093/toxsci/kfr274; PMID: 21987460
- Medina-Bolivar F, Wright R, Funk V, Sentz D, Barroso L, Wilkins TD, Petri W Jr., Cramer CL. A non-toxic lectin for antigen delivery of plant-based mucosal vaccines. Vaccine 2003; 21:997 - 1005; http://dx.doi.org/10.1016/S0264-410X(02)00551-0; PMID: 12547614