Abstract
The coxsackie A16 virus (CA16), along with enterovirus 71 (EV71), is a primary pathogen that causes hand, foot, and mouth disease (HFMD). To control HFMD, CA16, and EV71 vaccines are needed. In this study, an experimental inactivated CA16 vaccine was prepared using human diploid cells, and the vaccine’s immunogenicity was analyzed in mice and rhesus monkeys. The results showed that the neutralizing antibody was developed in a dose-dependent manner, and was sustained for 70 days with an average GMT (geometric mean titer) level of 80 to 90 in immunized mouse and for 56 days with GMT of higher than 300 in monkeys. The neutralizing antibody had a cross-neutralizing activity against different viral strains (genotype A and B), and the specific IFN-γ-secreting cell response was activated by these virus strains in an ELISPOT assay. This study provides evidence for the potential use of inactivated CA16 as a candidate for use in vaccines.
Introduction
Previous etiological studies of hand, foot, and mouth disease (HFMD) in children throughout many Asian-Pacific countries have demonstrated that 2 members of the Enterovirus A genus of the Picornaviridae family (Coxsackie A16 virus [CA16] and Enterovirus 71 [EV71]) are the primary pathogens that cause HFMD.Citation1-Citation3 There have been multiple reports demonstrating that HFMD fatalities are frequently caused by EV71 rather than CA16 infections.Citation4,Citation5 However, some studies have identified HFMD fatalities that are specifically caused by CA16 infection, noting the potential epidemiological threat that CA16 poses to the health of children.Citation6,Citation7 This finding suggests that an emphasis should be placed on developing candidate CA16 vaccines to help to control and prevent HFMD epidemics.Citation8 Nevertheless, the pathogenesis of HFMD caused by CA16 remains largely unknown.Citation9 As a result, inactivated or subunit vaccines are believed to be a feasible alternative for preventing CA16 infection due to their controllable safety compared with that of attenuated vaccines.Citation10-Citation12 Recently, it has been reported that an experimental, inactivated CA16 vaccine candidate provides effective protection against CA16 infection by inducing a neutralizing antibody response in mice; this response was demonstrated using an immunological analysis (neutralizing antibodies assay and lethal challenge analysis).Citation12 Although some studies report that mice could be a candidate model for HFMD, the characterization of this immune response in different animal models (especially in primates, who share a close relationship to humans) would provide more support for these studies.Citation13 In this study, we characterized the immune response of mice and rhesus monkeys induced by an experimental inactivated vaccine prepared from CA16 virus that was adapted to grow in a human diploid cell line. The results showed that the immune response induced by this candidate vaccine could produce a specific neutralizing antibody and a specific IFN-γ-secreting cellular response against viral strains that were of different or similar genotypes; thus, this inactivated CA16 is a candidate vaccine.
Results
Characterization of the inactivated CA16 vaccine prepared from human diploid cells
Because the target population for the inactivated CA16 vaccine includes infants, human diploid cells are generally considered to be the appropriate cell substrate for the vaccine preparation. The harvested virus was inactivated with formaldehyde at a volume ratio of viral stocks to formaldehyde of 1:4000 for 96 h before 50-fold concentration and chromatography purification. Antigen detection tests on different fractions of the purified virus revealed 2 distinctive distribution peaks containing viral antigens (), which were collected 75 and 103 min after column purification. As shown by electron microscopy, the majority of virions were found in the second peak (), with no virus observed in images from the first peak (data not shown). Thus, the virions from the second peak were selected for the preparation of the experimental vaccine. The subsequent protein gel analysis indicated that the typical VP0, VP1, VP2, and VP3 structural bands were observed for this virion sample (). The antigenicity of these structural proteins was further confirmed by western blotting (). A CA16 antigen ELISA kit was used to determine the antigen content of this inactivated CA16 vaccine, and the vaccine formulations were mixed with Al(OH)3 (0.5 mL/dose) for use in subsequent immunological experiments.Citation14
Figure 1. Preparation of the experimental inactivated CA16 vaccine and its characterization. (A) Purification of experimental inactivated CA16 vaccine in bulk and measurements of the antigen distribution. The inactivated CA16 bulk was purified through Sepharose 6FF; samples were collected every minute until the baseline became stable. The profile of the viral antigen titers for each sample (1 mL per sample) was measured using an ELISA assay with anti-CA16 polyclonal antiserum. (B) Electron microscopy images showing the antigen peak distributed in the second fraction containing virions. The black arrow indicates the complete viral particles (solid virion), and the white arrow indicates the virion-containing capsids without RNA (empty virion). The bar represents 100 nm. (C) SDS-PAGE analysis of the bulk-produced experimental vaccine. The bulk-produced experimental vaccine was analyzed on 12% polyacrylamide gels via silver staining. (D) Western blotting with anti-CA16 antibody. The CA16 antigens were detected via western blot using rabbit anti-CA16 sera.
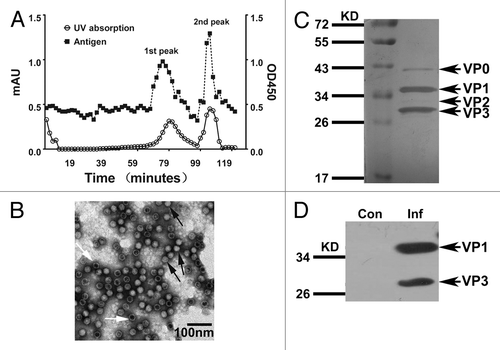
The dynamic profile of neutralizing antibody induced by the experimental inactivated CA16 vaccine in mice
On the basis of previous immunological data for multiple inactivated enterovirus vaccines, such as the hepatitis A and EV71 vaccines,Citation15,Citation16 we used different doses of the inactivated CA16 vaccine to immunize mice at days 0, 28, and 56 to observe the dynamic profile of the induced neutralizing antibodies. The neutralizing antibody responses increased after the primary immunization, first booster, and second booster until day 28 after the second booster. A positive correlation between the number of immunizations and neutralizing antibody GMTs were observed, as well as a remarkable dose effect between the immunization dose and the neutralizing antibody GMTs (). Additionally, the neutralizing antibody GMTs tended to show significant elevation when the antigen dose was greater than 200 EU (). Notably, the neutralizing antibody GMTs tended to increase after 3 immunizations () but decreased to 1/5 of the peak value at day 28 after the 3rd immunization (). As shown in the cross-neutralization assay using the pooled anti-sera collected at day 14 after the booster immunization against the genotype B (G20 and MA154) and genotype A strain (G10), the neutralizing antibody induced by the vaccine exhibited higher cross reactivity with the G20 and MA154 strains and lower reactivity to G10 ().
Figure 2. The dynamic profile and cross-neutralization reactivity for neutralizing antibody induced using immunization with the experimental inactivated CA16 vaccine in mice. (A) Neutralizing antibody dynamic profile. Neutralization titers (GMTs) of the serum from immunized mice via the 400 EU (n = 8), 200 EU (n = 8), 100 EU (n = 8), 50 EU (n = 8), 25 EU (n = 8), and 12.5 EU (n = 8) of inactivated CA16 vaccine or PBS (control, n = 8) collected at day 28, 35, 42, 70, and 84 after the first immunization were determined via neutralization test at the highest dilution that prevented the cells from experiencing cytopathic effects. Each symbol represents a dose, and the line indicates the variation of the antibody in the group. (B) Cross-neutralization test. The cross-neutralization of the serum was detected with the G20, G10, and MA154 virus strains, respectively. The neutralization titers (GMTs+95%CI) of the sera from immunized mice via 400 EU (n = 8), 200 EU (n = 8), 100 EU (n = 8), 50 EU (n = 8), 25 EU (n = 8), and 12.5 EU (n = 8) of the inactivated CA16 vaccine or PBS (control, n = 8) collected at day 70 after the first immunization were determined using a neutralization test at the highest dilution that prevented the cells from experiencing cytopathic effects.
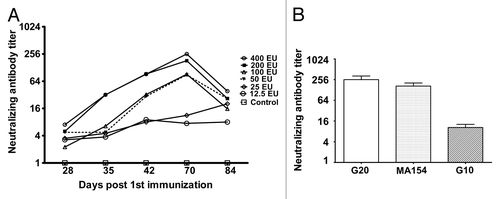
Immunoprotective efficacy against challenge by different viral strains in mice immunized with the candidate inactivated CA16 vaccine
Although there is currently no animal model for CA16 virus infection, the use of passive immunization in suckling mice for the observation of survival rates upon intracerebral viral challenge is frequently used to evaluate the efficacy of inactivated CA16 vaccine candidates.Citation17 We evaluated the immune protection efficacy of the inactivated CA16 vaccine candidate in suckling mice born from immunized maternal mice using intracerebral viral challenge to mimic the principal evaluation method used for the EV71 vaccine,Citation18 which is believed to provide additional evidence for the evaluation of inactivated CA16 vaccine candidates. In our study, the protection efficacy of the inactivated CA16 vaccine candidate was evaluated in suckling mice born from maternal mice immunized 3 times with different doses of vaccine; mice that received a lethal challenge using different viral strains served as controls. The results demonstrated clear immune protection efficacy in suckling mice born from maternal mice that were immunized with the inactivated CA16 vaccine () and similar efficacy for challenge with the genotype A strain (G10 []), the genotype B strains (MA154 []), and the original vaccine strain G20 (). Additionally, the results indicated a dose-dependent association between the immunization dosage and the immune protective efficacy ().
Figure 3. The immune protective efficacy of the candidate inactivated CA16 vaccine against infection with different challenge viruses in a neonatal passive immunity mouse model. Three groups of suckling mice (24–48 h after birth) obtained from the immunized maternal mice of each dosage group after the secondary immunization were injected intracerebrally with either CA16 virus G-20, MA154, or G10 (104CCID50 per mouse), and the control group from the parents was injected with the 20 µl Al(OH)3 adjuvant. Subsequently, the survival rates were recorded daily after infection for a period of 15 d. (A) G20 challenge group: 400 EU x 2n (n = 11–12), 200 EU × 2n (n = 12–13), 100 EU × 2n (n = 10–12), 50 EU × 2n (n = 12–15), 25 EU × 2n (n = 11–12), 12.5 EU × 2n (n = 12–13), n.c (negative control) × 2n (n = 11–12). 2n indicates the number (n) of mice in 2 replicates of a dose group. (B) G10 challenge group: 400 EU × 2n (n = 12–13), 200 EU × 2n (n = 10–11), 100 EU × 2n (n = 10–11), 50 EU × 2n (n = 12–15), 25 EU × 2n (n = 11–16), 12.5 EU × 2n (n = 13–15), n.c × 2n (n = 10–12). (C) MA154 challenge group: 400 EU × 2n (n = 10–11), 200 EU × 2n (n = 12–13), 100 EU × 2n (n = 10–13), 50 EU × 2n (n = 12–13), 25 EU × 2n (n = 11–15), 12.5 EU × 2n (n = 10–13), n.c × 2n (n = 12–14)
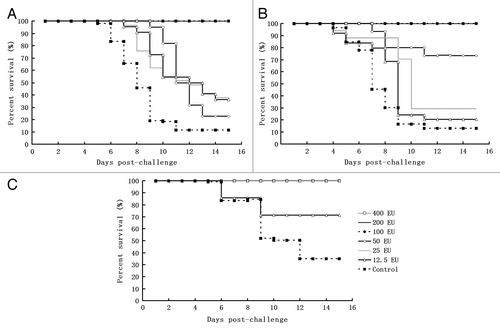
The dynamic profile of neutralizing antibodies induced by the inactivated CA16 vaccine candidate in rhesus monkeys
An immunological analysis in mice immunized with the inactivated vaccine candidate is generally recognized as an effective approach for evaluating vaccine efficacy. However, as with the immunological analysis of any novel inactivated viral vaccine, the comparison of data from mice with data from primates, who share a closer relationship with humans, is considered to be even more reliable and significant.Citation19 Therefore, we performed immunological studies in 4 groups of rhesus monkeys (4 monkeys included in each group), each immunized subcutaneously with one of 3 different doses (1000 EU, 600 EU, or 200 EU) of vaccine or Al(OH)3 adjuvant at days 0 and 28. The dynamic profile of neutralizing antibody responses in each immunized monkey was evaluated. The cross-neutralizing reactivity of each peak serum sample was also tested against different viral strains. At day 28 after the initial immunization, the antibody titer in experimental animals that had been immunized with any of the 3 doses of vaccine showed very low seroconversion (1:4), whereas the results showed high seroconversion (>1:64) at day 7 of the booster immunization. This latter result was demonstrated by an obvious elevation of GMTs that was consistently sustained until day 28 after the booster immunization and subsequently slowly declined during days 35–43 of the booster immunization (). In parallel, the dose-effect association of neutralizing GMTs was identified only in the low- and middle-dose groups. Although the reason for this finding remains largely unknown, the occurrence of an obvious neutralizing antibody response appears to suggest that the candidate vaccine possesses effective immunogenicity. Furthermore, the sera of the higher neutralizing antibody (1:512) at day 14 after booster immunization were pooled; with regard to the neutralizing potency against 3 different viral strains in the cross-neutralization assays, the response to the G10 strain was found to be comparatively lower (1:16) but still in the range of protective significance (), whereas the responses to the other 2 viral strains were found to be significantly higher ().
Figure 4. The dynamic profile and cross-neutralization reactivity for neutralizing antibody induced via immunization with the experimental inactivated CA16 vaccine in rhesus monkeys. (A) Neutralizing antibody dynamic profile. Neutralization titers (GMTs + 95%CI) of the sera collected from immunized rhesus monkeys via the 1000 EU (n = 4), 600 EU (n = 4), and 200 EU (n = 4) of inactivated CA16 vaccine or PBS (control, n = 4) at day 28, 35, 42, 56, 63, and 70 after the first immunization were determined via a neutralization test based upon the highest serum dilution that prevented cells from experiencing cytopathic effects. Each symbol represents a dose, and the line indicates the geometric mean titer (GMT) of the group. (B) Cross-neutralization reactivity. The cross-neutralization antibody titers were detected with G20, G10, or MA154. Neutralization titers (GMTs + 95%CI) of the sera from immunized rhesus monkeys via the 1000 EU (n = 4), 600 EU (n = 4), and 200 EU (n = 4) of inactivated CA16 vaccine or PBS (control, n = 4) collected at day 42 after the first immunization were determined via a neutralization test at the highest dilution that prevented the cells from experiencing cytopathic effects.
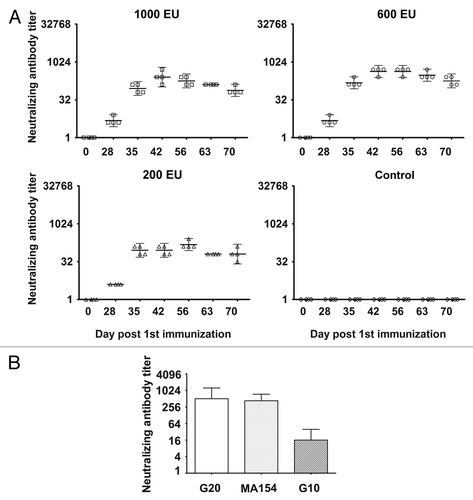
The specific IFN-γ-secreting cell response induced by the inactivated CA16 vaccine candidate in rhesus monkeys
The above experiments demonstrated that the inactivated CA16 vaccine has the capacity to induce a remarkable neutralizing antibody response in immunized rhesus monkeys. Currently, the neutralizing antibody response is widely recognized as an indicator for evaluating vaccine efficacy. However, characterizing the basic immune response induced in rhesus monkeys immunized with the candidate inactivated CA16 vaccine would provide direct evidence of the mechanisms of immunological responses in humans. Compared with the control monkeys, the IFN-γ-specific ELISPOT assay on PBMCs from immunized rhesus monkeys revealed an active response with remarkable cell proliferation specifically against CA16 antigen stimulation; this response hand the tendency to show dose-dependent effects at day 56 of the primary immunization (). Similar responses were also identified in PBMCs stimulated with other viral strain antigens of different genotypes (). The measurement of different pro-inflammatory cytokine levels in monkey serum from different time points before or after immunization failed to exhibit any variations ().
Figure 5. Characterization of immune responses induced in rhesus monkeys by the candidate inactivated CA16 vaccine. Four groups of rhesus monkeys were subcutaneously immunized with CA16 vaccines of different doses and Al(OH)3 adjuvant as described in the Figure S1 legend. PBMCs were collected on 0 and 56 d after first immunization and analyzed with the IFN-γ Elispot Kit. The pro-inflammatory factors of the sera collected at same time points were measured with a CBA Kit as described in Materials and Methods. (A) Stimulation with the G20 viral strain. (B) Stimulation with the G10 viral strain. (C) Stimulation with the MF154 viral strain. (D) Measurement of pro-inflammatory cytokine levels. The data are shown as means ± SD. Two replicates were performed for each sample.
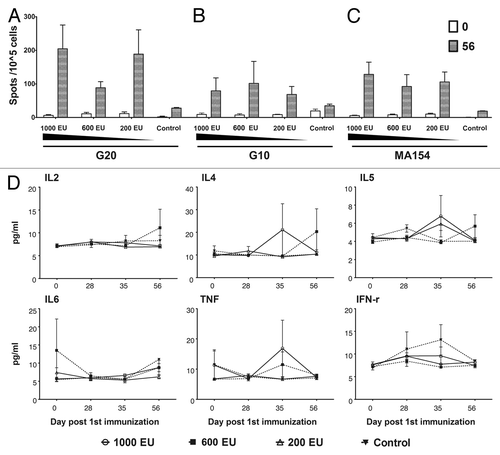
Discussion
Epidemiological studies have demonstrated that CA16 remains one of the primary pathogens causing HFMD and likely poses a significant challenge in vaccine development,Citation20 as secondary infection with CA16 has been occasionally observed in children who have had HFMD, especially among infants.Citation21 Nevertheless, whether such a secondary infection is due to a different viral strain or an abnormal immune response initiated during the first infection remains unknown. Therefore, further characterization of the immune response induced by the inactivated CA16 vaccine would contribute to a better understanding of the vaccine’s clinical significance.
Recently, some studies have demonstrated the efficacy of the inactivated CA16 vaccine candidate in a murine infection model.Citation12 Our current study provides further evidence for a protective immune response that offers cross-neutralization antibody reactivity in mice. Our study also provides evidence for the protective efficacy induced in suckling mice born from immunized maternal mice against lethal challenge with viral strains of different genotypes. This immune response was shown to generate neutralizing antibodies in a dose-dependent manner. Although we lack detailed serological data from patients infected with CA16 to use in an evaluation of the protective neutralizing antibody titer, the nearly 100% immune protective efficacy in animals associated with a neutralizing titer has generally been acceptable for a new vaccine. Whether this efficacy gradually declines with time warrants further investigation. Furthermore, the fact that this neutralizing antibody showed the capability to interact with various viral strains of different genotypes suggests the applicability of this vaccine to the prevention of CA16 infection in certain populations based upon the current epidemic situations.Citation22,Citation23 In the immunological analysis in rhesus monkeys, the increased amount of antigen in the high-dose group (1000 EU/dose) and the low-dose group (200 EU/dose) demonstrated that there was a persistently increasing neutralizing antibody response in monkeys after the booster immunization. Interestingly, the middle-dose group (600 EU/dose) had the highest neutralizing antibody GMT, and the average GMT levels were maintained at >1:300 between days 42 and 56 of the primary immunization; in contrast, the GMTs in the high- and low-dose groups were lower. Although the reasons for this finding remain largely unknown, this phenomenon may be used to partially elucidate the immune response mechanisms in rhesus monkeys. As shown by the neutralizing antibody response induced in the monkeys, the considerably higher antibody GMTs most likely exhibited a cross-neutralization ability to different genotype viral strains rather than the lower antibody GMTs; this is an essential issue to consider when determining the antigen dosage for the development of candidate inactivated CA16 vaccines.
Another interesting finding was that, regardless of the lower cross-neutralization reactivity of antiserum to different genotype strains, the IFN-γ-specific ELISPOT assay revealed an obvious cellular immune response that was induced promptly upon stimulation with antigens purified from different genotype strains. Furthermore, the specific IFN-γ-secreting cell response exhibited a dose-dependent response to stimulation with vaccine strains and related antigens. Taken together, these results allow us to draw conclusions regarding the systemic significance of the immune response induced by the inactivated CA16 vaccine candidate. This vaccine is capable of providing effective protection in mice against viral challenge and of inducing the neutralizing antibody response associated with specific IFN-γ-secreting cell responses in rhesus monkey. However, as for any novel candidate vaccine, further comprehensive and systematic studies are vital.
Materials and Methods
Viruses and cells
The CA16 G20 viral strain classified as genotype B was isolated in 2010 from a patient in the HFMD outbreak in the Guangxi Province of China. The G10 strain classified as genotype A was provided by the WHO in 1980 and has since been maintained in our lab. The MA154 strain classified as genotype B was isolated in 2011 from a child patient in the HFMD outbreak in Kunming, China. Vero cells at passages 146 to 148 were provided by the WHO for the production of polio vaccines. The human diploid cell line KMB17 was established by our instituteCitation24 and has been approved by the Chinese Food and Drug Administration (CFDA) for vaccine production. The 2 cell lines were grown into confluent monolayers in DMEM with 8% bovine serum for use in subsequent experiments.
Preparation of experimental inactivated CA16 vaccine
The G20 virus was adapted to grow in Vero cells and the human diploid cell line, KMB17, for 3 passages each. The viral stocks were tested for establishing primary, master, and working seed banks. The working seed lot was inoculated at a multiplicity of infection (MOI) of 0.05 onto KMB17 cells grown into a confluent monolayer in a cell factory. The virus was inactivated during harvest and concentrated 50-fold for subsequent Sepharose 6FF chromatography purification. The purified virus was diluted based on antigen quantification tests, and quality controls were performed. The dilution was absorbed in Al(OH)3 for subsequent filling and was qualified using controls for use in animal experiments.
Viral infectivity titration
The virus samples harvested from cell cultures were analyzed with a microtitration assay. Briefly, the virus sample was diluted sequentially 10-fold and used to inoculate monolayer of Vero cells (104 cells) in a 96-well plate with a volume of 50 µL/well and 8 wells for each dilution. The extent of the cytopathic effect in the inoculated cells was observed to determine the viral titer based upon the standard protocol.Citation25
Viral antigen titration
The viral antigen was titrated for each fraction using an enzyme-linked immunosorbent assay (ELISA). The experiments were performed following a previously described protocol.Citation14 The polyclonal anti-CA16 antiserum was collected from rabbits immunized with purified CA16 antigen; the purity of the anti-serum after separation with a Montage Antibody purification kitCitation26 was analyzed via SDS-PAGE.
Briefly, the ELISA plate was coated with purified polyclonal anti-CA16 antiserum; the purified CA16 virus collected via Sepharose 6FF chromatography and a reference standard were then added respectively. After incubation at 37 °C for 2 h, the plates were washed 5 times followed by incubation with HRP-conjugated anti-CA16 antiserum at 37 °C for 1 h. The plates were then again washed 5 times, and TMB peroxidase substrate (SureBlue; KPL) was added. The plates were maintained at 37 °C for 10 min, and the ending solution was then added. The results were read within 10 min at an absorbance of 450 nm in an ELISA Reader (Synergy 4; General Electric Co.).
Electron microscopy
The purified CA16 virus suspension was adsorbed onto carbon Formvar-coated copper grids and negatively stained with 2% phosphotungstic acid (pH 6.5) aqueous solution for 2 min for further viewing via transmission electron microscopy (Hitachi H7650).
SDS-PAGE and western blot analysis
The purified CA16 virus was mixed with sample buffer loaded onto a 12% SDS-PAGE gel for separation via an electrophoresis of 100 V and visualized via silver staining.Citation27 For immunoblotting, the viral proteins were directly electro-transferred onto a PVDF membrane (Merck Millipore). The membrane was sequentially treated with skim milk, rabbit anti-CVA16 serum (Institute of Medical Biology), and horseradish peroxidase (HRP)-conjugated goat anti-rabbit secondary antibody (Boster Biological Technology). The result was visualized using a chemiluminescent substrate and imaged using Kodak film (Carestream Kodak).
Testing the immune response to the inactivated CA16 vaccine in mice
Fifty-six adult ICR mice were divided into 7 experimental groups of 8 mice each (2 male and 6 female). Each group of mice was intraperitoneally (I.P.) immunized with the vaccine at 400 EU, 200 EU, 100 EU, 50 EU, 25 EU, or 12.5 EU /dose, respectively, or with Al(OH)3 adjuvant alone as a negative control. The males and females of the same group were initially held in different cages. After the primary injection, all mice received the booster injections on days 28 and 56. Blood samples were used for neutralization assays at days 28, 35, 42, 70, and 84 after the primary injection. The mice from each group were allowed to mate at day 28 after the second booster injection to breed neonatal mice for a lethal virus challenge, as described by Mao et al.Citation17
Testing the immune response to the inactivated CA16 vaccine in rhesus monkeys
A total of 16 rhesus monkeys (age 2 to 3.5 y) were divided into 4 groups of 4 monkeys each. Each group was subcutaneously immunized with inactivated vaccine at 1000 EU, 600 EU, or 200 EU/dose or with Al(OH)3 adjuvant alone as a negative control at day 0 and 28. Blood samples were tested for neutralizing antibodies at day 0, 28, 35, 42, 56, 63, and 70 after the primary injection, while the blood samples at day 0, 28, 35, and 56 were used for cytokine analysis. Peripheral blood mononuclear cell (PBMC) samples were collected from the monkeys at days 0 and 56 for the ELISPOT assay. All animal procedures were approved by the Office of Laboratory Animal Management of Yunnan Province, China.
Anti-CA16 neutralizing antibody titer measurements
Serum samples collected from immunized mice or rhesus monkeys were inactivated at 56 °C for 30 min, followed by serial dilution. Fifty microliters of each dilution was mixed with 100 CCID50 of virus and incubated for 1 h at 37 °C, followed by the addition of 100 µL of Vero cell suspension with 1 × 105 cells. A typical cytopathic effect developed at 5 d post-inoculation, and the virus titer was then evaluated via a microtitration assay using a standard protocol.Citation28
IFN-γ-specific ELISPOT assay
Blood samples from immunized rhesus monkeys were collected for each group at days 0 and 56 after the first immunization. The PBMC were isolated, pooled and counted. The cells were prepared in a density of 1 × 105 per well, and the ELISPOT assay was performed according to the standard protocol from the manufacturer (BD Biosciences).Citation29 The IFN-γ-secreting cells stimulated with purified inactivated virus antigen (20 Eu/well) were imaged and counted on the C.T.L. Immunospot Reader (Cellular Technology Limited).
Analysis of the pro-inflammatory cytokine levels in sera from rhesus monkeys
The sera from immunized monkeys were collected for analysis in detection assays targeting IL-2, IL-4, IL-5, IL-6, TNF-α, and IFN-γ. These assays were conducted simultaneously using a Th1/Th2 cytokine cytometric bead array kit (BD Biosciences). Briefly, a mixture of the anti-cytokine beads was added to the serum samples, and the samples were incubated with the included PE detection reagent in the dark at room temperature for 3 h before being washed twice. The intensity of the resulting fluorescence signal was measured using a fluorescence-activated cell sorter (FACS) flow cytometer (BD Biosciences) and analyzed using CBA software (BD Biosciences).
Statistical analyses
The data were expressed as means and standard deviations. Individual experiments were repeated 2 to 3 times per sample. GraphPad Prism software was used for the statistical analyses. The differences between the 2 groups were evaluated using one-way ANOVA. A P value of <0.05 was considered significant.
Abbreviations: | ||
CA16 | = | coxsackie virus A 16 type |
EV71 | = | enterovirus 71 |
HFMD | = | hand, foot, and mouth disease |
ELISPOT | = | enzyme-linked immunospot |
GMT | = | geometric mean tier |
EU | = | ELISA unit |
PBMC | = | peripheral blood mononuclear cell |
CFDA | = | Chinese Food and Drug Administration |
MOI | = | multiplicity of infection |
ELISA | = | enzyme-linked immunosorbent assay |
HRP | = | horseradish peroxidase |
IP | = | intraperitoneally |
CCID50 | = | cell culture infective dose 50% |
Additional material
Download Zip (625.2 KB)Disclosure of Potential Conflicts of Interest
No potential conflicts of interest were disclosed.
Acknowledgments
This work was supported by the National Basic Research Program (2011CB504903), National High-Tech R&D Program (2012AA02A404), Important National Science and Technology Specific Projects (2012ZX09101319002), National Natural Sciences Foundation of China (81171573, 31100127), and the Yunnan Natural Science Foundation (2011FB116).
References
- Huang XY, Kang K, Xu YL, Wei HY, Li XL, Ma H, You AG, Chen HM, Xu BL. [Etiology surveillance of hand-foot-mouth disease in Henan province between 2008 and 2011]. Zhonghua Yu Fang Yi Xue Za Zhi 2012; 46:883 - 7; PMID: 23363860
- Lü H-k. ZHANG Y-j, MIU Z-p, CAI J, QIN S-w, GONG L-m, CHEN Z-p. Epidemiological and pathogenic characteristics of hand,foot,and mouth disease in Zhejiang province, 2008-2009. Chin J Publ Health 2012; 28:105 - 7
- Rabenau HF, Richter M, Doerr HW. Hand, foot and mouth disease: seroprevalence of Coxsackie A16 and Enterovirus 71 in Germany. Med Microbiol Immunol 2010; 199:45 - 51; http://dx.doi.org/10.1007/s00430-009-0133-6; PMID: 19941005
- Ishimaru Y, Nakano S, Yamaoka K, Takami S. Outbreaks of hand, foot, and mouth disease by enterovirus 71. High incidence of complication disorders of central nervous system. Arch Dis Child 1980; 55:583 - 8; http://dx.doi.org/10.1136/adc.55.8.583; PMID: 6254449
- Jia L, Zhao CS, Zhang L, Li S, Zhang DT, Liu BW, Wang QY, Li XY. [Comparisons of epidemiological and clinical characteristics in children with hand-foot-mouth disease caused by Enterovirus 71 and Coxackievirus A16]. Zhongguo Dang Dai Er Ke Za Zhi 2011; 13:635 - 7; PMID: 21849112
- Xu W, Liu CF, Yan L, Li JJ, Wang LJ, Qi Y, Cheng RB, Xiong XY. Distribution of enteroviruses in hospitalized children with hand, foot and mouth disease and relationship between pathogens and nervous system complications. Virol J 2012; 9:8; http://dx.doi.org/10.1186/1743-422X-9-8; PMID: 22230340
- Wright HT Jr., Landing BH, Lennette EH, McALLISTER RM. Fatal infection in an infant associated with Coxsackie virus group A, type 16. N Engl J Med 1963; 268:1041 - 4; http://dx.doi.org/10.1056/NEJM196305092681904; PMID: 14001956
- Yi D, Sun L, Chen Q, Zhang N, Han J, Ye X, Su X. [Comparison of three detection methods for neutralizing antibodies induced by Coxsackievirus A16 vaccine]. Xi Bao Yu Fen Zi Mian Yi Xue Za Zhi 2013; 29:287 - 91; PMID: 23643087
- Yang E, Zhao H, Zhang Y, Liu J, Liao Y, Wang L, Cui P, Yang L, Liu L, Dong C, et al. A comparative study of the characteristics of two Coxsackie A virus type 16 strains (genotype B). Sci China Life Sci 2012; 55:336 - 42; http://dx.doi.org/10.1007/s11427-012-4313-z; PMID: 22566090
- Zhao H, Li HY, Han JF, Deng YQ, Li YX, Zhu SY, He YL, Qin ED, Chen R, Qin CF. Virus-like particles produced in Saccharomyces cerevisiae elicit protective immunity against Coxsackievirus A16 in mice. Appl Microbiol Biotechnol 2013; 97:10445 - 52; http://dx.doi.org/10.1007/s00253-013-5257-3; PMID: 24085395
- Liu Q, Yan K, Feng Y, Huang X, Ku Z, Cai Y, Liu F, Shi J, Huang Z. A virus-like particle vaccine for coxsackievirus A16 potently elicits neutralizing antibodies that protect mice against lethal challenge. Vaccine 2012; 30:6642 - 8; http://dx.doi.org/10.1016/j.vaccine.2012.08.071; PMID: 22959985
- Cai Y, Liu Q, Huang X, Li D, Ku Z, Zhang Y, Huang Z. Active immunization with a Coxsackievirus A16 experimental inactivated vaccine induces neutralizing antibodies and protects mice against lethal infection. Vaccine 2013; 31:2215 - 21; http://dx.doi.org/10.1016/j.vaccine.2013.03.007; PMID: 23499596
- Mao Q, Wang Y, Gao R, Shao J, Yao X, Lang S, Wang C, Mao P, Liang Z, Wang J. A neonatal mouse model of coxsackievirus A16 for vaccine evaluation. J Virol 2012; 86:11967 - 76; http://dx.doi.org/10.1128/JVI.00902-12; PMID: 22951825
- Zheng-ling L. LiHua L, Run-xiang Y. Research and preliminary application of Cox A16 antigen detection Kit. Chinese Journal of Health Laboratory Technology 2013; 4:801 - 5
- Raczniak GA, Bulkow LR, Bruce MG, Zanis CL, Baum RL, Snowball MM, Byrd KK, Sharapov UM, Hennessy TW, McMahon BJ. Long-term immunogenicity of hepatitis A virus vaccine in Alaska 17 years after initial childhood series. J Infect Dis 2013; 207:493 - 6; http://dx.doi.org/10.1093/infdis/jis710; PMID: 23204169
- Dong C, Wang J, Liu L, Zhao H, Shi H, Zhang Y, Jiang L, Li Q. Optimized development of a candidate strain of inactivated EV71 vaccine and analysis of its immunogenicity in rhesus monkeys. Hum Vaccin 2010; 6:1028 - 37; http://dx.doi.org/10.4161/hv.6.12.12982; PMID: 21150270
- Mao Q, Wang Y, Gao R, Shao J, Yao X, Lang S, Wang C, Mao P, Liang Z, Wang J. A neonatal mouse model of coxsackievirus A16 for vaccine evaluation. J Virol 2012; 86:11967 - 76; http://dx.doi.org/10.1128/JVI.00902-12; PMID: 22951825
- Yu CK, Chen CC, Chen CL, Wang JR, Liu CC, Yan JJ, Su IJ. Neutralizing antibody provided protection against enterovirus type 71 lethal challenge in neonatal mice. J Biomed Sci 2000; 7:523 - 8; http://dx.doi.org/10.1007/BF02253368; PMID: 11060501
- Liu L, Zhang Y, Wang J, Zhao H, Jiang L, Che Y, Shi H, Li R, Mo Z, Huang T, et al. Study of the integrated immune response induced by an inactivated EV71 vaccine. PLoS One 2013; 8:e54451; http://dx.doi.org/10.1371/journal.pone.0054451; PMID: 23372725
- Teng Z, Tan XJ, Shao JJ, Zhang Y, Kuang XZ, Zhang X, Xu WB. [Epidemiology and etiology of hand-foot-and-mouth disease in Shanghai, 2009]. Bing Du Xue Bao 2010; 26:437 - 42; PMID: 21344746
- Chatproedprai S, Theanboonlers A, Korkong S, Thongmee C, Wananukul S, Poovorawan Y. Clinical and molecular characterization of hand-foot-and-mouth disease in Thailand, 2008-2009. Jpn J Infect Dis 2010; 63:229 - 33; PMID: 20657060
- Mao Q, Wang Y, Yao X, Bian L, Wu X, Xu M, Liang Z. Coxsackievirus A16: Epidemiology, diagnosis, and vaccine. Hum Vaccin Immunother 2013; 10; Forthcoming PMID: 24231751
- Ni H, Yi B, Yin J, Fang T, He T, Du Y, Wang J, Zhang H, Xie L, Ding Y, et al. Epidemiological and etiological characteristics of hand, foot, and mouth disease in Ningbo, China, 2008-2011. J Clin Virol 2012; 54:342 - 8; http://dx.doi.org/10.1016/j.jcv.2012.04.021; PMID: 22652041
- Guo R, Cao YY, Dai ZZ, Qu SR, Zhuang JY. [Characteristics of a human diploid cell line, KMB-17]. Zhongguo Yi Xue Ke Xue Yuan Xue Bao 1981; 3:226 - 30; PMID: 6459859
- Ortega A, Amorós D, García de la Torre J. Global fit and structure optimization of flexible and rigid macromolecules and nanoparticles from analytical ultracentrifugation and other dilute solution properties. Methods 2011; 54:115 - 23; http://dx.doi.org/10.1016/j.ymeth.2010.12.004; PMID: 21163355
- Koticha DK, Barbagallo CA, Rapiejko PJ. Rapid, Ultrafiltration-based Method for Purification of Monoclonal Antibodies from Hybridoma Supernatants. 2008 [2013-10-10].
- Hochstrasser DF, Patchornik A, Merril CR. Development of polyacrylamide gels that improve the separation of proteins and their detection by silver staining. Anal Biochem 1988; 173:412 - 23; http://dx.doi.org/10.1016/0003-2697(88)90208-4; PMID: 3189819
- Bey E, Golombick T. A comparison of three methods for titration of poliovirus vaccines. J Virol Methods 1984; 9:123 - 30; http://dx.doi.org/10.1016/0166-0934(84)90004-1; PMID: 6096387
- Streeck H, Frahm N, Walker BD. The role of IFN-gamma Elispot assay in HIV vaccine research. Nat Protoc 2009; 4:461 - 9; http://dx.doi.org/10.1038/nprot.2009.7; PMID: 19282851