Abstract
Noroviruses (NoVs) are important pathogens causing epidemic acute gastroenteritis affecting millions of people worldwide. Due to the inability to cultivate NoVs, current NoV vaccine development relies on bioengineering technologies to produce virus-like particles (VLPs) and other subviral particles of NoVs as subunit vaccines. The first VLP vaccine has reached phase II clinical trials and several others are under development in pre-clinical research. Several subviral complexes made from the protruding (P) domains of NoV capsid share common features of easy production, high stability and high immunogenicity and thus are candidates for low cost vaccines. These P domain complexes can also be used as vaccine platforms to present foreign antigens for potential dual vaccines against NoVs and other pathogens. Development of NoV vaccines also faces other challenges, including genetic diversity of NoVs, limit understanding of NoV immunology and evolution, and lack of an efficient NoV animal model for vaccine assessment, which are discussed in this article.
Disease Burden of NoV Acute Gastroenteritis
NoVs are the most important viral pathogens causing epidemics of acute gastroenteritis in both developed and developing countries affecting people of all ages. They also lead to endemics in developing countries. The viruses are highly contagious, transmitted through oral/fecal route by person-to-person contact and often cause large outbreaks in closed and semi-closed communities and institutions through contaminated water and/or food. While the disease is often self-limited, increasing epidemiology data suggest that NoVs can cause severe diarrhea, particularly in young and the elderly. It is estimated that NoVs are responsible for up to 21 million illnesses, 1.9 million outpatient visits, 400 000 emergency department visits, 71 000 hospitalizations and 800 deaths in the United States,1 and 218 000 deaths worldwide each year.2 Thus, NoVs are a threat to public health. Since introduction of rotavirus vaccines several years ago, development of an effective NoV vaccine has been the top priority for prevention of viral gastroenteritis.
Genetic Classification and Host Receptors of NoVs
The Norovirus genus in the calicivirus family contains five genogroups (GI-GV), in which GI, GII and GIV infect humans. GI and GII, the major cause of epidemics of acute gastroenteritis in humans, are genetically diverse containing over 30 genotypes. Due to the lack of a cell culture, a classification based on viral neutralization of human NoVs remains unavailable. On the other hand, NoVs recognize the polymorphic histo-blood group antigens (HBGAs) as receptors or attachment factors in a strain-specific manner. The binding of NoVs to specific HBGAs has been found associated with their susceptibility to humans3-5 and serum antibodies against NoV-HBGA interaction are found correlated with the protection of the individuals against NoV challenge.6 These findings are important for understanding of the host immunity and provide novel tools for vaccine development against NoVs.
Advancement of NoV-Like Particle (VLP) Vaccine
The inability to cultivate NoVs in cell culture is a major challenge in the development of NoV vaccines. As a result, the traditional strategies of live attenuate and inactivated vaccine are not possible, while a subunit vaccine based on recombinant NoV antigens must be a choice. NoVs are a group of small, single-stranded, positive-sense RNA viruses constituting the Norovirus genus in the Calicivirus family. Structurally NoVs are covered by a protein capsid that is formed by a major (VP1) and a minor (VP2) structural protein. Expression of the major capsid protein VP1 assembles spontaneously into VLPs that are morphologically and antigenically similar to authentic capsids7-11 () and retain binding function to HBGA receptors.12-17 VLPs generally preserve the virus-specific molecular patterns and high density of B- and T-cell epitopes to induce potent innate, humoral, and cellular immune responses, respectively.18,19 Thus, NoV VLPs are an excellent choice for a NoV vaccine. NoV VLPs can be produced by a variety of expression systems, including insect cells via recombinant baculoviruses,8,10 mammalian cells via a Venezuelan equine encephalitis (VEE) replicon,20 a vesicular stomatitis virus (VSV) vector,21 or a plasmid,22 yeast (Pichia pastoris),23 and several transgenic plants, including tomato, potato, and tobacco.24-29 Among these approaches the baculovirus expression system is straightforward and highly efficient for large-scale productions of highly purified VLPs for NoV vaccine development.30-34
Figure 1. Cryo-electron microscopy of norovirus-like particle (VLP) and P particle. The VLP (left) is composed of 180 capsid proteins (VP1s) with the P2 subdomain on its outermost surface. The P particle that formed by 24 P domains also has the P2 subdomain on its outermost surface. The P2 subdomain of norovirus is responsible for virus-host interactions and immune responses of the virus and thus both norovirus VLPs and P particles share similar antigenic properties.
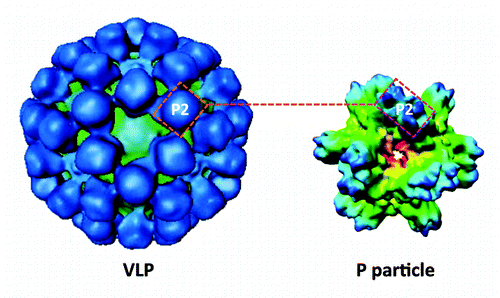
Preclinical Studies of NoV Vaccine
NoV VLP vaccines derived from various production approaches have been extensively evaluated in preclinical animal trials (). In early studies on transgenic plant (tomato, potato and tobacco) -expressed Norwalk virus (NV) VLPs, specific serum IgG and secretory IgA responses were detected after the mice were fed with the transgenic plants.24,27 Similar results were obtained in mice following oral administration of the baculovirus-expressed NV VLPs with or without cholera toxin as adjuvant.35 Superior to oral administration, intranasal immunization of VLPs without an adjuvant resulted in significantly higher immune responses.36 An addition of adjuvants, such as the mutant Escherichia coli heat-labile toxin LT(R192G)36 and a dry powder formulation (GelVac) of an inert in situ gelling polysaccharide (GelSite) extracted from Aloe vera,37 further enhanced the immune responses.
Table 1. Summary some preclinical trials for development subunit vaccine against norovirus
Immune responses of animals to NoV VLPs through inoculation of viral vectors expressing NoV VLPs were also studied. For example, inoculation of a single dose of the rVSV-VP1 to mice stimulated significantly stronger humoral and cellular immune responses than those induced by the VLP vaccination.21 Similarly, inoculation of NV VLP-expressing alphavirus vectors resulted in specific systemic and mucosal immune responses in mice20 (). These alphavirus vectors were also used to study the heterotypic immune responses of VLP vaccines. Analysis of patient sera after infection by different GI and GII NoVs through NoV-HBGA blocking assays, heterotypic immune responses were observed.38 Such heterotypic responses were also observed in mice following immunization with vaccine cocktails containing multiple NoV VLPs.39
Due to the genetic diversity of human NoVs, strategies of NoV VLP vaccines for broad protection have also been explored. A GII.4 “Consensus” VLP that was engineered based on three GII.4 variants induced serum antibody in rabbits being reactive to VLPs derived from several GII.4 variants circulating in over 30 y.40 In addition, a bivalent vaccine formulation containing the GI.1 NV and the GII.4 consensus VLPs induced an increased breadth of immune response to diverse variants within the two NoV genogroups in comparison with a monovalent vaccine. Among several vaccinations via different administration routes with various adjuvants, the highest homologous and heterologous antibody titers to the bivalent vaccine were elicited by the intramuscular injection using Alhydrogel [Al(OH)3] as adjuvant.40
Clinical Trials of VLP Vaccines
Following demonstrations of safety and immunogenicity in animals, the baculovirus-expressed NV VLPs were further studied in phase I and phase II trials for safety, immunogenicity and efficacy in healthy volunteers. A summary of these studies has been published recently.41 In a trial with 20 adults that were orally administered with NV VLPs (250 µg) without an adjuvant, all vaccinees responded with 4-folds or higher increases in serum IgG titers, among which 83% of the subjects responded after the first vaccine dose, while no further IgG titer increase was observed after the second dose.42 In a repeated trial with higher doses (–up to 2000 µg), all vaccinees developed significant rises in IgA anti-VLP antibody-secreting cells (ASCs), while 90% of the subjects who received lower dose (250 µg) vaccine developed rises in serum anti-VLP IgG; neither the rates of seroconversion nor antibody titers increased significantly at the higher doses. These results may suggest an immune tolerance at high doses. About 30–40% of volunteers developed mucosal anti-VLP IgA, while lymphoproliferative responses and IFN-γ production were observed transiently among those who received 250 µg or 500 µg but not 2000 µg of VLP.43 Similar responses were also observed in human volunteers who received transgenic potato expressing NV VLPs, with significant increases in the numbers of specific IgA antibody-secreting cells, serum IgG, and/or specific stool IgA.25
The immune responses of NV VLP vaccine with adjuvant of the monophosphoryl lipid A (MPL) and the mucoadherent chitosan were further studied in volunteers following an intranasal delivery. While no vaccine-related, seriously adverse effects observed, specific IgG and IgA antibodies increased significantly in all subjects who received the 50- or 100-μg vaccine dose. Vaccinees also developed IgA ASCs that expressed molecules associated with homing to mucosal and peripheral lymphoid tissues.44 Further repeat of the study revealed B memory [B(M)] responses in volunteers. All subjects immunized with 100 μg of the NV VLP vaccine and 90% of those who received 50 μg had significant IgA or IgG B(M) responses. The B(M) cell frequencies correlated with serum antibody levels and mucosally-primed ASC responses.45
Following these studies, two candidate VLP vaccines that are derived from VLPs of the prototype NV (GI.1)8 and a consensus GII.4 VLP based on a 2002 and two 2006 GII.4 isolates,40 respectively, have been moved to clinical trials for safety and efficacy through human volunteer challenge studies. In a study of the GI.1 NV VLP vaccine, volunteers were vaccinated intranasally and then challenged orally with the homologous NVs.46 NV-specific IgA seroresponse with 4-folds or higher increase of serum antibody titer was detected in 70% of the 47 vaccine recipients. Vaccination significantly reduced the frequencies of gastroenteritis and virus infection. These data indicated NV VLP vaccine effectively protected vaccinees from infection and illness of NV.46
In a mostly recent study on a bivalent VLP vaccine (GI.1 NV/GII.4 consensus) performed by Takeda Pharmaceutical Company Limited (http://www.takeda.com), volunteers were vaccinated intramuscularly with two doses followed by an oral challenge with a GII.4 2003 NoV (Cin-1) (http://www.takeda.com/news/2013/20131007_6021.html). The results showed a 52% reduction against vomiting and/or diarrhea of any severity. In those who experienced symptoms, severity of illness as measured by the Vesikari scoring system47,48 was significantly reduced in vaccinees vs. placebo recipients. In summary, the non-replicating NoV VLP vaccines are safe and effective for protection against infection and illness of NoVs.
NoV P Domain Complexes as Vaccine Candidates
The NoV capsid protein VP1 comprises two major domains, the shell (S) and the protruding (P) domains, linked by a short flexible hinge.9 Expression of the P domain in E. coli results in variable complexes, including the P domain dimers,49-52 the P particle that contains 12 P dimers53,54 (), the smaller P particle that contains 6 P dimers55 and polyvalent P domain complexes that contain many P dimers.56 All these P complexes retain HBGA binding property. Among the complexes the P particle is highly stable, easily to produce and highly immunogenic and therefore has been selected as vaccine candidate for further development. The polyvalent P domain complexes that are made by fusion of two to three NoV P domains representing different GI and GII NoVs56 provide an opportunity to develop a dual or multivalent vaccine for broad protection against NoVs.
Both the P particle and the polyvalent P domain complexes induced significantly higher antibody and CD4+ T cells responses in mice than those induced by the free P dimers.56,57 The P particles also induced high titers of NoV-specific immunoglobulin in chicken egg yolks (IgY), providing a strategy for therapeutic treatment against NoV diseases.58,59 Thus, these P domain complexes are excellent vaccine candidates against NoVs.
NoV P Complexes as a Vaccine Platform for Dual Vaccine Development
The NoV P complexes have also been proposed as vaccine platforms for presentation of foreign antigens for immune enhancement. There are six surface loops on the distal end of each P dimer (three for each P domain), corresponding to the outermost surface of the P particle60 and VLP.9 Thus, insertion of a foreign antigen into one of the loops results in 24 copies of the antigen on the surface of the P particle, or many copies on the polyvalent P complex, and therefore significantly enhances the immunogenicity of the inserted antigen. A number of small peptides and protein antigens have been inserted into the loops and are well presented by the P domain complexes.56,61-63 Importantly, the immunogenicity of the P domain complexes retained, making these chimeric P domain complexes dual vaccines against NoVs and other pathogens.
Three promising dual vaccine candidates have been made and evaluated through preclinical animal trials. The first P particle chimeric vaccine (P particle-VP8*) contains a surface insertion of the neutralization antigen VP8* of rotavirus (RV).61,64 This vaccine induced strong immune responses to both the RV VP8* and NoV P antigens and protected mice against RV infection.56,61 Another dual vaccine (P particle-M2e) contains an insertion of the conserved M2e epitope of influenza A viruses. It elicited high titers of M2e- and P domain-specific antibody and fully protected mice from lethal challenges with influenza viruses.62 Finally, the complex vaccine (NoV P-HEV P) containing the P antigens of NoV and hepatitis E virus (HEV) induced strong antibody responses against both NoV and HEV with high neutralizing or HBGA blocking activity against the two viruses.63 These three NoV P antigen-based complexes are promising dual vaccine candidates against NoV and RV, NoV and influenza virus, and NoV and HEV.
Efforts in Development of Animal Models for NoVs
Large efforts have been made in developing an animal model for NoVs in different species, including non-human primates, pigs, calves, and mice. However, due to apparent host species barriers, an efficient small animal model that fully supports the replication and illness of human NoVs remains lacking. Among those animal models that demonstrated limit NoV replication and/or illness, the gnotobiotic (Gn) pig model could be a choice for vaccine assessment, although further improvement of the model is needed.
Non-human primate models
Early studies in the 1970s revealed that NV-challenged chimpanzees exhibited subclinical infections, including seroresponses and virus shedding, but not vomiting or diarrhea.65 Similar scenario was also observed in rhesus macaques after NoV inoculation.66 Subsequent studies in seeking an efficient non-human primate model for NoVs observed both clinical illness and viral shedding in newborn pigtail macaques (Macaca nemestrina).67 However, none of these models was used afterward, excepted for the chimpanzee model that was used recently to study the duration of immunity after NV infection and to assess the NV and GII.4 VLP vaccine against NV infection.68 Unfortunately, further use of great apes for invasive experimentation has been banned in the United States (http://blogs.nature.com/news/2012/07/bill-ending-us-chimp-research-advances.html).
The gnotobiotic (Gn) pig model
Gn pigs are another promising animal model for human NoVs partially due to their similarities with human in gut structure and physiology, including the common A and H HBGAs.69,70 Challenge of pigs with human NoVs revealed mild to modest diarrhea with modest virus shedding in stools, rectal swab fluids and intestinal contents.69,71 Like in human, NoVs mainly replicate in pigs in some epithelial cells in the duodenum and jejunum. In addition, infected Gn pigs resulted in systemic and intestinal humoral and cellular immune responses.72 Neonatal pigs (4–5 d of age) appeared more susceptible to human NoVs than older pigs (33–34 d of age).69 Interestingly, administration of a cholesterol-lowering drug, simvastatin, increased the susceptibility of the older pigs to a comparable level of the neonatal pigs.69,73 The Gn pig model has helped our understanding in NoV pathogenesis69-71 and is current used for evaluation of vaccines and antivirals against human NoVs73 (Yuan and Jiang, unpublished data).
The Gn calf model
Gn claves were also studied as a potential animal model of human NoVs. Like pigs, claves share similar A antigens with humans.74 Inoculation of human NoV (GII.4-HS66) to Gn claves caused diarrhea and virus shedding in feces.75 Intestinal lesions were observed in duodenum and jejunum with detection of viral capsid antigen in the jejunum. Seroconversion with the high numbers of ASCs in the intestine was also observed. These data indicated NoV replication and entero-pathogenicity in Gn calves. However, the higher cost of the Gn calf model compared with the Gn pig model may limit its application as an evaluation tool of NoV vaccine.
A mouse model
This small animal model was proposed recently by showing limited replication of human NoVs in immune-deficient mice.76 After challenged through an intraperitoneal route, both humanized and nonhumanized BALB/c Rag-γc-deficient mice seemingly supported replication of a GII.4 NoV, as shown by increased viral loads over input and expression of the structural and nonstructural NoV proteins in macrophage-like cells in the spleens and livers. While this model may not be useful for vaccine evaluation due to the requirement of immune-deficient status of the mice for NoV replication, it may be useful for studying the pathogenesis and assessment of antivirals against NoVs.
Development of Human Challenge Models for NoVs
The early human challenge studies in the 1970s with the prototype NV (GI.1) and Hawaii virus (GII.1) provided large amount of information on the onset of disease, clinical manifestations, duration of illness of NoV gastroenteritis, and host immunity after NoV infection.77-82 The observation that NV infected subjects were protected against subsequent challenge of the same virus for 6 to 24 months, indicating the feasibility of a NoV vaccine,80 although such protection may be strain-specific.82 Thus, the highly diverse NoVs, comprising of 9 GI and 22 GII genotypes83,84 with unknown antigenic relationships, represent a further challenge in NoV vaccine development.
Another important factors related to human challenge model is HBGA phenotypes of human subjects that may control the host susceptibility to NoV infections, which has been shown for two NoVs, NV (GI.1) and Cin-1 (GII.4),4,85 but not for the Snow Mountain virus (SMV, GII.2).86 In vitro experiments showed that human NoVs are diverse in interaction with the polymorphic HBGAs. In addition, volunteers who were experimentally infected with NV revealed a strong correlation of HBGA blocking antibodies with protection against clinical gastroenteritis and therefore, the HBGA blocking assay is considered as a surrogate of neutralization for NoVs.6,87 Thus, the host geno- and phenotypes of HBGAs should be considered in selecting subjects for a challenge study as this may significantly impact the outcomes of clinical infection.
Currently human challenge models of GI.1 NV, GII.4 Cin-1, and GII.2 SMV, have been established, among which the ones for the NV and Cin-1 have been used for the safety and efficacy studies of two VLP vaccines representing GI.1 and GII.4, respectively.46,85 Further improvement of the available human challenge models and establishment of models for more NoV types are necessary. First, challenge models for additional NoV types would help the determination of cross-protection among different antigenic types of NoVs, which is an important issue in NoV vaccine development. Second, the role of HBGAs in the host susceptibility of the SMV and other genotypes needs to be determined. Third, since the subjects in a challenge model are normally adults who usually have pre-existing NoV specific antibodies, the roles of such antibodies in protection against NoV infection and impact on subsequent vaccination of non-replicating NoV vaccine need to be clarified. Furthermore, the 50% human infection doses (ID50s) for NV have been determined recently for subjects with specific HBGA types.88 Such infection doses should also be determined for the remaining challenge viruses for an optimal evaluation of vaccines for maximal protection. Finally, the duration of immune response and protective immunity following a vaccination needs to be studied, particularly for the elderly whose immunity may be weaned by aging.
Vaccine for Broad Protection against Diverse NoV Types
NoVs are highly diverse containing over 30 genotypes in the two major genogroups of human NoVs.84 The antigenic diversity behind this genetic diversity is a further challenge in the development of NoV vaccine for broad protection. In addition, the antigenic types of different variants in the single predominant GII.4 genotype change continually, probably were driven by the host herd immunity in a way similar to the epochal evolution of the influenza viruses.89,90 Genetic and in vitro blocking studies have identified several antigenic epitopes on the GII.4 NoVs that are correlated with the chronological changes of GII.4 variants in the past decades.91-95 Thus, continual monitoring the NoV epidemiology to predict epidemic GII.4 variants for future vaccines is necessary. Noteworthy, genetic analysis of NoVs showed that the HBGA binding interfaces of NoVs are conserved among genotypes within each of the two major genogroups (GI and GII) of human NoVs, indicating a strong positive selection by the human HBGAs. In fact, the amino acid sequences of the HBGA binding sites are almost 100% conserved among all GII.4 variants circulated in the past three decades.96 Thus, in addition to the herd immunity, the human HBGAs should also play a role in the epochal evolution of GII.4 NoVs.
Characterization of GII.4 variants by monoclonal antibodies (mAbs) supported the antigenic changes predicted by genetic analysis. MAbs recognizing either type-common or type-specific epitopes among GII.4 variants have been identified.92,93,95,97 Cross-reactive antigenic types among different GII.4 variants also have been shown by polyclonal serum antibodies of patients infected by a GII.4 NoV.96 Four-fold or higher increases of antibody titers between the acute and convalescent serum samples against different GII.4 variants were measured by both antibody detection ELISA and HBGA blocking assays.96 These results suggest that the shared antigenic epitopes among GII.4 variants could be targets for broadly protective vaccines against different GII.4 variants. Thus, after determination of the levels of shared antigenic types among different genotypes within each of the two major genogroups of human NoVs, a cocktail vaccine containing the major antigenic types of NoVs may be developed for broad protection. The aforementioned bivalent GI.1/GII.4 VLP vaccine is an example of such cocktail vaccine. Similarly, a cocktail vaccine against NoV and RV based on mixed NoV VLPs (GII.4 or GII.4 and GI.3) with the tubular RV recombinant VP6 protein98,99 has been developed, which induced cross-reactive antibodies to heterologous NoV VLPs (GII.12 and GI.1 VLPs) and to different RVs. In addition, two bivalent vaccines comprising polyvalent P domains of GII.4 (VA387)/GII.9 (VA207) and GII.4 (VA387)/GI.3 (VA115) NoVs, respectively, provided a good example for simple, efficient cocktail vaccines.56 Thus, after elucidation of the antigenic relatedness among different NoVs in both GI and GII, a broadly protective NoV vaccine is possible.
Conclusion and Future Perspective
By taking advantages of recombinant technology, variable subviral particles, including VLPs and P domain complexes of NoVs, have been developed and studied as subunit vaccines against NoVs. The first VLP vaccines derived from the prototype NV and the predominant GII.4 NoVs have reached phases II clinical trials with a promising outcomes, while a number of other VLP and P domain complex-based vaccines are following in preclinical researches. The P particle-based vaccine candidates are easily produced in E. coli and stable and therefore a promising low cost vaccine. The P domain complexes also serve as vaccine platforms to present foreign antigens for potential dual or even multivalent vaccines against NoVs and other pathogens.
Due to apparent a host species barrier, a highly efficient, low cost small animal model for human NoVs remains lacking. The Gn pig model supports limited viral replication and clinical illness after NoV-challenge, therefore may be a choice for further improvement as a tool for evaluation of NoV vaccines. Human volunteer challenge model for three NoVs are readily available for phases I and II clinical trials of NoV vaccines. On the other hand, further improvement of the models for better understanding of host-pathogen interaction and immunology and for better vaccine evaluation is needed. Due to the genetic and antigenic diversity of NoVs and the antigenic drift of some genotypes, selection of a cocktail vaccine containing the major antigenic types is necessary for broad protection. In this regard new human challenge models for additional genotypes will be needed.
Potential Conflict of Interest
M.T. and X.J. receive royalty from LigoCyte for two licenses on the P particle vaccine and the P particle-VP8 dual vaccine technologies. The authors have no other relevant affiliations or financial involvement with any organization or entity with a financial interest in or financial conflict with the subject matter or materials discussed in the manuscript apart from those disclosed.
Acknowledgments
The laboratories of the authors are supported by the National Institute of Health (R01AI089634, P01 HD13021 to X.J. and R21AI092434 to M.T), Department of Agricultures (NIFA, AFRI to X. J.) of the United States of America, and an institutional Clinical and Translational Science Award (NIH/NCRR 8UL1TR000077–04 to M.T.)
References
- Hall AJ, Lopman BA, Payne DC, Patel MM, Gastañaduy PA, Vinjé J, Parashar UD. Norovirus disease in the United States. Emerg Infect Dis 2013; 19:1198 - 205; http://dx.doi.org/10.3201/eid1908.130465; PMID: 23876403
- Patel MM, Widdowson MA, Glass RI, Akazawa K, Vinjé J, Parashar UD. Systematic literature review of role of noroviruses in sporadic gastroenteritis. Emerg Infect Dis 2008; 14:1224 - 31; http://dx.doi.org/10.3201/eid1408.071114; PMID: 18680645
- Hutson AM, Atmar RL, Graham DY, Estes MK. Norwalk virus infection and disease is associated with ABO histo-blood group type. J Infect Dis 2002; 185:1335 - 7; http://dx.doi.org/10.1086/339883; PMID: 12001052
- Lindesmith L, Moe C, Marionneau S, Ruvoen N, Jiang X, Lindblad L, Stewart P, LePendu J, Baric R. Human susceptibility and resistance to Norwalk virus infection. Nat Med 2003; 9:548 - 53; http://dx.doi.org/10.1038/nm860; PMID: 12692541
- Frenck R, Bernstein DI, Xia M, Huang P, Zhong W, Parker S, Dickey M, McNeal M, Jiang X. Predicting susceptibility to norovirus GII.4 by use of a challenge model involving humans. J Infect Dis 2012; 206:1386 - 93; http://dx.doi.org/10.1093/infdis/jis514; PMID: 22927452
- Reeck A, Kavanagh O, Estes MK, Opekun AR, Gilger MA, Graham DY, Atmar RL. Serological correlate of protection against norovirus-induced gastroenteritis. J Infect Dis 2010; 202:1212 - 8; http://dx.doi.org/10.1086/656364; PMID: 20815703
- Jiang X, Matson DO, Ruiz-Palacios GM, Hu J, Treanor J, Pickering LK. Expression, self-assembly, and antigenicity of a snow mountain agent-like calicivirus capsid protein. J Clin Microbiol 1995; 33:1452 - 5; PMID: 7650166
- Jiang X, Wang M, Graham DY, Estes MK. Expression, self-assembly, and antigenicity of the Norwalk virus capsid protein. J Virol 1992; 66:6527 - 32; PMID: 1328679
- Prasad BV, Hardy ME, Dokland T, Bella J, Rossmann MG, Estes MK. X-ray crystallographic structure of the Norwalk virus capsid. Science 1999; 286:287 - 90; http://dx.doi.org/10.1126/science.286.5438.287; PMID: 10514371
- Jiang X, Zhong WM, Farkas T, Huang PW, Wilton N, Barrett E, Fulton D, Morrow R, Matson DO. Baculovirus expression and antigenic characterization of the capsid proteins of three Norwalk-like viruses. Arch Virol 2002; 147:119 - 30; http://dx.doi.org/10.1007/s705-002-8306-5; PMID: 11855626
- Herbst-Kralovetz M, Mason HS, Chen Q. Norwalk virus-like particles as vaccines. Expert Rev Vaccines 2010; 9:299 - 307; http://dx.doi.org/10.1586/erv.09.163; PMID: 20218858
- Huang P, Farkas T, Marionneau S, Zhong W, Ruvoën-Clouet N, Morrow AL, Altaye M, Pickering LK, Newburg DS, LePendu J, et al. Noroviruses bind to human ABO, Lewis, and secretor histo-blood group antigens: identification of 4 distinct strain-specific patterns. J Infect Dis 2003; 188:19 - 31; http://dx.doi.org/10.1086/375742; PMID: 12825167
- Huang P, Farkas T, Zhong W, Tan M, Thornton S, Morrow AL, Jiang X. Norovirus and histo-blood group antigens: demonstration of a wide spectrum of strain specificities and classification of two major binding groups among multiple binding patterns. J Virol 2005; 79:6714 - 22; http://dx.doi.org/10.1128/JVI.79.11.6714-6722.2005; PMID: 15890909
- Tan M, Jiang X. Norovirus and its histo-blood group antigen receptors: an answer to a historical puzzle. Trends Microbiol 2005; 13:285 - 93; http://dx.doi.org/10.1016/j.tim.2005.04.004; PMID: 15936661
- Tan M, Jiang X. Norovirus-host interaction: implications for disease control and prevention. Expert Rev Mol Med 2007; 9:1 - 22; http://dx.doi.org/10.1017/S1462399407000348; PMID: 17623487
- Tan M, Jiang X. Norovirus gastroenteritis, carbohydrate receptors, and animal models. PLoS Pathog 2010; 6:e1000983; http://dx.doi.org/10.1371/journal.ppat.1000983; PMID: 20865168
- Tan M, Jiang X. 2010. Virus-Host Interaction and Celluar Receptors of Caliciviruses, p. 111-130. In Hansman G, Jiang X, Green K (ed.), Caliciruses. Caister Academic Press, Norwich.
- Plummer EM, Manchester M. Viral nanoparticles and virus-like particles: platforms for contemporary vaccine design. Wiley Interdiscip Rev Nanomed Nanobiotechnol 2010; In press PMID: 20872839
- Zhao Q, Li S, Yu H, Xia N, Modis Y. Virus-like particle-based human vaccines: quality assessment based on structural and functional properties. Trends Biotechnol 2013; 31:654 - 63; http://dx.doi.org/10.1016/j.tibtech.2013.09.002; PMID: 24125746
- Harrington PR, Yount B, Johnston RE, Davis N, Moe C, Baric RS. Systemic, mucosal, and heterotypic immune induction in mice inoculated with Venezuelan equine encephalitis replicons expressing Norwalk virus-like particles. J Virol 2002; 76:730 - 42; http://dx.doi.org/10.1128/JVI.76.2.730-742.2002; PMID: 11752163
- Ma Y, Li J. Vesicular stomatitis virus as a vector to deliver virus-like particles of human norovirus: a new vaccine candidate against an important noncultivable virus. J Virol 2011; 85:2942 - 52; http://dx.doi.org/10.1128/JVI.02332-10; PMID: 21228240
- Taube S, Kurth A, Schreier E. Generation of recombinant norovirus-like particles (VLP) in the human endothelial kidney cell line 293T. Arch Virol 2005; 150:1425 - 31; http://dx.doi.org/10.1007/s00705-005-0517-x; PMID: 15789263
- Xia M, Farkas T, Jiang X. Norovirus capsid protein expressed in yeast forms virus-like particles and stimulates systemic and mucosal immunity in mice following an oral administration of raw yeast extracts. J Med Virol 2007; 79:74 - 83; http://dx.doi.org/10.1002/jmv.20762; PMID: 17133551
- Mason HS, Ball JM, Shi JJ, Jiang X, Estes MK, Arntzen CJ. Expression of Norwalk virus capsid protein in transgenic tobacco and potato and its oral immunogenicity in mice. Proc Natl Acad Sci U S A 1996; 93:5335 - 40; http://dx.doi.org/10.1073/pnas.93.11.5335; PMID: 8643575
- Tacket CO, Mason HS, Losonsky G, Estes MK, Levine MM, Arntzen CJ. Human immune responses to a novel norwalk virus vaccine delivered in transgenic potatoes. J Infect Dis 2000; 182:302 - 5; http://dx.doi.org/10.1086/315653; PMID: 10882612
- Souza AC, Vasques RM, Inoue-Nagata AK, Lacorte C, Maldaner FR, Noronha EF, Nagata T. Expression and assembly of Norwalk virus-like particles in plants using a viral RNA silencing suppressor gene. Appl Microbiol Biotechnol 2013; 97:9021 - 7; http://dx.doi.org/10.1007/s00253-013-5077-5; PMID: 23925532
- Zhang X, Buehner NA, Hutson AM, Estes MK, Mason HS. Tomato is a highly effective vehicle for expression and oral immunization with Norwalk virus capsid protein. Plant Biotechnol J 2006; 4:419 - 32; http://dx.doi.org/10.1111/j.1467-7652.2006.00191.x; PMID: 17177807
- Santi L, Huang Z, Mason H. 2006. Virus-like particles production in green plants. Methods (San Diego, Calif 40:66-76.
- Santi L, Batchelor L, Huang Z, Hjelm B, Kilbourne J, Arntzen CJ, Chen Q, Mason HS. An efficient plant viral expression system generating orally immunogenic Norwalk virus-like particles. Vaccine 2008; 26:1846 - 54; http://dx.doi.org/10.1016/j.vaccine.2008.01.053; PMID: 18325641
- Kissmann J, Ausar SF, Foubert TR, Brock J, Switzer MH, Detzi EJ, Vedvick TS, Middaugh CR. Physical stabilization of Norwalk virus-like particles. J Pharm Sci 2008; 97:4208 - 18; http://dx.doi.org/10.1002/jps.21315; PMID: 18300304
- Koho T, Mäntylä T, Laurinmäki P, Huhti L, Butcher SJ, Vesikari T, Kulomaa MS, Hytönen VP. Purification of norovirus-like particles (VLPs) by ion exchange chromatography. J Virol Methods 2012; 181:6 - 11; http://dx.doi.org/10.1016/j.jviromet.2012.01.003; PMID: 22265819
- Koho T, Huhti L, Blazevic V, Nurminen K, Butcher SJ, Laurinmäki P, Kalkkinen N, Rönnholm G, Vesikari T, Hytönen VP, et al. Production and characterization of virus-like particles and the P domain protein of GII.4 norovirus. J Virol Methods 2012; 179:1 - 7; http://dx.doi.org/10.1016/j.jviromet.2011.05.009; PMID: 21600929
- Herbst-Kralovetz M, Mason HS, Chen Q. Norwalk virus-like particles as vaccines. Expert Rev Vaccines 2010; 9:299 - 307; http://dx.doi.org/10.1586/erv.09.163; PMID: 20218858
- Willyard C. First vaccines targeting ‘cruise ship virus’ sail into clinical trials. Nat Med 2013; 19:1076 - 7; http://dx.doi.org/10.1038/nm0913-1076; PMID: 24013733
- Ball JM, Hardy ME, Atmar RL, Conner ME, Estes MK. Oral immunization with recombinant Norwalk virus-like particles induces a systemic and mucosal immune response in mice. J Virol 1998; 72:1345 - 53; PMID: 9445035
- Guerrero RA, Ball JM, Krater SS, Pacheco SE, Clements JD, Estes MK. Recombinant Norwalk virus-like particles administered intranasally to mice induce systemic and mucosal (fecal and vaginal) immune responses. J Virol 2001; 75:9713 - 22; http://dx.doi.org/10.1128/JVI.75.20.9713-9722.2001; PMID: 11559804
- Velasquez LS, Hjelm BE, Arntzen CJ, Herbst-Kralovetz MM. An intranasally delivered Toll-like receptor 7 agonist elicits robust systemic and mucosal responses to Norwalk virus-like particles. Clin Vaccine Immunol 2010; 17:1850 - 8; http://dx.doi.org/10.1128/CVI.00230-10; PMID: 20962211
- LoBue AD, Lindesmith L, Yount B, Harrington PR, Thompson JM, Johnston RE, Moe CL, Baric RS. Multivalent norovirus vaccines induce strong mucosal and systemic blocking antibodies against multiple strains. Vaccine 2006; 24:5220 - 34; http://dx.doi.org/10.1016/j.vaccine.2006.03.080; PMID: 16650512
- LoBue AD, Thompson JM, Lindesmith L, Johnston RE, Baric RS. Alphavirus-adjuvanted norovirus-like particle vaccines: heterologous, humoral, and mucosal immune responses protect against murine norovirus challenge. J Virol 2009; 83:3212 - 27; http://dx.doi.org/10.1128/JVI.01650-08; PMID: 19176631
- Parra GI, Bok K, Taylor R, Haynes JR, Sosnovtsev SV, Richardson C, Green KY. Immunogenicity and specificity of norovirus Consensus GII.4 virus-like particles in monovalent and bivalent vaccine formulations. Vaccine 2012; 30:3580 - 6; http://dx.doi.org/10.1016/j.vaccine.2012.03.050; PMID: 22469864
- Richardson C, Bargatze RF, Goodwin R, Mendelman PM. Norovirus virus-like particle vaccines for the prevention of acute gastroenteritis. Expert Rev Vaccines 2013; 12:155 - 67; http://dx.doi.org/10.1586/erv.12.145; PMID: 23414407
- Ball JM, Graham DY, Opekun AR, Gilger MA, Guerrero RA, Estes MK. Recombinant Norwalk virus-like particles given orally to volunteers: phase I study. Gastroenterology 1999; 117:40 - 8; http://dx.doi.org/10.1016/S0016-5085(99)70548-2; PMID: 10381908
- Tacket CO, Sztein MB, Losonsky GA, Wasserman SS, Estes MK. Humoral, mucosal, and cellular immune responses to oral Norwalk virus-like particles in volunteers. Clin Immunol 2003; 108:241 - 7; http://dx.doi.org/10.1016/S1521-6616(03)00120-7; PMID: 14499247
- El-Kamary SS, Pasetti MF, Mendelman PM, Frey SE, Bernstein DI, Treanor JJ, Ferreira J, Chen WH, Sublett R, Richardson C, et al. Adjuvanted intranasal Norwalk virus-like particle vaccine elicits antibodies and antibody-secreting cells that express homing receptors for mucosal and peripheral lymphoid tissues. J Infect Dis 2010; 202:1649 - 58; http://dx.doi.org/10.1086/657087; PMID: 20979455
- Ramirez K, Wahid R, Richardson C, Bargatze RF, El-Kamary SS, Sztein MB, Pasetti MF. Intranasal vaccination with an adjuvanted Norwalk virus-like particle vaccine elicits antigen-specific B memory responses in human adult volunteers. Clin Immunol 2012; 144:98 - 108; http://dx.doi.org/10.1016/j.clim.2012.05.006; PMID: 22710446
- Atmar RL, Bernstein DI, Harro CD, Al-Ibrahim MS, Chen WH, Ferreira J, Estes MK, Graham DY, Opekun AR, Richardson C, et al. Norovirus vaccine against experimental human Norwalk Virus illness. N Engl J Med 2011; 365:2178 - 87; http://dx.doi.org/10.1056/NEJMoa1101245; PMID: 22150036
- Ruuska T, Vesikari T. Rotavirus disease in Finnish children: use of numerical scores for clinical severity of diarrhoeal episodes. Scand J Infect Dis 1990; 22:259 - 67; http://dx.doi.org/10.3109/00365549009027046; PMID: 2371542
- Ruuska T, Vesikari T. A prospective study of acute diarrhoea in Finnish children from birth to 2 1/2 years of age. Acta Paediatr Scand 1991; 80:500 - 7; http://dx.doi.org/10.1111/j.1651-2227.1991.tb11893.x; PMID: 1872172
- Tan M, Hegde RS, Jiang X. The P domain of norovirus capsid protein forms dimer and binds to histo-blood group antigen receptors. J Virol 2004; 78:6233 - 42; http://dx.doi.org/10.1128/JVI.78.12.6233-6242.2004; PMID: 15163716
- Bu W, Mamedova A, Tan M, Xia M, Jiang X, Hegde RS. Structural basis for the receptor binding specificity of Norwalk virus. J Virol 2008; 82:5340 - 7; http://dx.doi.org/10.1128/JVI.00135-08; PMID: 18385236
- Cao S, Lou Z, Tan M, Chen Y, Liu Y, Zhang Z, Zhang XC, Jiang X, Li X, Rao Z. Structural basis for the recognition of blood group trisaccharides by norovirus. J Virol 2007; 81:5949 - 57; http://dx.doi.org/10.1128/JVI.00219-07; PMID: 17392366
- Chen Y, Tan M, Xia M, Hao N, Zhang XC, Huang P, Jiang X, Li X, Rao Z. Crystallography of a Lewis-binding norovirus, elucidation of strain-specificity to the polymorphic human histo-blood group antigens. PLoS Pathog 2011; 7:e1002152; http://dx.doi.org/10.1371/journal.ppat.1002152; PMID: 21811409
- Tan M, Fang P, Chachiyo T, Xia M, Huang P, Fang Z, Jiang W, Jiang X. Noroviral P particle: structure, function and applications in virus-host interaction. Virology 2008; 382:115 - 23; http://dx.doi.org/10.1016/j.virol.2008.08.047; PMID: 18926552
- Tan M, Jiang X. The p domain of norovirus capsid protein forms a subviral particle that binds to histo-blood group antigen receptors. J Virol 2005; 79:14017 - 30; http://dx.doi.org/10.1128/JVI.79.22.14017-14030.2005; PMID: 16254337
- Tan M, Fang PA, Xia M, Chachiyo T, Jiang W, Jiang X. Terminal modifications of norovirus P domain resulted in a new type of subviral particles, the small P particles. Virology 2011; 410:345 - 52; http://dx.doi.org/10.1016/j.virol.2010.11.017; PMID: 21185050
- Wang L, Huang P, Fang H, Xia M, Zhong W, McNeal MM, Jiang X, Tan M. Polyvalent complexes for vaccine development. Biomaterials 2013; 34:4480 - 92; http://dx.doi.org/10.1016/j.biomaterials.2013.02.041; PMID: 23498893
- Fang H, Tan M, Xia M, Wang L, Jiang X. Norovirus P particle efficiently elicits innate, humoral and cellular immunity. PLoS One 2013; 8:e63269; http://dx.doi.org/10.1371/journal.pone.0063269; PMID: 23638188
- Dai YC, Wang YY, Zhang XF, Tan M, Xia M, Wu XB, Jiang X, Nie J. Evaluation of anti-norovirus IgY from egg yolk of chickens immunized with norovirus P particles. J Virol Methods 2012; 186:126 - 31; http://dx.doi.org/10.1016/j.jviromet.2012.07.002; PMID: 22867844
- Dai YC, Zhang XF, Tan M, Huang P, Lei W, Fang H, Zhong W, Jiang X. A dual chicken IgY against rotavirus and norovirus. Antiviral Res 2013; ; 97::; 293 - -; 300; PMID: 23267830
- Tan M, Huang P, Xia M, Fang P-A, Zhong W, McNeal M, Wei C, Jiang W, Jiang X. Norovirus P particle, a novel platform for vaccine development and antibody production. J Virol 2011; 85:753 - 64; http://dx.doi.org/10.1128/JVI.01835-10; PMID: 21068235
- Tan M, Huang P, Xia M, Fang PA, Zhong W, McNeal M, Wei C, Jiang W, Jiang X. Norovirus P particle, a novel platform for vaccine development and antibody production. J Virol 2011; 85:753 - 64; http://dx.doi.org/10.1128/JVI.01835-10; PMID: 21068235
- Xia M, Tan M, Wei C, Zhong W, Wang L, McNeal M, Jiang X. A candidate dual vaccine against influenza and noroviruses. Vaccine 2011; 29:7670 - 7; http://dx.doi.org/10.1016/j.vaccine.2011.07.139; PMID: 21839795
- Wang L, Cao D, Wei C, Meng XJ, Jiang X, Tan M. A dual vaccine candidate against norovirus and hepatitis E virus. Vaccine 2014; ; 32::; 445 - -; 52; PMID: 24291540
- Tan M, Xia M, Huang P, Wang L, Zhong W, McNeal M, Wei C, Jiang X. Norovirus P Particle as a Platform for Antigen Presentation. Procedia in Vaccinology 2011; 4:19 - 26; http://dx.doi.org/10.1016/j.provac.2011.07.004
- Wyatt RG, Greenberg HB, Dalgard DW, Allen WP, Sly DL, Thornhill TS, Chanock RM, Kapikian AZ. Experimental infection of chimpanzees with the Norwalk agent of epidemic viral gastroenteritis. J Med Virol 1978; 2:89 - 96; http://dx.doi.org/10.1002/jmv.1890020203; PMID: 97364
- Rockx BH, Bogers WM, Heeney JL, van Amerongen G, Koopmans MP. Experimental norovirus infections in non-human primates. J Med Virol 2005; 75:313 - 20; http://dx.doi.org/10.1002/jmv.20273; PMID: 15602728
- Subekti DS, Tjaniadi P, Lesmana M, McArdle J, Iskandriati D, Budiarsa IN, Walujo P, Suparto IH, Winoto I, Campbell JR, et al. Experimental infection of Macaca nemestrina with a Toronto Norwalk-like virus of epidemic viral gastroenteritis. J Med Virol 2002; 66:400 - 6; http://dx.doi.org/10.1002/jmv.2159; PMID: 11793394
- Bok K, Parra GI, Mitra T, Abente E, Shaver CK, Boon D, Engle R, Yu C, Kapikian AZ, Sosnovtsev SV, et al. Chimpanzees as an animal model for human norovirus infection and vaccine development. Proc Natl Acad Sci U S A 2011; 108:325 - 30; http://dx.doi.org/10.1073/pnas.1014577107; PMID: 21173246
- Bui T, Kocher J, Li Y, Wen K, Li G, Liu F, Yang X, LeRoith T, Tan M, Xia M, et al. Median infectious dose of human norovirus GII.4 in gnotobiotic pigs is decreased by simvastatin treatment and increased by age. J Gen Virol 2013; 94:2005 - 16; http://dx.doi.org/10.1099/vir.0.054080-0; PMID: 23804568
- Cheetham S, Souza M, McGregor R, Meulia T, Wang Q, Saif LJ. Binding patterns of human norovirus-like particles to buccal and intestinal tissues of gnotobiotic pigs in relation to A/H histo-blood group antigen expression. J Virol 2007; 81:3535 - 44; http://dx.doi.org/10.1128/JVI.01306-06; PMID: 17215284
- Cheetham S, Souza M, Meulia T, Grimes S, Han MG, Saif LJ. Pathogenesis of a genogroup II human norovirus in gnotobiotic pigs. J Virol 2006; 80:10372 - 81; http://dx.doi.org/10.1128/JVI.00809-06; PMID: 17041218
- Souza M, Costantini V, Azevedo MS, Saif LJ. A human norovirus-like particle vaccine adjuvanted with ISCOM or mLT induces cytokine and antibody responses and protection to the homologous GII.4 human norovirus in a gnotobiotic pig disease model. Vaccine 2007; 25:8448 - 59; http://dx.doi.org/10.1016/j.vaccine.2007.09.040; PMID: 18022293
- Jung K, Wang Q, Kim Y, Scheuer K, Zhang Z, Shen Q, Chang KO, Saif LJ. The effects of simvastatin or interferon-α on infectivity of human norovirus using a gnotobiotic pig model for the study of antivirals. PLoS One 2012; 7:e41619; http://dx.doi.org/10.1371/journal.pone.0041619; PMID: 22911825
- Liu Y, Huang P, Tan M, Liu Y, Biesiada J, Meller J, Castello AA, Jiang B, Jiang X. Rotavirus VP8*: phylogeny, host range, and interaction with histo-blood group antigens. J Virol 2012; 86:9899 - 910; http://dx.doi.org/10.1128/JVI.00979-12; PMID: 22761376
- Souza M, Azevedo MS, Jung K, Cheetham S, Saif LJ. Pathogenesis and immune responses in gnotobiotic calves after infection with the genogroup II.4-HS66 strain of human norovirus. J Virol 2008; 82:1777 - 86; http://dx.doi.org/10.1128/JVI.01347-07; PMID: 18045944
- Taube S, Kolawole AO, Höhne M, Wilkinson JE, Handley SA, Perry JW, Thackray LB, Akkina R, Wobus CE. A mouse model for human norovirus. MBio 2013; e00450-13:4; http://dx.doi.org/10.1128/mBio.00450-13; PMID: 23860770
- Dolin R, Levy AG, Wyatt RG, Thornhill TS, Gardner JD. Viral gastroenteritis induced by the Hawaii agent. Jejunal histopathology and serologic response. Am J Med 1975; 59:761 - 8; http://dx.doi.org/10.1016/0002-9343(75)90461-1; PMID: 811119
- Dolin R, Blacklow NR, DuPont H, Buscho RF, Wyatt RG, Kasel JA, Hornick R, Chanock RM. Biological properties of Norwalk agent of acute infectious nonbacterial gastroenteritis. Proc Soc Exp Biol Med 1972; 140:578 - 83; http://dx.doi.org/10.3181/00379727-140-36508; PMID: 4624851
- Parrino TA, Schreiber DS, Trier JS, Kapikian AZ, Blacklow NR. Clinical immunity in acute gastroenteritis caused by Norwalk agent. N Engl J Med 1977; 297:86 - 9; http://dx.doi.org/10.1056/NEJM197707142970204; PMID: 405590
- Johnson PC, Mathewson JJ, DuPont HL, Greenberg HB. Multiple-challenge study of host susceptibility to Norwalk gastroenteritis in US adults. J Infect Dis 1990; 161:18 - 21; http://dx.doi.org/10.1093/infdis/161.1.18; PMID: 2153184
- Johnson PC, Hoy J, Mathewson JJ, Ericsson CD, DuPont HL. Occurrence of Norwalk virus infections among adults in Mexico. J Infect Dis 1990; 162:389 - 93; http://dx.doi.org/10.1093/infdis/162.2.389; PMID: 2165112
- Wyatt RG, Dolin R, Blacklow NR, DuPont HL, Buscho RF, Thornhill TS, Kapikian AZ, Chanock RM. Comparison of three agents of acute infectious nonbacterial gastroenteritis by cross-challenge in volunteers. J Infect Dis 1974; 129:709 - 14; http://dx.doi.org/10.1093/infdis/129.6.709; PMID: 4209723
- Zheng DP, Ando T, Fankhauser RL, Beard RS, Glass RI, Monroe SS. Norovirus classification and proposed strain nomenclature. Virology 2006; 346:312 - 23; http://dx.doi.org/10.1016/j.virol.2005.11.015; PMID: 16343580
- Kroneman A, Vega E, Vennema H, Vinjé J, White PA, Hansman G, Green K, Martella V, Katayama K, Koopmans M. Proposal for a unified norovirus nomenclature and genotyping. Arch Virol 2013; 158:2059 - 68; http://dx.doi.org/10.1007/s00705-013-1708-5; PMID: 23615870
- Frenck R, Bernstein DI, Xia M, Huang P, Zhong W, Parker S, Dickey M, McNeal M, Jiang X. Predicting susceptibility to norovirus GII.4 by use of a challenge model involving humans. J Infect Dis 2012; 206:1386 - 93; http://dx.doi.org/10.1093/infdis/jis514; PMID: 22927452
- Lindesmith L, Moe C, Lependu J, Frelinger JA, Treanor J, Baric RS. Cellular and humoral immunity following Snow Mountain virus challenge. J Virol 2005; 79:2900 - 9; http://dx.doi.org/10.1128/JVI.79.5.2900-2909.2005; PMID: 15709009
- Lindesmith LC, Donaldson E, Leon J, Moe CL, Frelinger JA, Johnston RE, Weber DJ, Baric RS. Heterotypic humoral and cellular immune responses following Norwalk virus infection. J Virol 2010; 84:1800 - 15; http://dx.doi.org/10.1128/JVI.02179-09; PMID: 20007270
- Atmar RL, Opekun AR, Gilger MA, Estes MK, Crawford SE, Neill FH, Ramani S, Hill H, Ferreira J, Graham DY. Determination of the 50% human infectious dose for norwalk virus. J Infect Dis 2014; 209:1016 - 22; http://dx.doi.org/10.1093/infdis/jit620; PMID: 24253285
- Lindesmith LC, Donaldson EF, Lobue AD, Cannon JL, Zheng DP, Vinje J, Baric RS. Mechanisms of GII.4 norovirus persistence in human populations. PLoS Med 2008; 5:e31; http://dx.doi.org/10.1371/journal.pmed.0050031; PMID: 18271619
- Siebenga JJ, Vennema H, Renckens B, de Bruin E, van der Veer B, Siezen RJ, Koopmans M. Epochal evolution of GGII.4 norovirus capsid proteins from 1995 to 2006. J Virol 2007; 81:9932 - 41; http://dx.doi.org/10.1128/JVI.00674-07; PMID: 17609280
- Debbink K, Donaldson EF, Lindesmith LC, Baric RS. Genetic mapping of a highly variable norovirus GII.4 blockade epitope: potential role in escape from human herd immunity. J Virol 2012; 86:1214 - 26; http://dx.doi.org/10.1128/JVI.06189-11; PMID: 22090110
- Debbink K, Lindesmith LC, Donaldson EF, Baric RS. Norovirus immunity and the great escape. PLoS Pathog 2012; 8:e1002921; http://dx.doi.org/10.1371/journal.ppat.1002921; PMID: 23093932
- Lindesmith LC, Beltramello M, Donaldson EF, Corti D, Swanstrom J, Debbink K, Lanzavecchia A, Baric RS. Immunogenetic mechanisms driving norovirus GII.4 antigenic variation. PLoS Pathog 2012; 8:e1002705; http://dx.doi.org/10.1371/journal.ppat.1002705; PMID: 22615565
- Lindesmith LC, Costantini V, Swanstrom J, Debbink K, Donaldson EF, Vinjé J, Baric RS. Emergence of a norovirus GII.4 strain correlates with changes in evolving blockade epitopes. J Virol 2013; 87:2803 - 13; http://dx.doi.org/10.1128/JVI.03106-12; PMID: 23269783
- Lindesmith LC, Debbink K, Swanstrom J, Vinjé J, Costantini V, Baric RS, Donaldson EF. Monoclonal antibody-based antigenic mapping of norovirus GII.4-2002. J Virol 2012; 86:873 - 83; http://dx.doi.org/10.1128/JVI.06200-11; PMID: 22090098
- Yang Y, Xia M, Tan M, Huang P, Zhong W, Pang XL, Lee BE, Meller J, Wang T, Jiang X. Genetic and phenotypic characterization of GII-4 noroviruses that circulated during 1987 to 2008. J Virol 2010; 84:9595 - 607; http://dx.doi.org/10.1128/JVI.02614-09; PMID: 20592096
- Lindesmith LC, Donaldson EF, Baric RS. Norovirus GII.4 strain antigenic variation. J Virol 2011; 85:231 - 42; http://dx.doi.org/10.1128/JVI.01364-10; PMID: 20980508
- Tamminen K, Lappalainen S, Huhti L, Vesikari T, Blazevic V. Trivalent combination vaccine induces broad heterologous immune responses to norovirus and rotavirus in mice. PLoS One 2013; 8:e70409; http://dx.doi.org/10.1371/journal.pone.0070409; PMID: 23922988
- Blazevic V, Lappalainen S, Nurminen K, Huhti L, Vesikari T. Norovirus VLPs and rotavirus VP6 protein as combined vaccine for childhood gastroenteritis. Vaccine 2011; 29:8126 - 33; http://dx.doi.org/10.1016/j.vaccine.2011.08.026; PMID: 21854823
- Velasquez LS, Hjelm BE, Arntzen CJ, Herbst-Kralovetz MM. An intranasally delivered Toll-like receptor 7 agonist elicits robust systemic and mucosal responses to Norwalk virus-like particles. Clin Vaccine Immunol 2010; 17:1850 - 8; http://dx.doi.org/10.1128/CVI.00230-10; PMID: 20962211
- Ma Y, Li J. Vesicular stomatitis virus as a vector to deliver virus-like particles of human norovirus: a new vaccine candidate against an important noncultivable virus. J Virol 2011; 85:2942 - 52; http://dx.doi.org/10.1128/JVI.02332-10; PMID: 21228240
- Fang H, Tan M, Xia M, Wang L, Jiang X. Norovirus P particle efficiently elicits innate, humoral and cellular immunity. PLoS One 2013; 8:e63269; http://dx.doi.org/10.1371/journal.pone.0063269; PMID: 23638188