Abstract
Rotavirus (RV) is a common cause of severe gastroenteritis (GE) in children worldwide. Live oral RV vaccines protect against severe RVGE, but the immune correlates of protection are not yet clearly defined. Inner capsid VP6 protein is a highly conserved, abundant, and immunogenic RV protein, and VP6-specific mucosal antibodies, especially IgA, have been implicated to protect against viral challenge in mice. In the present study systemic and mucosal IgG and IgA responses were induced by immunizing BALB/c mice intranasally with a combination of recombinant RV VP6 protein (subgroup II [SGII]) and norovirus (NoV) virus-like particles (VLPs) used in a candidate vaccine. Following immunization mice were challenged orally with murine RV strain EDIMwt (SG non-I-non-II, G3P10[16]). In order to determine neutralizing activity of fecal samples, sera, and vaginal washes (VW) against human Wa RV (SGII, G1P1A[8]) and rhesus RV (SGI, G3P5B[3]), the RV antigen production was measured with an ELISA-based antigen reduction neutralization assay. Only VWs of immunized mice inhibited replication of both RVs, indicating heterotypic protection of induced antibodies. IgA antibody depletion and blocking experiments using recombinant VP6 confirmed that neutralization was mediated by anti-VP6 IgA antibodies. Most importantly, after the RV challenge significant reduction in viral shedding was observed in feces of immunized mice. These results suggest a significant role for mucosal RV VP6-specific IgA for the inhibition of RV replication in vitro and in vivo. In addition, these results underline the importance of non-serotype-specific immunity induced by the conserved subgroup-specific RV antigen VP6 in clearance of RV infection.
Introduction
Group A rotavirus (RV) is common etiological agent of severe gastroenteritis (GE) in infants and young children worldwide with great mortality in the developing world.Citation1 RV particles possess a triple-layered capsid enclosing a genome of 11 segments of double-stranded RNA.Citation2 The external layer of infectious RV particles is formed by 2 proteins, the glycoprotein VP7 and VP4 (forming spikes with hemagglutinating activity in some RV strains), which define the G (glycoprotein) and P (protease-sensitive) genotypes, respectively, of the virus.Citation2 Both of these proteins are essential for virus attachment and entry to the host cellsCitation3,Citation4 and contain major antigenic epitopes which induce type-specific RV neutralizing antibodies (NAbs).Citation2 The intermediate layer of the RV surrounding the VP2 core consists of VP6, which contains viral group (A-G/H) and subgroup (SGI, II, I+II, non-I-non-II for group A) specific antigenic determinants.Citation2,Citation5 The inner capsid protein VP6 is highly conserved with approximately 90% homology at the amino acid level among group A RVs.Citation6 It is also the most abundantCitation2 and highly immunogenic RV protein.Citation7–Citation10 Development of serum VP6-specific antibodies, especially IgA, has been regarded as an indicator of protection after natural RV infection or vaccination.Citation11
Two live attenuated oral RV vaccines, the pentavalent human bovine (WC3) reassortant rotavirus vaccine (RotaTeq®, Merck) and the monovalent G1P1A[8] human rotavirus vaccine (Rotarix®, GlaxoSmithKline) were licensed in 2006 and are now used extensively, but the mechanisms or effectors of protection against RVGE are not clearly defined.Citation1,Citation11 A role of type-specific NAbs to external VP4 and VP7 proteins in the induction of protective immunity after natural RV infection and oral immunization with live RVs is evident,Citation12 but other mechanisms are also important in protection.Citation1,Citation11 This is indirectly indicated by the finding that monovalent Rotarix® vaccine and the pentavalent RotaTeq® vaccine show similar levels of clinical protection against severe RVGE caused by different RV genotypes.Citation13,Citation14 Moreover, the levels of NAbs induced by the vaccines are low and therefore cannot account for the high level of protection of these vaccines.Citation15,Citation16 The evidence that the immune response to VP4 and VP7 is not absolutely required for protection is also supported by the induction of protection against RV infection in mice and rabbits by inactivated double-layered (dl) RV particles,Citation17,Citation18 dl2/6- virus-like particles (VLPs),Citation19–Citation21 chimeric VP6 proteinCitation22,Citation23 or DNA encoding VP6.Citation24,Citation25 The above studies suggest a significant role of VP6 in RV protective immunity, although dl2/6-VLPs have failed to induce protection against disease in gnotobiotic piglets.Citation26
VP6-specific mucosal (intestinal) antibodies, especially IgA antibodies in mice immunized with recombinant VP6 (rVP6) or dl2/6-VLPs have been implicated as correlates of protection against RV challenge.Citation25,Citation27,Citation28 Moreover, it has been reported that anti-VP6 polymeric IgA (pIgA) impairs RV infection by intracellular inhibition of RV replication.Citation29–Citation33 While it is generally accepted that antibodies directed against the internal RV protein VP6 have no neutralizing activity in vitro, there are a few reports to the contrary,Citation34–Citation37 mainly relating to the inhibitory activity of llama-derived single-chain antibody fragments.Citation35–Citation37 To add to the evidence, in the present study we show that mucosal VP6-specific IgA antibodies inhibit RV infection in vitro. Furthermore, VP6-specific immune response induced in vivo protection in BALB/c mice challenged with murine RV strain EDIM.
Results
Intranasal immunization induced high systemic and mucosal antibody responses
High systemic and mucosal IgG and IgA responses were induced by intranasal (IN) immunization of mice with the candidate combination vaccine containing equal quantities of RV rVP6 protein and norovirus (NoV) VLPs as determined by enzyme-linked immunosorbent assay (ELISA). IN immunization route was used to obtain maximum amount of mucosal antibodies, specifically IgA, as IgA antibodies have been implicated in protection against RV infection in vivoCitation25,Citation27–Citation30 and in inhibition of RV replication in vitro.Citation31–Citation33
Two immunization doses resulted in high levels of anti-VP6 IgG and moderate levels of anti-VP6 IgA antibodies in the serum of the experimental group with end point titers of >4.4log10 (OD490 2.4 at a 1:200 dilution) and >2.5log10 (OD490 0.4 at a 1:10 dilution), respectively (). Further, considerably high levels of IgG antibodies were detected in groupwise pooled 10% fecal suspensions (OD490 1.4 at a 1:2 dilution) and vaginal washes (VWs, OD490 2.2 at 1:2 dilution) of immunized animals (). Similarly, IgA antibodies were detected in the mucosal secretions, with OD490 values of 0.9 and 1.7 (ratio 0.5) for 1:2 diluted fecal samples and VWs, respectively (). The ratios of serum IgA to VW and fecal IgA were 0.5 and 1.8, respectively. All samples from control mice were negative for both RV-specific IgG and RV-specific IgA antibodies ().
Figure 1. Detection of serum and mucosal VP6-specific antibody responses following intranasal immunization. Endpoint titration of VP6-specific IgG (A) and IgA (B) antibodies in serum of individual mice (5 mice/group) after 2 intranasal immunizations with the combination vaccine containing rotavirus rVP6 and norovirus VLPs, each at a 10 µg dose. Control (ctrl) mice received only PBS. Mean titers of sera at study week 5 are shown. Error bars represent standard error of the means. (C) Endpoint titration of VP6-specific IgG and IgA antibodies in mucosal samples of experimental groups. Mean titers of groupwise pooled (5 mice/group) vaginal washes (VW) and fecal suspensions of at least 2 (2–4) independent experiments at week 5 are shown. (D) Characterization of different IgA forms in mucosal samples from immunized mice by immunoblot analysis under non-reducing conditions. Polymeric IgA (pIgA) forms including secretory IgA (SIgA) were confirmed upon immunodetection with goat anti-mouse IgA. Molecular weight markers (in kDa) are indicated with arrows.
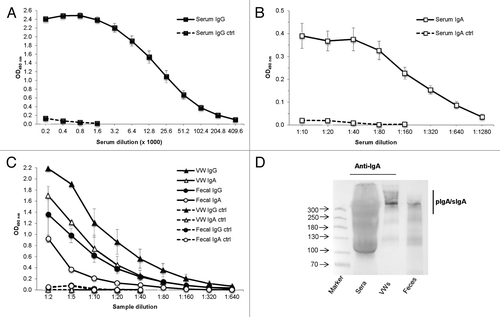
Mucosal samples contain polymeric forms of IgA
Molecular forms of IgA were determined in sera, feces, and VWs of immunized mice by immunoblotting under non-reducing conditions. Most IgA antibodies in mucosal samples but not in the serum samples of the experimental group were found in the polymeric forms ().
In vitro inhibition of RV infection
The ability of VP6-specific antibodies to inhibit RV infection was studied in vitro by an ELISA-based RV antigen reduction neutralization assay.Citation38,Citation39 Functionality of the neutralization assay against RV Wa and RRV was confirmed using human RV-positive and RV-negative sera (data not shown). Mouse samples after 2 immunizations were tested for neutralization against these 2 RV strains, namely Wa (SGII, G1P1A[8]) homologous to the rVP6 used for immunization, and RRV (SGI, G3P5B[3]) (). No neutralizing effect was detected in the sera () of immunized or control groups. Several attempts to test the neutralizing activity of fecal samples failed each time, as the samples from the mice, including control, and even at high dilutions, were toxic for cell cultures—an observation made by others as well.Citation40 Instead, VWs containing levels of mucosal antibodies similar to those of the fecal suspensions () were used in neutralization assays. VWs from immunized group neutralized both RV strains belonging to different SGs. These results indicate cross-reactive neutralizing activity of VP6-specific mucosal antibodies in vitro. More precisely, inhibition of infectivity of RV Wa and RRV was detected with neutralizing titers of 569 and 213, respectively (). The VW samples from control mice did not inhibit RV infection, whereas positive human control serum always neutralized both viruses.
Figure 2. Inhibition activity of sera and vaginal washes of intranasally immunized mice against human rotavirus (RV) Wa (homologous VP6 to the immunizing rVP6 protein) or rhesus RV (RRV) using an ELISA-based antigen reduction neutralization assay. Mice were immunized with the combination vaccine containing RV rVP6 and norovirus virus-like particles. Control mice received no immunogen. Sera of RV-seropositive (RV+) and RV-seronegative (RV–) human donors were used as assay controls. The reciprocal of the sample dilution that generated >60% reduction in virus infectivity was considered its titer. If the highest dilution (1:10) did not yield neutralization of >60%, a titer of 5 was assigned as the neutralization titer of the sample. Results of study week 5 are represented as the geometric mean neutralizing titer of at least 2 independent experiments, each done in duplicate, with standard errors. NT, not tested.
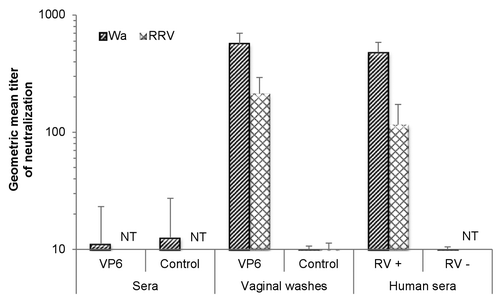
VP6-specific mucosal IgA mediates RV inhibition
The neutralizing activity of the VWs was confirmed to be associated with the VP6 binding by blocking experiments. The neutralization ability against Wa was completely blocked by preincubation of the VW samples with rVP6 protein compared with the untreated VW samples (P = 0.001) (). In contrast, preincubation of serial VW dilutions with unrelated recombinant NoV GII-4 VLPs had no effect (P = 0.073) on the neutralizing activity (). Similar results were observed with RRV, when preincubating the samples with rVP6 reduced the neutralization (P = 0.001) by immune VWs (). Furthermore, IgA antibodies were shown to mediate the RV Wa and RRV inhibition. When IgA of VWs was depleted with magnetic beads prior to use in a neutralization assay, the neutralizing activity for RV Wa as well as RRV was abolished (). An IgA ELISA assay confirmed removal of the IgA antibodies from the VWs of the experimental group with the finding of OD490 values of 0.80 and 0.01 for the 1:10 dilutions of VWs before and after the depletion, respectively. IgA depletion did not drastically alter the IgG content of the samples, which retained significant amount of IgG (OD490 1.4 vs. OD490 0.8).
Figure 3. VP6-specific mucosal IgA mediates RV inhibition in vitro. (A) Blocking of the inhibition of human rotavirus (RV) Wa and rhesus RV (RRV) infection in vitro by VP6-specific antibodies of mice immunized intranasally with the combination vaccine containing RV rVP6 and norovirus (NoV) virus-like particles (VLPs). Control (Ctrl) mice received no immunogen. Vaginal wash (VW) samples from immunized mice were preincubated with rVP6 protein or NoV GII-4 VLPs prior the neutralization assay or left untreated. (B) IgA antibodies mediate RV Wa and RRV inhibition in vitro. IgA was depleted (–IgA) from VWs of immunized mice prior to neutralization assay, resulting in reduced inhibitory activity compared with untreated VWs. The dashed line indicates the 60% cut-off for reduction in virus infectivity. Results of at least 2 independent experiments, each done in duplicate, with standard errors are shown.
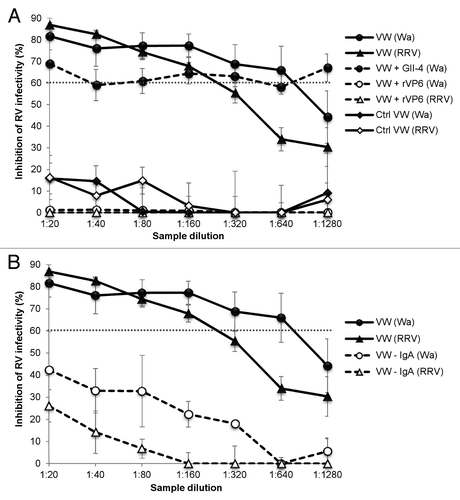
Protective efficacy against RV challenge
To determine the protection conferred by the combination vaccine, immunizations were repeated and mice from immunized and control groups were challenged with murine RV EDIMwt at study week 6. Viral shedding curves show that the viral antigen shedding in feces of VP6 immunized mice was decreased significantly (P = 0.021) compared with the control mice (). High shedding was observed from day 2 to day 5 in control group, whereas the immunized group shed virus only at day 3. Total reduction in shedding of the vaccinated group was 62.0% (± 18.3%) compared with the control group. For undetermined reasons in one mouse antigen shedding was higher than in any of control mice. More consistent reduction of virus shedding (77.5 ± 12.6%) was detected in remaining 4 of the 5 animals immunized (). Since all experimental mice had similar levels of serum VP6-specific IgG and IgA (data not shown) before the challenge, the failure in protection of the particular mouse cannot be explained with the pre-existing antibody levels. In addition, intestinal IgA antibodies were detected prior to the challenge as well (OD490 0.3 ± 0.08 at 1:10 dilution) and the level was similar to the level shown in .
Figure 4. Effect of intranasal immunization with candidate vaccine on rotavirus shedding. Groups of mice were immunized intranasally twice with the candidate vaccine containing rotavirus (RV) rVP6 and norovirus virus-like particles, each at a 10 µg dose. Three weeks after the second dose mice were challenged orally with EDIMwt (100-fold DD50) and the quantity of RV antigen shed in fecal samples was determined up to 8 d post-challenge by ELISA. Each point represents the daily average of a group of mice (4 mice/group) with standard error of the means.
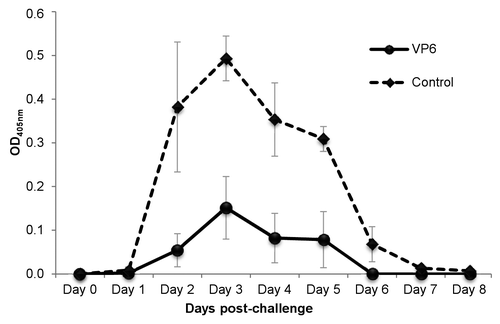
Discussion
We have previously shown that a candidate combination vaccine against NoV and RV containing a mixture of NoV VLPs and RV VP6 protein delivered parenterally to BALB/c mice induced high levels of systemic VP6-specific cross-reactive serum IgG antibodies as well as T cells.Citation41–Citation43 Further, mucosal antibodies induced by intramuscular (IM) application of the combination vaccine inhibited RV infection in vitro.Citation43 Since mucosal VP6-specific IgA antibodies have been associated with protection against RV in vivoCitation25,Citation27–Citation30 and inhibition of RV replication in vitro,Citation31–Citation33 in the present work we have used IN delivery of the combined vaccine in order to induce high levels of mucosal VP6-specific antibody responses. Our results show that mucosal IgA antibodies inhibited RV infection in vitro. Importantly, in vivo protection from RV challenge was induced by immunization with VP6 protein.
Although in vivo protection from RV challenge correlating with VP6-specific mucosal (intestinal) IgG and IgA antibodies in mice immunized with rVP6 has been documented,Citation25,Citation27,Citation28 antibodies against VP6 have been thought to have no neutralizing activity in vitro. However, few studies have described neutralizing activity of VP6-specific serum antibodies,Citation34–Citation37 mainly relating to small-sized llama-derived antibody fragments against VP6,Citation35–Citation37 in a traditional in vitro assay. Nevertheless, anti-VP6 IgA mucosal antibodies have been indicated to impair RV infection in vivo and in vitro by intracellular neutralization.Citation29–Citation33
Intranasal immunization with different antigens has been shown to be effective in inducing antigen-specific secretory IgA (SIgA) in intestinal surfaces but also in other mucosal sites including vagina.Citation44,Citation45 Similarly, in the present study mucosal VP6-specific IgG and IgA were detected in considerable quantities in intestine and VWs of immunized mice. However, neutralization experiments of RVs by fecal suspensions failed in spite of several attempts. Failures to detect VP6-specific in vitro neutralization activity can most likely be explained by toxicity of the fecal suspensions to the MA104 cells, as seen previously by others.Citation40 For this reason, the VWs containing comparable levels of mucosal IgG and IgA antibodies as fecal suspensions were used for the neutralization experiments, where antibody content of these VWs served as a proxy indicator for fecal antibodies. Samples of VWs were able to inhibit infection of MA104 cells with human Wa RV strain homologous to the immunization protein rVP6, as well as with a heterologous rhesus RV strain. A similar inhibition was also observed previously with VWs from mice immunized IM with the candidate vaccine, rVP6 or dl2/6-VLPsCitation43,Citation46 although the neutralization titer to Wa RV was increased by a factor of 3.6 with IN administration, possible due to the higher levels of mucosal antibodies in VWs of IN immunized mice. Neutralization of both RV strains irrespective of subgroup or G- and P-type indicates heterotypic protection of these antibodies in vitro, which is in concordance with the work of others.Citation30,Citation35
Preincubation of VWs with rVP6 protein efficiently reduced the inhibition of RV infectivity by blocking antibody binding sites and consequently neutralization activity. By contrast, an unrelated protein, GII-4 VLPs derived from NoV capsid VP1, did not reduce this activity. These results strongly suggest that heterotypic neutralization activity was conferred by VP6-specific antibodies. In addition, depletion experiments using magnetic beads indicated that IgA antibodies were mediating the RV inhibition. However, the role of mucosal IgG antibodies as mediators of the inhibition warrants further investigation. Although neutralizing activity of sera containing considerable levels of VP6 specific IgA was not detected, potential neutralizing capacity of serum VP6-specific IgA cannot be definitively excluded. Since no inhibition of RV infectivity by sera could be seen, preferably, VP6-specific IgA in the polymeric form present in mucosal washes may be responsible for prevention of RV infectivity. Generally, the reliability of using anti-RV serum IgA antibody titers as a correlate of protection or vaccine efficacy is controversial.Citation47–Citation50 Recently, studies in developing countries have indicated that RV-specific IgA levels in serum are not an optimal correlate of protection following vaccination.Citation47
The mechanisms by which VP6-specific antibodies exert the neutralizing effect are not yet understood, but it has been shown that VP6-specific pIgA neutralizes RV by inhibiting virus replication intracellularly.Citation31,Citation32 Indeed, recent data by Aiyegbo and coworkersCitation33 indicate that the neutralizing activity of human VP6-specific IgA antibody results from the inhibition of viral transcription inside the cells during polymeric Ig receptor-mediated transcytosis of pIgA, which in turn suggests that intracellular neutralization by naturally-occurring RV VP6-specific pIgAs likely contributes to human protective immunity to RV. This mechanism could also partly account for the RV inhibition we have observed, although our studies have not specifically examined intracellular mechanisms with polarized cells.Citation32,Citation33,Citation51 This assumption is based on non-reducing immunoblot analysis, where mostly polymeric forms of IgA were detected in the mucosal samples. These pIgAs as well as SIgA have been shown to bind to intact RV particlesCitation32 through the holes in the capsid or via areas of partial decapsidationCitation52 and could therefore interact with dl particles inside the cell. In support of the above, we have also detected direct binding of VP6-specific mucosal but not serum IgA antibodies to the non-denatured RVs coated onto ELISA plates (data not shown). Alternatively to the post-entry infection events, RV infection could be inhibited during the virus entry, as shown by Gualtero and colleagues.Citation34 They suggested that VP6-specific antibodies blocked binding of VP6 to heat shock cognate protein 70, a co-receptor for RV entry, into the susceptible cells.
In this study, in vivo protection against RV challenge induced by human-derived RV VP6 protein was also assessed. Protective efficacy was measured in an adult mouse model based on decreased RV antigen shedding originally described by Ward et al.Citation53 Consequently, the candidate vaccine administered IN induced 77.5% reduction in RV replication in mice challenged with murine RV strain EDIM suggesting that the candidate vaccine is effective in conferring immunoprotection against RV challenge. Although 1 of 5 mice was not protected for unknown reason, very recent publication describing similar reduction in RV shedding in mice immunized with VP6 tubular structuresCitation54 support our observation. These results indicate the importance of VP6-specific immune response in heterotypic protection, which is in concordance with the previously published results where protection against 2 different murine RV strains, EDIM and EMcN, was demonstrated after mucosal immunizations with E. coli–expressed MBP-VP6 derived from human CJN strain.Citation23 Although VP6-specific mucosal IgA antibodies have been implicated as correlates of protection against RV challenge in mice immunized with VP6 or dl2/6-VLPs,Citation25,Citation27,Citation28 other studies imply no protective role for VP6 specific mucosal antibodies.Citation22,Citation23,Citation55 These differences may be explained with the induction of different effectors or mediators of protective immune responses depending on nature of RV VP6 protein used for immunization, e.g., being a monomerCitation55 or oligomeric structures.Citation28 In addition, the difference may come from an adjuvant being used or not.Citation28,Citation55 Nonetheless, the protection we observed in adult mouse shedding model not necessarily assure protection in RV animal disease models. For example dl2/6-VLPs have not conferred protection against RV disease in RV-infected gnotobiotic piglets.Citation26 Since the vaccine formulation necessary to induce protection from RV disease or virus shedding may differ, the protective efficacy of vaccine formulation will need to be tested in different animal models and eventually in humans.
In conclusion, in the present study we show that VP6-specific mucosal IgA antibodies induced by IN immunization inhibited RV infection in vitro. Most importantly, the mice immunized with the candidate vaccine containing RV VP6 protein were protected against RV challenge in vivo. Although the mechanisms of protection were not explored directly, our results give important insights on the non-serotype-specific protective immunity to RV induced by the VP6 protein immunization.
Materials and Methods
Rotavirus rVP6 production and characterization
Human RV rVP6 protein originating from a fecal sample from a RV G1P1A[8]-positive patient was produced in Sf9 insect cells by the Bac-to-Bac baculovirus expression system (Invitrogen, Cat. 10359-016) and purified by ultracentrifugation and ultrafiltration as previously described.Citation41,Citation42,Citation46 The rVP6 protein and NoV VLPs were mixed in equal quantities to produce a combination for a candidate vaccineCitation41 used for immunization in a series of preclinical studies including the present study. In addition, the purified rVP6 was used as an antigen in ELISA.
Immunization of mice and sample collection
Female 7-wk-old BALB/c OlaHsd mice (Harlan Laboratories) were immunized IN twice (at weeks 0 and 3) with the candidate vaccine containing a mixture of recombinant RV VP6 and NoV VLPs,Citation41,Citation42 each at a dose of 10 µg per immunization point, without an external adjuvant. The immunogen was administered in a 25-µL volume by gradual inoculation in each nostril. Naïve mice receiving carrier only (sterile PBS) were used as controls. The immunization procedures were performed 3 separate times in different groups of mice (4–5 mice/group).
Blood samples, feces, and VWs were collected at week 0 (pre-immune sample) and at the time of termination at week 5. Fresh feces of mice were suspended to 10% w/v in cold TNC buffer (10 mM Tris, Cat. T1378; 100 mM NaCl, Cat. 31434N; 1 mM CaCl2, pH 7.4, Cat. C5670) supplemented with 1% aprotinin (Cat. A6279) and 10 μM leupeptin (Cat. L2884) (all from Sigma–Aldrich). Serum samples were prepared according to Tamminen et al.Citation56 Since IN delivery is effective in inducing SIgA antibodies in different locations including the vagina,Citation44,Citation45 vaginal secretions were collected by washing twice with 125 μl of cold PBS (4–5 times up and down) followed by centrifugation at 12 000 × g for 10 min at +4 °C. Antibody content of these VWs served as a surrogate for mucosal immune responses. All procedures were performed in accordance with the regulations and guidelines of the Finnish Animal Experiment Board.
Humoral immune response detection
Induction of VP6-specific systemic and mucosal antibody responses was determined by measuring levels of RV VP6-specific IgG and IgA in sera, fecal suspensions, and VWs. Sera of each mouse at 1:200 dilution and 2-fold dilution series were tested for total RV VP6-specific IgG antibodies by ELISA assay,Citation41,Citation46 where Costar High Binding 96-well half area polystyrene plates (Corning Inc., Cat. 3690) were coated with 40 ng/well of rVP6 protein in PBS. For detection of serum and mucosal VP6-specific IgA, as well as mucosal IgG antibodies (sera, stool suspensions, and VWs tested at 1:2 or 1:5 dilutions and 2-fold dilution series), the plates were coated with 50 ng/well of rVP6. VP6-specific antibodies were detected with horseradish peroxidase (HRP)-conjugated goat anti-mouse IgG (Sigma-Aldrich, Cat. A4416) or anti-mouse IgA (Sigma-Aldrich, Cat. A4789) at a dilution of 1:4000 and SIGMA FAST o-phenylenediamine dihydrochloride (OPD) substrate (Sigma-Aldrich, Cat. P9187-50SET). Optical density at 490 nm (OD490) was measured by Victor2 1420 microplate reader (Perkin Elmer) and a sample was considered positive if the OD490 was above the set cut-off value (mean OD490 of control mice + 3 × SD) and at least 0.1. The titers were defined as the reciprocal of the highest sample dilution with a mean OD490 above the cut-off value.
Immunoblotting for characterization of IgA forms
The quality of serum and mucosal samples of immunized mice was assessed by electrophoresis followed by immunodetection analysis. Pools of sera, stool samples and VWs were prepared and separated by sodium dodecyl sulfate PAGE (SDS-PAGE) with 4–15% Mini-PROTEAN® TGX™ (Bio-Rad Laboratories, Cat. 456-1083) gels under non-reducing conditions. Each sample pool was mixed with an equal volume of Laemmli sample buffer (Bio-Rad, Cat. 161-0737) without β-mercaptoethanol and incubated for 5 min at 95 °C before being electrophoresed in 1 × Tris/Glycine/SDS buffer (Bio-Rad, Cat. 161-0732) for ~45 min at 200 V. This allowed the detection of SIgA complexes followed by immunoblotting to the nitrocellulose membrane (Bio-Rad, Cat. 162-0115) with 1:1000 diluted goat anti-mouse IgA-HRP antibody (Sigma-Aldrich, Cat. A4789). Bound antibodies were detected with OPTI-4CN™ Substrate kit (Bio-Rad, Cat. 170-8235) according to the manufacturer’s instructions.
Preparation of viral stocks
Fetal rhesus monkey kidney (MA104) cells were maintained in Earle’s minimum essential medium (Gibco, Cat. 21090-022) supplemented with 10% fetal bovine serum (Sigma, Cat. F9665), 2 mM L-glutamine (Gibco, Cat. 25030-024), 100 U/mL penicillin (Gibco, Cat. 15140-122), and 100 µg/mL streptomycin (Gibco, Cat. 15140-122) at 37 °C in a 5% humidified CO2 incubator. Human RV strain Wa (SGII, G1P1A[8]) and rhesus rotavirus (RRV, SGI, G3P5B[3]) were propagated in MA104 cells in the presence of 0.5 µg/mL of trypsin from porcine pancreas (Sigma-Aldrich, Cat. T4799). After observing the cytopathic effect, viral stocks were prepared from infected cells by 3 cycles of freezing–thawing, centrifuging at 2000 × g for 10 min for clarification, and storing at −70 °C. The viral titer of the stocks in plaque-forming units per milliliter (PFU/mL) of virus was determined by virus plaque assay.Citation57
The murine RV strain EDIMwt (SG non-I-non-II, G3P10[16]) used in challenge study was originally obtained from Dr Richard Ward (Gamble Institute of Medical Research, Cincinnati, Ohio). The EDIM virus, pooled from fecal specimens of infected neonatal mice, was prepared as previously described.Citation53
Determination of neutralizing anti-RV antibodies in vitro
Neutralizing activity of VP6-specific antibodies in sera, fecal samples, and VWs of immunized mice was determined by measuring the reduction in RV antigen production with an ELISA-based antigen reduction neutralization assay (NELISA) as described previously by others.Citation38,Citation39 The functionality of the assay was first confirmed against RV Wa by using human polyclonal anti-RV serum obtained from a patient with RV infection, later always included as a positive assay control. A human polyclonal serum negative for RV was used as a negative assay control. The stool samples from human serum donors were first analyzed for RV detection by RT-PCR.Citation58 Both sera were then characterized for the presence or absence of RV-specific immunoglobulins by an ELISA.Citation59 A series of 2-fold dilutions (1:10 to 1:1280) of each groupwise pooled specimen from immunized and control mice were mixed with equal volumes of Wa RV or RRV containing 125 PFU and preincubated at +37 °C for 60 min to ensure that anti-VP6 antibodies would bind to any exposed VP6 in the virion. Confluent MA104 monolayers in 96-well plates (Nunc, Cat. 167008) were overlaid with the mixture and the plates were centrifuged at 1000 × g for 60 min. The virus inoculum was replaced with medium supplemented with 4 µg/mL of trypsin followed by incubation at 37 °C for 15 h, lysing of the cells by a cycle of freezing–thawing, and storage at −80 °C. RV antigen production of each sample in duplicate was measured by an ELISA using insect-cell-derived rVP6 as an internal standard. A reduction of OD450 value in the duplicate wells by >60% compared with the untreated virus control wells (trypsin activated RV Wa or RRV without the test sample) was considered to indicate neutralizing ability of the sample. Neutralizing titers were expressed as the reciprocal of the highest sample dilution yielding neutralization. If the highest sample dilution (1:10) failed to neutralize, a titer of 5 was assigned as the neutralization titer of the sample.
To determine whether VP6-specific antibodies were responsible for the neutralizing activity, the antibody binding sites were blocked by preincubation of VW serial dilutions with 10 μg of the rVP6 protein or with recombinant baculovirus produced unrelated GII-4 capsid VP1 protein derived from NoVCitation41 at +37 °C for 1 h prior to neutralization assay.
VP6-specific antibody depletion
To assess whether IgA antibodies mediated the RV inhibition, IgA of VW was depleted with magnetic bead treatment, where 6.7 × 107/mL of Dynabeads M-280 Streptavidin (Life Technologies, Cat. 11205D) combined with 40 µg of biotinylated goat anti-mouse IgA (Life Technologies, Cat. M31115) were incubated with the sample prior to neutralization assay. The IgA-depleted supernatant separated from the beads by the magnet (DynaMag™-Spin, Life Technologies, Cat. 12320D) was employed in the NELISA to test the residual neutralization activity. The level of IgA depletion of the VW was verified by the ELISA as described above.
Virulent murine RV challenge and detection of RV antigen in fecal samples
For the protection study 8–10 mice/group were immunized as described above. Three weeks after the last immunization half of the immunized mice were challenged orally with 1 × 104 FFU (100-fold the diarrheal dose DD50) of the murine RV strain EDIMwt. The feces of challenged mice were collected prior to challenge (day 0) and daily for 8 d after the challenge, and the presence of RV antigen in fecal samples suspended in TNC buffer was determined with ELISA as previously described.Citation60 Fecal antigen shedding was expressed as the net OD value (OD of the post-challenge fecal sample minus the OD of pre-challenge sample from the same mouse). A sample was considered positive if the net OD405 was ≥0.1. Viral shedding curves for each animal were plotted and the reduction in viral load was calculated by comparing the mean area under the curve of the immunized mice to the mean area under the curve of the control group. A >50% reduction in virus shedding was considered significant protection from virus challenge. Mice were sacrificed at day 8 after the challenge. To confirm the success of immunizations, pre-challenge sera from study week 5 were tested for VP6-specific IgG and IgA antibodies as described above.
Statistical analyses
Mann–Whitney U-test was employed to assess the statistical difference between 2 independent groups. Analyses were conducted by IBM SPSS Statistics -software (SPSS Inc.) version 20.0, where P ≤ 0.05 was considered to be statistically significant.
Abbreviations: | ||
dl | = | double-layered |
ELISA | = | enzyme-linked immunosorbent assay |
FFU | = | focus forming units |
GE | = | gastroenteritis |
HRP | = | horseradish peroxidase |
IM | = | intramuscular |
IN | = | intranasal |
NAbs | = | neutralizing antibodies |
NELISA | = | ELISA-based antigen reduction neutralization assay |
NoV | = | norovirus |
OD | = | optical density |
OPD | = | o-phenylenediamine dihydrochloride |
PFU | = | plaque-forming units |
pIgA | = | polymeric IgA |
RV | = | rotavirus |
rVP6 | = | recombinant VP6 |
SDS-PAGE | = | sodium dodecyl sulphate polyacrylamide gel electrophoresis |
SG | = | subgroup |
SIgA | = | secretory IgA |
VLP | = | virus-like particle |
VW | = | vaginal wash |
Disclosure of Potential Conflicts of Interest
No potential conflicts of interest were disclosed.
Acknowledgments
We gratefully acknowledge the technical assistance given by the laboratory personnel of the Vaccine Research Center of University of Tampere Medical School. Special thanks are due to Eeva Jokela and Marianne Karlsberg for their contribution to the laboratory methods. We are also grateful to Vanessa Hernández of Instituto de Biotecnología (Universidad Nacional Autónoma de México) for technical assistance in RV challenge.
References
- Desselberger U, Huppertz HI. Immune responses to rotavirus infection and vaccination and associated correlates of protection. J Infect Dis 2011; 203:188 - 95; http://dx.doi.org/10.1093/infdis/jiq031; PMID: 21288818
- Estes MK, Kapikian A. Rotaviruses. In: Knipe DM, Howley PM, Griffin DE, Lamb RA, Martin MA, Roizman B, Straus SE, eds. Fields Virology, 5th Edn. Philadelphia: Lippincott Williams & Wilkins, 2007:1917–74.
- Ludert JE, Feng N, Yu JH, Broome RL, Hoshino Y, Greenberg HB. Genetic mapping indicates that VP4 is the rotavirus cell attachment protein in vitro and in vivo. J Virol 1996; 70:487 - 93; PMID: 8523562
- Graham KL, Halasz P, Tan Y, Hewish MJ, Takada Y, Mackow ER, Robinson MK, Coulson BS. Integrin-using rotaviruses bind alpha2beta1 integrin alpha2 I domain via VP4 DGE sequence and recognize alphaXbeta2 and alphaVbeta3 by using VP7 during cell entry. J Virol 2003; 77:9969 - 78; http://dx.doi.org/10.1128/JVI.77.18.9969-9978.2003; PMID: 12941907
- Matthijnssens J, Otto PH, Ciarlet M, Desselberger U, Van Ranst M, Johne R. VP6-sequence-based cutoff values as a criterion for rotavirus species demarcation. Arch Virol 2012; 157:1177 - 82; http://dx.doi.org/10.1007/s00705-012-1273-3; PMID: 22430951
- Tang B, Gilbert JM, Matsui SM, Greenberg HB. Comparison of the rotavirus gene 6 from different species by sequence analysis and localization of subgroup-specific epitopes using site-directed mutagenesis. Virology 1997; 237:89 - 96; http://dx.doi.org/10.1006/viro.1997.8762; PMID: 9344910
- Estes MK, Crawford SE, Penaranda ME, Petrie BL, Burns JW, Chan WK, Ericson B, Smith GE, Summers MD. Synthesis and immunogenicity of the rotavirus major capsid antigen using a baculovirus expression system. J Virol 1987; 61:1488 - 94; PMID: 3033276
- Svensson L, Sheshberadaran H, Vene S, Norrby E, Grandien M, Wadell G. Serum antibody responses to individual viral polypeptides in human rotavirus infections. J Gen Virol 1987; 68:643 - 51; http://dx.doi.org/10.1099/0022-1317-68-3-643; PMID: 3029295
- Svensson L, Sheshberadaran H, Vesikari T, Norrby E, Wadell G. Immune response to rotavirus polypeptides after vaccination with heterologous rotavirus vaccines (RIT 4237, RRV-1). J Gen Virol 1987; 68:1993 - 9; http://dx.doi.org/10.1099/0022-1317-68-7-1993; PMID: 3037019
- Ishida S, Feng N, Tang B, Gilbert JM, Greenberg HB. Quantification of systemic and local immune responses to individual rotavirus proteins during rotavirus infection in mice. J Clin Microbiol 1996; 34:1694 - 700; PMID: 8784572
- Franco MA, Angel J, Greenberg HB. Immunity and correlates of protection for rotavirus vaccines. Vaccine 2006; 24:2718 - 31; http://dx.doi.org/10.1016/j.vaccine.2005.12.048; PMID: 16446014
- Offit PA, Blavat G. Identification of the two rotavirus genes determining neutralization specificities. J Virol 1986; 57:376 - 8; PMID: 3001359
- Ruiz-Palacios GM, Pérez-Schael I, Velázquez FR, Abate H, Breuer T, Clemens SC, Cheuvart B, Espinoza F, Gillard P, Innis BL, et al, Human Rotavirus Vaccine Study Group. Safety and efficacy of an attenuated vaccine against severe rotavirus gastroenteritis. N Engl J Med 2006; 354:11 - 22; http://dx.doi.org/10.1056/NEJMoa052434; PMID: 16394298
- Vesikari T, Matson DO, Dennehy P, Van Damme P, Santosham M, Rodriguez Z, Dallas MJ, Heyse JF, Goveia MG, Black SB, et al, Rotavirus Efficacy and Safety Trial (REST) Study Team. Safety and efficacy of a pentavalent human-bovine (WC3) reassortant rotavirus vaccine. N Engl J Med 2006; 354:23 - 33; http://dx.doi.org/10.1056/NEJMoa052664; PMID: 16394299
- Block SL, Vesikari T, Goveia MG, Rivers SB, Adeyi BA, Dallas MJ, Bauder J, Boslego JW, Heaton PM, Pentavalent Rotavirus Vaccine Dose Confirmation Efficacy Study Group. Efficacy, immunogenicity, and safety of a pentavalent human-bovine (WC3) reassortant rotavirus vaccine at the end of shelf life. Pediatrics 2007; 119:11 - 8; http://dx.doi.org/10.1542/peds.2006-2058; PMID: 17200266
- Vesikari T. Rotavirus vaccination: a concise review. Clin Microbiol Infect 2012; 18:Suppl 5 57 - 63; http://dx.doi.org/10.1111/j.1469-0691.2012.03981.x; PMID: 22882248
- McNeal MM, Rae MN, Conner ME, Ward RL. Stimulation of local immunity and protection in mice by intramuscular immunization with triple- or double-layered rotavirus particles and QS-21. Virology 1998; 243:158 - 66; http://dx.doi.org/10.1006/viro.1998.9060; PMID: 9527925
- McNeal MM, Rae MN, Bean JA, Ward RL. Antibody-dependent and -independent protection following intranasal immunization of mice with rotavirus particles. J Virol 1999; 73:7565 - 73; PMID: 10438846
- Ciarlet M, Crawford SE, Barone C, Bertolotti-Ciarlet A, Ramig RF, Estes MK, Conner ME. Subunit rotavirus vaccine administered parenterally to rabbits induces active protective immunity. J Virol 1998; 72:9233 - 46; PMID: 9765471
- O’Neal CM, Crawford SE, Estes MK, Conner ME. Rotavirus virus-like particles administered mucosally induce protective immunity. J Virol 1997; 71:8707 - 17; PMID: 9343229
- Bertolotti-Ciarlet A, Ciarlet M, Crawford SE, Conner ME, Estes MK. Immunogenicity and protective efficacy of rotavirus 2/6-virus-like particles produced by a dual baculovirus expression vector and administered intramuscularly, intranasally, or orally to mice. Vaccine 2003; 21:3885 - 900; http://dx.doi.org/10.1016/S0264-410X(03)00308-6; PMID: 12922123
- Choi AH, Basu M, McNeal MM, Clements JD, Ward RL. Antibody-independent protection against rotavirus infection of mice stimulated by intranasal immunization with chimeric VP4 or VP6 protein. J Virol 1999; 73:7574 - 81; PMID: 10438847
- Choi AH, McNeal MM, Basu M, Flint JA, Stone SC, Clements JD, Bean JA, Poe SA, VanCott JL, Ward RL. Intranasal or oral immunization of inbred and outbred mice with murine or human rotavirus VP6 proteins protects against viral shedding after challenge with murine rotaviruses. Vaccine 2002; 20:3310 - 21; http://dx.doi.org/10.1016/S0264-410X(02)00315-8; PMID: 12213401
- Herrmann JE, Chen SC, Fynan EF, Santoro JC, Greenberg HB, Wang S, Robinson HL. Protection against rotavirus infections by DNA vaccination. J Infect Dis 1996; 174:Suppl 1 S93 - 7; http://dx.doi.org/10.1093/infdis/174.Supplement_1.S93; PMID: 8752297
- Chen SC, Jones DH, Fynan EF, Farrar GH, Clegg JC, Greenberg HB, Herrmann JE. Protective immunity induced by oral immunization with a rotavirus DNA vaccine encapsulated in microparticles. J Virol 1998; 72:5757 - 61; PMID: 9621034
- Yuan L, Geyer A, Hodgins DC, Fan Z, Qian Y, Chang KO, Crawford SE, Parreño V, Ward LA, Estes MK, et al. Intranasal administration of 2/6-rotavirus-like particles with mutant Escherichia coli heat-labile toxin (LT-R192G) induces antibody-secreting cell responses but not protective immunity in gnotobiotic pigs. J Virol 2000; 74:8843 - 53; http://dx.doi.org/10.1128/JVI.74.19.8843-8853.2000; PMID: 10982326
- Lee S, Belitsky BR, Brinker JP, Kerstein KO, Brown DW, Clements JD, Keusch GT, Tzipori S, Sonenshein AL, Herrmann JE. Development of a Bacillus subtilis-based rotavirus vaccine. Clin Vaccine Immunol 2010; 17:1647 - 55; http://dx.doi.org/10.1128/CVI.00135-10; PMID: 20810679
- Zhou H, Guo L, Wang M, Qu J, Zhao Z, Wang J, Hung T. Prime immunization with rotavirus VLP 2/6 followed by boosting with an adenovirus expressing VP6 induces protective immunization against rotavirus in mice. Virol J 2011; 8:3; http://dx.doi.org/10.1186/1743-422X-8-3; PMID: 21205330
- Burns JW, Siadat-Pajouh M, Krishnaney AA, Greenberg HB. Protective effect of rotavirus VP6-specific IgA monoclonal antibodies that lack neutralizing activity. Science 1996; 272:104 - 7; http://dx.doi.org/10.1126/science.272.5258.104; PMID: 8600516
- Schwartz-Cornil I, Benureau Y, Greenberg H, Hendrickson BA, Cohen J. Heterologous protection induced by the inner capsid proteins of rotavirus requires transcytosis of mucosal immunoglobulins. J Virol 2002; 76:8110 - 7; http://dx.doi.org/10.1128/JVI.76.16.8110-8117.2002; PMID: 12134016
- Feng N, Lawton JA, Gilbert J, Kuklin N, Vo P, Prasad BV, Greenberg HB. Inhibition of rotavirus replication by a non-neutralizing, rotavirus VP6-specific IgA mAb. J Clin Invest 2002; 109:1203 - 13; http://dx.doi.org/10.1172/JCI14397; PMID: 11994409
- Corthésy B, Benureau Y, Perrier C, Fourgeux C, Parez N, Greenberg H, Schwartz-Cornil I. Rotavirus anti-VP6 secretory immunoglobulin A contributes to protection via intracellular neutralization but not via immune exclusion. J Virol 2006; 80:10692 - 9; http://dx.doi.org/10.1128/JVI.00927-06; PMID: 16956954
- Aiyegbo MS, Sapparapu G, Spiller BW, Eli IM, Williams DR, Kim R, Lee DE, Liu T, Li S, Woods VL Jr., et al. Human rotavirus VP6-specific antibodies mediate intracellular neutralization by binding to a quaternary structure in the transcriptional pore. PLoS One 2013; 8:e61101; http://dx.doi.org/10.1371/journal.pone.0061101; PMID: 23671563
- Gualtero DF, Guzmán F, Acosta O, Guerrero CA. Amino acid domains 280-297 of VP6 and 531-554 of VP4 are implicated in heat shock cognate protein hsc70-mediated rotavirus infection. Arch Virol 2007; 152:2183 - 96; http://dx.doi.org/10.1007/s00705-007-1055-5; PMID: 17876681
- Garaicoechea L, Olichon A, Marcoppido G, Wigdorovitz A, Mozgovoj M, Saif L, Surrey T, Parreño V. Llama-derived single-chain antibody fragments directed to rotavirus VP6 protein possess broad neutralizing activity in vitro and confer protection against diarrhea in mice. J Virol 2008; 82:9753 - 64; http://dx.doi.org/10.1128/JVI.00436-08; PMID: 18632867
- Aladin F, Einerhand AW, Bouma J, Bezemer S, Hermans P, Wolvers D, Bellamy K, Frenken LG, Gray J, Iturriza-Gómara M. In vitro neutralisation of rotavirus infection by two broadly specific recombinant monovalent llama-derived antibody fragments. PLoS One 2012; 7:e32949; http://dx.doi.org/10.1371/journal.pone.0032949; PMID: 22403728
- Vega CG, Bok M, Vlasova AN, Chattha KS, Gómez-Sebastián S, Nuñez C, Alvarado C, Lasa R, Escribano JM, Garaicoechea LL, et al. Recombinant monovalent llama-derived antibody fragments (VHH) to rotavirus VP6 protect neonatal gnotobiotic piglets against human rotavirus-induced diarrhea. PLoS Pathog 2013; 9:e1003334; http://dx.doi.org/10.1371/journal.ppat.1003334; PMID: 23658521
- Knowlton DR, Spector DM, Ward RL. Development of an improved method for measuring neutralizing antibody to rotavirus. J Virol Methods 1991; 33:127 - 34; http://dx.doi.org/10.1016/0166-0934(91)90013-P; PMID: 1658027
- Ward RL, Kapikian AZ, Goldberg KM, Knowlton DR, Watson MW, Rappaport R. Serum rotavirus neutralizing-antibody titers compared by plaque reduction and enzyme-linked immunosorbent assay-based neutralization assays. J Clin Microbiol 1996; 34:983 - 5; PMID: 8815124
- Ruggeri FM, Johansen K, Basile G, Kraehenbuhl JP, Svensson L. Antirotavirus immunoglobulin A neutralizes virus in vitro after transcytosis through epithelial cells and protects infant mice from diarrhea. J Virol 1998; 72:2708 - 14; PMID: 9525588
- Blazevic V, Lappalainen S, Nurminen K, Huhti L, Vesikari T. Norovirus VLPs and rotavirus VP6 protein as combined vaccine for childhood gastroenteritis. Vaccine 2011; 29:8126 - 33; http://dx.doi.org/10.1016/j.vaccine.2011.08.026; PMID: 21854823
- Vesikari T, Blazevic V, Nurminen K, Huhti L, Lappalainen S, Jokela E. Norovirus capsid and rotavirus VP6 protein for use as combined vaccine. Finnish patent 122520, 15 March 2012.
- Tamminen K, Lappalainen S, Huhti L, Vesikari T, Blazevic V. Trivalent combination vaccine induces broad heterologous immune responses to norovirus and rotavirus in mice. PLoS One 2013; 8:e70409; http://dx.doi.org/10.1371/journal.pone.0070409; PMID: 23922988
- Guerrero RA, Ball JM, Krater SS, Pacheco SE, Clements JD, Estes MK. Recombinant Norwalk virus-like particles administered intranasally to mice induce systemic and mucosal (fecal and vaginal) immune responses. J Virol 2001; 75:9713 - 22; http://dx.doi.org/10.1128/JVI.75.20.9713-9722.2001; PMID: 11559804
- Amuguni JH, Lee S, Kerstein KO, Brown DW, Belitsky BR, Herrmann JE, Keusch GT, Sonenshein AL, Tzipori S. Sublingually administered Bacillus subtilis cells expressing tetanus toxin C fragment induce protective systemic and mucosal antibodies against tetanus toxin in mice. Vaccine 2011; 29:4778 - 84; http://dx.doi.org/10.1016/j.vaccine.2011.04.083; PMID: 21565244
- Lappalainen S, Tamminen K, Vesikari T, Blazevic V. Comparative immunogenicity in mice of rotavirus VP6 tubular structures and virus-like particles. Hum Vaccin Immunother 2013; 9:1991 - 2001; http://dx.doi.org/10.4161/hv.25249; PMID: 23777748
- Angel J, Franco MA, Greenberg HB. Rotavirus immune responses and correlates of protection. Curr Opin Virol 2012; 2:419 - 25; http://dx.doi.org/10.1016/j.coviro.2012.05.003; PMID: 22677178
- Patel M, Glass RI, Jiang B, Santosham M, Lopman B, Parashar U. A systematic review of anti-rotavirus serum IgA antibody titer as a potential correlate of rotavirus vaccine efficacy. J Infect Dis 2013; 208:284 - 94; http://dx.doi.org/10.1093/infdis/jit166; PMID: 23596320
- Blutt SE, Conner ME. The Gastrointestinal Frontier: IgA and Viruses. Front Immunol 2013; 4:402; http://dx.doi.org/10.3389/fimmu.2013.00402; PMID: 24348474
- Cheuvart B, Neuzil KM, Steele AD, Cunliffe N, Madhi SA, Karkada N, Han HH, Vinals C. Association of serum anti-rotavirus immunoglobulin A antibody seropositivity and protection against severe rotavirus gastroenteritis: Analysis of clinical trials of human rotavirus vaccine. Hum Vaccin Immunother 2013; 10:58 - 64; http://dx.doi.org/10.4161/hv.27097; PMID: 24240068
- Mazanec MB, Kaetzel CS, Lamm ME, Fletcher D, Nedrud JG. Intracellular neutralization of virus by immunoglobulin A antibodies. Proc Natl Acad Sci U S A 1992; 89:6901 - 5; http://dx.doi.org/10.1073/pnas.89.15.6901; PMID: 1323121
- Tosser G, Delaunay T, Kohli E, Grosclaude J, Pothier P, Cohen J. Topology of bovine rotavirus (RF strain) VP6 epitopes by real-time biospecific interaction analysis. Virology 1994; 204:8 - 16; http://dx.doi.org/10.1006/viro.1994.1505; PMID: 7522377
- Ward RL, McNeal MM, Sheridan JF. Development of an adult mouse model for studies on protection against rotavirus. J Virol 1990; 64:5070 - 5; PMID: 2168987
- Pastor AR, Rodríguez-Limas WA, Contreras MA, Esquivel E, Esquivel-Guadarrama F, Ramírez OT, Palomares LA. The assembly conformation of rotavirus VP6 determines its protective efficacy against rotavirus challenge in mice. Vaccine 2014; In press http://dx.doi.org/10.1016/j.vaccine.2014.02.018; PMID: 24583002
- McNeal MM, Stone SC, Basu M, Bean JA, Clements JD, Hendrickson BA, Choi AH, Ward RL. Protection against rotavirus shedding after intranasal immunization of mice with a chimeric VP6 protein does not require intestinal IgA. Virology 2006; 346:338 - 47; http://dx.doi.org/10.1016/j.virol.2005.11.016; PMID: 16375942
- Tamminen K, Huhti L, Koho T, Lappalainen S, Hytönen VP, Vesikari T, Blazevic V. A comparison of immunogenicity of norovirus GII-4 virus-like particles and P-particles. Immunology 2012; 135:89 - 99; http://dx.doi.org/10.1111/j.1365-2567.2011.03516.x; PMID: 22044070
- DiStefano DJ, Gould SL, Munshi S, Robinson DK. Titration of human-bovine rotavirus reassortants using a tetrazolium-based colorimetric end-point dilution assay. J Virol Methods 1995; 55:199 - 208; http://dx.doi.org/10.1016/0166-0934(95)00057-2; PMID: 8537458
- Pang XL, Joensuu J, Hoshino Y, Kapikian AZ, Vesikari T. Rotaviruses detected by reverse transcription polymerase chain reaction in acute gastroenteritis during a trial of rhesus-human reassortant rotavirus tetravalent vaccine: implications for vaccine efficacy analysis. J Clin Virol 1999; 13:9 - 16; http://dx.doi.org/10.1016/S1386-6532(98)00013-4; PMID: 10405887
- Ward RL, Bernstein DI, Shukla R, Young EC, Sherwood JR, McNeal MM, Walker MC, Schiff GM. Effects of antibody to rotavirus on protection of adults challenged with a human rotavirus. J Infect Dis 1989; 159:79 - 88; http://dx.doi.org/10.1093/infdis/159.1.79; PMID: 2535868
- Esquivel FR, Lopez S, Guitierrez-X L, Arias C. The internal rotavirus protein VP6 primes for an enhanced neutralizing antibody response. Arch Virol 2000; 145:813 - 25; http://dx.doi.org/10.1007/s007050050674; PMID: 10893159