Abstract
The mucosal immune system displays several adaptations reflecting the exposure to the external environment. The efficient induction of mucosal immune responses also requires specific approaches, such as the use of appropriate administration routes and specific adjuvants and/or delivery systems. In contrast to vaccines delivered via parenteral routes, experimental, and clinical evidences demonstrated that mucosal vaccines can efficiently induce local immune responses to pathogens or tumors located at mucosal sites as well as systemic response. At least in part, such features can be explained by the compartmentalization of mucosal B and T cell populations that play important roles in the modulation of local immune responses. In the present review, we discuss molecular and cellular features of the mucosal immune system as well as novel immunization approaches that may lead to the development of innovative and efficient vaccines targeting pathogens and tumors at different mucosal sites.
Introduction
Mucous membranes comprise an extensive surface area that lines cavities and internal organs directly facing the external environment. Such high exposure makes the mucosa the port of entry of a large number of infectious agents, requiring prompt and effective defense mechanisms. However, mucosal tissues also need to acquire tolerance against non-dangerous inhaled or orally absorbed antigens and maintain a dynamic equilibrium with the microbiota.Citation1 Indeed, the mucosal immune system has evolved tightly controlled surveillance and immunity induction mechanisms. The large number of deaths attributed to mucosal infections in children, approximately 10 million annually,Citation2 reinforces the challenge for the induction of an efficient immune response at mucosal sites. Although several mucosal infections have great epidemiological impact, only few mucosal vaccines against mucosal pathogens (poliovirus, Vibrio cholerae, Salmonella typhi, rotavirus, and influenza) have been commercially approved ().Citation3 This situation may change as some clinical trials are ongoing for mucosally administered vaccines to prevent infections against influenza virus, Bordetella pertussis, Enterotoxigenic Escherichia coli (ETEC), Vibrio cholerae, Shigella sonnei, and norovirusCitation4,Citation5. In addition, cancer vaccines against tumors located at mucosal sites (colon, head and neck, lung, genital tract) have failed to provide clinical benefits when administered by systemic routes.Citation6,Citation7 The increasing understanding of the mucosal immune system and the current mucosal vaccine strategies should lead to the development of innovative and potent vaccines against mucosal pathogens and tumors located at mucosal sites.Citation8,Citation9
Table 1. List of commercially available vaccines administered by the mucosal routes*,**
The Mucosa-Associated Lymphoid Tissue
The mucosal immunological components can be divided into two main parts: organized mucosa-associated lymphoid tissues (MALTs), where antigen-specific immune responses are initiated, and diffuse lamina propria regions, which are the effector sites for antibody production (IgA) and T cell responses.Citation10 MALTs represent a complex immunological network structure, situated along the surfaces of various kinds of mucosal tissues, including the gut-associated lymphoid tissues (GALT) called the Peyer’s Patches (PPs), the nasopharynx- associated lymphoid tissue (NALT), the bronchus associated lymphoid tissue (BALT), the conjunctiva-associated lymphoid tissue (CALT), and the vaginal-associated lymphoid tissue (VALT). The organization of each MALT is similar to that of a lymph node (LN) with B-cell-rich follicles and T-cell-rich interfollicular areas in close contact with dendritic cells (). Some interspecies differences exist in the nature and the regulation of MALT. For example, as opposed to rodents, humans generally do not have NALT anatomically, but they possess oropharyngeal lymphoid tissues, which seem to correspond functionally to NALT.Citation8 In addition, BALT is not constitutively present in all mammalian species, but is induced in response to microbial exposure or other types of pulmonary inflammation.Citation11 These inducible tissues may be more properly referred to as a tertiary or ectopic lymphoid tissue and are composed by organized structures with T- and B-cell areas, high content of endothelial venules (HEVs) in the T-cell zone and an overlying lymphoepithelium.Citation12
Figure 1. Mucosal associated lymphoid tissue (MALT) are organized lymphoid structures present in surface area in contact with environment such as the lung (bronchus-associated lymphoid tissue (BALT), the nose (Nasal-associated lymphoid tissue (NALT) and the gut (Gut-associated lymphoid tissue (GALT). Peyer’s patch present in the GALT are often presented as a model of the MALT organization. It is located in the lamina propria layer of the small intestine and in the ileum in humans. This lymphoid structure between the lumen of the intestine and the mesenteric lymph node is the place of the priming of a mucosal immune response.
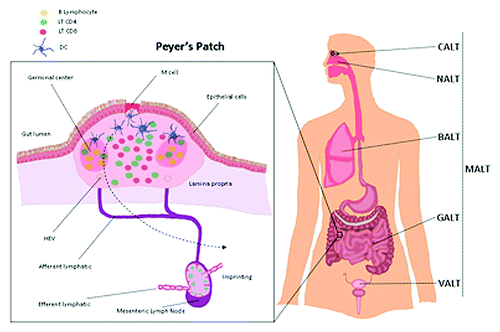
Antigen Sampling and Presentation at Mucosal Surfaces
The epithelial cell layers associated with mucous membranes form physical and immunological barriers that are not impenetrable, but control the cross-talk between the lumen and the lamina propria using multiple antigen sampling strategies.Citation13 The epithelial cells lining the mucous membranes express pattern recognition receptors and antimicrobial effector molecules, which enables them to respond to microorganisms. These mucosal epithelial cells initiate the first steps in the host-pathogen interaction and largely influence the type of immune response elicited by the host.Citation14,Citation15 In intestinal and airway epithelia, whose intercellular spaces are sealed by tight junctions, specialized epithelial microfold cells (M cells) deliver samples of foreign material by transepithelial transport from the lumen to the MALT.Citation16 M cells have reduced microvilli, a thin mucus layer and a pocket-like cell structure that holds dendritic cells (DCs) and/or lymphocytes, allowing an easier contact with pathogens and enhancing the contact with antigens.Citation17
DCs work as the MALT sentinels, moving into the epithelium in close contact with M cells, sampling luminal antigens, and, then, migrating back to local (MALTs) or distant organized lymphoid tissues of draining lymph nodes (DLN). DCs are involved in the induction of immune responses against pathogens, as well as tolerance to commensal microbiota and food.Citation18,Citation19 The tolerogenic functions of intestinal DCs are associated with higher IL-10 secretion, compared with splenic DCsCitation20 and induction of increased IL-4 and IL-10 production by naïve CD4 T cells,Citation21 properties likely associated with their tolerogenic functions. In mice PPs tolerogenic DCs are mainly represented by CD11b+CD8- cells, whereas CD11b-CD8- and CD11b-CD8+ DCs produce IL-12 and prime T cells for IFNγ production.Citation22 Another DC subpopulation described to play a specialized role in mucosal tissues, is represented by CD11b+CD103+ cells, which are highly capable of migrating from the lamina propria to DLN in a CCR7-dependent manner.Citation23 Through this process, CD103+ DCs transport antigens from mucosal sites and present them to CD8+ and CD4+T cells in DLNs, resulting also in the expression of homing molecules on these cells (imprinting).Citation21 Mucosal DCs also express FcRn, a receptor which binds the Fc portion of IgG at acidic pH, and play a major role in antigen cross-presentation leading of potent mucosal CD8+T cell responses.Citation24
Effector immune responses at mucosal tissues
Mucosal antibody response
IgA is found in the serum and in secretions (sIgA). In human serum, the predominant form of IgA is IgA1 of which around 90% is monomeric and 10% is dimeric or polymeric. The IgA2 subclass predominates over the IgA1 subclass in the mucosae. IgA monomer requires the joining chain (J-chain produced by IgA-plasma cells) to form a dimer or a polymer. A particular hallmark of mucosal immunity is the local induction and production of secretory IgA (S-IgA) by activated B cells in MALT germinal centers or lymph nodes. B cell isotype switching for IgA production is mainly stimulated by TGF-β, while retinoic acid, IL-4, IL-10, and IL-6 are important co-factors for differentiation into plasma cells and the enhancement of IgA secretion.Citation25,Citation26 These mediators are abundantly present at most mucosal surfaces, and are produced by DCs, epithelial cells, stromal cells, and mucosal lymphocytes.Citation27-Citation29 MALT post-switched IgA+ B cells disseminate in the blood via the efferent lymphatic vessel and some reach the mucosal effector tissues,Citation30,Citation31 where they differentiate into IgA-producing plasma cells. In mucosal tissue dimeric or polymeric IgA, but not monomeric IgA show a high affinity for the polymeric Ig receptor (pIgR) localized at the basolateral surface of epithelial cells, which actively transport IgA across the mucosal epithelium to the lumen. In the mucosae and not in the blood, dimeric or polymeric IgA also include a secretory component (SC) corresponding to a part of the pIgR.Citation32 The final S-IgA has unique properties, including acid and protease resistance and interactions with mucus and effector leukocytes.Citation26,Citation33 Interestingly, IgA antibodies are unable to fix complement, functioning mainly as a neutralizing antibody, which results in a non-inflammatory immune response that limits the damage at mucosal tissues. IgA antibodies secreted onto the mucosal surfaces are particularly important in preventing infection by inhibiting the adhesion of bacteria, viruses, or other pathogens to epithelial cells.Citation34,Citation35 The IgA complex, including the SC, bind to M cells and may have a role of ‘selection’, excluding pathogenic bacteria or fungi from the epithelial surface through its anchoring within the mucus and favoring biofilm formation of non-pathogenic bacteria in the space in close contact with epithelial cells.Citation36 Other immunoglobulin isotypes may also play a role in mucosal tissues and MALT B cells may switch to different immunoglobulin isotypes according to the anatomical site and the specific inducing conditions. For example in some species, IgG-secreting cells predominate over IgA-secreting cells in BALT.Citation37
Cellular immune responses at mucosal sites
T cells receive antigenic activation and co-stimulation by antigen-presenting cells (APCs) that support their clonal expansion, as well as the cytokine cues that dictate their differentiation and homing to peripheral tissues.Citation38,Citation39,Citation38,Citation40 Activated and memory T cells downregulate molecules used for LN entry and upregulate molecules involved in migration to non-lymphoid tissues, such as the skin and the intestinal mucosa.Citation40 This memory T cell population, called tissue resident memory cells (TRM), has been shown to stably reside in the peripheral tissues and not to enter the circulation for a prolonged period of time.Citation40 The presence of TRM cells has been described in many mucosal tissues including skin,Citation41-Citation43 lungs,Citation44,Citation45 salivary glands,Citation46 and intestinal epithelium.Citation47 TRM rapidly acquire effector functions upon secondary antigenic stimulation and are highly protective against subsequent local infection.Citation48,Citation49 The presence of TRM in the mouse lungs has been described as a better surrogate marker, than memory specific T cells in the blood, for reinfection protection in different pre-clinical studies in mice.Citation50 In non-human primates, the presence of TRM against SIV was essential to control the viral load.Citation51 The DC-mediated imprinting of specific homing molecules on T cell surface is influenced by the LN microenvironment and, particularly, by the local production of TGF-β ().Citation38 Gut and skin tissue-specific homing molecules expression on T cells also requires vitamin A/Retinoic Acid and vitamin D-derived metabolites, respectively.Citation52,Citation53 Homing markers expression and their regulation is still not well defined for all mucosal tissues, but it is well established that T cell expression of α4β7, which binds to intestinal MADCAM-1 on endothelial cells, and CCR9, attracted by intestinally produced CCL25, enable T cells entry into the small intestine.Citation54,Citation55 Furthermore, expression of CCR10 and P- and E-selectin ligands, induces T-cell skin homing mediated by cutaneous CCL27 and P- and E-selectins ().Citation56-Citation58
Table 2. Tissue-resident CD8+ T cells key homing markers involved in migration to different sites
Compartmentalization and immunity at distant mucosal sites
Mucosal invasion by pathogens prime immune responses at both mucosal and systemic compartments. Similarly, several vaccine studies showed that mucosal immunization has also been efficient in generating immune responses detectable at distant mucosal tissues and in the blood.Citation58 In contrast, immunization via parenteral administration routes usually fails to induce mucosal immunity or induce weaker responses than those detected after mucosal immunization.Citation58-Citation60 Mucosal immune stimulation after pathogen invasion or vaccination, besides being capable of inducing systemic immunity, activates B and T cells that may migrate to peripheral environments different from the one where stimulation was initiated. The possible interconnection between immunity at different mucosal surfaces, raised the concept of a “common mucosal immune system.” In fact, mucosally primed T and B cells display preferential migration patterns to specific mucosal surfaces or tissues, that are shared among mucosal sites.Citation35,Citation61 For example, IgA+B cells in the MALT usually express CCR10 on their surfaces, which favors migration to CCL28 expressed by epithelial cells in the gut, lung, breast, vaginal, and salivary glands.Citation62,Citation63 Interestingly, the female sex hormone estradiol regulates the CCL28 expression.Citation64 Intranasal (i.n) or sublingual immunization induced specific IgA secretion and activation of CD8+T cells in the genital tract and in some cases in the intestine.Citation65,Citation66 Indeed, DCs activated at a particular immunization site, recirculate to distant lymphoid organs and induce distant T cell priming, explaining the genital immune response observed after i.n immunization with the B subunit of cholera toxin (CTB) coupled to OVA.Citation67 In clinical settings, however, an intravaginal boost was required to attract T cells to the genital tract after i.n immunization with the cholera toxin coupled to OVA.Citation68 Additionally, oral, nasal or intravaginal immunization with CTB were able to induce comparable secreted IgA levels in the vaginal tract but no cellular immune responses.Citation69 In fact, inducing mucosal immunity at different anatomical sites after local priming may vary according to the specific mucosal tissue, type of immune response or vaccination approach.
Potential Role of Microbiota in the Activity of Mucosal Vaccines
Mucosal tissues are colonized by a vast number of non-pathogenic microbes that exert a significant influence on mucosal immune regulation and, therefore, can be a factor in determining the effectiveness of mucosal vaccines. For example, the manipulation of the intestinal flora was shown to play a large role in T cell regulation.Citation70,Citation71 Oral vaccines against rotavirus, V. cholerae and Escherichia coli (ETEC) have been shown to be less effective in developing countries. Difference in the nutritional status, but also in gut microflora, as well as ongoing persistent infections with helminths and parasites have been raised as determinant hypothesis to explain this differential efficiency.Citation72 For the future, we could expect the development of programs to deeply analyze and correlate the individual and geographical characteristics of the microbiota and the efficacy of mucosal vaccines.Citation27
Strategies to Induce Mucosal Immune Responses
Immunization routes
DC priming at one specific mucosal site determines the subsequent homing of T cell to specific mucosal sites.Citation73 Subsequent studies confirmed that this phenomenon applies to different mucosal administration routes such as the intranasal, intratracheal, oral, intrarectal, sublingual, intravaginal, and intraocular routes. Various groups showed that the mucosal delivery, especially by the oral route, of mucosal non-pathogenic live recombinant bacteria (Listeria monocytogenes, Salmonella typhimurium, Bacillus subtilis, lactic acid bacteria…) expressing antigen is efficient to protect against mucosal pathogens.Citation74-Citation78 The intranasal immunization route has been intensively studied and shown to induce robust humoral and cellular immune responses at the lung mucosa against virus and bacterial infection.Citation79-Citation82 In addition, the i.n. immunization route favors Th17 polarization,Citation83 known to be involved in the generation of protective immunity against funghi and bacteria located at mucosal sites.Citation84 A comparative clinical trial performed with children immunized with a live attenuated influenza vaccine (Flumist) showed that administration by the i.n route was more efficient, than the same formulation delivered intramuscularly in the mounting of protective immunity.Citation85 These results led to the approval of the vaccine administered by the i.n. route by the US Food and Drug Administration (FDA), a breakthrough for mucosal vaccines in which the oral administration route had long been the only alternative for vaccination in humans.
Other mucosal immunization routes have also raised interest and generated encouraging results. In mice, the sublingual vaccination proved to induce strong mucosal pulmonary and genital immune responses associated with protection against influenza, RSV or SARS lethal virus challenges and genital infections.Citation86-Citation88 However, in preclinical models, the sublingual route of immunization appeared to be less powerful than the i.n route in eliciting protective mucosal immune responses.Citation89 In humans, however, an HPV vaccine administered by the sublingual route induced less anti-HPV antibodies in the cervix, than the i.m. route.Citation90,Citation91
The intravaginal route may be less effective in the induction of mucosal immune response. Intravaginal immunization with subunit vaccines elicit weak or no mucosal immune response both in rodents and in humans.Citation92,Citation93 In most cases, only live vectors have been shown to elicit mucosal immune response by this immunization route.Citation88,Citation94 Intravaginal immunization with live HPV pseudovirions particles or systemic immunization combined with intravaginal administration of TLR agonist are promising approaches against sexually transmitted pathogens, because of its ability to induce local CD8+ TCitation88,Citation95 and CD4+T cell responses, as well as antibody responses. However, the efficacy of this route may depend on the hormonal cycle of the women, as it has been shown that the estradiol inhibits CD8+T cell priming.Citation96 Proteins or subunit vaccines could be administered by the intrarectal route but it would require the association of an adjuvant to elicit mucosal immune response and protection against infectious challenge.Citation97,Citation98 Nevertheless, intrarectal immunization with subunit vaccines also seem to be less efficient than those comprising recombinant virus-based vaccines.Citation99,Citation100 Intrarectal immunization with a peptide prime and recombinant vaccinia boost regimen activated high avidity CD8+ CTLs in the gut mucosa, that correlated with lower SIV virus dissemination in macaques.Citation51,Citation101 The administration of an anti-HSV vaccine at the ocular mucosa induced both antibody (systemic and mucosal) and cellular immune responses leading to protective immunity to ocular virus challenge in mice.Citation101 In general, mucosal routes of immunizations are capable to induce mucosal and systemic immune responses, while systemic immunization induce systemic and, in a clearly lesser extent, mucosal immune responses.
Mucosal adjuvants
As with vaccines administered via parenteral routes, immune responses induced by vaccines delivered via mucosal routes can be drastically enhanced with the use of adjuvants. In preclinical models, the most largely employed adjuvants to induce mucosal immune responses have been non-toxic derivatives of cholera toxin (CT) and Escherichia coli heat labile enterotoxin (LT).Citation102,Citation103 Although devoid of their enterotoxicity and capable to efficiently increase both antibody and cellular immune responses, LT-derivatives proved to be toxic to humans after i.n. delivery due to the nerve tissue tropism leading to accumulation in the olfactory nerve and the bulb and inducing transient facial paralysis.Citation104 Some groups attempt to preserve the adjuvant activity of LT while decreasing its toxicity.Citation105,Citation106 Toll-like receptor (TLR) ligands have been described to enhance mucosal immunity when co-administered with antigens by mucosal routes. Recently, US FDA has approved the use of a TLR4 agonist, the Monophosphoryl lipid A (MPL), combined with alum (AS04) for intramuscular immunization of the Cervarix prophylactic vaccine against HPV infections, which promotes Th1-biased response.Citation107,Citation108 AS01, an adjuvant system containing liposomes, MPL and saponin, increased mucosal immunity in non-human primates.Citation109 Accordingly, i.n. vaccination with TLR4 agonists resulted in robust immune responses to both the carrier protein and bacterial polysaccharide components of the Hemophilus influenza type B virus in murine models.Citation110 Micellar and emulsion formulations of a synthetic TLR4 agonist, Glucopyranosyl Lipid Adjuvant (GLA) administered by the i.n route induced Th17-biased systemic and mucosal antibody responses.Citation111 The TLR9 ligand CpG, induce Th1-biased immune responses after sublingual or i.n administration.Citation112,Citation113 In a preclinical model, CpG administration was possibly linked to enhanced hepatitis pathogenesis induced by concanavalin-A production, but the CpG was not administered by a mucosal route in this case.Citation114
Another TLR ligand, the TLR3-specific double-stranded RNA oligonucleotide has been shown to modulate local immune responses after i.n administration.Citation115 In addition, poly(I:C) proved to be an effective adjuvant for an i.n. delivered influenza vaccine.Citation116 The TLR5 ligand, flagellin, combined with various antigens has been shown to enhance IgA response after i.n administration and protect against various toxins and pathogens challenge in preclinical models.Citation117-Citation119 Indeed, Salmonella flagellins has been shown to be flexible adjuvants leading to activation of antibody and T cell responses based on the administration via mucosal and parenteral routes either as recombinant protein genetically fused with the target antigen or admixed with the soluble or particulate antigens.Citation120 Recently, it has been shown that the i.n delivery of virus like particles (VLPs) in combination with TLR7 or TLR9 agonists led to a significantly better dose-sparing effects than TLR3, TLR5 or TLR8 agonists for the induction of specific and functional antibody responses in the respiratory, gastrointestinal, and reproductive tracts.Citation121
Chitosan microparticles and cationic chitosan derivatives are obtained from natural crab shells, composed of chitin derivatives, and are potent activators of macrophages and NK cells. Their administration by the i.n route combined with antigen induced high levels of IgA in the serum of mice and non-human primates.Citation122 Chitosan seems to favor a Th2 biased immune response and its safety has already been validated in mice and humans.Citation122,Citation123 Recent findings have evidenced α-Galactosylceramid (α-GalCer) as a promising mucosal adjuvant. This CD1d ligand activates natural killer T (NKT) invariant cells, promoting DC maturation and cross-presentation.Citation124,Citation125 When administered via the i.n route in combination with various antigens, α-GalCer induced mucosal antibody responses, as well as CD8+T cells responses,Citation126,Citation127 devoid of measurable toxicity and without redirecting antigen to the nervous system, as shown for cholera toxin.Citation128 Administration of α-GalCer by the i.n route, but not by the systemic route, allowed repeated stimulation of NKT in the lung.Citation129 This adjuvant has also been used to sensitize DC to activates NKT cells in patients presenting recurrent head and neck carcinoma, without described associated toxicity.Citation130 Another class of promising mucosal adjuvants is represented by some already clinically used antibiotics such as polymyxin B (PMB) and colistin (CL). Intranasal immunization with PMB or CL with ovalbumin (OVA), increased OVA-specific antibody responses in a dose-dependent manner both at mucosal and the systemic compartments without detectable inflammatory damages.Citation131 Overall, novel mucosal adjuvants are particularly promising in preclinical models and, at least some of them (such as TLR3, TLR4, TLR5, TLR9 agonists and αGalCer) are also strong inducers of mucosal cellular immune responses.
Mucosal Homing Signals Delivered by Adjuvants or Immunomodulators
Several studies suggest that the local delivery of a mucosal stimulus or a peripheral mucosal imprinting of T cells favor their homing to mucosal sites. Shin and colleagues were the first to show that parenteral vaccination, which elicited systemic T-cell responses (prime), followed by recruitment of activated T cells through topical chemokine application in the genital tract (pull), reduced the spread of HSV-2 infection to the sensory neurons and prevented the development of clinical disease.Citation132 Mucosal signaling also appear necessary to recruit anti-tumor T cells after systemic vaccination for the generation of anti-tumor protective immunity at mucosal sites. Indeed, subcutaneous (s.c) immunization against HPV-associated tumors had no significant effect in the regression of genital tumors expressing the E6 and E7 proteins, while the same vaccination associated with intravaginal administration of CpG led to the regression of more than 75% of the genital tumors in mice.Citation95 Other studies showed that s.c immunization with protein-based vaccine combined with all-trans retinoic acid, induced robust upregulation of gut homing receptors, resulting in T and B cells migration to the gut and protecting mice from cholera toxin–induced diarrhea.Citation133
Targeting Mucosal M Cells and Dendritic Cells
To improve the efficacy of mucosal vaccines, other strategies aim to deliver antigens to M cells or mucosal DCs. M cells are one of the intestinal barriers for the efficient delivery of vaccines to mucosal tissues, thus, targeting M cells improves the transcytosis of antigen to the MALT, such as intestinal PPs. A peptide sequence, identified as having M cells directing properties, when coupled to various vaccine compounds, increased humoral immune response.Citation134,Citation135 Some bacterium species, such as Lactobacillus acidophilus, have been engineered to express antigen fused to a DC targeting peptide.Citation136 After oral delivery, this vaccine strategy improves mucosal immunity and protects against a lethal anthrax challenge.Citation136 Similar genetically modified spores of Bacillus subtilis engineered to express adhesins capable to recognize receptors expressed on gut epithelial cells and M cells enhance the induction of mucosal and systemic immune responses to an encoded antigen target.Citation137 Our group has developed a vector, composed of the non-toxic B subunit of Shiga toxin which target DCs and favor antigen cross-presentation.Citation138-Citation140 We showed that i.n delivery of antigen coupled to STxB target mediastinal LNs DC cells and induced potent mucosal CD8+T cells responses and antigen specific IgA at mucosal sites.Citation141 In contrast to other toxin-derived delivery vehicules, we did not observe toxicity after StxB-based vaccines intranasal administration in mice during a 6 mo follow up (unpublished results). This extends the good safety profile reported by our group and others when STxB-based vaccine were administered by systemic routes.Citation142-Citation145
Particulate Delivery Systems
Vaccines administered by mucosal routes face physical, chemical, and microbiota-imposed constraints, augmenting the risk of antigen degradation. Therefore, vaccine entrapment in non-viral particules can protect the antigens from degradation or denaturation, enhance their sustained release and allow the co-delivery of antigens and adjuvants. In addition, non-pathogen based vehicules elicit a less pronounced anti-vector immunity which allows repetitive immunization. PLA (poly(lactic acid) or PLGA (poly(lactic-co-glycolic acid) nanoparticles are interesting protein carriers that offer antigen protection, increased penetration across mucosal surfaces and controlled release of encapsulated antigen.Citation13,Citation146 In humans, the oral delivery of PLG-encapsulated CS6 antigen from E. coli induced mucosal IgA responses, but complementary studies are required to assess its potency compared with free.Citation147 In preclinical models, other modified lipid-based delivery systems such as liposomes, ISCOMS, virosomes, proteosomes have shown encouraging results in mucosal vaccination settings.Citation13,Citation148 In healthy volunteers, the intranasal administration of a proteosome-based influenza vaccine seemed to be efficacious and well tolerated.Citation149
Lastly, β-glucans composed of carbohydrate polymers found in the cell walls of fungi, yeast, plants, and bacteria, which bind to dectin-1 and CR2, have demonstrated intrinsic adjuvant activity for the enhancement of humoral and cellular.Citation150 β-glucan particles also act as a delivery platform for mucosal antigens. Oral administration of ovalbumin-loaded glucan microparticulates increased the levels of OVA-specific IgA, and secretory IgA in the intestinal fluids.Citation151
Relevance of Inducing Mucosal Immunity against Pathogens
The mucosal immunity acquired by natural influenza infection, primarily due to the production of S-IgA in the respiratory tract, is more effective and cross-protective against subsequent viral infection than the systemic immunity induced by parenteral vaccines in humans and mice.Citation152 In agreement with this observation, many comparative studies of mucosal (i.n, rectal, oral) and parenteral (i.m. or s.c) administration routes with different vaccines have demonstrated the superior efficacy of mucosal routes in obtaining mucosal immune responses at local or even remote sites.Citation153 In these studies, the mucosal route of immunization led to higher protection levels against mucosal pathogens, such as herpes virus, influenza virus, and Mycobacterium tuberculosis.Citation154-Citation156 In humans, the oral vaccine against poliomyelitis, based in attenuated virus strains, induces the production of secretory IgA in intestinal mucosa, preventing the infection of enterocytes and virus spreading. Instead, the inactivated version of the vaccine administered via a parenteral route protects against neurological forms of the disease, but does not affect intestinal virus replication.Citation30 Nevertheless, other studies have shown that parenteral vaccination may induce mucosal immunity, being effective against some mucosal infections.Citation157 In most cases, these studies have been performed with live viral vectors, which when administered by the systemic routes, can diffuse to mucosal sites. Nonetheless, a direct comparison of the relative effectiveness of mucosal and parenteral administration routes has not been conducted. Moreover, it is necessary to differentiate vaccines designed to induce the production of antibodies from those developed for higher activation of CD8+T-cell immunity.Citation158 Indeed, antibodies induced by parenteral vaccines may be found at mucosal sites by passive transudation of circulating antibodies. This observation explains the effectiveness of certain anti-influenza or anti-HPV vaccines that are administered by parenteral routes and protect against respiratory and genital infections. Moreover, it suggests a possible greater constraint in the phenomena of recirculation of T cells than of antibodies.
Mucosal Cancer Vaccines
Various cancer vaccines have been developed against mucosal tumors, including lung cancer.Citation159 The vaccines Mage-A3 and Stimuvax have recently been tested in phase II and III clinical trials, respectively, but failed to demonstrate a clinical benefit in the treatment of non-small cell lung cancer.Citation160,Citation161 Our group has monitored the effects of a lung cancer vaccine based on the use of a recombinant vaccinia virus encoding the Muc 1 antigen expressed by adenocarcinomas of the lung. Although activation of anti-Muc-1 CD8+ T cells were detected in the blood after vaccination, the clinical response was not significant.Citation159,Citation162 One hypothesis relies on the necessity to elicit anti-tumor T cells at the tumor mucosal sites, rather than only in the peripheral blood. Since all these lung cancer vaccines were administered by the i.m. route, we tested in murine models if mucosal immunization would impact the efficacy of cancer vaccines against tumors located in the lung or in the tongue. In the first experiments, using an STxB-based vaccine, we demonstrated that the i.n route of vaccination was more efficient to induce mucosal immune responses in the mediastinal and cervical lymph node, as well as in the broncho-alveolar lavage, than i.m. immunization.Citation141 In line with these results, we observed that the i.n route of immunization was more efficient to inhibit the growth of head and neck or lung cancer, than the i.m. or s.c route. The i.n (mucosal) immunization also led to a more robust tumor infiltration by anti-tumor CD8+ T, cells than the i.m. route. Finally, the i.n administration of the cancer vaccine specifically stimulated the expression of the mucosal integrins CD49a and CD103 on CD8+ T cells, which was not observed following systemic immunization. Blockade of CD49a decreased both CD8+ T-cell tumor infiltration and the therapeutic efficacy of the vaccine. A link was thus established between the route of vaccination and the imprinting of a mucosal homing program on vaccine induced CD8+ T cells. Interestingly, we showed that lung DCs, but not the spleen DCs were able to induce the CD49a expression on the surface of CD8+ T cells.Citation141 A similar pattern of T cells homing molecules was also reported after intrapulmonary vaccination.Citation163 This imprinting ability of lung DCs was confirmed in humans, as it was shown that CD1c lung DCs co-cultured with CD8+T cells promote upregulation of CD103 and CD49a on these cells.Citation164
Other studies support the role of mucosal immunity in protecting against mucosal tumors. The oral administration of antigen combined with cholera toxin generated CD8+ T cell responses capable of controlling the growth of gastric tumors expressing ovalbumin, whereas the same vaccine formulation did not reproduce the anti-tumor effect when administered subcutaneously.Citation165 The adoptive transfer of anti-tumor CD8+ T cells induced by s.c. immunization could protect against the development of subcutaneous tumors but not gastric tumors.Citation166 Similarly, the immunization of mice with DCs by the s.c. route allowed the control of subcutaneous tumors, but not lung metastases.Citation167 Also, intrarectal vaccination with the CEA antigen vectorized in a recombinant vaccinia virus enhanced mucosal CEA-specific IgA antibody titers and cytotoxic CD8+T cells activation, and more importantly, the prevention of the progression of spontaneous colorectal cancer.Citation168 Other studies showed that the immunization route should be tailored to each mucosal tumor site. As an example, both s.c. and intravaginal, but not i.n vaccination, induced high numbers of anti-tumor CD8+T-cells in the bladder, as well as bladder tumor regression.Citation169 In contrast, studies conducted by the Nardelli-Haefliger’s group showed that parenteral immunization was more effective than mucosal immunization to control the growth of intrauterine tumor.Citation170 In this model, it is possible that other effectors, such as antibodies, play a role in the tumor rejection, as the phenomenon of compartmentalization is less evident in humoral responses. Furthermore, the intravaginal immunization route is generally less effective in inducing effector T cell responses, which can be related to the low presence of lymphoid structures in the vagina, since the majority of structures are diffuse clusters of immune cells, and also to the hormonal dependence of this route. Nevertheless, the induction of mucosal CD8+T cell response after intravaginal vaccination has been previously reported.Citation92 In humans, the i.m. administration of long peptides derived from the E6 and E7 HPV proteins led to the regression of high grade vulvar dysplasia. However, as no control groups were included, it is difficult to conclude the real efficacy of this vaccine.Citation171 Except for this last study, no cancer vaccine administered by the systemic route showed any clinical benefit in controlling genital tumors. In general, these examples show that immunizations via mucosal routes are usually more effective than immunizations performed by parenteral routes in the development of protective immunity to mucosal tumors. In humans, some positive correlations have been reported between the CD8+T cell infiltration and the prognosis of some mucosal tumors (colorectal cancer, lung tumors),Citation159,Citation172 supporting the role of CD8+T cells in tumor growth control. However, human cancer vaccines targeting tumors at mucosal sites have not sought to evaluate the usefulness of the mucosal immunization routes up to now. Of course other parameters, such as the difference in the microenvironment of mucosal and non-mucosal tumors and the possibility of tolerance against self-mucosal tumor antigens, have also to be considered for the success of cancer vaccines.Citation144,Citation171,Citation173,Citation174
Conclusion
Prophylactic vaccines against mucosal pathogens (influenza, rotavirus, HPV..) require the presence of antibodies at the mucosal pathogen entry site, either directly induced by the mucosal route of immunization, or after transudation of serum antibodies elicited by the conventional routes of immunization.Citation175 In most prophylactic vaccines, the induction of antibody leads to a good and durable protection against pathogens. Of note, in rare disease models, the induction of humoral immunity or a Th2 polarization may not be beneficial.Citation176,Citation177 For chronic mucosal infection and cancer, cell mediated immunity, especially based in CD8+T cell activation, seems to be mandatory for the success of a vaccine. In contrast to the antibody induction, the level of T cell responses compartmentalization may explain the importance to elicit them directly at the tumor or chronic infection mucosal site. Mucosal vaccine strategies aiming to direct the immune response to the site of pathogen invasion or to the anatomic site of tumor location should thus maximize the efficiency of the immune responses against pathogens and tumors. Various approaches and tools, such as immunization routes and incorporation of adjuvant and/or delivery systems, are already available for the design of optimal mucosal anti-infectious or anti-cancer vaccines. Considering the infectious diseases, these systems have been broadly exploited, but will be extended in the future. Since all therapeutic cancer vaccine trials failed to provide clinical benefit, the development of mucosal cancer vaccine has to be evaluated to strengthen their efficacy.
Disclosure of Potential Conflicts of Interest
No potential conflicts of interest were disclosed.
Acknowledgments
This work was supported by grants from Fondation ARC pour la recherche contre le cancer, Labex immuno-oncology, Canceropole-région Ile-de-France, Agence Nationale de la Recherche (ANR), Site intégré de recherche intégré en cancérologie (SIRIC-Carpem), Institut National contre le Cancer (INCA), Labex d’immuno-oncologie, Ligue contre le cancer, and Fundação de Amparo a Pesquisa do Estado de São Paulo (FAPESP).
References
- Sato S, Kiyono H. The mucosal immune system of the respiratory tract. Current opinion in virology 2012; 2:225-32.
- Brandtzaeg P. Mucosal immunity: induction, dissemination, and effector functions. Scand J Immunol 2009; 70:505 - 15; http://dx.doi.org/10.1111/j.1365-3083.2009.02319.x; PMID: 19906191
- Takahashi I, Nochi T, Yuki Y, Kiyono H. New horizon of mucosal immunity and vaccines. Curr Opin Immunol 2009; 21:352 - 8; http://dx.doi.org/10.1016/j.coi.2009.04.002; PMID: 19493665
- Atmar RL, Bernstein DI, Harro CD, Al-Ibrahim MS, Chen WH, Ferreira J, Estes MK, Graham DY, Opekun AR, Richardson C, et al. Norovirus vaccine against experimental human Norwalk Virus illness. N Engl J Med 2011; 365:2178 - 87; http://dx.doi.org/10.1056/NEJMoa1101245; PMID: 22150036
- http://www.clinicaltrials.gov/ct.
- Nardelli-Haefliger D, Dudda JC, Romero P. Vaccination route matters for mucosal tumors. Science translational medicine 2013; 5:172fs4.
- Badoual C, Sandoval F, Pere H, Hans S, Gey A, Merillon N, Van Ryswick C, Quintin-Colonna F, Bruneval P, Brasnu D, et al. Better understanding tumor-host interaction in head and neck cancer to improve the design and development of immunotherapeutic strategies. Head Neck 2010; 32:946 - 58; PMID: 20191626
- Kiyono H, Fukuyama S. NALT- versus Peyer’s-patch-mediated mucosal immunity. Nat Rev Immunol 2004; 4:699 - 710; http://dx.doi.org/10.1038/nri1439; PMID: 15343369
- Nizard M, Sandoval F, Badoual C, Pere H, Terme M, Hans S, Benhamouda N, Granier C, Brasnu D, Tartour E. Immunotherapy of HPV-associated head and neck cancer: Critical parameters. Oncoimmunology 2013; 2:e24534; http://dx.doi.org/10.4161/onci.24534; PMID: 23894716
- Cesta MF. Normal structure, function, and histology of mucosa-associated lymphoid tissue. Toxicol Pathol 2006; 34:599 - 608; http://dx.doi.org/10.1080/01926230600865531; PMID: 17067945
- Tschernig T, Pabst R. Bronchus-associated lymphoid tissue (BALT) is not present in the normal adult lung but in different diseases. Pathobiology 2000; 68:1 - 8; http://dx.doi.org/10.1159/000028109; PMID: 10859525
- Dieu-Nosjean MC, Antoine M, Danel C, Heudes D, Wislez M, Poulot V, Rabbe N, Laurans L, Tartour E, de Chaisemartin L, et al. Long-term survival for patients with non-small-cell lung cancer with intratumoral lymphoid structures. Journal of clinical oncology: official journal of the American Society of Clinical Oncology 2008; 26:4410-7.
- Pavot V, Rochereau N, Genin C, Verrier B, Paul S. New insights in mucosal vaccine development. Vaccine 2012; 30:142 - 54; http://dx.doi.org/10.1016/j.vaccine.2011.11.003; PMID: 22085556
- Abreu MT, Fukata M, Arditi M. TLR signaling in the gut in health and disease. J Immunol 2005; 174:4453 - 60; http://dx.doi.org/10.4049/jimmunol.174.8.4453; PMID: 15814663
- Fritz JH, Le Bourhis L, Magalhaes JG, Philpott DJ. Innate immune recognition at the epithelial barrier drives adaptive immunity: APCs take the back seat. Trends Immunol 2008; 29:41 - 9; http://dx.doi.org/10.1016/j.it.2007.10.002; PMID: 18054284
- Kraehenbuhl JP, Neutra MR. Epithelial M cells: differentiation and function. Annu Rev Cell Dev Biol 2000; 16:301 - 32; http://dx.doi.org/10.1146/annurev.cellbio.16.1.301; PMID: 11031239
- Siebers A, Finlay BB. M cells and the pathogenesis of mucosal and systemic infections. Trends Microbiol 1996; 4:22 - 9; http://dx.doi.org/10.1016/0966-842X(96)81501-0; PMID: 8824791
- Contractor N, Louten J, Kim L, Biron CA, Kelsall BL. Cutting edge: Peyer’s patch plasmacytoid dendritic cells (pDCs) produce low levels of type I interferons: possible role for IL-10, TGFbeta, and prostaglandin E2 in conditioning a unique mucosal pDC phenotype. J Immunol 2007; 179:2690 - 4; http://dx.doi.org/10.4049/jimmunol.179.5.2690; PMID: 17709480
- Salazar-Gonzalez RM, Niess JH, Zammit DJ, Ravindran R, Srinivasan A, Maxwell JR, Stoklasek T, Yadav R, Williams IR, Gu X, et al. CCR6-mediated dendritic cell activation of pathogen-specific T cells in Peyer’s patches. Immunity 2006; 24:623 - 32; http://dx.doi.org/10.1016/j.immuni.2006.02.015; PMID: 16713979
- Iwasaki A, Kelsall BL. Unique functions of CD11b+, CD8 alpha+, and double-negative Peyer’s patch dendritic cells. J Immunol 2001; 166:4884 - 90; http://dx.doi.org/10.4049/jimmunol.166.8.4884; PMID: 11290765
- Johansson-Lindbom B, Svensson M, Wurbel MA, Malissen B, Márquez G, Agace W. Selective generation of gut tropic T cells in gut-associated lymphoid tissue (GALT): requirement for GALT dendritic cells and adjuvant. J Exp Med 2003; 198:963 - 9; http://dx.doi.org/10.1084/jem.20031244; PMID: 12963696
- Jaensson E, Uronen-Hansson H, Pabst O, Eksteen B, Tian J, Coombes JL, Berg PL, Davidsson T, Powrie F, Johansson-Lindbom B, et al. Small intestinal CD103+ dendritic cells display unique functional properties that are conserved between mice and humans. J Exp Med 2008; 205:2139 - 49; http://dx.doi.org/10.1084/jem.20080414; PMID: 18710932
- Svensson M, Johansson-Lindbom B, Zapata F, Jaensson E, Austenaa LM, Blomhoff R, Agace WW. Retinoic acid receptor signaling levels and antigen dose regulate gut homing receptor expression on CD8+ T cells. Mucosal Immunol 2008; 1:38 - 48; http://dx.doi.org/10.1038/mi.2007.4; PMID: 19079159
- Baker K, Rath T, Flak MB, Arthur JC, Chen Z, Glickman JN, Zlobec I, Karamitopoulou E, Stachler MD, Odze RD, et al. Neonatal Fc receptor expression in dendritic cells mediates protective immunity against colorectal cancer. Immunity 2013; 39:1095 - 107; http://dx.doi.org/10.1016/j.immuni.2013.11.003; PMID: 24290911
- Cerutti A. The regulation of IgA class switching. Nat Rev Immunol 2008; 8:421 - 34; http://dx.doi.org/10.1038/nri2322; PMID: 18483500
- Strugnell RA, Wijburg OL. The role of secretory antibodies in infection immunity. Nat Rev Microbiol 2010; 8:656 - 67; http://dx.doi.org/10.1038/nrmicro2384; PMID: 20694027
- Pasetti MF, Simon JK, Sztein MB, Levine MM. Immunology of gut mucosal vaccines. Immunol Rev 2011; 239:125 - 48; http://dx.doi.org/10.1111/j.1600-065X.2010.00970.x; PMID: 21198669
- Hammerschmidt SI, Ahrendt M, Bode U, Wahl B, Kremmer E, Förster R, Pabst O. Stromal mesenteric lymph node cells are essential for the generation of gut-homing T cells in vivo. J Exp Med 2008; 205:2483 - 90; http://dx.doi.org/10.1084/jem.20080039; PMID: 18852290
- Mueller SN, Germain RN. Stromal cell contributions to the homeostasis and functionality of the immune system. Nat Rev Immunol 2009; 9:618 - 29; PMID: 19644499
- Mora JR, Iwata M, Eksteen B, Song SY, Junt T, Senman B, Otipoby KL, Yokota A, Takeuchi H, Ricciardi-Castagnoli P, et al. Generation of gut-homing IgA-secreting B cells by intestinal dendritic cells. Science 2006; 314:1157 - 60; http://dx.doi.org/10.1126/science.1132742; PMID: 17110582
- Gohda M, Kunisawa J, Miura F, Kagiyama Y, Kurashima Y, Higuchi M, Ishikawa I, Ogahara I, Kiyono H. Sphingosine 1-phosphate regulates the egress of IgA plasmablasts from Peyer’s patches for intestinal IgA responses. J Immunol 2008; 180:5335 - 43; http://dx.doi.org/10.4049/jimmunol.180.8.5335; PMID: 18390715
- Kaetzel CS. The polymeric immunoglobulin receptor: bridging innate and adaptive immune responses at mucosal surfaces. Immunol Rev 2005; 206:83 - 99; http://dx.doi.org/10.1111/j.0105-2896.2005.00278.x; PMID: 16048543
- McGuckin MA, Lindén SK, Sutton P, Florin TH. Mucin dynamics and enteric pathogens. Nat Rev Microbiol 2011; 9:265 - 78; http://dx.doi.org/10.1038/nrmicro2538; PMID: 21407243
- Kunisawa J, Nochi T, Kiyono H. Immunological commonalities and distinctions between airway and digestive immunity. Trends Immunol 2008; 29:505 - 13; http://dx.doi.org/10.1016/j.it.2008.07.008; PMID: 18835748
- Meeusen EN. Exploiting mucosal surfaces for the development of mucosal vaccines. Vaccine 2011; 29:8506 - 11; http://dx.doi.org/10.1016/j.vaccine.2011.09.010; PMID: 21945494
- Mantis NJ, Rol N, Corthésy B. Secretory IgA’s complex roles in immunity and mucosal homeostasis in the gut. Mucosal Immunol 2011; 4:603 - 11; http://dx.doi.org/10.1038/mi.2011.41; PMID: 21975936
- Sminia T, van der Brugge-Gamelkoorn GJ, Jeurissen SH. Structure and function of bronchus-associated lymphoid tissue (BALT). Crit Rev Immunol 1989; 9:119 - 50; PMID: 2663024
- Sigmundsdottir H, Butcher EC. Environmental cues, dendritic cells and the programming of tissue-selective lymphocyte trafficking. Nat Immunol 2008; 9:981 - 7; http://dx.doi.org/10.1038/ni.f.208; PMID: 18711435
- Masopust D, Vezys V, Usherwood EJ, Cauley LS, Olson S, Marzo AL, Ward RL, Woodland DL, Lefrançois L. Activated primary and memory CD8 T cells migrate to nonlymphoid tissues regardless of site of activation or tissue of origin. J Immunol 2004; 172:4875 - 82; http://dx.doi.org/10.4049/jimmunol.172.8.4875; PMID: 15067066
- Ariotti S, Haanen JB, Schumacher TN. Behavior and function of tissue-resident memory T cells. Adv Immunol 2012; 114:203 - 16; http://dx.doi.org/10.1016/B978-0-12-396548-6.00008-1; PMID: 22449783
- Gebhardt T, Wakim LM, Eidsmo L, Reading PC, Heath WR, Carbone FR. Memory T cells in nonlymphoid tissue that provide enhanced local immunity during infection with herpes simplex virus. Nat Immunol 2009; 10:524 - 30; http://dx.doi.org/10.1038/ni.1718; PMID: 19305395
- Khanna KM, Bonneau RH, Kinchington PR, Hendricks RL. Herpes simplex virus-specific memory CD8+ T cells are selectively activated and retained in latently infected sensory ganglia. Immunity 2003; 18:593 - 603; http://dx.doi.org/10.1016/S1074-7613(03)00112-2; PMID: 12753737
- Wakim LM, Waithman J, van Rooijen N, Heath WR, Carbone FR. Dendritic cell-induced memory T cell activation in nonlymphoid tissues. Science 2008; 319:198 - 202; http://dx.doi.org/10.1126/science.1151869; PMID: 18187654
- Teijaro JR, Turner D, Pham Q, Wherry EJ, Lefrançois L, Farber DL. Cutting edge: Tissue-retentive lung memory CD4 T cells mediate optimal protection to respiratory virus infection. J Immunol 2011; 187:5510 - 4; http://dx.doi.org/10.4049/jimmunol.1102243; PMID: 22058417
- Purwar R, Campbell J, Murphy G, Richards WG, Clark RA, Kupper TS. Resident memory T cells (T(RM)) are abundant in human lung: diversity, function, and antigen specificity. PLoS One 2011; 6:e16245; http://dx.doi.org/10.1371/journal.pone.0016245; PMID: 21298112
- Hofmann M, Pircher H. E-cadherin promotes accumulation of a unique memory CD8 T-cell population in murine salivary glands. Proc Natl Acad Sci U S A 2011; 108:16741 - 6; http://dx.doi.org/10.1073/pnas.1107200108; PMID: 21930933
- Masopust D, Choo D, Vezys V, Wherry EJ, Duraiswamy J, Akondy R, Wang J, Casey KA, Barber DL, Kawamura KS, et al. Dynamic T cell migration program provides resident memory within intestinal epithelium. J Exp Med 2010; 207:553 - 64; http://dx.doi.org/10.1084/jem.20090858; PMID: 20156972
- Masopust D, Schenkel JM. The integration of T cell migration, differentiation and function. Nat Rev Immunol 2013; 13:309 - 20; http://dx.doi.org/10.1038/nri3442; PMID: 23598650
- Carbone FR, Mackay LK, Heath WR, Gebhardt T. Distinct resident and recirculating memory T cell subsets in non-lymphoid tissues. Curr Opin Immunol 2013; 25:329 - 33; http://dx.doi.org/10.1016/j.coi.2013.05.007; PMID: 23746791
- Woodland DL, Kohlmeier JE. Migration, maintenance and recall of memory T cells in peripheral tissues. Nat Rev Immunol 2009; 9:153 - 61; http://dx.doi.org/10.1038/nri2496; PMID: 19240755
- Belyakov IM, Kuznetsov VA, Kelsall B, Klinman D, Moniuszko M, Lemon M, Markham PD, Pal R, Clements JD, Lewis MG, et al. Impact of vaccine-induced mucosal high-avidity CD8+ CTLs in delay of AIDS viral dissemination from mucosa. Blood 2006; 107:3258 - 64; http://dx.doi.org/10.1182/blood-2005-11-4374; PMID: 16373659
- Iwata M, Hirakiyama A, Eshima Y, Kagechika H, Kato C, Song SY. Retinoic acid imprints gut-homing specificity on T cells. Immunity 2004; 21:527 - 38; http://dx.doi.org/10.1016/j.immuni.2004.08.011; PMID: 15485630
- Sigmundsdottir H, Pan J, Debes GF, Alt C, Habtezion A, Soler D, Butcher EC. DCs metabolize sunlight-induced vitamin D3 to ‘program’ T cell attraction to the epidermal chemokine CCL27. Nat Immunol 2007; 8:285 - 93; http://dx.doi.org/10.1038/ni1433; PMID: 17259988
- Stagg AJ, Kamm MA, Knight SC. Intestinal dendritic cells increase T cell expression of alpha4beta7 integrin. Eur J Immunol 2002; 32:1445 - 54; http://dx.doi.org/10.1002/1521-4141(200205)32:5<1445::AID-IMMU1445>3.0.CO;2-E; PMID: 11981833
- Kunkel EJ, Campbell JJ, Haraldsen G, Pan J, Boisvert J, Roberts AI, Ebert EC, Vierra MA, Goodman SB, Genovese MC, et al. Lymphocyte CC chemokine receptor 9 and epithelial thymus-expressed chemokine (TECK) expression distinguish the small intestinal immune compartment: Epithelial expression of tissue-specific chemokines as an organizing principle in regional immunity. J Exp Med 2000; 192:761 - 8; http://dx.doi.org/10.1084/jem.192.5.761; PMID: 10974041
- Campbell DJ, Butcher EC. Rapid acquisition of tissue-specific homing phenotypes by CD4(+) T cells activated in cutaneous or mucosal lymphoid tissues. J Exp Med 2002; 195:135 - 41; http://dx.doi.org/10.1084/jem.20011502; PMID: 11781372
- Dudda JC, Simon JC, Martin S. Dendritic cell immunization route determines CD8+ T cell trafficking to inflamed skin: role for tissue microenvironment and dendritic cells in establishment of T cell-homing subsets. J Immunol 2004; 172:857 - 63; http://dx.doi.org/10.4049/jimmunol.172.2.857; PMID: 14707056
- Lycke N. Recent progress in mucosal vaccine development: potential and limitations. Nat Rev Immunol 2012; 12:592 - 605; http://dx.doi.org/10.1038/nri3251; PMID: 22828912
- Gallichan WS, Rosenthal KL. Long-lived cytotoxic T lymphocyte memory in mucosal tissues after mucosal but not systemic immunization. J Exp Med 1996; 184:1879 - 90; http://dx.doi.org/10.1084/jem.184.5.1879; PMID: 8920875
- Belyakov IM, Derby MA, Ahlers JD, Kelsall BL, Earl P, Moss B, Strober W, Berzofsky JA. Mucosal immunization with HIV-1 peptide vaccine induces mucosal and systemic cytotoxic T lymphocytes and protective immunity in mice against intrarectal recombinant HIV-vaccinia challenge. Proc Natl Acad Sci U S A 1998; 95:1709 - 14; http://dx.doi.org/10.1073/pnas.95.4.1709; PMID: 9465081
- Macpherson AJ, McCoy KD, Johansen FE, Brandtzaeg P. The immune geography of IgA induction and function. Mucosal Immunol 2008; 1:11 - 22; http://dx.doi.org/10.1038/mi.2007.6; PMID: 19079156
- Kunkel EJ, Kim CH, Lazarus NH, Vierra MA, Soler D, Bowman EP, Butcher EC. CCR10 expression is a common feature of circulating and mucosal epithelial tissue IgA Ab-secreting cells. J Clin Invest 2003; 111:1001 - 10; http://dx.doi.org/10.1172/JCI17244; PMID: 12671049
- Pan J, Kunkel EJ, Gosslar U, Lazarus N, Langdon P, Broadwell K, Vierra MA, Genovese MC, Butcher EC, Soler D. A novel chemokine ligand for CCR10 and CCR3 expressed by epithelial cells in mucosal tissues. J Immunol 2000; 165:2943 - 9; http://dx.doi.org/10.4049/jimmunol.165.6.2943; PMID: 10975800
- Cha HR, Ko HJ, Kim ED, Chang SY, Seo SU, Cuburu N, Ryu S, Kim S, Kweon MN. Mucosa-associated epithelial chemokine/CCL28 expression in the uterus attracts CCR10+ IgA plasma cells following mucosal vaccination via estrogen control. J Immunol 2011; 187:3044 - 52; http://dx.doi.org/10.4049/jimmunol.1100402; PMID: 21832166
- Hervouet C, Luci C, Cuburu N, Cremel M, Bekri S, Vimeux L, Marañon C, Czerkinsky C, Hosmalin A, Anjuère F. Sublingual immunization with an HIV subunit vaccine induces antibodies and cytotoxic T cells in the mouse female genital tract. Vaccine 2010; 28:5582 - 90; http://dx.doi.org/10.1016/j.vaccine.2010.06.033; PMID: 20600505
- Ruane D, Brane L, Reis BS, Cheong C, Poles J, Do Y, Zhu H, Velinzon K, Choi JH, Studt N, et al. Lung dendritic cells induce migration of protective T cells to the gastrointestinal tract. J Exp Med 2013; 210:1871 - 88; http://dx.doi.org/10.1084/jem.20122762; PMID: 23960190
- Hervouet C, Luci C, Bekri S, Juhel T, Bihl F, Braud VM, Czerkinsky C, Anjuère F. Antigen-bearing dendritic cells from the sublingual mucosa recirculate to distant systemic lymphoid organs to prime mucosal CD8 T cells. Mucosal Immunol 2014; 7:280 - 91; http://dx.doi.org/10.1038/mi.2013.45; PMID: 23801305
- Marks E, Helgeby A, Andersson JO, Schön K, Lycke NY. CD4⁺ T-cell immunity in the female genital tract is critically dependent on local mucosal immunization. Eur J Immunol 2011; 41:2642 - 53; http://dx.doi.org/10.1002/eji.201041297; PMID: 21681740
- Rudin A, Johansson EL, Bergquist C, Holmgren J. Differential kinetics and distribution of antibodies in serum and nasal and vaginal secretions after nasal and oral vaccination of humans. Infect Immun 1998; 66:3390 - 6; PMID: 9632610
- Chung H, Pamp SJ, Hill JA, Surana NK, Edelman SM, Troy EB, Reading NC, Villablanca EJ, Wang S, Mora JR, et al. Gut immune maturation depends on colonization with a host-specific microbiota. Cell 2012; 149:1578 - 93; http://dx.doi.org/10.1016/j.cell.2012.04.037; PMID: 22726443
- Kamada N, Núñez G. Regulation of the immune system by the resident intestinal bacteria. Gastroenterology 2014; 146:1477 - 88; http://dx.doi.org/10.1053/j.gastro.2014.01.060; PMID: 24503128
- Madhi SA, Cunliffe NA, Steele D, Witte D, Kirsten M, Louw C, Ngwira B, Victor JC, Gillard PH, Cheuvart BB, et al. Effect of human rotavirus vaccine on severe diarrhea in African infants. N Engl J Med 2010; 362:289 - 98; http://dx.doi.org/10.1056/NEJMoa0904797; PMID: 20107214
- Mora JR, Bono MR, Manjunath N, Weninger W, Cavanagh LL, Rosemblatt M, Von Andrian UH. Selective imprinting of gut-homing T cells by Peyer’s patch dendritic cells. Nature 2003; 424:88 - 93; http://dx.doi.org/10.1038/nature01726; PMID: 12840763
- Luiz WB, Cavalcante RC, Paccez JD, Souza RD, Sbrogio-Almeida ME, Ferreira RC, Ferreira LC. Boosting systemic and secreted antibody responses in mice orally immunized with recombinant Bacillus subtilis strains following parenteral priming with a DNA vaccine encoding the enterotoxigenic Escherichia coli (ETEC) CFA/I fimbriae B subunit. Vaccine 2008; 26:3998 - 4005; http://dx.doi.org/10.1016/j.vaccine.2008.05.030; PMID: 18597902
- Im EJ, Borducchi EN, Provine NM, McNally AG, Li S, Frankel FR, Barouch DH. An attenuated Listeria monocytogenes vector primes more potent simian immunodeficiency virus-specific mucosal immunity than DNA vaccines in mice. J Virol 2013; 87:4751 - 5; http://dx.doi.org/10.1128/JVI.03085-12; PMID: 23388715
- Neeson P, Boyer J, Kumar S, Lewis MG, Mattias L, Veazey R, Weiner D, Paterson Y. A DNA prime-oral Listeria boost vaccine in rhesus macaques induces a SIV-specific CD8 T cell mucosal response characterized by high levels of alpha4beta7 integrin and an effector memory phenotype. Virology 2006; 354:299 - 315; http://dx.doi.org/10.1016/j.virol.2006.06.036; PMID: 16904153
- Ribelles P, Benbouziane B, Langella P, Suárez JE, Bermúdez-Humarán LG. Protection against human papillomavirus type 16-induced tumors in mice using non-genetically modified lactic acid bacteria displaying E7 antigen at its surface. Appl Microbiol Biotechnol 2013; 97:1231 - 9; http://dx.doi.org/10.1007/s00253-012-4575-1; PMID: 23212671
- Nardelli-Haefliger D, Roden RB, Benyacoub J, Sahli R, Kraehenbuhl JP, Schiller JT, Lachat P, Potts A, De Grandi P. Human papillomavirus type 16 virus-like particles expressed in attenuated Salmonella typhimurium elicit mucosal and systemic neutralizing antibodies in mice. Infect Immun 1997; 65:3328 - 36; PMID: 9234794
- Hemann EA, Kang SM, Legge KL. Protective CD8 T cell-mediated immunity against influenza A virus infection following influenza virus-like particle vaccination. J Immunol 2013; 191:2486 - 94; http://dx.doi.org/10.4049/jimmunol.1300954; PMID: 23885108
- Price GE, Soboleski MR, Lo CY, Misplon JA, Quirion MR, Houser KV, Pearce MB, Pappas C, Tumpey TM, Epstein SL. Single-dose mucosal immunization with a candidate universal influenza vaccine provides rapid protection from virulent H5N1, H3N2 and H1N1 viruses. PLoS One 2010; 5:e13162; http://dx.doi.org/10.1371/journal.pone.0013162; PMID: 20976273
- Baron SD, Singh R, Metzger DW. Inactivated Francisella tularensis live vaccine strain protects against respiratory tularemia by intranasal vaccination in an immunoglobulin A-dependent fashion. Infect Immun 2007; 75:2152 - 62; http://dx.doi.org/10.1128/IAI.01606-06; PMID: 17296747
- Sui Z, Chen Q, Fang F, Zheng M, Chen Z. Cross-protection against influenza virus infection by intranasal administration of M1-based vaccine with chitosan as an adjuvant. Vaccine 2010; 28:7690 - 8; http://dx.doi.org/10.1016/j.vaccine.2010.09.019; PMID: 20870054
- Zygmunt BM, Rharbaoui F, Groebe L, Guzman CA. Intranasal immunization promotes th17 immune responses. J Immunol 2009; 183:6933 - 8; http://dx.doi.org/10.4049/jimmunol.0901144; PMID: 19890060
- Iwakura Y, Ishigame H, Saijo S, Nakae S. Functional specialization of interleukin-17 family members. Immunity 2011; 34:149 - 62; http://dx.doi.org/10.1016/j.immuni.2011.02.012; PMID: 21349428
- Ashkenazi S, Vertruyen A, Arístegui J, Esposito S, McKeith DD, Klemola T, Biolek J, Kühr J, Bujnowski T, Desgrandchamps D, et al, CAIV-T Study Group. Superior relative efficacy of live attenuated influenza vaccine compared with inactivated influenza vaccine in young children with recurrent respiratory tract infections. Pediatr Infect Dis J 2006; 25:870 - 9; http://dx.doi.org/10.1097/01.inf.0000237829.66310.85; PMID: 17006279
- Song JH, Nguyen HH, Cuburu N, Horimoto T, Ko SY, Park SH, Czerkinsky C, Kweon MN. Sublingual vaccination with influenza virus protects mice against lethal viral infection. Proc Natl Acad Sci U S A 2008; 105:1644 - 9; http://dx.doi.org/10.1073/pnas.0708684105; PMID: 18227512
- Kim SH, Kim JY, Choi Y, Nguyen HH, Song MK, Chang J. Mucosal vaccination with recombinant adenovirus encoding nucleoprotein provides potent protection against influenza virus infection. PLoS One 2013; 8:e75460; http://dx.doi.org/10.1371/journal.pone.0075460; PMID: 24086536
- Çuburu N, Graham BS, Buck CB, Kines RC, Pang YY, Day PM, Lowy DR, Schiller JT. Intravaginal immunization with HPV vectors induces tissue-resident CD8+ T cell responses. J Clin Invest 2012; 122:4606 - 20; http://dx.doi.org/10.1172/JCI63287; PMID: 23143305
- Shim BS, Choi Y, Cheon IS, Song MK. Sublingual delivery of vaccines for the induction of mucosal immunity. Immune Netw 2013; 13:81 - 5; http://dx.doi.org/10.4110/in.2013.13.3.81; PMID: 23885221
- Pedersen G, Cox R. The mucosal vaccine quandary: intranasal vs. sublingual immunization against influenza. Hum Vaccin Immunother 2012; 8:689 - 93; http://dx.doi.org/10.4161/hv.19568; PMID: 22495121
- Huo Z, Bissett SL, Giemza R, Beddows S, Oeser C, Lewis DJ. Systemic and mucosal immune responses to sublingual or intramuscular human papilloma virus antigens in healthy female volunteers. PLoS One 2012; 7:e33736; http://dx.doi.org/10.1371/journal.pone.0033736; PMID: 22438987
- Luci C, Hervouet C, Rousseau D, Holmgren J, Czerkinsky C, Anjuère F. Dendritic cell-mediated induction of mucosal cytotoxic responses following intravaginal immunization with the nontoxic B subunit of cholera toxin. J Immunol 2006; 176:2749 - 57; http://dx.doi.org/10.4049/jimmunol.176.5.2749; PMID: 16493030
- Klein K, Mann JF, Rogers P, Shattock RJ. Polymeric penetration enhancers promote humoral immune responses to mucosal vaccines. Journal of controlled release: official journal of the Controlled Release Society 2014.
- Echchannaoui H, Bianchi M, Baud D, Bobst M, Stehle JC, Nardelli-Haefliger D. Intravaginal immunization of mice with recombinant Salmonella enterica serovar Typhimurium expressing human papillomavirus type 16 antigens as a potential route of vaccination against cervical cancer. Infect Immun 2008; 76:1940 - 51; http://dx.doi.org/10.1128/IAI.01484-07; PMID: 18332214
- Domingos-Pereira S, Decrausaz L, Derré L, Bobst M, Romero P, Schiller JT, Jichlinski P, Nardelli-Haefliger D. Intravaginal TLR agonists increase local vaccine-specific CD8 T cells and human papillomavirus-associated genital-tumor regression in mice. Mucosal Immunol 2013; 6:393 - 404; http://dx.doi.org/10.1038/mi.2012.83; PMID: 22968420
- Seavey MM, Mosmann TR. Estradiol-induced vaginal mucus inhibits antigen penetration and CD8(+) T cell priming in response to intravaginal immunization. Vaccine 2009; 27:2342 - 9; http://dx.doi.org/10.1016/j.vaccine.2009.02.025; PMID: 19428849
- Soler E, Parez N, Passet B, Dubuquoy C, Riffault S, Pillot M, Houdebine LM, Schwartz-Cornil I. Recombinant rotavirus inner core proteins produced in the milk of transgenic rabbits confer a high level of protection after intrarectal delivery. Vaccine 2007; 25:6373 - 80; http://dx.doi.org/10.1016/j.vaccine.2007.06.011; PMID: 17629366
- Alkadah A, Thiam F, Mounier M, Charpilienne A, Poncet D, Kohli E, Basset C. Different profile and distribution of antigen specific T cells induced by intranasal and intrarectal immunization with rotavirus 2/6-VLP with and without LT-R192G. Vaccine 2013; 31:1924 - 30; http://dx.doi.org/10.1016/j.vaccine.2013.02.019; PMID: 23453731
- Polacino PS, Stallard V, Klaniecki JE, Pennathur S, Montefiori DC, Langlois AJ, Richardson BA, Morton WR, Benveniste RE, Hu SL. Role of immune responses against the envelope and the core antigens of simian immunodeficiency virus SIVmne in protection against homologous cloned and uncloned virus challenge in Macaques. J Virol 1999; 73:8201 - 15; PMID: 10482571
- Belyakov IM, Isakov D, Zhu Q, Dzutsev A, Berzofsky JA. A novel functional CTL avidity/activity compartmentalization to the site of mucosal immunization contributes to protection of macaques against simian/human immunodeficiency viral depletion of mucosal CD4+ T cells. J Immunol 2007; 178:7211 - 21; http://dx.doi.org/10.4049/jimmunol.178.11.7211; PMID: 17513770
- Hu K, Dou J, Yu F, He X, Yuan X, Wang Y, Liu C, Gu N. An ocular mucosal administration of nanoparticles containing DNA vaccine pRSC-gD-IL-21 confers protection against mucosal challenge with herpes simplex virus type 1 in mice. Vaccine 2011; 29:1455 - 62; http://dx.doi.org/10.1016/j.vaccine.2010.12.031; PMID: 21185849
- Griffiths KL, Stylianou E, Poyntz HC, Betts GJ, Fletcher HA, McShane H. Cholera toxin enhances vaccine-induced protection against Mycobacterium tuberculosis challenge in mice. PLoS One 2013; 8:e78312; http://dx.doi.org/10.1371/journal.pone.0078312; PMID: 24194918
- Rhee JH, Lee SE, Kim SY. Mucosal vaccine adjuvants update. Clinical and experimental vaccine research 2012; 1:50-63.
- Mutsch M, Zhou W, Rhodes P, Bopp M, Chen RT, Linder T, Spyr C, Steffen R. Use of the inactivated intranasal influenza vaccine and the risk of Bell’s palsy in Switzerland. N Engl J Med 2004; 350:896 - 903; http://dx.doi.org/10.1056/NEJMoa030595; PMID: 14985487
- da Hora VP, Conceição FR, Dellagostin OA, Doolan DL. Non-toxic derivatives of LT as potent adjuvants. Vaccine 2011; 29:1538 - 44; http://dx.doi.org/10.1016/j.vaccine.2010.11.091; PMID: 21163247
- Nawar HF, Arce S, Russell MW, Connell TD. Mutants of type II heat-labile enterotoxin LT-IIa with altered ganglioside-binding activities and diminished toxicity are potent mucosal adjuvants. Infect Immun 2007; 75:621 - 33; http://dx.doi.org/10.1128/IAI.01009-06; PMID: 17118982
- Didierlaurent AM, Morel S, Lockman L, Giannini SL, Bisteau M, Carlsen H, Kielland A, Vosters O, Vanderheyde N, Schiavetti F, et al. AS04, an aluminum salt- and TLR4 agonist-based adjuvant system, induces a transient localized innate immune response leading to enhanced adaptive immunity. J Immunol 2009; 183:6186 - 97; http://dx.doi.org/10.4049/jimmunol.0901474; PMID: 19864596
- Schwarz TF, Spaczynski M, Schneider A, Wysocki J, Galaj A, Perona P, Poncelet S, Zahaf T, Hardt K, Descamps D, et al, HPV Study Group for Adult Women. Immunogenicity and tolerability of an HPV-16/18 AS04-adjuvanted prophylactic cervical cancer vaccine in women aged 15-55 years. Vaccine 2009; 27:581 - 7; http://dx.doi.org/10.1016/j.vaccine.2008.10.088; PMID: 19022320
- Cranage MP, Fraser CA, Cope A, McKay PF, Seaman MS, Cole T, Mahmoud AN, Hall J, Giles E, Voss G, et al. Antibody responses after intravaginal immunisation with trimeric HIV-1 CN54 clade C gp140 in Carbopol gel are augmented by systemic priming or boosting with an adjuvanted formulation. Vaccine 2011; 29:1421 - 30; http://dx.doi.org/10.1016/j.vaccine.2010.12.034; PMID: 21187177
- Fernandez S, Cisney ED, Ulrich RG. Enhancement of serum and mucosal immune responses to a Haemophilus influenzae Type B vaccine by intranasal delivery. Clin Vaccine Immunol 2013; 20:1690 - 6; http://dx.doi.org/10.1128/CVI.00215-13; PMID: 23986319
- Arias MA, Van Roey GA, Tregoning JS, Moutaftsi M, Coler RN, Windish HP, Reed SG, Carter D, Shattock RJ. Glucopyranosyl Lipid Adjuvant (GLA), a Synthetic TLR4 agonist, promotes potent systemic and mucosal responses to intranasal immunization with HIVgp140. PLoS One 2012; 7:e41144; http://dx.doi.org/10.1371/journal.pone.0041144; PMID: 22829921
- Huang CF, Wu TC, Chu YH, Hwang KS, Wang CC, Peng HJ. Effect of neonatal sublingual vaccination with native or denatured ovalbumin and adjuvant CpG or cholera toxin on systemic and mucosal immunity in mice. Scand J Immunol 2008; 68:502 - 10; http://dx.doi.org/10.1111/j.1365-3083.2008.02172.x; PMID: 18822109
- Pesce I, Monaci E, Muzzi A, Tritto E, Tavarini S, Nuti S, De Gregorio E, Wack A. Intranasal administration of CpG induces a rapid and transient cytokine response followed by dendritic and natural killer cell activation and recruitment in the mouse lung. J Innate Immun 2010; 2:144 - 59; http://dx.doi.org/10.1159/000254948; PMID: 20375632
- Jiang W, Sun R, Zhou R, Wei H, Tian Z. TLR-9 activation aggravates concanavalin A-induced hepatitis via promoting accumulation and activation of liver CD4+ NKT cells. J Immunol 2009; 182:3768 - 74; http://dx.doi.org/10.4049/jimmunol.0800973; PMID: 19265155
- McNally B, Willette M, Ye F, Partida-Sanchez S, Flaño E. Intranasal administration of dsRNA analog poly(I:C) induces interferon-α receptor-dependent accumulation of antigen experienced T cells in the airways. PLoS One 2012; 7:e51351; http://dx.doi.org/10.1371/journal.pone.0051351; PMID: 23236482
- Ainai A, Ichinohe T, Tamura S, Kurata T, Sata T, Tashiro M, Hasegawa H. Zymosan enhances the mucosal adjuvant activity of poly(I:C) in a nasal influenza vaccine. J Med Virol 2010; 82:476 - 84; http://dx.doi.org/10.1002/jmv.21694; PMID: 20087927
- Nguyen CT, Kim SY, Kim MS, Lee SE, Rhee JH. Intranasal immunization with recombinant PspA fused with a flagellin enhances cross-protective immunity against Streptococcus pneumoniae infection in mice. Vaccine 2011; 29:5731 - 9; http://dx.doi.org/10.1016/j.vaccine.2011.05.095; PMID: 21696869
- Hong SH, Byun YH, Nguyen CT, Kim SY, Seong BL, Park S, Woo GJ, Yoon Y, Koh JT, Fujihashi K, et al. Intranasal administration of a flagellin-adjuvanted inactivated influenza vaccine enhances mucosal immune responses to protect mice against lethal infection. Vaccine 2012; 30:466 - 74; http://dx.doi.org/10.1016/j.vaccine.2011.10.058; PMID: 22051136
- Braga CJ, Rittner GM, Muñoz Henao JE, Teixeira AF, Massis LM, Sbrogio-Almeida ME, Taborda CP, Travassos LR, Ferreira LC. Paracoccidioides brasiliensis vaccine formulations based on the gp43-derived P10 sequence and the Salmonella enterica FliC flagellin. Infect Immun 2009; 77:1700 - 7; http://dx.doi.org/10.1128/IAI.01470-08; PMID: 19204092
- Braga CJ, Massis LM, Sbrogio-Almeida ME, Alencar BC, Bargieri DY, Boscardin SB, Rodrigues MM, Ferreira LC. CD8+ T cell adjuvant effects of Salmonella FliCd flagellin in live vaccine vectors or as purified protein. Vaccine 2010; 28:1373 - 82; http://dx.doi.org/10.1016/j.vaccine.2009.11.003; PMID: 19932669
- Hjelm BE, Kilbourne J, Herbst-Kralovetz MM. TLR7 and 9 agonists are highly effective mucosal adjuvants for norovirus virus-like particle vaccines. Hum Vaccin Immunother 2013; 9:10; PMID: 24280723
- Kobayashi T, Fukushima K, Sannan T, Saito N, Takiguchi Y, Sato Y, Hasegawa H, Ishikawa K. Evaluation of the effectiveness and safety of chitosan derivatives as adjuvants for intranasal vaccines. Viral Immunol 2013; 26:133 - 42; http://dx.doi.org/10.1089/vim.2012.0057; PMID: 23509985
- Zaharoff DA, Rogers CJ, Hance KW, Schlom J, Greiner JW. Chitosan solution enhances both humoral and cell-mediated immune responses to subcutaneous vaccination. Vaccine 2007; 25:2085 - 94; http://dx.doi.org/10.1016/j.vaccine.2006.11.034; PMID: 17258843
- Adotevi O, Vingert B, Freyburger L, Shrikant P, Lone YC, Quintin-Colonna F, Haicheur N, Amessou M, Herbelin A, Langlade-Demoyen P, et al. B subunit of Shiga toxin-based vaccines synergize with alpha-galactosylceramide to break tolerance against self antigen and elicit antiviral immunity. J Immunol 2007; 179:3371 - 9; http://dx.doi.org/10.4049/jimmunol.179.5.3371; PMID: 17709554
- Fujii S, Shimizu K, Smith C, Bonifaz L, Steinman RM. Activation of natural killer T cells by alpha-galactosylceramide rapidly induces the full maturation of dendritic cells in vivo and thereby acts as an adjuvant for combined CD4 and CD8 T cell immunity to a coadministered protein. J Exp Med 2003; 198:267 - 79; http://dx.doi.org/10.1084/jem.20030324; PMID: 12874260
- Kamijuku H, Nagata Y, Jiang X, Ichinohe T, Tashiro T, Mori K, Taniguchi M, Hase K, Ohno H, Shimaoka T, et al. Mechanism of NKT cell activation by intranasal coadministration of alpha-galactosylceramide, which can induce cross-protection against influenza viruses. Mucosal Immunol 2008; 1:208 - 18; http://dx.doi.org/10.1038/mi.2008.2; PMID: 19079180
- Ko SY, Ko HJ, Chang WS, Park SH, Kweon MN, Kang CY. alpha-Galactosylceramide can act as a nasal vaccine adjuvant inducing protective immune responses against viral infection and tumor. J Immunol 2005; 175:3309 - 17; http://dx.doi.org/10.4049/jimmunol.175.5.3309; PMID: 16116223
- Youn HJ, Ko SY, Lee KA, Ko HJ, Lee YS, Fujihashi K, Boyaka PN, Kim SH, Horimoto T, Kweon MN, et al. A single intranasal immunization with inactivated influenza virus and alpha-galactosylceramide induces long-term protective immunity without redirecting antigen to the central nervous system. Vaccine 2007; 25:5189 - 98; http://dx.doi.org/10.1016/j.vaccine.2007.04.081; PMID: 17548137
- Courtney AN, Thapa P, Singh S, Wishahy AM, Zhou D, Sastry J. Intranasal but not intravenous delivery of the adjuvant α-galactosylceramide permits repeated stimulation of natural killer T cells in the lung. Eur J Immunol 2011; 41:3312 - 22; http://dx.doi.org/10.1002/eji.201041359; PMID: 21818755
- Kunii N, Horiguchi S, Motohashi S, Yamamoto H, Ueno N, Yamamoto S, Sakurai D, Taniguchi M, Nakayama T, Okamoto Y. Combination therapy of in vitro-expanded natural killer T cells and alpha-galactosylceramide-pulsed antigen-presenting cells in patients with recurrent head and neck carcinoma. Cancer Sci 2009; 100:1092 - 8; http://dx.doi.org/10.1111/j.1349-7006.2009.01135.x; PMID: 19302288
- Yoshino N, Endo M, Kanno H, Matsukawa N, Tsutsumi R, Takeshita R, Sato S. Polymyxins as novel and safe mucosal adjuvants to induce humoral immune responses in mice. PLoS One 2013; 8:e61643; http://dx.doi.org/10.1371/journal.pone.0061643; PMID: 23593492
- Shin H, Iwasaki A. Generating protective immunity against genital herpes. Trends Immunol 2013; 34:487 - 94; http://dx.doi.org/10.1016/j.it.2013.08.001; PMID: 24012144
- Hammerschmidt SI, Friedrichsen M, Boelter J, Lyszkiewicz M, Kremmer E, Pabst O, Förster R. Retinoic acid induces homing of protective T and B cells to the gut after subcutaneous immunization in mice. J Clin Invest 2011; 121:3051 - 61; http://dx.doi.org/10.1172/JCI44262; PMID: 21737878
- Jiang T, Singh B, Li HS, Kim YK, Kang SK, Nah JW, Choi YJ, Cho CS. Targeted oral delivery of BmpB vaccine using porous PLGA microparticles coated with M cell homing peptide-coupled chitosan. Biomaterials 2014; 35:2365 - 73; http://dx.doi.org/10.1016/j.biomaterials.2013.11.073; PMID: 24342722
- Yamamoto M, Pascual DW, Kiyono H. M cell-targeted mucosal vaccine strategies. Curr Top Microbiol Immunol 2012; 354:39 - 52; http://dx.doi.org/10.1007/82_2011_134; PMID: 21688209
- Mohamadzadeh M, Duong T, Sandwick SJ, Hoover T, Klaenhammer TR. Dendritic cell targeting of Bacillus anthracis protective antigen expressed by Lactobacillus acidophilus protects mice from lethal challenge. Proc Natl Acad Sci U S A 2009; 106:4331 - 6; http://dx.doi.org/10.1073/pnas.0900029106; PMID: 19246373
- de Souza RD, Batista MT, Luiz WB, Cavalcante RC, Amorim JH, Bizerra RS, Martins EG, Ferreira LC. Bacillus subtilis spores as vaccine adjuvants: further insights into the mechanisms of action. PLoS One 2014; 9:e87454; http://dx.doi.org/10.1371/journal.pone.0087454; PMID: 24475289
- Haicheur N, Bismuth E, Bosset S, Adotevi O, Warnier G, Lacabanne V, Regnault A, Desaymard C, Amigorena S, Ricciardi-Castagnoli P, et al. The B subunit of Shiga toxin fused to a tumor antigen elicits CTL and targets dendritic cells to allow MHC class I-restricted presentation of peptides derived from exogenous antigens. J Immunol 2000; 165:3301 - 8; http://dx.doi.org/10.4049/jimmunol.165.6.3301; PMID: 10975847
- Vingert B, Adotevi O, Patin D, Jung S, Shrikant P, Freyburger L, Eppolito C, Sapoznikov A, Amessou M, Quintin-Colonna F, et al. The Shiga toxin B-subunit targets antigen in vivo to dendritic cells and elicits anti-tumor immunity. Eur J Immunol 2006; 36:1124 - 35; http://dx.doi.org/10.1002/eji.200535443; PMID: 16568496
- Smith DC, Lord JM, Roberts LM, Tartour E, Johannes L. 1st class ticket to class I: protein toxins as pathfinders for antigen presentation. Traffic 2002; 3:697 - 704; http://dx.doi.org/10.1034/j.1600-0854.2002.31001.x; PMID: 12230467
- Sandoval F, Terme M, Nizard M, Badoual C, Bureau MF, Freyburger L, Clement O, Marcheteau E, Gey A, Fraisse G, et al. Mucosal imprinting of vaccine-induced CD8(+) T cells is crucial to inhibit the growth of mucosal tumors. Science translational medicine 2013; 5:172ra20.
- Mejias MP, Ghersi G, Craig PO, Panek CA, Bentancor LV, Baschkier A, Goldbaum FA, Zylberman V, Palermo MS. Immunization with a chimera consisting of the B subunit of Shiga toxin type 2 and brucella lumazine synthase confers total protection against Shiga toxins in mice. J Immunol 2013; 191:2403 - 11; http://dx.doi.org/10.4049/jimmunol.1300999; PMID: 23918978
- Gupta P, Singh MK, Singh Y, Gautam V, Kumar S, Kumar O, Dhaked RK. Recombinant Shiga toxin B subunit elicits protection against Shiga toxin via mixed Th type immune response in mice. Vaccine 2011; 29:8094 - 100; http://dx.doi.org/10.1016/j.vaccine.2011.08.040; PMID: 21856355
- Pere H, Montier Y, Bayry J, Quintin-Colonna F, Merillon N, Dransart E, Badoual C, Gey A, Ravel P, Marcheteau E, et al. A CCR4 antagonist combined with vaccines induces antigen-specific CD8+ T cells and tumor immunity against self antigens. Blood 2011; 118:4853 - 62; http://dx.doi.org/10.1182/blood-2011-01-329656; PMID: 21908423
- Johannes L, Tartour E. Correspondence to Creydt VP et al., Cytotoxic effect of Shiga toxin-2 holotoxin and its B subunit on human renal tubular epithelial cells, Microbes Infect. 8(2) (2006) 410-419. Microbes and infection / Institut Pasteur 2006; 8:2331-2.
- Samstein RM, Perica K, Balderrama F, Look M, Fahmy TM. The use of deoxycholic acid to enhance the oral bioavailability of biodegradable nanoparticles. Biomaterials 2008; 29:703 - 8; http://dx.doi.org/10.1016/j.biomaterials.2007.10.026; PMID: 18006053
- Katz DE, DeLorimier AJ, Wolf MK, Hall ER, Cassels FJ, van Hamont JE, Newcomer RL, Davachi MA, Taylor DN, McQueen CE. Oral immunization of adult volunteers with microencapsulated enterotoxigenic Escherichia coli (ETEC) CS6 antigen. Vaccine 2003; 21:341 - 6; http://dx.doi.org/10.1016/S0264-410X(02)00613-8; PMID: 12531630
- Lawson LB, Norton EB, Clements JD. Defending the mucosa: adjuvant and carrier formulations for mucosal immunity. Curr Opin Immunol 2011; 23:414 - 20; http://dx.doi.org/10.1016/j.coi.2011.03.009; PMID: 21511452
- Treanor J, Nolan C, O’Brien D, Burt D, Lowell G, Linden J, Fries L. Intranasal administration of a proteosome-influenza vaccine is well-tolerated and induces serum and nasal secretion influenza antibodies in healthy human subjects. Vaccine 2006; 24:254 - 62; http://dx.doi.org/10.1016/j.vaccine.2005.07.088; PMID: 16129526
- De Smet R, Allais L, Cuvelier C. Recent advances in oral vaccine development: Yeast-derived β-glucan particles. Hum Vaccin Immunother 2014; 10:10; http://dx.doi.org/10.4161/hv.28166; PMID: 24553259
- De Smet R, Demoor T, Verschuere S, Dullaers M, Ostroff GR, Leclercq G, Allais L, Pilette C, Dierendonck M, De Geest BG, et al. beta-Glucan microparticles are good candidates for mucosal antigen delivery in oral vaccination. Journal of controlled release: official journal of the Controlled Release Society 2013; 172:671-8.
- Tamura S. Studies on the usefulness of intranasal inactivated influenza vaccines. Vaccine 2010; 28:6393 - 7; http://dx.doi.org/10.1016/j.vaccine.2010.05.019; PMID: 20493820
- Neutra MR, Kozlowski PA. Mucosal vaccines: the promise and the challenge. Nat Rev Immunol 2006; 6:148 - 58; http://dx.doi.org/10.1038/nri1777; PMID: 16491139
- Perrone LA, Ahmad A, Veguilla V, Lu X, Smith G, Katz JM, Pushko P, Tumpey TM. Intranasal vaccination with 1918 influenza virus-like particles protects mice and ferrets from lethal 1918 and H5N1 influenza virus challenge. J Virol 2009; 83:5726 - 34; http://dx.doi.org/10.1128/JVI.00207-09; PMID: 19321609
- Forbes EK, Sander C, Ronan EO, McShane H, Hill AV, Beverley PC, Tchilian EZ. Multifunctional, high-level cytokine-producing Th1 cells in the lung, but not spleen, correlate with protection against Mycobacterium tuberculosis aerosol challenge in mice. J Immunol 2008; 181:4955 - 64; http://dx.doi.org/10.4049/jimmunol.181.7.4955; PMID: 18802099
- Gallichan WS, Woolstencroft RN, Guarasci T, McCluskie MJ, Davis HL, Rosenthal KL. Intranasal immunization with CpG oligodeoxynucleotides as an adjuvant dramatically increases IgA and protection against herpes simplex virus-2 in the genital tract. J Immunol 2001; 166:3451 - 7; http://dx.doi.org/10.4049/jimmunol.166.5.3451; PMID: 11207303
- Kaufman DR, Liu J, Carville A, Mansfield KG, Havenga MJ, Goudsmit J, Barouch DH. Trafficking of antigen-specific CD8+ T lymphocytes to mucosal surfaces following intramuscular vaccination. J Immunol 2008; 181:4188 - 98; http://dx.doi.org/10.4049/jimmunol.181.6.4188; PMID: 18768876
- Czerkinsky C, Holmgren J. Mucosal delivery routes for optimal immunization: targeting immunity to the right tissues. Curr Top Microbiol Immunol 2012; 354:1 - 18; http://dx.doi.org/10.1007/82_2010_112; PMID: 21053117
- Tartour E, Zitvogel L. Lung cancer: potential targets for immunotherapy. The lancet. Respir Med 2013; 1:551 - 63
- Kroemer G, Zitvogel L, Galluzzi L. Victories and deceptions in tumor immunology: Stimuvax(®). Oncoimmunology 2013; 2:e23687; http://dx.doi.org/10.4161/onci.23687; PMID: 23483762
- Vansteenkiste J, Zielinski M, Linder A, Dahabreh J, Gonzalez EE, Malinowski W, Lopez-Brea M, Vanakesa T, Jassem J, Kalofonos H, et al. Adjuvant MAGE-A3 immunotherapy in resected non-small-cell lung cancer: phase II randomized study results. Journal of clinical oncology: official journal of the American Society of Clinical Oncology 2013; 31:2396-403.
- Ramlau R, Quoix E, Rolski J, Pless M, Lena H, Levy E, Krzakowski M, Hess D, Tartour E, Chenard MP, et al. A phase II study of Tg4010 (Mva-Muc1-Il2) in association with chemotherapy in patients with stage III/IV Non-small cell lung cancer. Journal of thoracic oncology: official publication of the International Association for the Study of Lung Cancer 2008; 3:735-44.
- Li AV, Moon JJ, Abraham W, Suh H, Elkhader J, Seidman MA, Yen M, Im EJ, Foley MH, Barouch DH, et al. Generation of effector memory T cell-based mucosal and systemic immunity with pulmonary nanoparticle vaccination. Science translational medicine 2013; 5:204ra130.
- Yu CI, Becker C, Wang Y, Marches F, Helft J, Leboeuf M, Anguiano E, Pourpe S, Goller K, Pascual V, et al. Human CD1c+ dendritic cells drive the differentiation of CD103+ CD8+ mucosal effector T cells via the cytokine TGF-β. Immunity 2013; 38:818 - 30; http://dx.doi.org/10.1016/j.immuni.2013.03.004; PMID: 23562160
- Wakabayashi A, Nakagawa Y, Shimizu M, Moriya K, Nishiyama Y, Takahashi H. Suppression of an already established tumor growing through activated mucosal CTLs induced by oral administration of tumor antigen with cholera toxin. J Immunol 2008; 180:4000 - 10; http://dx.doi.org/10.4049/jimmunol.180.6.4000; PMID: 18322209
- Bourquin C, von der Borch P, Zoglmeier C, Anz D, Sandholzer N, Suhartha N, Wurzenberger C, Denzel A, Kammerer R, Zimmermann W, et al. Efficient eradication of subcutaneous but not of autochthonous gastric tumors by adoptive T cell transfer in an SV40 T antigen mouse model. J Immunol 2010; 185:2580 - 8; http://dx.doi.org/10.4049/jimmunol.0903231; PMID: 20644173
- Mullins DW, Sheasley SL, Ream RM, Bullock TN, Fu YX, Engelhard VH. Route of immunization with peptide-pulsed dendritic cells controls the distribution of memory and effector T cells in lymphoid tissues and determines the pattern of regional tumor control. J Exp Med 2003; 198:1023 - 34; http://dx.doi.org/10.1084/jem.20021348; PMID: 14530375
- Kim-Schulze S, Kim HS, Wainstein A, Kim DW, Yang WC, Moroziewicz D, Mong PY, Bereta M, Taback B, Wang Q, et al. Intrarectal vaccination with recombinant vaccinia virus expressing carcinoembronic antigen induces mucosal and systemic immunity and prevents progression of colorectal cancer. J Immunol 2008; 181:8112 - 9; http://dx.doi.org/10.4049/jimmunol.181.11.8112; PMID: 19018004
- Domingos-Pereira S, Derré L, Warpelin-Decrausaz L, Haefliger JA, Romero P, Jichlinski P, Nardelli-Haefliger D. Intravaginal and subcutaneous immunization induced vaccine specific CD8 T cells and tumor regression in the bladder. J Urol 2014; 191:814 - 22; http://dx.doi.org/10.1016/j.juro.2013.08.009; PMID: 23954582
- Decrausaz L, Domingos-Pereira S, Duc M, Bobst M, Romero P, Schiller JT, Jichlinski P, Nardelli-Haefliger D. Parenteral is more efficient than mucosal immunization to induce regression of human papillomavirus-associated genital tumors. International journal of cancer Journal international du cancer 2011; 129:762-72.
- Kenter GG, Welters MJ, Valentijn AR, Lowik MJ, Berends-van der Meer DM, Vloon AP, Essahsah F, Fathers LM, Offringa R, Drijfhout JW, et al. Vaccination against HPV-16 oncoproteins for vulvar intraepithelial neoplasia. N Engl J Med 2009; 361:1838 - 47; http://dx.doi.org/10.1056/NEJMoa0810097; PMID: 19890126
- Pages F. Tumor-associated immune parameters for personalized patient care. Science translational medicine 2013; 5:214fs42.
- Devaud C, Westwood JA, John LB, Flynn JK, Paquet-Fifield S, Duong CP, Yong CS, Pegram HJ, Stacker SA, Achen MG, et al. Tissues in different anatomical sites can sculpt and vary the tumor microenvironment to affect responses to therapy. Molecular therapy: the journal of the American Society of Gene Therapy 2014; 22:18-27.
- Badoual C, Hans S, Merillon N, Van Ryswick C, Ravel P, Benhamouda N, Levionnois E, Nizard M, Si-Mohamed A, Besnier N, et al. PD-1-expressing tumor-infiltrating T cells are a favorable prognostic biomarker in HPV-associated head and neck cancer. Cancer Res 2013; 73:128 - 38; http://dx.doi.org/10.1158/0008-5472.CAN-12-2606; PMID: 23135914
- Petaja T, Pedersen C, Poder A, Strauss G, Catteau G, Thomas F, Lehtinen M, Descamps D. Long-term persistence of systemic and mucosal immune response to HPV-16/18 AS04-adjuvanted vaccine in preteen/adolescent girls and young women. International journal of cancer Journal international du cancer 2011; 129:2147-57.
- Boelen A, Andeweg A, Kwakkel J, Lokhorst W, Bestebroer T, Dormans J, Kimman T. Both immunisation with a formalin-inactivated respiratory syncytial virus (RSV) vaccine and a mock antigen vaccine induce severe lung pathology and a Th2 cytokine profile in RSV-challenged mice. Vaccine 2000; 19:982 - 91; http://dx.doi.org/10.1016/S0264-410X(00)00213-9; PMID: 11115725
- Flipse J, Wilschut J, Smit JM. Molecular mechanisms involved in antibody-dependent enhancement of dengue virus infection in humans. Traffic 2013; 14:25 - 35; PMID: 22998156
- Ray SJ, Franki SN, Pierce RH, Dimitrova S, Koteliansky V, Sprague AG, Doherty PC, de Fougerolles AR, Topham DJ. The collagen binding alpha1beta1 integrin VLA-1 regulates CD8 T cell-mediated immune protection against heterologous influenza infection. Immunity 2004; 20:167 - 79; http://dx.doi.org/10.1016/S1074-7613(04)00021-4; PMID: 14975239
- Tang VA, Rosenthal KL. Intravaginal infection with herpes simplex virus type-2 (HSV-2) generates a functional effector memory T cell population that persists in the murine genital tract. J Reprod Immunol 2010; 87:39 - 44; http://dx.doi.org/10.1016/j.jri.2010.06.155; PMID: 20688399
- Kelly KA, Chan AM, Butch A, Darville T. Two different homing pathways involving integrin β7 and E-selectin significantly influence trafficking of CD4 cells to the genital tract following Chlamydia muridarum infection. Am J Reprod Immunol 2009; 61:438 - 45; http://dx.doi.org/10.1111/j.1600-0897.2009.00704.x; PMID: 19392981