Abstract
Overexpressed tumor-self antigens represent the largest group of candidate vaccine targets. Those exhibiting a role in oncogenesis may be some of the least studied but perhaps most promising. This review considers this subset of self antigens by highlighting vaccine efforts for some of the better known members and focusing on TPD52, a new promising vaccine target. We shed light on the importance of both preclinical and clinical vaccine studies demonstrating that tolerance and autoimmunity (presumed to preclude this class of antigens from vaccine development) can be overcome and do not present the obstacle that might have been expected. The potential of this class of antigens for broad application is considered, possibly in the context of low tumor burden or adjuvant therapy, as is the need to understand mechanisms of tolerance that are relatively understudied.
Abbreviations
TPD52 | = | tumor protein D52 |
mD52 | = | murine TPD52 |
hD52 | = | human TPD52 |
TAA | = | tumor associated antigen |
Treg | = | T regulatory cell |
ODN | = | oligodeoxynucleotide |
TRAMP | = | Transgenic adenocarcinoma of the mouse prostate |
CTLA-4 | = | cytotoxic T lymphocyte-associated antigen 4 |
WT-1 | = | Wilms tumor-1 |
VEGFR2 | = | vascular endothelial growth factor receptor 2 |
ALK | = | Anaplastic lymphoma kinase |
Her-2/neu | = | human epithelial growth factor receptor 2 |
AR | = | androgen receptor |
CTL | = | cytotoxic T lymphocyte |
HLA | = | human leukocyte antigen |
Introduction
Current statistics reveal an increase in cancer incidence with very little decrease in mortality. It is estimated that about 1 660 290 new cancer cases were diagnosed in the USA in 2013, with 580 350 (35%) deaths.Citation1 Improvement of treatments to decrease mortality may be met through immune-based therapies. The employment of the immune system to treat malignant tumors, commonly referred to as tumor immunotherapy, encompasses two general categories: passive and active.Citation2 Passive immunotherapy largely involves the administration of specific antibodies, cytokines, or cells (most commonly tumor antigen-specific T cells generated ex vivo, known as adoptive cell therapy). Passive administration of T cells or of monoclonal antibodies against the T cells inhibitory receptor CTLA-4, which is referred to as immune checkpoint blockade, has recently gained international acclaim as the breakthrough of the year (2013),Citation3 though not without immune related adverse effects.Citation4 Active immunotherapy can be most easily defined by vaccination. While passive immunotherapies often engage the immune system independent of the knowledge of defined tumor antigens (an exception being some forms of adoptive cell therapyCitation5), vaccines elicit specific immune responses by targeting defined tumor antigens.Citation6
With the exception of vaccines to prevent cervical cancer by targeting select serotypes of human papillomaviruses,Citation7 most if not all vaccine clinical trials have focused on therapy. In this approach the vaccine is administered when tumors are present in the patient, with the primary goal of shrinking the tumor mass. However, the majority of clinical trials to treat established solid tumors by vaccination have yielded disappointing results.Citation8 This lack of success is underscored by the existence of only one FDA approved cell-based therapeutic vaccine Sipuleucel T (Provenge®), approved in 2010.Citation8 However this is not to say that cancer vaccination research is without merit. On the contrary, much work is still being done to advance and improve cancer vaccines.
The idea of preventive cancer vaccination has recently gained new attention,Citation9 and may represent the next significant advance in the field of immune-based cancer treatments. The logic behind preventive vaccination is sound and supported by centuries of success against infectious agents. In this case, why hasn't vaccination been applied more broadly to cancer prevention? It is reasonable to speculate that cancer vaccines have been applied as an option for compassionate administration as a last effort for patients who have failed conventional therapy. This is essentially the approved application for Sipuleucel T, which is not curative but extends life for some late stage prostate cancer patients.Citation10 Advances in early cancer detection have opened the door to develop vaccines to prevent primary and recurrent tumors rather than shrink existing tumor masses.
Whether the vaccine is therapeutic or preventive, it is clear that future success will depend on the character of the tumor antigen targeted by the vaccine. The current collective of tumor antigens ranges from specific to associated, non-self to self, and comprises hundreds of vaccine candidates (far too many to discuss in a single review article). In this light, an emerging group of cancer vaccine target antigens, defined primarily by their overexpression in tumor cells compared with low but detectable levels in normal cells, and a role in oncogenesis, represents the focus of this review. Other related topics such as comparisons of vaccine strategies, or the use of adjuvants from different clinical studies, have been recently reviewed elsewhere.Citation11
Tumor Antigen Classifications
Tumor antigens recognized by the immune system have been most often defined based on the nature of the antigen (protein, mucin) and its expression pattern, a practice that gave rise to numerous categories and complexities. Examples include, viral proteins specifically associated with virus-induced malignancies (approximately 12% of all cancers),Citation12 and oncogene and tumor suppressor gene products or their mutant variants.Citation6 Oncofetal antigens and melanoma associated antigens represent those with restricted expression in non-malignant tissues,Citation13,14 whereas cancer testis antigens are only expressed, as the name suggests, in the tumor and in testes, with testes being protected by mechanisms of immune privilege.Citation15 Overexpressed antigens are a large and diverse group that includes any protein found at increased levels in tumors compared with normal healthy cells and tissues.
Recently, Coulie and colleagues classified tumor antigens as either high tumor specificity or low tumor specificity, each with two sub-categories; mutation (most tumors) or tumor-specific expression (many tumors) being associated with high tumor specificity, and tissue-specific expression (melanomas) or overexpression (some tumors) being associated with low tumor specifity.Citation16 The overall premise of this classification is whether or not the immune system (T cells in particular) recognizes the tumor antigen.
illustrates a simplified classification based on the immunologic character of the tumor antigen relative to its potential immunogenicity. Arguably tumor-specific antigens represent the obvious choice for vaccine targets, with no or limited expression in normal cells, inferring the potential for a stronger immune response without tolerance. Some examples of tumor-specific antigens include viral antigens (non-self), cancer testis antigens, and melanoma associated antigens (altered-self, with respect to limited expression in normal cells). Antigens from each of these groups are in the advanced stages of vaccine development. Tumor associated antigens (variable expression in normal cells) may represent a more obscure class of antigens, but ironically also represent the largest class of candidate vaccine target antigens. It is presumed that tumor associated antigens will induce a weaker immune response insufficient for tumor rejection, which may be why many have been understudied as vaccine targets. A subclass of overexpressed tumor associated antigens involved in the generation of oncogenesis and critical for maintaining the oncogenic phenotype may represent some of the most promising, widely applicable vaccine targets.
Figure 1. Immunologic character of tumor antigens. Simplified tumor antigen classification based on the immunologic character of the tumor antigen relative to its potential immunogenicity. Depicted are two main classes of antigens represented by tumor-specific and -associated antigens defined by normal cell expression, and four sub-classes ranging from stronger to weaker immunity. Non-self (e.g., viral proteins) and altered-self (e.g., mutant protein or restricted expression) antigens are proposed to elicit strong immune responses, and self antigens, whether involved in oncogenesis (tumor-dependent) or not (tumor-independent), would elicit weaker immune responses.
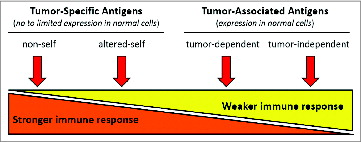
Overexpressed Oncogenic Tumor-Self Antigens
A National Cancer Institute sponsored project to prioritize cancer vaccine target antigens for translational research revealed that aberrantly expressed self-proteins represent the largest number of candidate antigens for vaccine development (nearly half of those categorized).Citation17 The study used preweighted objective criteria for prioritizing candidate tumor vaccine target antigens and selected 75 antigens for comparison and ranking (from hundreds considered). Nine criteria were used to rank the antigens. Therapeutic function was considered as the most important followed by immunogenicity, specificity, and oncogenicity. The relative weights of the remaining five criteria were orders of magnitude less than those applied to the top four.Citation17 Cellular localization of expression (internal or surface) carried the least weight of importance, but circulating antigen was determined to be not preferable. Therapeutic function data resulting from clinical trials were heavily considered in selecting the 75 antigens that were ranked.Citation17 Because the goal of the study was to accelerate translational (clinical) research, this bias was logical and acknowledged. However, some newer and potentially promising antigens were not ranked, largely because relevant studies were still in the pre-clinical phase. To summarize the conclusions, an ideal vaccine target antigen would be immunogenic, eliciting a response that eliminates tumor cells leaving normal cells unharmed (immunogenicity, therapeutic function, and specificity) and play a role in inducing or maintaining the oncogenic phenotype making the antigen indispensable to the tumor.
When considering the specificity criterion and focusing only on those antigens exhibiting aberrant expression (varying degrees of normal cell expression) 87% (65/75) of the ranked antigens can be classified as having some normal cell expression.Citation17 If post-translational modifications (e.g., the mucin, MUC1), and tissue specific (e.g., gp100), mixed (e.g., ALK), stromal (e.g., VEGFR2), and oncofetal (e.g., WT1) expression are excluded from the aberrant expression class, only overexpressed antigens remain, representing nearly 40% of the ranked antigens (28/75). If overexpressed antigens that do not play a role in oncogenesis are excluded from the list of 75, there are 9 overexpressed tumor-self antigens that are immunogenic and oncogenic (two criteria considered to be heavily weighted and important for an ideal tumor vaccine antigen).Citation17 In the following sections, we will briefly highlight several of the ranked overexpressed oncogenic tumor-self antigens, and end by focusing on perhaps the newest, widely shared overexpressed oncogenic tumor-self antigen, tumor protein D52 (TPD52).
Limited tumor expression
Androgen Receptor
The androgen receptor (AR) is a steroid receptor involved in prostate development, and a target for hormone deprivation therapy for advanced metastatic prostate cancer. Its function ascribes the AR with a role in prostate oncogenesis. AR is expressed in normal tissues with the prostate being a major site, and is widely overexpressed in prostate cancer, and in a subset of breast cancers.Citation18,19 Pre-existing AR-specific antibodies and T cells have been detected in prostate cancer patients, demonstrating immunogenicity and the potential for targeting the AR by vaccination.Citation20 The fine specificity of the AR-specific CTL responses in prostate cancer patients was defined by recognition of two HLA-A2-restricted peptides, AR805 and AR811, with the latter capable of eliciting specific CTLs following immunization of A2/DR1 transgenic mice.Citation21 An AR-based DNA vaccine targeting the AR ligand-binding domain (LBD) effectively induced CTLs responses in HHDII-DR1 (HLA-A2+ and HLA-DR1+) transgenic mice which were capable of lysing HLA-A2+ human prostate cancer cells.Citation22 In vivo prostate tumor regression was also observed in rats immunized with the AR-LBD DNA vaccine, supporting the potential for targeting the AR with vaccination.Citation22 These studies demonstrate that AR is immunogenic in patients and AR-based vaccines are capable of in vivo tumor rejection in pre-clinical animal models.
Shared tumor expression
We defined shared tumor expression as overexpression in multiple different cancers, but not as widely shared or potentially universal in tumor overexpression as that reported for human telomerase reverse transcriptase (hTERT) and survivin (discussed in the following section). The following are three examples of shared overexpressed oncogenic tumor-self antigens that are currently being evaluated in clinical trials as vaccine targets. Her-2/neu and p53 are perhaps the most commonly recognized and extensively studied TAAs of this category.
Her-2/neu
The transmembrane tyrosine kinase Her-2/neu is one of the most studied cancer proteins and therapeutic targets (nearly 1000 reviews). Her-2/neu is overexpressed in multiple cancers including lung, prostate and most notably breast cancer.Citation23 To date the majority of clinical vaccine trials have been conducted in patients with breast cancer.Citation24,25 Early evidence supporting Her-2/neu as a vaccine target came from the demonstration that specific CTLs exist in the peripheral blood of breast cancer patients.Citation26 Clinical trials evaluating vaccines targeting the intracellular and extracellular domains of Her-2/neu demonstrated the generation of specific T cells without the induction of autoimmunity and no significant toxicity, supporting the use of vaccines in the adjuvant setting.Citation27
p53
The important role played by p53 in cancers of multiple types has been recognized for decades.Citation28,29 CTLs have been generated against mutated and non-mutated epitopes of p53 without inducing autoimmunityCitation30 and pre-existing p53-specific CTLs have been demonstrated in cancer patients.Citation31,32 Clinical trials are underway evaluating therapeutic vaccines targeting p53 in ovarian cancer, colorectal cancer, and non-small cell lung cancer.Citation33-37 Overall, p53-specific immune responses have been observed in patients, but significant reductions in tumor burdens have not been demonstrated. It has been suggested that multiple epitope vaccines, Treg elimination, and/or CTLA-4 blockade should be assessed in combination with p53 vaccines to increase their clinical efficacy.Citation38
EphA2
EphA2 is a cell surface-expressed receptor tyrosine kinase and is one of 14 members of the Eph family (named for erythropoietin-producing hepatocellular carcinoma cell lines).Citation39 This family and its ligands play key roles in normal development and in tumorigenesis, where EphA2 is overexpressed in multiple cancers including brain malignancies, which have been the focus of most vaccine trials.Citation40 The vaccine potential of targeting EphA2 in gliomas was initially demonstrated by generating HLA-A2-restricted CTLs from the peripheral blood of HLA-A2+ normal donors and glioma patients using a single synthetic peptide (TLADFDPRV), and by vaccination of HHD mice with the same EphA2 peptide, demonstrating that tolerance could be broken by vaccination without inducing autoimmunity.Citation41 Subsequent studies in murine models of colon, liver and brain cancer further support the potential of EphA2 as a vaccine target.Citation42-44 Initial clinical trials testing EphA2 vaccines against adult and pediatric brain cancers have shown them to be safe, immunogenic and promising.Citation45,46
Widely shared (universal) tumor expression
There are but a few tumor associated antigens that appear to be universal for most if not all cancers, and these have shown promise as candidates for vaccine development. The two most studied universal tumor associated antigens are hTERT and the anti-apoptotic protein survivin. Neither of these antigens are specific for tumor cells, but both are over-active and/or aberrantly expressed in tumor cells compared with normal or non-malignant cells, and play a role in prolonging survival of tumor cells by preventing natural death mechanisms associated with proliferation such as telomere shortening and apoptosis. These attributes classify hTERT and survivin as shared overexpressed oncogenic tumor-self antigens.
hTERT
The activity of hTERT is a rate limiting step in the proliferative capacity of advanced cancers and represents a prototypical and universal cancer antigen and marker.Citation47,48 Variations in hTERT function in normal self-renewing tissues and tumors exist that can be taken advantage of for vaccine development.Citation49 hTERT was first characterized as a widely shared tumor associated antigen by demonstrating the induction of specific CTLs against more than 85% of human cancers, and by the identification of an HLA-A2 peptide vaccine candidate, 1540 (ILAKFLHWL).Citation50 Circulating 1540-specific CTLs were detected in nearly 91% of HLA-A2+ patients with 6 different cancers supporting vaccine development.Citation51 Additional CTL-specific hTERT-peptides include peptides restricted by additional HLAs.Citation52 A 16 amino acid hTERT peptide (GV1001) comprised of HLA class II and class I epitopes capable of inducing both CD4+ and CD8+ T cells when administered is also under investigation as a vaccine.Citation53 Several clinical trials assessing the efficacy of hTERT vaccines in patients with multiple cancers have been conducted or are underway, and although variations were reported in terms of tumor reductions, specific T cells were commonly induced in the majority of patients, with no adverse toxicities.Citation54-62
Survivin
Survivin is an inhibitor of apoptosis and acts upstream of the main effector proteases of apoptosis, caspase 3 and 7, and is active in a cell-cycle regulated manner during the G2/S phase, as a safeguard against activated cell death during successive rounds of cell division.Citation63,64 Like hTERT, survivin is expressed at low levels in normal, differentiated adult tissues but is overexpressed in cancers originating from a variety of tissues including lung, colon, breast, pancreas, and prostate cancer and several hematopoietic cancers.Citation65-68 The immunogenic potential of survivin was initially demonstrated by the induction of specific HLA-A2-restricted CD8+ cytotoxic T cell responses by dendritic cells that had processed and presented recombinant survivin protein.Citation69 CTLs specific for survivin were also detected in the peripheral blood of cancer patients supporting its potential as a vaccine target.Citation70 A survivin peptide vaccine administered with IFNα demonstrated efficacy and benefit for patients with advanced pancreatic cancer,Citation71 although contrasting clinical benefits were observed for similar vaccine approaches in in advanced melanoma patients.Citation72,73
Tumor protein D52
A third example of a new overexpressed oncogenic tumor self antigen with widely shared tumor expression is tumor protein D52 (TPD52) and like p53, survivin and hTERT is an intracellular protein. Early clinical support for the promise of TPD52 as a vaccine target antigen came from the identification of circulating serum antibodies with specificity for TPD52 in patients with breast cancer.Citation74,75
Expression of TPD52 in cancer
TPD52 is an overexpressed tumor self-protein actively involved in transformation, leading to increased proliferation and metastasis. TPD52 overexpression has been demonstrated in several human malignancies including breast,Citation76-78 prostate,Citation79-81 and ovarian carcinomas.Citation82 Expression microarray and other analyses predict TPD52 overexpression in many other cancers including multiple myeloma,Citation83,84 Burkitt's lymphoma,Citation85,86 pancreatic cancer,Citation87 testicular germ cell tumors,Citation88-90 and melanomas,Citation91,92 as well as multiple other adult and pediatric cancers.Citation93
Murine ortholog of TPD52
The murine ortholog of TPD52 (mD52) parallels normal tissue expression patterns and known functions of human TPD52 (hD52), with 86% amino acid identity.Citation94 mD52 induced anchorage independent growth and spontaneous lung metastasis when overexpressed in normal, non-tumorigenic cells.Citation95 Reduction of hD52 expression via RNAi resulted in increased apoptosis in human breast cancer cells, and hD52 overexpression was associated with decreased overall survival in human breast cancer patients.Citation96 These studies demonstrate that TPD52 overexpression is important for initiating and maintaining an oncogenic and metastatic phenotype and may be important for tumor cell survival. TPD52 is naturally expressed and involved in tumor formation and metastasis in human cells (hD52) and in mouse cells (mD52). This makes mD52 a unique and powerful overexpressed tumor-self antigen for study as a cancer vaccine target in murine models of cancer.
TPD52 as a vaccine target
The first demonstration that tumor protective immunity could be induced against TPD52 involved a recombinant protein-based mD52 vaccine that induced protection against tumor challenge when administered with CpG-ODN as a molecular adjuvant. mD52 protein administered without CpG-ODN failed to elicit an immune response, indicating that the TLR agonist was necessary to break tolerance.Citation97 Subcutaneous injection of mD52 protein with CpG-ODN required concomitant CD4+CD25+ T regulatory (Treg) cell depletion to improve tumor protection.Citation98 DNA-based vaccine approaches using the TRAMP model of prostate cancer demonstrated that mD52 DNA vaccination induced an immune response that prevented tumors with increased efficacy when administered with GM-CSF and induced long-term immunologic memory.Citation99 When mD52 DNA vaccination was compared head-to-head with hD52 DNA vaccination, the partial xeno-antigen (hD52) was more effective at protecting against tumor challenge, however both strategies induced durable responses that rejected secondary tumor challenge months later.Citation100 The T cell cytokine secretion patterns for all the TPD52 vaccine studies demonstrated that a TH-1-type cellular immune response was responsible for tumor rejectionCitation97-99 and that a complete response may be hindered by a potentially unique subset of CD8+ IL-10+ regulatory T cells.Citation100 An overlapping peptide-based mD52 vaccine, evaluated independently, demonstrated efficacy in a murine breast cancer model.Citation101 Important facts have been revealed by preclinical TPD52 vaccine studies to date (summarized in ). First, the successful use of the basic vaccine formulation demonstrated that a tumor self-protein can be immunogenic when delivered as a simple protein, peptides or plasmid DNA. Second, TPD52 vaccines prevent tumor formation without inducing autoimmunity,Citation97 even when classical CD4+ CD25+ Treg cells were depleted.Citation98,100 These studies suggest that TPD52-specific T cells are present and not completely eliminated by central tolerance, and that peripheral tolerance is involved in obstructing complete tumor rejection to include suppression by an as yet undefined but potentially unique subset of CD8+ Treg cells.Citation100 An additional note-worthy observation from our preclinical vaccine studies is that DNA-based vaccines (most notably xenogeneic hD52 DNA) appear to be more potent and effective suggesting that TLR-9 plays a role as a molecular adjuvant. This is supported by the requirement for the inclusion of CpG ODN with recombinant protein to induce protective immunity.
Table 1. TPD52 vaccines in murine models of sarcoma and prostate cancer
As a first step to human studies and eventual clinical trials, we generated CTLs specific for hD52 from the peripheral blood of an HLA-A2+ male normal donor by in vitro stimulation with a synthetic peptide (QLFHSFSV; modified at P2 and P9 to increase affinity for HLA-A2) derived from the amino acid sequence of hD52 using established protocols.Citation102 These CTLs only lysed HLA-A2+ prostate cancer cell lines that naturally overexpressed hD52 ().Citation103 This initial experiment further supports the potential use of TPD52 as a vaccine target in humans.
Figure 2. Generation of CTLs from normal PBLs with D52 peptide. The data show hD52-specific HLA-A2-restricted killing of human prostate cell lines. hD52 expression in the human prostate cell lines was determined by 30 cycle RT-PCR using hD52-specific primers and GAPDH as an internal reference. (A) Human normal prostate cell line 568 NPTX and tumor cell line LnCap are hD52-low expressors. (B) Killing of human prostate cancer cell lines determined using a standard lysis assay at an E:T of 5:1. Targets: human prostate cancer and normal-derived cells from HLA-A2+ patient 568 (normal = NPTX, tumor = CP1TX and CP2TX) were generated as previously described,Citation103 human prostate cancer cell lines PC-3 (HLA-A2-) and LnCap (HLA-A2+) are commercially available. Methods: The method used to generate hD52 peptide-specific CTLs by IVS with hD52 peptide Q(A/L)FSHSFS(I/V) has been previously explained in detail.Citation102
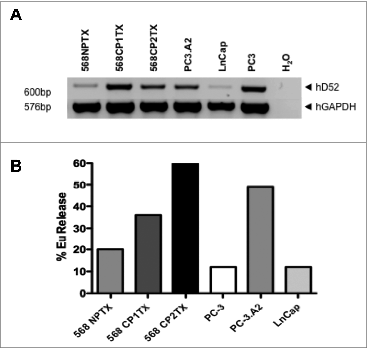
Concluding Remarks
Employing the immune system to fight cancer has long been viewed as promising, as is evidenced by the extensive list of publications attesting to the hard work of many. This promise is now being realized by FDA approval of two of the newest treatments for prostate cancer and melanoma, Sipuleucel T and CTLA-4 blockade.Citation3,8 Both of these immunotherapies rely on eliciting T cell-mediated immunity which requires antigen recognition and specificity. Sipuleucel T is a therapeutic vaccine, and CTLA-4 (T cell checkpoint) blockade is being explored in combination with vaccination.Citation104 Most vaccine trials focus on patients with advanced cancer (therapeutic vaccines) and have largely produced disappointing results.Citation8 Historically vaccines have been successful only when applied to prevent disease. Perhaps immunization to prevent primary cancer, recurrence and/or metastasis as opposed to eliminating already existing primary and metastatic tumors is the next step in cancer vaccine development.
Regardless of the application, cancer vaccines are only as good as the antigens they target. In this context it is logical to argue that the antigen content of the vaccine formulation is more important than the delivery vehicle. A review of the literature will reveal that much of the past vaccine development work has focused on generating new delivery vehicles and formulations to make a few well-studied antigens more potent in murine models of cancer and clinical trials. This focus on a minority of antigens is also true for the study of oncogenes in general.Citation93 The recent prioritization of vaccine antigens for translational studies supports the notion that it is time to find new, better antigens on which to focus vaccine efforts.Citation16
What constitutes an ideal cancer vaccine antigen? Many would argue, and logically so, that completely foreign (e.g., viral) non-self proteins would be the most immunogenic () and therefore effective. Much of the early preclinical experimental literature and the FDA approval of preventive cervical cancer vaccines would be in agreement.Citation7 Unfortunately most clinically significant cancers are not (or at least have yet) to be associated with viruses. This truth led to the TAA discovery movement and the development of various technologies for its accomplishment.Citation6 This produced many candidate vaccine antigens that with further effort, could yield ideal targets for the next generation of cancer vaccines, among them being the overexpressed tumor-self antigens. This large group of understudied vaccine targets represents (as the name implies) self proteins with limited or low but detectable expression in many normal healthy cells and tissues and overexpression in multiple to most cancers. A subset of this group comprises those with the added desirable feature of being involved in oncogenesis, making their expression indispensible to the tumor.Citation17 Preclinical murine models of cancer have been critical for development of this group of antigens as vaccine targets, and for demonstrating that these self proteins are immunogenic and thus capable of eliciting immunity that will kill tumors and not healthy cells (no autoimmunity). Perhaps the newest promising overexpressed oncogenic tumor-self antigen is the oncoprotein TPD52.Citation93
Disclosure of Potential Conflicts of Interest
No potential conflicts of interest were disclosed.
Funding
This work was supported by a grant from the DOD CDMRP PCRP: Award Number W81XWH-08–1-0660; and by funds from Texas Tech University Health Sciences Center.
References
- American Cancer Society. Cancer Facts & Figures 2013. Atlanta: American Cancer Society; 2013.
- Mellman I, Coukos G, Dranoff G. Cancer immunotherapy comes of age. Nature 2011; 480:480-9; PMID:22193102; http://dx.doi.org/10.1038/nature10673
- Couzin-Frankel J. Breakthrough of the year 2013. Cancer immunotherapy. Science 2013; 342:1432-3; PMID:24357284; http://dx.doi.org/10.1126/science.342.6165.1432
- Weber JS, Kähler KC, Hauschild A. Management of immune-related adverse events and kinetics of response with ipilimumab. J Clin Oncol 2012; 30:2691-7; PMID:22614989; http://dx.doi.org/10.1200/JCO.2012.41.6750
- Restifo NP, Dudley ME, Rosenberg SA. Adoptive immunotherapy for cancer: harnessing the T cell response. Nat Rev Immunol 2012; 12:269-81; PMID:22437939; http://dx.doi.org/10.1038/nri3191
- Lewis JD, Reilly BD, Bright RK. Tumor-associated antigens: from discovery to immunity. Int Rev Immunol 2003; 22:81-112; PMID:12962271; http://dx.doi.org/10.1080/08830180305221
- Schiller JT, Castellsagué X, Garland SM. A review of clinical trials of human papillomavirus prophylactic vaccines. Vaccine 2012; 30(Suppl 5):F123-38; PMID:23199956; http://dx.doi.org/10.1016/j.vaccine.2012.04.108
- Ogi C, Aruga A. Clinical evaluation of therapeutic cancer vaccines. Hum Vaccin Immunother 2013; 9:1049-57; PMID:23454867; http://dx.doi.org/10.4161/hv.23917
- Weiner LM, Surana R, Murray J. Vaccine prevention of cancer: can endogenous antigens be targeted? Cancer Prev Res (Phila) 2010; 3:410-5; PMID:20332297; http://dx.doi.org/10.1158/1940-6207.CAPR-10-0040
- Michael A, Relph K, Annels N, Pandha H. Prostate cancer vaccines. Expert Rev Vaccines 2013; 12:253-62; PMID:23496665; http://dx.doi.org/10.1586/erv.13.27
- Aranda F, Vacchelli E, Eggermont A, Galon J, Sautès-Fridman C, Tartour E, Zitvogel L, Kroemer G, Galluzzi L. Trial Watch: Peptide vaccines in cancer therapy. Oncoimmunology 2013; 2:e26621; PMID:24498550; http://dx.doi.org/10.4161/onci.26621
- Mesri EA, Feitelson MA, Munger K. Human viral oncogenesis: a cancer hallmarks analysis. Cell Host Microbe 2014; 15:266-82; PMID:24629334; http://dx.doi.org/10.1016/j.chom.2014.02.011
- Coggin JH Jr., Barsoum AL, Rohrer JW, Thurnher M, Zeis M. Contemporary definitions of tumor specific antigens, immunogens and markers as related to the adaptive responses of the cancer-bearing host. Anticancer Res 2005; 25(3c):2345-55; PMID:16080461
- Yang JC. The adoptive transfer of cultured T cells for patients with metastatic melanoma. Clin Dermatol 2013; 31:209-19; PMID:23438384; http://dx.doi.org/10.1016/j.clindermatol.2012.08.019
- Kulkarni P, Shiraishi T, Rajagopalan K, Kim R, Mooney SM, Getzenberg RH. Cancer/testis antigens and urological malignancies. Nat Rev Urol 2012; 9:386-96; PMID:22710665; http://dx.doi.org/10.1038/nrurol.2012.117
- Coulie PG, Van den Eynde BJ, van der Bruggen P, Boon T. Tumour antigens recognized by T lymphocytes: at the core of cancer immunotherapy. Nat Rev Cancer 2014; 14:135-46; PMID:24457417; http://dx.doi.org/10.1038/nrc3670
- Cheever MA, Allison JP, Ferris AS, Finn OJ, Hastings BM, Hecht TT, Mellman I, Prindiville SA, Viner JL, Weiner LM, et al. The prioritization of cancer antigens: a national cancer institute pilot project for the acceleration of translational research. Clin Cancer Res 2009; 15:5323-37; PMID:19723653; http://dx.doi.org/10.1158/1078-0432.CCR-09-0737
- Augello MA, Den RB, Knudsen KE. AR function in promoting metastatic prostate cancer. [epub ahead of print]. Cancer Metastasis Rev 2014; PMID:24425228; http://dx.doi.org/10.1007/s10555-013-9471-3
- McNamara KM, Yoda T, Nurani AM, Shibahara Y, Miki Y, Wang L, Nakamura Y, Suzuki K, Yang Y, Abe E, et al. Androgenic pathways in the progression of triple-negative breast carcinoma: a comparison between aggressive and non-aggressive subtypes. [epub ahead of print]. Breast Cancer Res Treat 2014; 145:281-93; PMID:24715382; http://dx.doi.org/10.1007/s10549-014-2942-6
- Olson BM, McNeel DG. Antibody and T-cell responses specific for the androgen receptor in patients with prostate cancer. Prostate 2007; 67:1729-39; PMID:17879963; http://dx.doi.org/10.1002/pros.20652
- Olson BM, McNeel DG. CD8+ T cells specific for the androgen receptor are common in patients with prostate cancer and are able to lyse prostate tumor cells. Cancer Immunol Immunother 2011; 60:781-92; PMID:21350948; http://dx.doi.org/10.1007/s00262-011-0987-5
- Olson BM, Johnson LE, McNeel DG. The androgen receptor: a biologically relevant vaccine target for the treatment of prostate cancer. Cancer Immunol Immunother 2013; 62:585-96; PMID:23108626; http://dx.doi.org/10.1007/s00262-012-1363-9
- Seliger B, Kono K, Rongcun Y, Kiessling R. Cytotoxic T cell epitopes and tissue distribution of the HER-2/neu proto-oncogene: Implications for vaccine development. In W.M. Kast (Ed.) Peptide-based Cancer Vaccines. Georgetown, TX: Eurekah.com & Landes Bioscience, 2000.
- Disis ML, Cheever MA. Clinical trials of HER-2/neu peptide-based vaccines. In W.M. Kast (Ed.) Peptide-based Cancer Vaccines. Georgetown, Texas: Eurekah.com & Landes Bioscience, 2000.
- Wiedermann U, Davis AB, Zielinski CC. Vaccination for the prevention and treatment of breast cancer with special focus on Her-2/neu peptide vaccines. Breast Cancer Res Treat 2013; 138:1-12; PMID:23340862; http://dx.doi.org/10.1007/s10549-013-2410-8
- Linehan DC, Goedegebuure PS, Peoples GE, Rogers SO, Eberlein TJ. Tumor-specific and HLA-A2-restricted cytolysis by tumor-associated lymphocytes in human metastatic breast cancer. J Immunol 1995; 155:4486-91; PMID:7594611
- Baxevanis CN, Voutsas IF, Gritzapis AD, Perez SA, Papamichail M. HER-2/neu as a target for cancer vaccines. Immunotherapy 2010; 2:213-26; PMID:20635929; http://dx.doi.org/10.2217/imt.09.89
- Levine AJ. p53, the cellular gatekeeper for growth and division. Cell 1997; 88:323-31; PMID:9039259; http://dx.doi.org/10.1016/S0092-8674(00)81871-1
- Hollstein M, Sidransky D, Vogelstein B, Harris CC. p53 mutations in human cancers. Science 1991; 253:49-53; PMID:1905840; http://dx.doi.org/10.1126/science.1905840
- Vierboom MPM, Nijman HW, Offringa R, van der Voort EI, van Hall T, van den Broek L, Fleuren GJ, Kenemans P, Kast WM, Melief CJ. Tumor eradication by wild-type p53-specific cytotoxic T lymphocytes. J Exp Med 1997; 186:695-704; PMID:9271585; http://dx.doi.org/10.1084/jem.186.5.695
- Hoffmann TK, Donnenberg AD, Finkelstein SD, Donnenberg VS, Friebe-Hoffmann U, Myers EN, Appella E, DeLeo AB, Whiteside TL. Frequencies of tetramer+ T cells specific for the wild-type sequence p53(264-272) peptide in the circulation of patients with head and neck cancer. Cancer Res 2002; 62:3521-9; PMID:12067999
- Pedersen AE, Stryhn A, Justesen S, Harndahl M, Rasmussen S, Donskov F, Claesson MH, Pedersen JW, Wandall HH, Svane IM, et al. Wildtype p53-specific antibody and T-cell responses in cancer patients. J Immunother 2011; 34:629-40; PMID:21989411; http://dx.doi.org/10.1097/CJI.0b013e3182281381
- Leffers N, Vermeij R, Hoogeboom BN, Schulze UR, Wolf R, Hamming IE, van der Zee AG, Melief KJ, van der Burg SH, Daemen T, et al. Long-term clinical and immunological effects of p53-SLP® vaccine in patients with ovarian cancer. Int J Cancer 2012; 130:105-12; PMID:21328579; http://dx.doi.org/10.1002/ijc.25980
- Rahma OE, Ashtar E, Czystowska M, Szajnik ME, Wieckowski E, Bernstein S, Herrin VE, Shams MA, Steinberg SM, Merino M, et al. A gynecologic oncology group phase II trial of two p53 peptide vaccine approaches: subcutaneous injection and intravenous pulsed dendritic cells in high recurrence risk ovarian cancer patients. Cancer Immunol Immunother 2012; 61:373-84; PMID:21927947; http://dx.doi.org/10.1007/s00262-011-1100-9
- Zeestraten EC, Speetjens FM, Welters MJ, Saadatmand S, Stynenbosch LF, Jongen R, Kapiteijn E, Gelderblom H, Nijman HW, Valentijn AR, et al. Addition of interferon-α to the p53-SLP® vaccine results in increased production of interferon-γ in vaccinated colorectal cancer patients: a phase I/II clinical trial. Int J Cancer 2013; 132:1581-91; PMID:22948952; http://dx.doi.org/10.1002/ijc.27819
- Hall RD, Gray JE, Chiappori AA. Beyond the standard of care: a review of novel immunotherapy trials for the treatment of lung cancer. Cancer Control 2013; 20:22-31; PMID:23302904
- Iclozan C, Antonia S, Chiappori A, Chen DT, Gabrilovich D. Therapeutic regulation of myeloid-derived suppressor cells and immune response to cancer vaccine in patients with extensive stage small cell lung cancer. Cancer Immunol Immunother 2013; 62:909-18; PMID:23589106; http://dx.doi.org/10.1007/s00262-013-1396-8
- Vermeij R, Leffers N, Melief CJ, Daemen T, Nijman HW. Antigen-specific immunotherapy in ovarian cancer and p53 as tumor antigen. Curr Pharm Des 2012; 18:3804-11; PMID:22591425; http://dx.doi.org/10.2174/138161212802002805
- Park JE, Son AI, Zhou R. Roles of EphA2 in development and disease. Genes (Basel) 2013; 4:334-57; PMID:24705208; http://dx.doi.org/10.3390/genes4030334
- Wykosky J, Debinski W. The EphA2 receptor and ephrinA1 ligand in solid tumors: function and therapeutic targeting. Mol Cancer Res 2008; 6:1795-806; PMID:19074825; http://dx.doi.org/10.1158/1541-7786.MCR-08-0244
- Hatano M, Eguchi J, Tatsumi T, Kuwashima N, Dusak JE, Kinch MS, Pollack IF, Hamilton RL, Storkus WJ, Okada H. EphA2 as a glioma-associated antigen: a novel target for glioma vaccines. Neoplasia 2005; 7:717-22; PMID:16207473; http://dx.doi.org/10.1593/neo.05277
- Yamaguchi S, Tatsumi T, Takehara T, Sakamori R, Uemura A, Mizushima T, Ohkawa K, Storkus WJ, Hayashi N. Immunotherapy of murine colon cancer using receptor tyrosine kinase EphA2-derived peptide-pulsed dendritic cell vaccines. Cancer 2007; 110:1469-77; PMID:17685394; http://dx.doi.org/10.1002/cncr.22958
- Yamaguchi S, Tatsumi T, Takehara T, Sasakawa A, Hikita H, Kohga K, Uemura A, Sakamori R, Ohkawa K, Hayashi N. Dendritic cell-based vaccines suppress metastatic liver tumor via activation of local innate and acquired immunity. Cancer Immunol Immunother 2008; 57:1861-9; PMID:18438665; http://dx.doi.org/10.1007/s00262-008-0514-5
- Ueda R, Fujita M, Zhu X, Sasaki K, Kastenhuber ER, Kohanbash G, McDonald HA, Harper J, Lonning S, Okada H. Systemic inhibition of transforming growth factor-beta in glioma-bearing mice improves the therapeutic efficacy of glioma-associated antigen peptide vaccines. Clin Cancer Res 2009; 15:6551-9; PMID:19861464; http://dx.doi.org/10.1158/1078-0432.CCR-09-1067
- Yeung JT, Hamilton RL, Okada H, Jakacki RI, Pollack IF. Increased expression of tumor-associated antigens in pediatric and adult ependymomas: implication for vaccine therapy. J Neurooncol 2013; 111:103-11; PMID:23179498; http://dx.doi.org/10.1007/s11060-012-0998-x
- Okada H, Kalinski P, Ueda R, Hoji A, Kohanbash G, Donegan TE, Mintz AH, Engh JA, Bartlett DL, Brown CK, et al. Induction of CD8+ T-cell responses against novel glioma-associated antigen peptides and clinical activity by vaccinations with alpha-type 1 polarized dendritic cells and polyinosinic-polycytidylic acid stabilized by lysine and carboxymethylcellulose in patients with recurrent malignant glioma. J Clin Oncol 2011; 29:330-6; PMID:21149657; http://dx.doi.org/10.1200/JCO.2010.30.7744
- Bodnar AG, Ouellette M, Frolkis M, Holt SE, Chiu CP, Morin GB, Harley CB, Shay JW, Lichtsteiner S, Wright WE. Extension of life-span by introduction of telomerase into normal human cells. Science 1998; 279:349-52; PMID:9454332; http://dx.doi.org/10.1126/science.279.5349.349
- Meyerson M, Counter CM, Eaton EN, Ellisen LW, Steiner P, Caddle SD, Ziaugra L, Beijersbergen RL, Davidoff MJ, Liu Q, et al. hEST2, the putative human telomerase catalytic subunit gene, is up-regulated in tumor cells and during immortalization. Cell 1997; 90:785-95; PMID:9288757; http://dx.doi.org/10.1016/S0092-8674(00)80538-3
- Beatty GL, Vonderheide RH. Telomerase as a universal tumor antigen for cancer vaccines. Expert Rev Vaccines 2008; 7:881-7; PMID:18767939; http://dx.doi.org/10.1586/14760584.7.7.881
- Vonderheide RH, Hahn WC, Schultze JL, Nadler LM. The telomerase catalytic subunit is a widely expressed tumor-associated antigen recognized by cytotoxic T lymphocytes. Immunity 1999; 10:673-9; PMID:10403642; http://dx.doi.org/10.1016/S1074-7613(00)80066-7
- Filaci G, Fravega M, Setti M, Traverso P, Millo E, Fenoglio D, Negrini S, Ferrera F, Romagnoli A, Basso M, et al. Frequency of telomerase-specific CD8+ T lymphocytes in patients with cancer. Blood 2006; 107:1505-12; PMID:16249379; http://dx.doi.org/10.1182/blood-2005-01-0258
- Carpenter EL, Vonderheide RH. Telomerase-based immunotherapy of cancer. Expert Opin Biol Ther 2006; 6:1031-9; PMID:16989585; http://dx.doi.org/10.1517/14712598.6.10.1031
- Kyte JA. Cancer vaccination with telomerase peptide GV1001. Expert Opin Investig Drugs 2009; 18:687-94; PMID:19388882; http://dx.doi.org/10.1517/13543780902897631
- Vonderheide RH, Domchek SM, Schultze JL, George DJ, Hoar KM, Chen DY, Stephans KF, Masutomi K, Loda M, Xia Z, et al. Vaccination of cancer patients against telomerase induces functional antitumor CD8+ T lymphocytes. Clin Cancer Res 2004; 10:828-39; PMID:14871958; http://dx.doi.org/10.1158/1078-0432.CCR-0620-3
- Immunological and Clinical Outcomes following telomerase peptide vaccination in patients with metastatic breast cancer. Proc. Am. As. Cancer Res 2006; 4003a
- Brunsvig PF, Aamdal S, Gjertsen MK, Kvalheim G, Markowski-Grimsrud CJ, Sve I, Dyrhaug M, Trachsel S, Møller M, Eriksen JA, et al. Telomerase peptide vaccination: a phase I/II study in patients with non-small cell lung cancer. Cancer Immunol Immunother 2006; 55:1553-64; PMID:16491401; http://dx.doi.org/10.1007/s00262-006-0145-7
- Su Z, Dannull J, Yang BK, Dahm P, Coleman D, Yancey D, Sichi S, Niedzwiecki D, Boczkowski D, Gilboa E, et al. Telomerase mRNA-transfected dendritic cells stimulate antigen-specific CD8+ and CD4+ T cell responses in patients with metastatic prostate cancer. J Immunol 2005; 174:3798-807; PMID:15749921; http://dx.doi.org/10.4049/jimmunol.174.6.3798
- Brunsvig PF, Kyte JA, Kersten C, Sundstrøm S, Møller M, Nyakas M, Hansen GL, Gaudernack G, Aamdal S. Telomerase peptide vaccination in NSCLC: a phase II trial in stage III patients vaccinated after chemoradiotherapy and an 8-year update on a phase I/II trial. Clin Cancer Res 2011; 17:6847-57; PMID:21918169; http://dx.doi.org/10.1158/1078-0432.CCR-11-1385
- Hunger RE, Kernland Lang K, Markowski CJ, Trachsel S, Møller M, Eriksen JA, Rasmussen AM, Braathen LR, Gaudernack G. Vaccination of patients with cutaneous melanoma with telomerase-specific peptides. Cancer Immunol Immunother 2011; 60:1553-64; PMID:21681371; http://dx.doi.org/10.1007/s00262-011-1061-z
- Inderberg-Suso EM, Trachsel S, Lislerud K, Rasmussen AM, Gaudernack G. Widespread CD4+ T-cell reactivity to novel hTERT epitopes following vaccination of cancer patients with a single hTERT peptide GV1001. Oncoimmunology 2012; 1:670-86; PMID:22934259; http://dx.doi.org/10.4161/onci.20426
- Fenoglio D, Traverso P, Parodi A, Tomasello L, Negrini S, Kalli F, Battaglia F, Ferrera F, Sciallero S, Murdaca G, et al. A multi-peptide, dual-adjuvant telomerase vaccine (GX301) is highly immunogenic in patients with prostate and renal cancer. Cancer Immunol Immunother 2013; 62:1041-52; PMID:23591981; http://dx.doi.org/10.1007/s00262-013-1415-9
- Vetsika EK, Konsolakis G, Aggouraki D, Kotsakis A, Papadimitraki E, Christou S, Menez-Jamet J, Kosmatopoulos K, Georgoulias V, Mavroudis D. Immunological responses in cancer patients after vaccination with the therapeutic telomerase-specific vaccine Vx-001. Cancer Immunol Immunother 2012; 61:157-68; PMID:21858533; http://dx.doi.org/10.1007/s00262-011-1093-4
- LaCasse EC, Baird S, Korneluk RG, MacKenzie AE. The inhibitors of apoptosis (IAPs) and their emerging role in cancer. Oncogene 1998; 17:3247-59; PMID:9916987; http://dx.doi.org/10.1038/sj.onc.1202569
- Stauber RH, Mann W, Knauer SK. Nuclear and cytoplasmic survivin: molecular mechanism, prognostic, and therapeutic potential. Cancer Res 2007; 67:5999-6002; PMID:17616652; http://dx.doi.org/10.1158/0008-5472.CAN-07-0494
- Adida C, Haioun C, Gaulard P, Lepage E, Morel P, Briere J, Dombret H, Reyes F, Diebold J, Gisselbrecht C, et al. Prognostic significance of survivin expression in diffuse large B-cell lymphomas. Blood 2000; 96:1921-5; PMID:10961895
- Schmidt SM, Schag K, Müller MR, Weck MM, Appel S, Kanz L, Grünebach F, Brossart P. Survivin is a shared tumor-associated antigen expressed in a broad variety of malignancies and recognized by specific cytotoxic T cells. Blood 2003; 102:571-6; PMID:12576330; http://dx.doi.org/10.1182/blood-2002-08-2554
- Andersen MH, thor SP. Survivin–a universal tumor antigen. Histol Histopathol 2002; 17:669-75; PMID:11962766
- Andersen MH, Svane IM, Becker JC, Straten PT. The universal character of the tumor-associated antigen survivin. Clin Cancer Res 2007; 13:5991-4; PMID:17947459; http://dx.doi.org/10.1158/1078-0432.CCR-07-0686
- Schmitz M, Diestelkoetter P, Weigle B, Schmachtenberg F, Stevanovic S, Ockert D, Rammensee HG, Rieber EP. Generation of survivin-specific CD8+ T effector cells by dendritic cells pulsed with protein or selected peptides. Cancer Res 2000; 60:4845-9; PMID:10987296
- Andersen MH, Pedersen LO, Becker JC, Straten PT. Identification of a cytotoxic T lymphocyte response to the apoptosis inhibitor protein survivin in cancer patients. Cancer Res 2001; 61:869-72; PMID:11221872
- Kameshima H, Tsuruma T, Kutomi G, Shima H, Iwayama Y, Kimura Y, Imamura M, Torigoe T, Takahashi A, Hirohashi Y, et al. Immunotherapeutic benefit of α-interferon (IFNα) in survivin2B-derived peptide vaccination for advanced pancreatic cancer patients. Cancer Sci 2013; 104:124-9; PMID:23078230; http://dx.doi.org/10.1111/cas.12046
- Filipazzi P, Pilla L, Mariani L, Patuzzo R, Castelli C, Camisaschi C, Maurichi A, Cova A, Rigamonti G, Giardino F, et al. Limited induction of tumor cross-reactive T cells without a measurable clinical benefit in early melanoma patients vaccinated with human leukocyte antigen class I-modified peptides. Clin Cancer Res 2012; 18:6485-96; PMID:23032742; http://dx.doi.org/10.1158/1078-0432.CCR-12-1516
- Becker JC, Andersen MH, Hofmeister-Müller V, Wobser M, Frey L, Sandig C, Walter S, Singh-Jasuja H, Kämpgen E, Opitz A, et al. Survivin-specific T-cell reactivity correlates with tumor response and patient survival: a phase-II peptide vaccination trial in metastatic melanoma. Cancer Immunol Immunother 2012; 61:2091-103; PMID:22565484; http://dx.doi.org/10.1007/s00262-012-1266-9
- Scanlan MJ, Gout I, Gordon CM, Williamson B, Stockert E, Gure AO, Jäger D, Chen YT, Mackay A, O’Hare MJ, et al. Humoral immunity to human breast cancer: antigen definition and quantitative analysis of mRNA expression. Cancer Immun 2001; 1:4; PMID:12747765
- Anderson KS, Sibani S, Wallstrom G, Qiu J, Mendoza EA, Raphael J, Hainsworth E, Montor WR, Wong J, Park JG, et al. Protein microarray signature of autoantibody biomarkers for the early detection of breast cancer. J Proteome Res 2011; 10:85-96; PMID:20977275; http://dx.doi.org/10.1021/pr100686b
- Balleine RL, Fejzo MS, Sathasivam P, Basset P, Clarke CL, Byrne JA. The hD52 (TPD52) gene is a candidate target gene for events resulting in increased 8q21 copy number in human breast carcinoma. Genes Chromosomes Cancer 2000; 29:48-57; PMID:10918393; http://dx.doi.org/10.1002/1098-2264(2000)9999:9999≤::AID-GCC1005≥3.0.CO;2-O
- Byrne JA, Tomasetto C, Garnier JM, Rouyer N, Mattei MG, Bellocq JP, Rio MC, Basset P. A screening method to identify genes commonly overexpressed in carcinomas and the identification of a novel complementary DNA sequence. Cancer Res 1995; 55:2896-903; PMID:7796418
- Pollack JR, Sørlie T, Perou CM, Rees CA, Jeffrey SS, Lonning PE, Tibshirani R, Botstein D, Børresen-Dale AL, Brown PO. Microarray analysis reveals a major direct role of DNA copy number alteration in the transcriptional program of human breast tumors. Proc Natl Acad Sci U S A 2002; 99:12963-8; PMID:12297621; http://dx.doi.org/10.1073/pnas.162471999
- Wang R, Xu J, Saramäki O, Visakorpi T, Sutherland WM, Zhou J, Sen B, Lim SD, Mabjeesh N, Amin M, et al. PrLZ, a novel prostate-specific and androgen-responsive gene of the TPD52 family, amplified in chromosome 8q21.1 and overexpressed in human prostate cancer. Cancer Res 2004; 64:1589-94; PMID:14996714; http://dx.doi.org/10.1158/0008-5472.CAN-03-3331
- Wang R, Xu J, Mabjeesh N, Zhu G, Zhou J, Amin M, He D, Marshall FF, Zhau HE, Chung LW. PrLZ is expressed in normal prostate development and in human prostate cancer progression. Clin Cancer Res 2007; 13:6040-8; PMID:17947466; http://dx.doi.org/10.1158/1078-0432.CCR-07-0640
- Rubin MA, Varambally S, Beroukhim R, Tomlins SA, Rhodes DR, Paris PL, Hofer MD, Storz-Schweizer M, Kuefer R, Fletcher JA, et al. Overexpression, amplification, and androgen regulation of TPD52 in prostate cancer. Cancer Res 2004; 64:3814-22; PMID:15172988; http://dx.doi.org/10.1158/0008-5472.CAN-03-3881
- Byrne JA, Balleine RL, Schoenberg Fejzo M, Mercieca J, Chiew YE, Livnat Y, St Heaps L, Peters GB, Byth K, Karlan BY, et al. Tumor protein D52 (TPD52) is overexpressed and a gene amplification target in ovarian cancer. Int J Cancer 2005; 117:1049-54; PMID:15986428; http://dx.doi.org/10.1002/ijc.21250
- Largo C, Alvarez S, Saez B, Blesa D, Martin-Subero JI, González-García I, Brieva JA, Dopazo J, Siebert R, Calasanz MJ, et al. Identification of overexpressed genes in frequently gained/amplified chromosome regions in multiple myeloma. Haematologica 2006; 91:184-91; PMID:16461302
- Tiacci E, Orvietani PL, Bigerna B, Pucciarini A, Corthals GL, Pettirossi V, Martelli MP, Liso A, Benedetti R, Pacini R, et al. Tumor protein D52 (TPD52): a novel B-cell/plasma-cell molecule with unique expression pattern and Ca(2+)-dependent association with annexin VI. Blood 2005; 105:2812-20; PMID:15576473; http://dx.doi.org/10.1182/blood-2004-07-2630
- Dave SS, Fu K, Wright GW, Lam LT, Kluin P, Boerma EJ, Greiner TC, Weisenburger DD, Rosenwald A, Ott G, et al.; Lymphoma/Leukemia Molecular Profiling Project. Molecular diagnosis of Burkitt's lymphoma. N Engl J Med 2006; 354:2431-42; PMID:16760443; http://dx.doi.org/10.1056/NEJMoa055759
- Hummel M, Bentink S, Berger H, Klapper W, Wessendorf S, Barth TF, Bernd HW, Cogliatti SB, Dierlamm J, Feller AC, et al.; Molecular Mechanisms in Malignant Lymphomas Network Project of the Deutsche Krebshilfe. A biologic definition of Burkitt's lymphoma from transcriptional and genomic profiling. N Engl J Med 2006; 354:2419-30; PMID:16760442; http://dx.doi.org/10.1056/NEJMoa055351
- Loukopoulos P, Shibata T, Katoh H, Kokubu A, Sakamoto M, Yamazaki K, Kosuge T, Kanai Y, Hosoda F, Imoto I, et al. Genome-wide array-based comparative genomic hybridization analysis of pancreatic adenocarcinoma: identification of genetic indicators that predict patient outcome. Cancer Sci 2007; 98:392-400; PMID:17233815; http://dx.doi.org/10.1111/j.1349-7006.2007.00395.x
- Skotheim RI, Autio R, Lind GE, Kraggerud SM, Andrews PW, Monni O, Kallioniemi O, Lothe RA. Novel genomic aberrations in testicular germ cell tumors by array-CGH, and associated gene expression changes. Cell Oncol 2006; 28:315-26; PMID:17167184
- Korkola JE, Heck S, Olshen AB, Reuter VE, Bosl GJ, Houldsworth J, Chaganti RS. In vivo differentiation and genomic evolution in adult male germ cell tumors. Genes Chromosomes Cancer 2008; 47:43-55; PMID:17943972; http://dx.doi.org/10.1002/gcc.20504
- McIntyre A, Summersgill B, Lu YJ, Missiaglia E, Kitazawa S, Oosterhuis JW, Looijenga LH, Shipley J. Genomic copy number and expression patterns in testicular germ cell tumours. Br J Cancer 2007; 97:1707-12; PMID:18059402; http://dx.doi.org/10.1038/sj.bjc.6604079
- Hoek KS. DNA microarray analyses of melanoma gene expression: a decade in the mines. Pigment Cell Res 2007; 20:466-84; PMID:17935490; http://dx.doi.org/10.1111/j.1600-0749.2007.00412.x
- Roesch A, Becker B, Bentink S, Spang R, Vogl A, Hagen I, Landthaler M, Vogt T. Ataxia telangiectasia-mutated gene is a possible biomarker for discrimination of infiltrative deep penetrating nevi and metastatic vertical growth phase melanoma. Cancer Epidemiol Biomarkers Prev 2007; 16:2486-90; PMID:18006941; http://dx.doi.org/10.1158/1055-9965.EPI-07-0224
- Byrne JA, Frost S, Chen Y, Bright RK. Tumor protein D52 (TPD52) and cancer-oncogene understudy or understudied oncogene? Tumour Biol 2014; 35(8):7369-82 PMID:24798974; http://dx.doi.org/10.1007/s13277-014-2006-x
- Byrne JA, Mattei MG, Basset P. Definition of the D52 gene/protein family through cloning of D52 homologues in human (hD53) and mouse (mD52). Genomics 1996; 35:523-32; PMID:8812487; http://dx.doi.org/10.1006/geno.1996.0393
- Lewis JD, Payton LA, Whitford JG, Byrne JA, Smith DI, Yang L, Bright RK. Induction of tumorigenesis and metastasis by the murine orthologue of tumor protein D52. Mol Cancer Res 2007; 5:133-44; PMID:17314271; http://dx.doi.org/10.1158/1541-7786.MCR-06-0245
- Shehata M, Bièche I, Boutros R, Weidenhofer J, Fanayan S, Spalding L, Zeps N, Byth K, Bright RK, Lidereau R, et al. Nonredundant functions for tumor protein D52-like proteins support specific targeting of TPD52. Clin Cancer Res 2008; 14:5050-60; PMID:18698023; http://dx.doi.org/10.1158/1078-0432.CCR-07-4994
- Payton LA, Lewis JD, Byrne JA, Bright RK. Vaccination with metastasis-related tumor associated antigen TPD52 and CpG/ODN induces protective tumor immunity. Cancer Immunol Immunother 2008; 57:799-811; PMID:17962942; http://dx.doi.org/10.1007/s00262-007-0416-y
- Bright JD, Schultz HN, Byrne JA, Bright RK. Injection site and regulatory T cells influence durable vaccine-induced tumor immunity to an over-expressed self tumor associated antigen. Oncoimmunology 2013; 2:e25049; PMID:24073379; http://dx.doi.org/10.4161/onci.25049
- Lewis JD, Sullivan LA, Byrne JA, de Riese W, Bright RK. Memory and cellular immunity induced by a DNA vaccine encoding self antigen TPD52 administered with soluble GM-CSF. Cancer Immunol Immunother 2009; 58:1337-49; PMID:19169682; http://dx.doi.org/10.1007/s00262-009-0659-x
- Bright JD, Aldrich JF, Byrne JA, Bright RK. Vaccination with the prostate cancer overexpressed tumor self-protein TPD52 elicits protective tumor immunity and a potentially unique subset of CD8+ T cells. Austin J Clin Immunol 2014; 1:1-13
- Mirshahidi S, Kramer VG, Whitney JB, Essono S, Lee S, Dranoff G, Anderson KS, Ruprecht RM. Overlapping synthetic peptides encoding TPD52 as breast cancer vaccine in mice: prolonged survival. Vaccine 2009; 27:1825-33; PMID:19201387; http://dx.doi.org/10.1016/j.vaccine.2009.01.089
- Bright RK, Kimchi ET, Shearer MH, Kennedy RC, Pass HI. SV40 Tag-specific cytotoxic T lymphocytes generated from the peripheral blood of malignant pleural mesothelioma patients. Cancer Immunol Immunother 2002; 50:682-90; PMID:11862420; http://dx.doi.org/10.1007/s00262-001-0240-8
- Bright RK, Vocke CD, Emmert-Buck MR, Duray PH, Solomon D, Fetsch P, Rhim JS, Linehan WM, Topalian SL. Generation and genetic characterization of immortal human prostate epithelial cell lines derived from primary cancer specimens. Cancer Res 1997; 57:995-1002; PMID:9041206
- Duraiswamy J, Kaluza KM, Freeman GJ, Coukos G. Dual blockade of PD-1 and CTLA-4 combined with tumor vaccine effectively restores T-cell rejection function in tumors. Cancer Res 2013; 73:3591-603; PMID:23633484; http://dx.doi.org/10.1158/0008-5472.CAN-12-4100