Abstract
This review examines the immunity, immunopathology, and contemporary problems of vaccine development against sexually transmitted Chlamydia trachomatis. Despite improved surveillance and treatment initiatives, the incidence of C. trachomatis infection has increased dramatically over the past 30 years in both the developed and developing world. Studies in animal models have shown that protective immunity to C. trachomatis is largely mediated by Th1 T cells producing IFN-γ which is needed to prevent dissemination of infection. Similar protection appears to develop in humans but in contrast to mice, immunity in humans may take years to develop. Animal studies and evidence from human infection indicate that immunity to C. trachomatis is accompanied by significant pathology in the upper genital tract. Although no credible evidence is currently available to indicate that autoimmunity plays a role, nevertheless, this underscores the necessity to design vaccines strictly based on chlamydial-specific antigens and to avoid those displaying even minimal sequence homologies with host molecules. Current advances in C. trachomatis vaccine development as well as alternatives for designing new vaccines for this disease are discussed. A novel approach for chlamydia vaccine development, based on targeting endogenous dendritic cells, is described.
Introduction
Chlamydia trachomatis is the most common sexually transmitted bacterial infection in the world with more than 92 million new cases reported per annum and with more than two-thirds of these occurring in developing countries.Citation1,2 Pelvic inflammatory disease (PID), ectopic pregnancy, and infertility are the major adverse clinical outcomes of public health importance.Citation3,4 Chlamydia control programs aimed at reducing the incidence, prevalence and morbidity of disease have been implemented in many developed countries. These programs involve the screening and detection of infected individuals, antimicrobial treatment and partner notification. Over the past 30 y the incidence of sexually transmitted chlamydia has dramatically increased in various countries including the USA,Citation5,6 Canada,Citation7 Sweden,Citation8 Norway, and Finland.Citation9 In addition, the World Health Organization (WHO) has identified high levels of transmission in Sub Saharan Africa and South East Asia.Citation2 Whether this increase correlates with a net increase in chlamydia disease or whether it is the result of more wide-spread screening and improved diagnosis is controversial. It is clear however, that the highest rates of chlamydia world-wide occur among young adults, particularly women aged 15–24 y old from lower socio-economic backgrounds.Citation10
Sexually transmitted C. trachomatis infects the endocervical epithelia of women and the urethral epithelia in men.Citation3 Infection remains subclinical in a large proportion of infected individuals (70–90% of women; 30–50% of men) who can still transmit infection.Citation3 Among untreated women, C. trachomatis can ascend along the endometrial epithelium to the fallopian tubes where it can establish persistent infection causing pelvic inflammatory disease (PID) (). Symptoms of PID range from none to severe abdominal pain, fever, dyspareunia, prolonged menstruation, and inter-menstrual bleeding.
Figure 1. Model of C. trachomatis immunity and pathology. Following cervical infection, an early acute inflammatory response occurs, characterized by secretion of pro-inflammatory cytokines and recruitment of immune cells. Cytokine production by immune cells synergizes with ongoing immune responses that ultimately controls infection but also may cause pathology. C. trachomatis can ascend via the endometrium to the upper genital tract. As a consequence, local pro-inflammatory mediators and cytokines are produced. In an attempt to control the infection, Chlamydia-specific T cells and corresponding cytokines infiltrate the oviduct. These inflammatory responses, if persistent, may lead to fibrosis, scarring, and reproductive sequelae. Abbreviations: MMP, matrix metalloproteinases; SIgA, secretary immunoglobulin A; EBS, Chlamydia elementary bodies; MIP2, macrophage inflammatory protein-2.
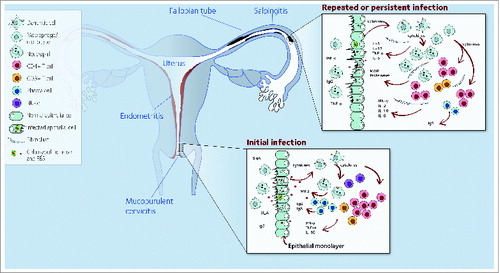
Studies in humans and animal models have identified some correlates of anti-chlamydial immunity. However, recent studies have also demonstrated that immune responses to chlamydial infection can be a significant source of pathology and disease. Vaccine development will need to ensure that immunisation does not cause exacerbation of inflammatory mechanisms which may lead to further tissue and organ damage.Citation11-15
A safe and reliable vaccine is considered to be the best approach to reduce global prevalence of C. trachomatis infection. While considerable progress has been made in recent years, an efficacious C. trachomatis vaccine remains elusive. Identification of correlates of immunity studies in both animal models and human studies, and clarification of protective immune mechanisms to infection, have impaired progress. The discovery of a number of new C. trachomatis and C. muridarum vaccine candidate antigens () are encouraging and has inspired renewed efforts to design a human vaccine. However, suitable adjuvants that help stimulate T cell-mediated immunity against intracellular bacteria are lacking. Likewise, a vaccine delivery system to induce and sustain the immune response to C. trachomatis is needed. Research in cancer immunotherapy and experimental vaccines for intracellular infections provides valuable insight for the design of a new vaccine against C. trachomatis infection. Vaccines that target endogenous immune cells, such as dendritic cells (DCs), have shown a significant enhancement of T-cell immunity in cancer.Citation16 This review examines various aspects of chlamydia immunity and immunopathology in the context of current vaccine development and explores the possibility of utilizing a DC targeting approach to design new C. trachomatis vaccines.
Table 1. C. trachomatis vaccine candidate antigens and immune responses
Immunity to C. trachomatis
The last two decades have seen significant progress in the understanding of immunity to C. trachomatis genital infection. It is now known that mice or guinea pigs can resist reinfection 30–60 d after primary infection, but these animals can be reinfected after 75 d, although the severity of disease is reduced.Citation17,18 However, the dynamics of susceptibility and host resistance to C. trachomatis infection in humans, as compared with animal models, differ substantially. Epidemiological studies, for example, show that under natural conditions T-cell immunity develops slowly perhaps reflecting the silent nature of C. trachomatis infection.Citation19
Chlamydia-Specific T cell Immunity
In both mice and guinea pigs, immunity to C. trachomatis is characterized by the development of antigen specific T-cells and antibodies. Antibodies are detectable within 10–14 d after infection. In mice, antibodies produced are long-lasting and can be detected in serum 300 d after infection.Citation18,20 The presence of chlamydia-specific antibodies, however, does not correlate with resolution of infection in humans and, in fact, seem to be more correlated with increased morbidity.Citation21,22 Nevertheless, studies in mice have shown that anti-chlamydia antibodies are more important in the control of re-infection rather than primary infection.Citation23,24 Specifically, B-cell deficient mice with no detectable antibody responses successfully resolved genital infection with C. muridarum .Citation23 However, mice depleted of both CD4+ T cells and CD8+ T cells, expressing normal anti-chlamydia antibody responses, successfully resisted reinfection.Citation24
Studies in mice consistently show that development of immunity to genital C. muridarum depends on chlamydia-specific CD4+ T cells and that production of IFN-γ by these cells is required to inhibit chlamydia growth. Gene knockout studies in mice have shown that mice lacking CD4+ T cells or lacking IFN-γ are impaired to control infection,Citation25,26 but immunity can be restored by adoptive transfer of CD4+ Th1 cells.Citation27 A more recent report showed that CD4+ T cells are necessary and sufficient for protection against chlamydial reinfection in murine genital mucosa.Citation28 CD4+ and CD8+ T cells accumulate in the upper genital tract of guinea pigsCitation29 and macaque monkeysCitation30 following C. trachomatis genital infection. Presence of both cell types correlated with resistance to reinfection. Furthermore, a study in humans showed that CD4+ and CD8+ T cells are recruited to the cervix of women with C. trachomatis infection and that these cells disappeared following antibiotic treatment.Citation31 Although these studies suggest that CD4+ and CD8+ T cells may be involved in the control of C. trachmatis infection, the importance of those cell populations have been clearly defined only for CD4+ T cells in the mouse where they are essential for protection.
T-Cell Effector Mechanisms
IFN-γ produced by T cells is critical to control chlamydia genital infection. In vitro cell culture of human cells have shown that IFN-γ activates infected cells to restrict intracellular growth of C. trachomatis by inducing the tryptophan-catabolizing enzyme, indoleamine 2,3-dioxygenase (IDO). Without tryptophan, C. trachomatis cannot survive. Thus, mutant cell lines responsive to IFN-γ but deficient in IDO activity, efficiently support C. trachomatis growth.Citation32 Likewise, infected cells cultured in medium with incremental levels of exogenous tryptophan indicated that chlamydial growth requires presence of this essential amino acid.Citation32,33 C. trachomatis strains isolated from the human urogenital tract can synthesize tryptophan if provided exogenous indol. Therefore, it is possible that indol produced by the microbiota of the female genital tract (FGT) could serve as a source for indol, thus circumventing or diminishing the protective effect of IFN-γ.Citation34,35 Additional antimicrobial mechanisms of C. trachomatis have also been documented such as the production of nitric oxide and oxygen free radicals by phagocytes, both of which inhibit the chlamydial growth following phagocytosis.Citation36 In contrast to human epithelial cells, mouse epithelial cells lack IDO, and IFN-γ mediated suppression of C. trachomatis growth is thought to be due to a family of IFN-γ inducible p47 GTPases.Citation37 Moreover, in mice, recent experimental evidence has demonstrated that chlamydia specific CD4+ T cell clones can inhibit the growth of C. muridarum in epithelial cells by degranulation-dependent mechanisms that appear to directly lyse chlamydial cells.Citation38 Thus, although IFN-γ inhibits chlamydia growth, the specific effector mechanisms differ substantially in mice compared to in humans. This has implications for vaccine development, as results from the mouse model may not be directly extrapolated to humans and a vaccine developed in mice may require further testing in non-human primates prior to human clinical trials.
Immunity and Duration of Infection
A relevant question in the natural history of C. trachomatis infection is the amount of time the infected host needs to control an untreated infection. In guinea pigs and mice, primary infection lasts a few weeks before anti-chlamydial immunity and resolution of infection develops naturally.Citation39 In humans however, the duration of infection can be much more extended. A review of human epidemiological studies on C. trachomatis infections reported that in the short-term intervals (weeks) between initial screening and follow-up, spontaneous resolution rates were between 11% and 44%.Citation40 In a long-term study of 82 women with untreated C. trachomatis cervical infection, it was demonstrated that 54% spontaneously resolved infection at one year, 82% at two years, 91% at three years, and 95% at four years.Citation19,41 Similarly, another follow-up cohort study has reported that only 45% of C. trachomatis positive women cleared infection after one-year.Citation41 From these studies, it is not known for certain if those long duration infections were unresolved infections or if they were re-infections. Nevertheless, under natural conditions, immunity to C. trachomatis infection in humans appears to take months to develop. Protracted development of immunity in humans may be due to a number of factors such as low virulence properties of the bacteria at the time of infection,Citation42 host immunosuppressive abilities of the microbe (reviewed in ref. 4), enhanced survival of chlamydia outside cells,Citation43 and ability to persist intracellularly.Citation44,45
Once infected, as many as 85–90 percent of C. trachomatis infections in men and women remain asymptomatic.Citation3,46 At least one third of asymptomatic infected women present local signs of infection.Citation3 The reason for the low virulence of C. trachomatis is not well understood. C. trachomatis contains a lipopolysaccharide (LPS) of reduced potency at triggering activation of host immunity. For example, C. trachomatis LPS is at least 100 times less potent at activating blood leukocytes than LPS from Salmonella. The reduced LPS response may explain, in part, why genital tract infection remains mostly asymptomatic. In addition, C. trachomatis downregulates MHC class I and II genes induced by IFN-γ.Citation47 Together, these observations suggest that by inducing immunosuppressive mechanisms on the host and by not inducing or stimulating sufficient immune responses, C. trachomatis has evolved effective mechanisms for survival which should be considered in the design of prophylactic vaccines.
Human Immunity
For ethical reasons, studies addressing the dynamics of anti-chlamydial immunity in humans have been difficult to develop. Once diagnosed, patients infected with C. trachomatis are required to be promptly treated. Nevertheless, evidence from epidemiological observations has shown that immunity in humans does develop slowly over time. Both the prevalence and intensity of infection among young women are significantly higher than those observed in older women, suggesting development of age acquired immunity.Citation48 Furthermore, women with reduced CD4+ T cell counts, due to human immunodeficiency virus (HIV) infection, possess an increased risk for developing C. trachomatis PID, indicating that CD4+ T cells are necessary to control infection in humans.Citation49 C. trachomatis infection rates are inversely correlated with duration of the disease in female commercial sex workers.Citation50 In this context, sex workers whose immune cells respond to C. trachomatis heat shock protein 60 by producing IFN-γ in vitro, showed reduced risk of acquiring C. trachomatis infection at follow-up.Citation51 Although studies to date are suggestive that Th1 responses correlate with protection, a recent study provides evidence that Chlamydia-specific Th2 responses may be key to controlling infection in humans.Citation52 This possibility requires further investigation.
Immunopathology to C. trachomatis
Innate immunity and pathology
Several studies in animal models and in humans have shown that the first contact of C. trachomatis with host cells during infection leads to secretion of pro-inflammatory cytokines including TNFα, IL-8, IL-1α, and GM-CSF.Citation11,53,54 These cytokines, which are required to trigger the immune response, can cause collateral tissue damage.Citation15,31, Citation53-55 For example, production of IL-1 by infected fallopian tube organ cultures have been associated with destruction of fallopian tube epithelia in vivo.Citation11 Signals derived from Toll-like receptor 2 (TLR2) have also been implicated in pathology. For example, mice without TLR2 do not develop significant pathology in the oviduct following C. muridarum infection.Citation56 Moreover, plasmid-cured C. muridarum mutants that retain the ability to infect the murine genital tract were unable to trigger TLR2-mediated signaling and did not develop oviduct pathology.Citation57 Recent observations demonstrate that mice lacking TLR2 do not develop significant pathology in the upper genital tract following infection with C. muridarum.Citation58
Most chlamydial isolates contain chlamydial plasmids. These plasmids have been found to play a significant role in chlamydial-associated pathology in primate ocular tissues.Citation59 In mice, C. muridarum organisms lacking chlamydial plasmids do not cause significant pathology in the upper genital tract.Citation60
Matrix metalloproteinases (MMPs) play an important role in cellular turnover and extracellular matrix remodelling in the female reproductive tract.Citation61 Various members of the MMPs, including MMP9, MMP13, MMP10, and others expressed by endometrial cells of mice infected with C. muridarum have been implicated in tissue damage in the mouse endometrium.Citation62 Supporting the effect of MMPs in pathogenesis is the fact that inhibition of MMPs with captopril protected mice against chronic disease by C. muridaum.Citation63 In humans, expression of MMP9 by fallopian tube cells infected with C. trachomatis was associated with epithelial tubal scarring.Citation64 Remarkably, the presence of high levels of α-defensins, antimicrobial peptides produced by activated neutrophils, were linked to endometritis in C. trachomatis infected women.Citation65 Thus, C. trachomatis infection upregulates a large number of innate inflammatory mediators within the reproductive tract, and the presence of these mediators have been associated with a poorer clinical outcome. The interactions of these mediators and how the inflammatory responses might be modified therapeutically, to limit the tissue damage, require further study.
Acquired immunity and pathology
Although the most potent host defense mechanism against C. trachomatis is based on T-cell immunity, human studies have shown correlation between anti-chlamydial T cell immunity and pathology in the FGT. For example, IFN-γ levels were significantly higher in cervical washes of women with recurrent chlamydial infection as compared with IFN-γ levels observed in women with primary infections.Citation66 These high IFN-γ levels were also associated with infertility.Citation67 Another study showed that cervical cells isolated from C. trachomatis infected women secrete IL-1, IL-6, IL-8, and IL-10 in vitro, a cytokine profile more compatible with disease exacerbation rather than immunity.Citation68 In this context, high levels of IL-10 in cervical washes of C. trachomatis infected women were linked to infertility.Citation55
Further studies in animals infected with sexually transmitted chlamydia have shown association of T cell-immunity and pathology in the upper genital tract.Citation12,13,15,69 For example, in macaques, cytotoxic CD8+ T cells and other immune cells infiltrate tubal tissue implants infected with C. trachomatis. Infiltrated cells expressed Th1 type cytokines including IFN-γ, IL-2, and IL-6 and accumulation of fibrotic tissue. Moreover, studies confirmed the presence of these CD8+ T cells in the fallopian tubes and their association with pathology in primates and guinea pigs following infection with C. trachomatis.Citation13,69
Chlamydia-specific CD4+ T cells have also been implicated in pathology. Protective CD4+ T cells were found to preferentially migrate to the upper genital tract of C.muridarun infected mice and their presence was associated with both immunityCitation70 and tubal pathology in the genital tract.Citation70,71 Interestingly, a recent study has demonstrated that IL-17, produced by Th17 cells, can promote modest immunity in mice infected with C. muridarum.Citation72 However, further studies are needed to examine the role of Th17 cells in C. trachomatis infection. Together, these observations suggest that a complex interplay of immune responses influence both immune protection and pathology in sexually transmitted C. trachomatis infections. Antigens in C. trachomatis subunit vaccines should be rigorously examined for specificity, so that potential cross-reactivity can be avoided.
Vaccine Development
Over the past 20 y, significant advances have been made in the development of a C. trachomatis vaccine. Firstly, studies in mice and epidemiological observations in humans, consistently show that cellular immunity to genital chlamydia infections depends on chlamydia-specific T cells producing IFN-γ and anti-chlamydia antibodies.Citation23,24,73 Secondly, a number of protective T cell antigens have now been identified ()Citation4, Citation74-85 (reviewed in ref. 86). Immunization of laboratory animals has shown that these antigens are recognized by chlamydia-specific T cells producing IFN-γ and partially protected animals following immunization.Citation4,75 Importantly, a study has demonstrated that the chlamydial major outer membrane protein (MOMP) antigen alone is insufficient to induce the necessary protection, however MOMP and a second antigen, outer membrane protein 2 (OMP2), can substantially increase resistance to reinfection.Citation83 This suggests that a vaccine is likely to be based on several, rather than single, protective antigens. Third, it is becoming clear that immune responses in the mucosa occur at local lymph nodes (LNs) and that effector cells migrate via blood to mucosal infection sites.Citation87,88 Finally, both locally produced IgA as well as systemic IgG are required to control infection in the genital tract,Citation89 although only IgG antibodies have been reported to have protective effects against C.muridarum in the mouse.Citation90
Vaccine Adjuvants
A major problem for a C. trachomatis vaccine is the lack of suitable non-toxic adjuvants that efficiently promote Th1 type of immunity. Immunization of mice with MOMP DNA without adjuvants,Citation91or MOMP with classical adjuvants such as Freund'sCitation81 showed moderate levels of T cell responses. However, immunization with MOMP incorporated into Vibrio cholera ghosts (rVCGs),Citation92,93 or Immune Stimulating Complexes (ISCOMS)Citation94 as adjuvanted delivery technologies, induced robust Th1 responses and protected mice against subsequent chlamydial infection.Citation4 Likewise, transcutaneous immunization of mice with MOMP incorporated in the delivery system, lipid C, a novel lipid-based matrix originally developed for oral immunization, induced partial protection of both genital and respiratory infections with C. muridarum.Citation95 Interestingly, intranasal immunization with antigen chlamydial protease-like activity factor (CPAF) and IL-12 as an adjuvant, resulted in significantly high levels of anti-IgG2a and anti-IgA antibodies in bronchoalveolar lavage and vaginal fluids. Immunized mice developed Th1 immunity against CPAF, and immunized animals significantly reduced bacterial shedding following challenge and accelerated resolution of infection as compared with non-immunized controls.Citation96 Overall, these observations showed that vaccine adjuvants are remarkably effective at triggering and sustaining Th1 immunity, leading to increased resistance to infection challenge.
The potential of TLRs-derived adjuvants
TLRs are specialized recognition receptors expressed by immune cells and most DCs. TLRs recognize pathogen-associated molecules known as PAMPs, expressed by pathogens. Binding of TLRs to their PAMP ligands activates DCs, by triggering the NF-κB signaling pathway, resulting in transcription of pro-inflammatory mediators including chemokines, adhesion molecules, matrix metalloproteases, nitric oxide synthase, several enzymes and Th1 type cytokines such as IL-12; all of which contribute to the development of Th1 immunity.Citation97 For example, experimental vaccines against Salmonella Typhimurium,Citation98 Toxoplasma gondii,Citation99 and Leishmania major Citation100 have employed CpG-containing oligodeoxynucleotide (CpG-ODN), a TLR9 agonist, as adjuvant in laboratory animals. Immunized animals develop remarkably high levels of Th1 immunity and become resistant to secondary infections. CpG-ODN has been employed in experimental vaccines against C. muridarum and C. caviae in guinea pigs and in Balb/C mice. These immunization studies showed that CpG-ODN induced potent Th1 type immunity and partial resistance against chlamydial infection.Citation82,101 CpG-ODN, and polyinosinic: polycytidylic acid (poly I:C), a TLR3 agonist, are presently undergoing human clinical trials in immunotherapies against cancer.Citation102,103 For example, phase I trials of a vaccine against ovarian tumor employing poly I:C as adjuvant, shows rapid induction of immune response based on antibodies CD8+ and CD4+ against ovarian tumor.Citation104 Another efficient TLR-mediated adjuvant is monophosphoryl lipid A, a TLR4 agonist, which is currently used in human papilloma virus (HPV) and human hepatitis B virus (HBV) vaccines in humans.Citation105 In sum, TLR-derived adjuvants are strong inducers of DCs activation and Th1 immunity and are prime adjuvant candidates for a future chlamydia vaccine.
Targeting Dendritic Cells (DCs): A Novel Approach for a C. trachomatis Vaccine
Caldwell and collaborators immunized mice with DCs pulsed with heat-killed chlamydia. Immunized mice developed a potent Th1 immunity and resisted chlamydial genital infection.Citation106 Brunham and co-workers have employed DCs pulsed with chlamydial immunopeptides in immunizations by adoptive transfer. Immunized mice developed Th1 protective immunity and partially resisted chlamydial lung and genital infections.Citation75 These data show that DCs can be effective inducers of anti-chlamydial immunity and therefore, are attractive tools to design novel T-cell vaccines, including a C. trachomatis vaccine.
DCs are professional antigen presenting cells (APCs) with a unique capacity to stimulate naïve T cells. DCs differentiate from bone-marrow CD34+ proliferating progenitors and colonize most peripheral tissues as immature non-dividing cells.Citation107 DCs have been found in mucosal tissueCitation108 and are recruited to cervical mucosa during C. trachomatis infection.Citation4,109 The diverse functions of DCs in immunity depend in part on the state of maturation. Mature DCs optimally prime and induce expansion of both CD4+ and CD8+ T cells producing IFN-γ. Citation107 Maturation of DCs occurs following detection of microbial PAMPs by TLRs on DCs.Citation107 Maturing DCs express high levels of the NF-kB family of transcription factors that induce expression of MHC class I and II antigens and co-stimulatory molecules CD80, CD86, CD40, as well as enhanced secretion of IL-12 and IFN-α;Citation110 all of which contribute to promote Th1 type of immunity.
Murine DCs Subgroups for Vaccine Targeting
Two main groups of DCs relevant to vaccine targeting have been characterized in the mouse. The first subgroup relevant to vaccine targeting expresses the inhibitory receptor, dendritic cell receptor 2 (DCR2). Antigens delivered to DCR2 DCs are processed in the MHC class II antigen pathway and presented to CD4+ T cells.Citation111 A second DCs subgroup expresses the endocytic mannose receptor DEC-205, which is a 205-kDa protein containing C-type lectin domains. Antigens phagocytized by DCs via DEC-205 are presented to both CD4+ T cells and to CD8+ T cells.Citation112 Remarkably, vaccines delivered via DEC-205 DCs have been shown to be 100–1000 times more efficient at priming peptide-specific CD4+ and CD8+ T cells than a peptide given in Complete Freund's Adjuvant (CFA).Citation16,113,114 Additionally, vaccines targeting DEC-205 dendritic cells have been tested in experimental vaccines for intracellular infections caused by Leishmania major Citation115 and HIV,Citation116 with significant high levels of both CD4+ and CD8+ T cell immunity produced.Citation115
Potential Human DCs for Vaccine Targeting
Various human DC markers have been identified. The C-type lectin domain family 9 member A (Clec9A), encodes a type II membrane protein expressed by human blood DCs. In the mouse, Clec9A DCs homologs promote CD4+ and CD8+ T cell responses.Citation117 An equivalent of the mouse DEC-205 receptor, has been identified in humans and antibodies to human DEC-205 have been developed.Citation118 In vitro, targeting of human DCs expressing DEC-205 results in induction of CD4+ and CD8+ T cells.Citation118 Similarly, the human homolog of mouse DCR2, (DCIR), has been identified on human plasmacytoid DCs (pDCs). Antigens targeted to pDCs via DCIR in vitro are efficiently presented to T cells.Citation119 The DC-specific intercellular adhesion molecule-3-grabbing non-integrin (DC-SIGN) is another marker expressed on a subset of human DCs. DC-SIGN is abundantly expressed on immature DCs and downregulated during DC maturation.Citation120,121 Recent immunization studies showed that DC-SIGN dendritic cells promote induction of antigen-specific CD4+ and CD8+ T-cell responses in DC-SIGN transgenic mice.Citation122 Together, these results show that simultaneous discovery of complementary DC groups in the mouse and in humans, can facilitate translation of immunization results from mice to humans. Furthermore, targeting DCs with C. trachomatis vaccines in the presence of safe TLR-mediated adjuvants such as Poly:IC promise to greatly enhance Th1 type of immunity and resistance to chlamydial infection.
Future Directions
A number of challenges remain before an efficacious vaccine against C. trachomatis can be formulated. The immune regulation of the FGT, which is highly influenced by sex hormones, is poorly understood. Thus, targeting T cell vaccines to the FGT without causing collateral pathology is a major concern. Related to this problem is the fact that a suitable adjuvant that vigorously promotes human T cell-mediated immunity is lacking. Experimental chlamydial vaccines have employed Freund's adjuvant, Vibrio cholera ghosts, and immuno-stimulating complexes, some of which have been tested in human clinical trials.Citation123 Current testing of TLR mediated adjuvants is encouraging. CpG oligonucleotide, poly I:C as well as monophosphoryl lipid A, are presently used in human vaccines or in clinical trials for other infections.Citation102,103,105 Hence, it is feasible that human C. trachomatis vaccine trials employing these or new adjuvants and available candidate antigens could commence in the foreseeable future.
DCs have emerged as attractive targets to develop novel chlamydia vaccines. The diverse functions of DCs in immunity and their unique ability to prime naïve T cells represent excellent opportunity to design novel chlamydia vaccines in the future. Vaccines targeting endogenous DCs with chlamydia antigens promise to induce and expand both CD4+ and CD8+ T cells producing IFN-γ, with the potential to efficiently control C. trachomatis infection.Citation124 An ideal future C. trachomatis vaccine would contain various chlamydia antigens targeted to endogenous DCs and a TLR-derived adjuvant as a source of DC maturation signal that would promote optimal DC processing and presentation of T-cell antigens. Targeting specific receptors on DCs requires recombinant antibody molecules and construction of these antibodies is not always feasible. Similarly, TLR-derived adjuvants can be toxic for host cells and chemical modifications are needed before they can be employed in humans. These challenges, which are currently being addressed in various experimental vaccines, will need to be considered when designing a chlamydia vaccine targeting DCs in humans.
Disclosure of Potential Conflicts of Interest
No potential conflicts of interest were disclosed.
References
- Belland R, Ojcius DM, Byrne GI. Chlamydia. Nat Rev Microbiol 2004; 2:530-1; PMID:15248311; http://dx.doi.org/10.1038/nrmicro931
- World Health Organization. Global Prevalence and Incidence of Selected Curable Sexually Transmitted Infections: Overview and Estimates [Internet]. Geneva: World Health Organization; 2001 [cited 2012 Aug 8]. Available from: http://www.who.int/hiv/pub/sti/who_hiv_aids_2001.02.pdf
- Peipert JF. Clinical practice. Genital chlamydial infections. N Engl J Med 2003; 349:2424-30; PMID:14681509; http://dx.doi.org/10.1056/NEJMcp030542
- Brunham RC, Rey-Ladino J. Immunology of Chlamydia infection: implications for a Chlamydia trachomatis vaccine. Nat Rev Immunol 2005; 5:149-61; PMID:15688042; http://dx.doi.org/10.1038/nri1551
- Centers for Disease Control and Prevention. Sexually transmitted disease surveillance 2009 [Internet]. Atlanta, US: Division of preventive STD prevention, Department of Health and Human Services, CDCP; 2010 [cited 2012 Aug 12]. Available from: http://www.cdc.gov/std/stats09/surv2009-Complete.pdf
- Centers for Disease Control and Prevention. Sexually transmitted disease surveillance 1993 [Internet]. Atlanta, US: Division of preventive STD prevention, Department of Health and Human Services, CDCP; 1994 [cited 2012 Sept 1]. Available from: http://wonder.cdc.gov/wonder/STD/OSTD3009.PCW.html
- Brunham RC, Pourbohloul B, Mak S, White R, Rekart ML. The unexpected impact of a Chlamydia trachomatis infection control program on susceptibility to reinfection. J Infect Dis 2005; 192:1836-44; PMID:16235186; http://dx.doi.org/10.1086/497341
- Low N. Screening programmes for chlamydial infection: when will we ever learn? BMJ 2007; 334:725-8; PMID:17413173; http://dx.doi.org/10.1136/bmj.39154.378079.BE
- Fenton KA, Lowndes CM. Recent trends in the epidemiology of sexually transmitted infections in the European Union. Sex Transm Infect 2004; 80:255-63; PMID:15295121; http://dx.doi.org/10.1136/sti.2004.009415
- Parrish DD, Kent CK. Access to care issues for African American communities: implications for STD disparities. Sex Transm Dis 2008; 35(Suppl):S19-22; PMID:18946368; http://dx.doi.org/10.1097/OLQ.0b013e31818f2ae1
- Hvid M, Baczynska A, Deleuran B, Fedder J, Knudsen HJ, Christiansen G, Birkelund S. Interleukin-1 is the initiator of Fallopian tube destruction during Chlamydia trachomatis infection. Cell Microbiol 2007; 9:2795-803; PMID:17614966; http://dx.doi.org/10.1111/j.1462-5822.2007.00996.x
- Igietseme JU, He Q, Joseph K, Eko FO, Lyn D, Ananaba G, Campbell A, Bandea C, Black CM. Role of T lymphocytes in the pathogenesis of Chlamydia disease. J Infect Dis 2009; 200:926-34; PMID:19656067; http://dx.doi.org/10.1086/605411
- Van Voorhis WC, Barrett LK, Sweeney YT, Kuo CC, Patton DL. Repeated Chlamydia trachomatis infection of Macaca nemestrina fallopian tubes produces a Th1-like cytokine response associated with fibrosis and scarring. Infect Immun 1997; 65:2175-82; PMID:9169748
- Gottlieb SL, Martin DH, Xu F, Byrne GI, Brunham RC. Summary: The natural history and immunobiology of Chlamydia trachomatis genital infection and implications for Chlamydia control. J Infect Dis 2010; 201(Suppl 2):S190-204; PMID:20524236; http://dx.doi.org/10.1086/652401
- Darville T, Hiltke TJ. Pathogenesis of genital tract disease due to Chlamydia trachomatis. J Infect Dis 2010; 201(Suppl 2):S114-25; PMID:20524234; http://dx.doi.org/10.1086/652397
- Boscardin SB, Hafalla JC, Masilamani RF, Kamphorst AO, Zebroski HA, Rai U, Morrot A, Zavala F, Steinman RM, Nussenzweig RS, et al. Antigen targeting to dendritic cells elicits long-lived T cell help for antibody responses. J Exp Med 2006; 203:599-606; PMID:16505139; http://dx.doi.org/10.1084/jem.20051639
- Barron AL, Rank RG, Moses EB. Immune response in mice infected in the genital tract with mouse pneumonitis agent (Chlamydia trachomatis biovar). Infect Immun 1984; 44:82-5; PMID:6706408
- Rank RG, Whittum-Hudson JA. Protective immunity to chlamydial genital infection: evidence from animal studies. J Infect Dis 2010; 201(Suppl 2):S168-77; PMID:20470052; http://dx.doi.org/10.1086/652399
- Molano M, Meijer CJ, Weiderpass E, Arslan A, Posso H, Franceschi S, Ronderos M, Muñoz N, van den Brule AJ. The natural course of Chlamydia trachomatis infection in asymptomatic Colombian women: a 5-year follow-up study. J Infect Dis 2005; 191:907-16; PMID:15717266; http://dx.doi.org/10.1086/428287
- Ramsey KH, Newhall WJ 5th, Rank RG. Humoral immune response to chlamydial genital infection of mice with the agent of mouse pneumonitis. Infect Immun 1989; 57:2441-6; PMID:2744854
- Punnonen R, Terho P, Nikkanen V, Meurman O. Chlamydial serology in infertile women by immunofluorescence. Fertil Steril 1979; 31:656-9; PMID:376359
- Witkin SS, Jeremias J, Neuer A, David S, Kligman I, Toth M, Willner E, Witkin K. Immune recognition of the 60kD heat shock protein: implications for subsequent fertility. Infect Dis Obstet Gynecol 1996; 4:152-8; PMID:18476087; http://dx.doi.org/10.1155/S1064744996000336
- Ramsey KH, Soderberg LS, Rank RG. Resolution of chlamydial genital infection in B-cell-deficient mice and immunity to reinfection. Infect Immun 1988; 56:1320-5; PMID:3258586
- Morrison SG, Morrison RP. Resolution of secondary Chlamydia trachomatis genital tract infection in immune mice with depletion of both CD4 +and CD8+ T cells. Infect Immun 2001; 69:2643-9; PMID:11254630; http://dx.doi.org/10.1128/IAI.69.4.2643-2649.2001
- Su H, Caldwell HD. CD4+ T cells play a significant role in adoptive immunity to Chlamydia trachomatis infection of the mouse genital tract. Infect Immun 1995; 63:3302-8; PMID:7642259
- Wang S, Fan Y, Brunham RC, Yang X. IFN-gamma knockout mice show Th2-associated delayed-type hypersensitivity and the inflammatory cells fail to localize and control chlamydial infection. Eur J Immunol 1999; 29:3782-92; PMID:10556835; http://dx.doi.org/10.1002/(SICI)1521-4141(199911)29:11≤3782::AID-IMMU3782≥3.0.CO;2-B
- Hawkins RA, Rank RG, Kelly KA. A Chlamydia trachomatis-specific Th2 clone does not provide protection against a genital infection and displays reduced trafficking to the infected genital mucosa. Infect Immun 2002; 70:5132-9; PMID:12183563; http://dx.doi.org/10.1128/IAI.70.9.5132-5139.2002
- Gondek DC, Olive AJ, Stary G, Starnbach MN. CD4+ T cells are necessary and sufficient to confer protection against Chlamydia trachomatis infection in the murine upper genital tract. J Immunol 2012; 189:2441-9; PMID:22855710; http://dx.doi.org/10.4049/jimmunol.1103032
- Rank RG, Bowlin AK, Kelly KA. Characterization of lymphocyte response in the female genital tract during ascending Chlamydial genital infection in the guinea pig model. Infect Immun 2000; 68:5293-8; PMID:10948157; http://dx.doi.org/10.1128/IAI.68.9.5293-5298.2000
- Van Voorhis WC, Barrett LK, Sweeney YT, Kuo CC, Patton DL. Analysis of lymphocyte phenotype and cytokine activity in the inflammatory infiltrates of the upper genital tract of female macaques infected with Chlamydia trachomatis. J Infect Dis 1996; 174:647-50; PMID:8769630; http://dx.doi.org/10.1093/infdis/174.3.647
- Ficarra M, Ibana JS, Poretta C, Ma L, Myers L, Taylor SN, Greene S, Smith B, Hagensee M, Martin DH, et al. A distinct cellular profile is seen in the human endocervix during Chlamydia trachomatis infection. Am J Reprod Immunol 2008; 60:415-25; PMID:18798835; http://dx.doi.org/10.1111/j.1600-0897.2008.00639.x
- Beatty WL, Belanger TA, Desai AA, Morrison RP, Byrne GI. Tryptophan depletion as a mechanism of gamma interferon-mediated chlamydial persistence. Infect Immun 1994; 62:3705-11; PMID:8063385
- Taylor MW, Feng GS. Relationship between interferon-gamma, indoleamine 2,3-dioxygenase, and tryptophan catabolism. FASEB J 1991; 5:2516-22; PMID:1907934
- Romanik M, Martirosian G, Wojciechowska-Wieja A, Cieślik K, Kaźmierczak W. [Co-occurence of indol-producing bacterial strains in the vagina of women infected with Chlamydia trachomatis]. Ginekol Pol 2007; 78:611-5; PMID:18050609
- Roshick C, Wood H, Caldwell HD, McClarty G. Comparison of gamma interferon-mediated antichlamydial defense mechanisms in human and mouse cells. Infect Immun 2006; 74:225-38; PMID:16368976; http://dx.doi.org/10.1128/IAI.74.1.225-238.2006
- Ramsey KH, Sigar IM, Rana SV, Gupta J, Holland SM, Byrne GI. Role for inducible nitric oxide synthase in protection from chronic Chlamydia trachomatis urogenital disease in mice and its regulation by oxygen free radicals. Infect Immun 2001; 69:7374-9; PMID:11705910; http://dx.doi.org/10.1128/IAI.69.12.7374-7379.2001
- Nelson DE, Virok DP, Wood H, Roshick C, Johnson RM, Whitmire WM, Crane DD, Steele-Mortimer O, Kari L, McClarty G, et al. Chlamydial IFN-gamma immune evasion is linked to host infection tropism. Proc Natl Acad Sci U S A 2005; 102:10658-63; PMID:16020528; http://dx.doi.org/10.1073/pnas.0504198102
- Jayarapu K, Kerr M, Ofner S, Johnson RM. Chlamydia-specific CD4 T cell clones control Chlamydia muridarum replication in epithelial cells by nitric oxide-dependent and -independent mechanisms. J Immunol 2010; 185:6911-20; PMID:21037093; http://dx.doi.org/10.4049/jimmunol.1002596
- Rank RG, Batteiger BE, Soderberg LS. Susceptibility to reinfection after a primary chlamydial genital infection. Infect Immun 1988; 56:2243-9; PMID:2457553
- Geisler WM. Duration of untreated, uncomplicated Chlamydia trachomatis genital infection and factors associated with chlamydia resolution: a review of human studies. J Infect Dis 2010; 201(Suppl 2):S104-13; PMID:20470048; http://dx.doi.org/10.1086/652402
- Morré SA, van den Brule AJ, Rozendaal L, Boeke AJ, Voorhorst FJ, de Blok S, Meijer CJ. The natural course of asymptomatic Chlamydia trachomatis infections: 45% clearance and no development of clinical PID after one-year follow-up. Int J STD AIDS 2002; 13(Suppl 2):12-8; PMID:12537719; http://dx.doi.org/10.1258/095646202762226092
- Ingalls RR, Rice PA, Qureshi N, Takayama K, Lin JS, Golenbock DT. The inflammatory cytokine response to Chlamydia trachomatis infection is endotoxin mediated. Infect Immun 1995; 63:3125-30; PMID:7542638
- Brunham RC, Plummer FA, Stephens RS. Bacterial antigenic variation, host immune response, and pathogen-host coevolution. Infect Immun 1993; 61:2273-6; PMID:8500868
- Beatty WL, Morrison RP, Byrne GI. Persistent chlamydiae: from cell culture to a paradigm for chlamydial pathogenesis. Microbiol Rev 1994; 58:686-99; PMID:7854252
- Belland RJ, Nelson DE, Virok D, Crane DD, Hogan D, Sturdevant D, Beatty WL, Caldwell HD. Transcriptome analysis of chlamydial growth during IFN-gamma-mediated persistence and reactivation. Proc Natl Acad Sci U S A 2003; 100:15971-6; PMID:14673075; http://dx.doi.org/10.1073/pnas.2535394100
- Beatty WL, Byrne GI, Morrison RP. Repeated and persistent infection with Chlamydia and the development of chronic inflammation and disease. Trends Microbiol 1994; 2:94-8; PMID:8156277; http://dx.doi.org/10.1016/0966-842X(94)90542-8
- Zhong G, Liu L, Fan T, Fan P, Ji H. Degradation of transcription factor RFX5 during the inhibition of both constitutive and interferon gamma-inducible major histocompatibility complex class I expression in chlamydia-infected cells. J Exp Med 2000; 191:1525-34; PMID:10790427; http://dx.doi.org/10.1084/jem.191.9.1525
- Datta SD, Sternberg M, Johnson RE, Berman S, Papp JR, McQuillan G, Weinstock H. Gonorrhea and chlamydia in the United States among persons 14 to 39 years of age, 1999 to 2002. Ann Intern Med 2007; 147:89-96; PMID:17638719; http://dx.doi.org/10.7326/0003-4819-147-2-200707170-00007
- Kimani J, Maclean IW, Bwayo JJ, MacDonald K, Oyugi J, Maitha GM, Peeling RW, Cheang M, Nagelkerke NJ, Plummer FA, et al. Risk factors for Chlamydia trachomatis pelvic inflammatory disease among sex workers in Nairobi, Kenya. J Infect Dis 1996; 173:1437-44; PMID:8648217; http://dx.doi.org/10.1093/infdis/173.6.1437
- Brunham RC, Kimani J, Bwayo J, Maitha G, Maclean I, Yang C, Shen C, Roman S, Nagelkerke NJ, Cheang M, et al. The epidemiology of Chlamydia trachomatis within a sexually transmitted diseases core group. J Infect Dis 1996; 173:950-6; PMID:8603976; http://dx.doi.org/10.1093/infdis/173.4.950
- Cohen CR, Koochesfahani KM, Meier AS, Shen C, Karunakaran K, Ondondo B, Kinyari T, Mugo NR, Nguti R, Brunham RC. Immunoepidemiologic profile of Chlamydia trachomatis infection: importance of heat-shock protein 60 and interferon- gamma. J Infect Dis 2005; 192:591-9; PMID:16028127; http://dx.doi.org/10.1086/432070
- Vicetti Miguel RD, Harvey SA, LaFramboise WA, Reighard SD, Matthews DB, Cherpes TL. Human female genital tract infection by the obligate intracellular bacterium Chlamydia trachomatis elicits robust Type 2 immunity. PLoS One 2013; 8:e58565; PMID:23555586; http://dx.doi.org/10.1371/journal.pone.0058565
- Rasmussen SJ, Eckmann L, Quayle AJ, Shen L, Zhang YX, Anderson DJ, Fierer J, Stephens RS, Kagnoff MF. Secretion of proinflammatory cytokines by epithelial cells in response to Chlamydia infection suggests a central role for epithelial cells in chlamydial pathogenesis. J Clin Invest 1997; 99:77-87; PMID:9011579; http://dx.doi.org/10.1172/JCI119136
- Ault KA, Tawfik OW, Smith-King MM, Gunter J, Terranova PF. Tumor necrosis factor-alpha response to infection with Chlamydia trachomatis in human fallopian tube organ culture. Am J Obstet Gynecol 1996; 175:1242-5; PMID:8942495; http://dx.doi.org/10.1016/S0002-9378(96)70035-2
- Agrawal T, Vats V, Salhan S, Mittal A. Determination of chlamydial load and immune parameters in asymptomatic, symptomatic and infertile women. FEMS Immunol Med Microbiol 2009; 55:250-7; PMID:19159426; http://dx.doi.org/10.1111/j.1574-695X.2008.00530.x
- Darville T, O’Neill JM, Andrews CW Jr., Nagarajan UM, Stahl L, Ojcius DM. Toll-like receptor-2, but not Toll-like receptor-4, is essential for development of oviduct pathology in chlamydial genital tract infection. J Immunol 2003; 171:6187-97; PMID:14634135; http://dx.doi.org/10.4049/jimmunol.171.11.6187
- O’Connell CM, Ingalls RR, Andrews CW Jr., Scurlock AM, Darville T. Plasmid-deficient Chlamydia muridarum fail to induce immune pathology and protect against oviduct disease. J Immunol 2007; 179:4027-34; PMID:17785841; http://dx.doi.org/10.4049/jimmunol.179.6.4027
- Dong X, Liu Y, Chang X, et al. Signaling via TNFR1 but not TLR2 contributes significantly to hydrosalpinx development following Chlamydia muridarum infection. Infect Immun 2014; 82:1833-9; PMID:24549331; http://dx.doi.org/10.1128/IAI.01668-13
- Kari L, Whitmire WM, Olivares-Zavaleta N, Goheen MM, Taylor LD, Carlson JH, Sturdevant GL, Lu C, Bakios LE, Randall LB, et al. A live-attenuated chlamydial vaccine protects against trachoma in nonhuman primates. J Exp Med 2011; 208:2217-23; PMID:21987657; http://dx.doi.org/10.1084/jem.20111266
- Lei L, Chen J, Hou S, Ding Y, Yang Z, Zeng H, Baseman J, Zhong G. Reduced live organism recovery and lack of hydrosalpinx in mice infected with plasmid-free Chlamydia muridarum. Infect Immun 2014; 82:983-92; PMID:24343644; http://dx.doi.org/10.1128/IAI.01543-13
- Fata JE, Ho AT, Leco KJ, Moorehead RA, Khokha R. Cellular turnover and extracellular matrix remodeling in female reproductive tissues: functions of metalloproteinases and their inhibitors. Cell Mol Life Sci 2000; 57:77-95; PMID:10949582; http://dx.doi.org/10.1007/s000180050500
- Ramsey KH, Sigar IM, Schripsema JH, Shaba N, Cohoon KP. Expression of matrix metalloproteinases subsequent to urogenital Chlamydia muridarum infection of mice. Infect Immun 2005; 73:6962-73; PMID:16177376; http://dx.doi.org/10.1128/IAI.73.10.6962-6973.2005
- Imtiaz MT, Schripsema JH, Sigar IM, Kasimos JN, Ramsey KH. Inhibition of matrix metalloproteinases protects mice from ascending infection and chronic disease manifestations resulting from urogenital Chlamydia muridarum infection. Infect Immun 2006; 74:5513-21; PMID:16988226; http://dx.doi.org/10.1128/IAI.00730-06
- Ault KA, Kelly KA, Ruther PE, Izzo AA, Izzo LS, Sigar IM, Ramsey KH. Chlamydia trachomatis enhances the expression of matrix metalloproteinases in an in vitro model of the human fallopian tube infection. Am J Obstet Gynecol 2002; 187:1377-83; PMID:12439534; http://dx.doi.org/10.1067/mob.2002.126850
- Wiesenfeld HC, Heine RP, Krohn MA, Hillier SL, Amortegui AA, Nicolazzo M, Sweet RL. Association between elevated neutrophil defensin levels and endometritis. J Infect Dis 2002; 186:792-7; PMID:12198613; http://dx.doi.org/10.1086/342417
- Agrawal T, Vats V, Salhan S, Mittal A. Mucosal and peripheral immune responses to chlamydial heat shock proteins in women infected with Chlamydia trachomatis. Clin Exp Immunol 2007; 148:461-8; PMID:17493018; http://dx.doi.org/10.1111/j.1365-2249.2007.03357.x
- Agrawal T, Vats V, Salhan S, Mittal A. Local markers for prediction of women at higher risk of developing sequelae to Chlamydia trachomatis infection. Am J Reprod Immunol 2007; 57:153-9; PMID:17217370; http://dx.doi.org/10.1111/j.1600-0897.2006.00459.x
- Agrawal T, Gupta R, Dutta R, Srivastava P, Bhengraj AR, Salhan S, Mittal A. Protective or pathogenic immune response to genital chlamydial infection in women–a possible role of cytokine secretion profile of cervical mucosal cells. Clin Immunol 2009; 130:347-54; PMID:19019735; http://dx.doi.org/10.1016/j.clim.2008.10.004
- Rank RG, Sanders MM, Patton DL. Increased incidence of oviduct pathology in the guinea pig after repeat vaginal inoculation with the chlamydial agent of guinea pig inclusion conjunctivitis. Sex Transm Dis 1995; 22:48-54; PMID:7709325; http://dx.doi.org/10.1097/00007435-199501000-00008
- Kelly KA, Walker JC, Jameel SH, Gray HL, Rank RG. Differential regulation of CD4 lymphocyte recruitment between the upper and lower regions of the genital tract during Chlamydia trachomatis infection. Infect Immun 2000; 68:1519-28; PMID:10678969; http://dx.doi.org/10.1128/IAI.68.3.1519-1528.2000
- Maxion HK, Kelly KA. Chemokine expression patterns differ within anatomically distinct regions of the genital tract during Chlamydia trachomatis infection. Infect Immun 2002; 70:1538-46; PMID:11854242; http://dx.doi.org/10.1128/IAI.70.3.1538-1546.2002
- Scurlock AM, Frazer LC, Andrews CW Jr., O’Connell CM, Foote IP, Bailey SL, Chandra-Kuntal K, Kolls JK, Darville T. Interleukin-17 contributes to generation of Th1 immunity and neutrophil recruitment during Chlamydia muridarum genital tract infection but is not required for macrophage influx or normal resolution of infection. Infect Immun 2011; 79:1349-62; PMID:21149587; http://dx.doi.org/10.1128/IAI.00984-10
- Farris CM, Morrison RP. Vaccination against Chlamydia genital infection utilizing the murine C. muridarum model. Infect Immun 2011; 79:986-96; PMID:21078844; http://dx.doi.org/10.1128/IAI.00881-10
- Barker CJ, Beagley KW, Hafner LM, Timms P. In silico identification and in vivo analysis of a novel T-cell antigen from Chlamydia, NrdB. Vaccine 2008; 26:1285-96; PMID:18261829; http://dx.doi.org/10.1016/j.vaccine.2007.12.048
- Karunakaran KP, Rey-Ladino J, Stoynov N, Berg K, Shen C, Jiang X, Gabel BR, Yu H, Foster LJ, Brunham RC. Immunoproteomic discovery of novel T cell antigens from the obligate intracellular pathogen Chlamydia. J Immunol 2008; 180:2459-65; PMID:18250455; http://dx.doi.org/10.4049/jimmu-nol.180.4.2459
- Meoni E, Faenzi E, Frigimelica E, Zedda L, Skibinski D, Giovinazzi S, Bonci A, Petracca R, Bartolini E, Galli G, et al. CT043, a protective antigen that induces a CD4+ Th1 response during Chlamydia trachomatis infection in mice and humans. Infect Immun 2009; 77:4168-76; PMID:19596772; http://dx.doi.org/10.1128/IAI.00344-09
- Soriani M, Petit P, Grifantini R, Petracca R, Gancitano G, Frigimelica E, Nardelli F, Garcia C, Spinelli S, Scarabelli G, et al. Exploiting antigenic diversity for vaccine design: the chlamydia ArtJ paradigm. J Biol Chem 2010; 285:30126-38; PMID:20592031; http://dx.doi.org/10.1074/jbc.M110.118513
- Chaganty BK, Murthy AK, Evani SJ, Li W, Guentzel MN, Chambers JP, Zhong G, Arulanandam BP. Heat denatured enzymatically inactive recombinant chlamydial protease-like activity factor induces robust protective immunity against genital chlamydial challenge. Vaccine 2010; 28:2323-9; PMID:20056182; http://dx.doi.org/10.1016/j.vaccine.2009.12.064
- Wang J, Chen L, Chen F, Zhang X, Zhang Y, Baseman J, Perdue S, Yeh IT, Shain R, Holland M, et al. A chlamydial type III-secreted effector protein (Tarp) is predominantly recognized by antibodies from humans infected with Chlamydia trachomatis and induces protective immunity against upper genital tract pathologies in mice. Vaccine 2009; 27:2967-80; PMID:19428908; http://dx.doi.org/10.1016/j.vaccine.2009.02.095
- Coler RN, Bhatia A, Maisonneuve JF, Probst P, Barth B, Ovendale P, Fang H, Alderson M, Lobet Y, Cohen J, et al. Identification and characterization of novel recombinant vaccine antigens for immunization against genital Chlamydia trachomatis. FEMS Immunol Med Microbiol 2009; 55:258-70; PMID:19281568; http://dx.doi.org/10.1111/j.1574-695X.2008.00527.x
- Pal S, Theodor I, Peterson EM, de la Maza LM. Immunization with the Chlamydia trachomatis mouse pneumonitis major outer membrane protein can elicit a protective immune response against a genital challenge. Infect Immun 2001; 69:6240-7; PMID:11553566; http://dx.doi.org/10.1128/IAI.69.10.6240-6247.2001
- Berry LJ, Hickey DK, Skelding KA, Bao S, Rendina AM, Hansbro PM, Gockel CM, Beagley KW. Transcutaneous immunization with combined cholera toxin and CpG adjuvant protects against Chlamydia muridarum genital tract infection. Infect Immun 2004; 72:1019-28; PMID:14742549; http://dx.doi.org/10.1128/IAI.72.2.1019-1028.2004
- Eko FO, He Q, Brown T, McMillan L, Ifere GO, Ananaba GA, Lyn D, Lubitz W, Kellar KL, Black CM, et al. A novel recombinant multisubunit vaccine against Chlamydia. J Immunol 2004; 173:3375-82; PMID:15322201; http://dx.doi.org/10.4049/jimm-unol.173.5.3375
- Goodall JC, Yeo G, Huang M, Raggiaschi R, Gaston JS. Identification of Chlamydia trachomatis antigens recognized by human CD4+ T lymphocytes by screening an expression library. Eur J Immunol 2001; 31:1513-22; PMID:11465108; http://dx.doi.org/10.1002/1521-4141(200105)31:5≤1513::AID-IMMU1513≥3.0.CO;2-U
- Starnbach MN, Loomis WP, Ovendale P, Regan D, Hess B, Alderson MR, Fling SP. An inclusion membrane protein from Chlamydia trachomatis enters the MHC class I pathway and stimulates a CD8+ T cell response. J Immunol 2003; 171:4742-9; PMID:14568950; http://dx.doi.org/10.4049/jimmunol.171.9.4742
- Hafner LM, Wilson DP, Timms P. Development status and future prospects for a vaccine against Chlamydia trachomatis infection. Vaccine 2014; 32:1563-71; PMID:23973245
- Yeaman GR, Guyre PM, Fanger MW, Collins JE, White HD, Rathbun W, Orndorff KA, Gonzalez J, Stern JE, Wira CR. Unique CD8+ T cell-rich lymphoid aggregates in human uterine endometrium. J Leukoc Biol 1997; 61:427-35; PMID:9103229
- Mitchell EA, Bergmeier LA, Doyle C, Brookes R, Hussain LA, Wang Y, Lehner T. Homing of mononuclear cells from iliac lymph nodes to the genital and rectal mucosa in non-human primates. Eur J Immunol 1998; 28:3066-74; PMID:9808175; http://dx.doi.org/10.1002/(SICI)1521-4141(199810)28:10≤3066::AID-IMMU3066≥3.0.CO;2-2
- Johansson M, Lycke NY. Immunology of the human genital tract. Curr Opin Infect Dis 2003; 16:43-9; PMID:12821829; http://dx.doi.org/10.1097/00001432-200302000-00008
- Morrison SG, Morrison RP. The protective effect of antibody in immunity to murine chlamydial genital tract reinfection is independent of immunoglobulin A. Infect Immun 2005; 73:6183-6; PMID:16113345; http://dx.doi.org/10.1128/IAI.73.9.6183-6186.2005
- Pal S, Barnhart KM, Wei Q, Abai AM, Peterson EM, de la Maza LM. Vaccination of mice with DNA plasmids coding for the Chlamydia trachomatis major outer membrane protein elicits an immune response but fails to protect against a genital challenge. Vaccine 1999; 17:459-65; PMID:10073724; http://dx.doi.org/10.1016/S0264-410X(98)00219-9
- Eko FO, Lubitz W, McMillan L, Ramey K, Moore TT, Ananaba GA, Lyn D, Black CM, Igietseme JU. Recombinant Vibrio cholerae ghosts as a delivery vehicle for vaccinating against Chlamydia trachomatis. Vaccine 2003; 21:1694-703; PMID:12639492; http://dx.doi.org/10.1016/S0264-410X(02)00677-1
- Eko FO, Okenu DN, Singh UP, He Q, Black C, Igietseme JU. Evaluation of a broadly protective Chlamydia-cholera combination vaccine candidate. Vaccine 2011; 29:3802-10; PMID:21421002; http://dx.doi.org/10.1016/j.vaccine.2011.03.027
- Dong-Ji Z, Yang X, Shen C, Lu H, Murdin A, Brunham RC. Priming with Chlamydia trachomatis major outer membrane protein (MOMP) DNA followed by MOMP ISCOM boosting enhances protection and is associated with increased immunoglobulin A and Th1 cellular immune responses. Infect Immun 2000; 68:3074-8; PMID:10816446; http://dx.doi.org/10.1128/IAI.68.6.3074-3078.2000
- Hickey DK, Aldwell FE, Beagley KW. Transcutaneous immunization with a novel lipid-based adjuvant protects against Chlamydia genital and respiratory infections. Vaccine 2009; 27:6217-25; PMID:19698810; http://dx.doi.org/10.1016/j.vaccine.2009.08.001
- Murthy AK, Chambers JP, Meier PA, Zhong G, Arulanandam BP. Intranasal vaccination with a secreted chlamydial protein enhances resolution of genital Chlamydia muridarum infection, protects against oviduct pathology, and is highly dependent upon endogenous gamma interferon production. Infect Immun 2007; 75:666-76; PMID:17118987; http://dx.doi.org/10.1128/IAI.01280-06
- Medzhitov R, Preston-Hurlburt P, Janeway CA Jr. A human homologue of the Drosophila Toll protein signals activation of adaptive immunity. Nature 1997; 388:394-7; PMID:9237759; http://dx.doi.org/10.1038/41131
- Berchtold C, Panthel K, Jellbauer S, Köhn B, Roider E, Partilla M, Heesemann J, Endres S, Bourquin C, Rüssmann H. Superior protective immunity against murine listeriosis by combined vaccination with CpG DNA and recombinant Salmonella enterica serovar typhimurium. Infect Immun 2009; 77:5501-8; PMID:19797070; http://dx.doi.org/10.1128/IAI.00700-09
- El-Malky M, Shaohong L, Kumagai T, Yabu Y, Noureldin MS, Saudy N, Maruyama H, Ohta N. Protective effect of vaccination with Toxoplasma lysate antigen and CpG as an adjuvant against Toxoplasma gondii in susceptible C57BL/6 mice. Microbiol Immunol 2005; 49:639-46; PMID:16034207; http://dx.doi.org/10.1111/j.1348-0421.2005.tb03656.x
- Rhee EG, Mendez S, Shah JA, Wu CY, Kirman JR, Turon TN, Davey DF, Davis H, Klinman DM, Coler RN, et al. Vaccination with heat-killed leishmania antigen or recombinant leishmanial protein and CpG oligodeoxynucleotides induces long-term memory CD4+ and CD8+ T cell responses and protection against leishmania major infection. J Exp Med 2002; 195:1565-73; PMID:12070284; http://dx.doi.org/10.1084/jem.20020147
- Andrew DW, Hafner LM, Beagley KW, Timms P. Partial protection against chlamydial reproductive tract infection by a recombinant major outer membrane protein/CpG/cholera toxin intranasal vaccine in the guinea pig Chlamydia caviae model. J Reprod Immunol 2011; 91:9-16; PMID:21856018; http://dx.doi.org/10.1016/j.jri.2011.06.100
- Butowski N, Lamborn KR, Lee BL, Prados MD, Cloughesy T, DeAngelis LM, Abrey L, Fink K, Lieberman F, Mehta M, et al. A North American brain tumor consortium phase II study of poly-ICLC for adult patients with recurrent anaplastic gliomas. J Neurooncol 2009; 91:183-9; PMID:18850068; http://dx.doi.org/10.1007/s11060-008-9705-3
- Kim YH, Girardi M, Duvic M, Kuzel T, Link BK, Pinter-Brown L, Rook AH. Phase I trial of a Toll-like receptor 9 agonist, PF-3512676 (CPG 7909), in patients with treatment-refractory, cutaneous T-cell lymphoma. J Am Acad Dermatol 2010; 63:975-83; PMID:20888065; http://dx.doi.org/10.1016/j.jaad.2009.12.052
- Sabbatini P, Tsuji T, Ferran L, Ritter E, Sedrak C, Tuballes K, Jungbluth AA, Ritter G, Aghajanian C, Bell-McGuinn K, et al. Phase I trial of overlapping long peptides from a tumor self-antigen and poly-ICLC shows rapid induction of integrated immune response in ovarian cancer patients. Clin Cancer Res 2012; 18:6497-508; PMID:23032745; http://dx.doi.org/10.1158/1078-0432.CCR-12-2189
- Garçon N, Chomez P, Van Mechelen M. GlaxoSmithKline Adjuvant Systems in vaccines: concepts, achievements and perspectives. Expert Rev Vaccines 2007; 6:723-39; PMID:17931153; http://dx.doi.org/10.1586/14760584.6.5.723
- Su H, Messer R, Whitmire W, Fischer E, Portis JC, Caldwell HD. Vaccination against chlamydial genital tract infection after immunization with dendritic cells pulsed ex vivo with nonviable Chlamydiae. J Exp Med 1998; 188:809-18; PMID:9730883; http://dx.doi.org/10.1084/jem.188.5.809
- Steinman RM, Pope M. Exploiting dendritic cells to improve vaccine efficacy. J Clin Invest 2002; 109:1519-26; PMID:12070296; http://dx.doi.org/10.1172/JCI0215962
- Parr MB, Kepple L, Parr EL. Langerhans cells phagocytose vaginal epithelial cells undergoing apoptosis during the murine estrous cycle. Biol Reprod 1991; 45:252-60; PMID:1786290; http://dx.doi.org/10.1095/biolreprod45.2.252
- Agrawal T, Vats V, Wallace PK, Singh A, Salhan S, Mittal A. Recruitment of myeloid and plasmacytoid dendritic cells in cervical mucosa during Chlamydia trachomatis infection. Clin Microbiol Infect 2009; 15:50-9; PMID:19046168; http://dx.doi.org/10.1111/j.1469-0691.2008.02113.x
- Medzhitov R. Toll-like receptors and innate immunity. Nat Rev Immunol 2001; 1:135-45; PMID:11905821; http://dx.doi.org/10.1038/35100529
- Dudziak D, Kamphorst AO, Heidkamp GF, Buchholz VR, Trumpfheller C, Yamazaki S, Cheong C, Liu K, Lee HW, Park CG, et al. Differential antigen processing by dendritic cell subsets in vivo. Science 2007; 315:107-11; PMID:17204652; http://dx.doi.org/10.1126/science.1136080
- Bozzacco L, Trumpfheller C, Huang Y, Longhi MP, Shimeliovich I, Schauer JD, Park CG, Steinman RM. HIV gag protein is efficiently cross-presented when targeted with an antibody towards the DEC-205 receptor in Flt3 ligand-mobilized murine DC. Eur J Immunol 2010; 40:36-46; PMID:19830741; http://dx.doi.org/10.1002/eji.200939748
- Mahnke K, Qian Y, Fondel S, Brueck J, Becker C, Enk AH. Targeting of antigens to activated dendritic cells in vivo cures metastatic melanoma in mice. Cancer Res 2005; 65:7007-12; PMID:16061687; http://dx.doi.org/10.1158/0008-5472.CAN-05-0938
- Trumpfheller C, Finke JS, López CB, Moran TM, Moltedo B, Soares H, Huang Y, Schlesinger SJ, Park CG, Nussenzweig MC, et al. Intensified and protective CD4+ T cell immunity in mice with anti-dendritic cell HIV gag fusion antibody vaccine. J Exp Med 2006; 203:607-17; PMID:16505141; http://dx.doi.org/10.1084/jem.20052005
- Matos I, Mizenina O, Lubkin A, Steinman RM, Idoyaga J. Targeting Leishmania major Antigens to Dendritic Cells In Vivo Induces Protective Immunity. PLoS One 2013; 8:e67453; PMID:23840706; http://dx.doi.org/10.1371/journal.pone.0067453
- Idoyaga J, Lubkin A, Fiorese C, Lahoud MH, Caminschi I, Huang Y, Rodriguez A, Clausen BE, Park CG, Trumpfheller C, et al. Comparable T helper 1 (Th1) and CD8 T-cell immunity by targeting HIV gag p24 to CD8 dendritic cells within antibodies to Langerin, DEC205, and Clec9A. Proc Natl Acad Sci U S A 2011; 108:2384-9; PMID:21262813; http://dx.doi.org/10.1073/pnas.1019547108
- Caminschi I, Proietto AI, Ahmet F, Kitsoulis S, Shin Teh J, Lo JC, Rizzitelli A, Wu L, Vremec D, van Dommelen SL, et al. The dendritic cell subtype-restricted C-type lectin Clec9A is a target for vaccine enhancement. Blood 2008; 112:3264-73; PMID:18669894; http://dx.doi.org/10.1182/blood-2008-05-155176
- Birkholz K, Schwenkert M, Kellner C, Gross S, Fey G, Schuler-Thurner B, Schuler G, Schaft N, Dörrie J. Targeting of DEC-205 on human dendritic cells results in efficient MHC class II-restricted antigen presentation. Blood 2010; 116:2277-85; PMID:20566893; http://dx.doi.org/10.1182/blood-2010-02-268425
- Meyer-Wentrup F, Benitez-Ribas D, Tacken PJ, Punt CJ, Figdor CG, de Vries IJ, Adema GJ. Targeting DCIR on human plasmacytoid dendritic cells results in antigen presentation and inhibits IFN-alpha production. Blood 2008; 111:4245-53; PMID:18258799; http://dx.doi.org/10.1182/blood-2007-03-081398
- Svajger U, Anderluh M, Jeras M, Obermajer N. C-type lectin DC-SIGN: an adhesion, signalling and antigen-uptake molecule that guides dendritic cells in immunity. Cell Signal 2010; 22:1397-405; PMID:20363321; http://dx.doi.org/10.1016/j.cellsig.2010.03.018
- Pereira CF, Torensma R, Hebeda K, Kretz-Rommel A, Faas SJ, Figdor CG, Adema GJ. In vivo targeting of DC-SIGN-positive antigen-presenting cells in a nonhuman primate model. J Immunother 2007; 30:705-14; PMID:17893563; http://dx.doi.org/10.1097/CJI.0b013e31812e6256
- Hesse C, Ginter W, Förg T, Mayer CT, Baru AM, Arnold-Schrauf C, Unger WW, Kalay H, van Kooyk Y, Berod L, et al. In vivo targeting of human DC-SIGN drastically enhances CD8+ T-cell-mediated protective immunity. Eur J Immunol 2013; 43:2543-53; PMID:23784881; http://dx.doi.org/10.1002/eji.201343429
- Sun HX, Xie Y, Ye YP. ISCOMs and ISCOMATRIX. Vaccine 2009; 27:4388-401; PMID:19450632; http://dx.doi.org/10.1016/j.vaccine.2009.05.032
- Steinman RM. Dendritic cells in vivo: a key target for a new vaccine science. Immunity 2008; 29:319-24; PMID:18799140; http://dx.doi.org/10.1016/j.immuni.2008.08.001