Abstract
Intanza® 9 μg (Sanofi Pasteur), a trivalent split-virion vaccine administered by intradermal (ID) injection, was approved in Europe in 2009 for the prevention of seasonal influenza in adults 18 to 59 years. Here, we examined the immune responses induced in adults by the ID 9 μg vaccine and the standard trivalent intramuscular (IM) vaccine (Vaxigrip® 15 μg, Sanofi Pasteur). This trial was a randomized, controlled, single-center, open-label study in healthy adults 18 to 40 years of age during the 2007/8 influenza season. Subjects received a single vaccination with the ID 9 μg (n = 38) or IM 15 μg (n = 42) vaccine. Serum, saliva, and peripheral blood mononuclear cells were collected up to 180 days post-vaccination.
Geometric mean hemagglutination inhibition titers, seroprotection rates, seroconversion rates, and pre-vaccination-to-post-vaccination ratios of geometric mean hemagglutination inhibition titers did not differ between the two vaccines. Compared with pre-vaccination, the vaccines induced similar increases in vaccine-specific circulating B cells at day 7 but did not induce significant increases in vaccine-specific memory B cells at day 180. Cell-mediated immunity to all three vaccine strains, measured in peripheral blood mononuclear cells, was high at baseline and not increased by either vaccine. Neither vaccine induced a mucosal immune response. These results show that the humoral and cellular immune responses to the ID 9 μg vaccine are similar to those to the standard IM 15 μg vaccine.
Abbreviations:
- BSA, bovine serum albumin
- CHMP, Committee for Medicinal Products for Human Use
- ELISA, enzyme-linked immunosorbent assay
- ELISPOT, enzyme-linked immunospot
- HI, hemagglutination inhibition
- ID, intradermal
- Ig, immunoglobulin
- IM, intramuscular
- PBMC, peripheral blood mononuclear cells
- PBS, phosphate-buffered saline
Introduction
Intramuscular (IM) vaccination with trivalent influenza vaccines induces significant protective antibody responses and remains the cornerstone in preventing seasonal influenza.Citation1 However, due to a variety of reasons, such as not feeling at risk of contracting influenza and fear of needles, seasonal influenza vaccination remains underused, especially in adults.Citation1,2 Intradermal (ID) injection is an alternative route for delivering seasonal influenza vaccines that also induces a strong protective immune response.Citation3 ID immunization takes advantage of the high concentration of resident dendritic cells in the skin as well as the extensive vascular and lymphatic network in the dermis, which facilitates recruitment of blood-derived dendritic cells and migration of antigen-presenting cells to the lymph nodes.Citation3-5
Intanza® (Sanofi Pasteur) was the first approved ID vaccine against seasonal influenza. Intanza is a trivalent split-virion influenza vaccine administered using the Soluvia™ microinjection system (Becton Dickinson), which facilitates ID injection.Citation6 The vaccine was approved in 2009 by the European Medicines Agency for the prevention of influenza in adults (≥18 y; 9 μg hemagglutinin per strain) and the elderly (≥60 y; 15 μg hemagglutinin per strain).Citation7 When administered to adults, it induces a similar protective response, as measured by hemagglutination inhibition (HI) titers, as the standard IM trivalent split-virion vaccine (Vaxigrip® 15 μg, Sanofi Pasteur).Citation8,9
Both humoral immunity and cell-mediated immunity contribute to protection against influenza. Serum HI and serum single radial hemolysis (SRH) titers are used as correlates of protection by influenza vaccines.Citation10,11 However, circulating antibodies are thought to be most protective against the homologous infecting strain.Citation12 Cell-mediated immunity, in contrast, has the potential to act against both heterologous and homologous strains. Circulating mucosal antibodies, especially immunoglobulin (Ig) A, may also help protect against influenza infection or aid in viral clearance, although their role in humans remains unclear.Citation13 The aim of the current study was to characterize and compare humoral and cellular responses in adult subjects following vaccination with the ID split-virion influenza vaccine, Intanza 9 μg, and the IM influenza vaccine standard, Vaxigrip 15 μg.
Results
Subjects
Of the 80 subjects included in the study, 38 received the ID 9 μg influenza vaccine and 42 received the IM 15 μg vaccine. One subject each in the ID 9 μg and IM 15 μg vaccine groups voluntarily discontinued before the end of the study, one subject in the ID 9 μg vaccine group was lost to follow up, and one subject in the ID 9 μg vaccine group did not comply with the study visit schedule. Thus, 35 in the ID 9 μg group and 41 in the IM 15 μg group completed the study. Ages (mean ± standard deviation = 26.6 ± 5.7 in the ID 9 μg and 25.7 ± 4.8 in the IM 15 μg group) and sex distributions (68.4% female in the ID 9 μg and 71.4% female in the IM 15 μg group) were similar.
Humoral immune response
Serum HI antibody concentrations
Geometric mean titers (GMTs) were similar for the ID 9 μg and IM 15 μg groups before vaccination, increased to similar levels 14 d after vaccination, and did not further increase between day 14 and day 21 (A). At both post-vaccination time points (days 14 and 21), GMTs were similar between the ID 9 μg and IM 15 μg groups for the two A strains (A/H1N1 and A/H3N2). GMTs for the B strain, however, tended to be lower in the ID 9 μg group than in the IM 15 μg group (91.2 [95% CI, 64.6–129] vs. 181 [95% CI, 129–255] at day 14 and 78.5 [95% CI, 54.5–113] vs. 156 [95% CI, 114–214] at day 21).
Figure 1. Humoral immune response in subjects receiving the ID and IM influenza vaccines (A) Geometric mean titers (GMTs) at days 0, 14, 21, and 180. (B) Seroprotection rates pre-vaccination (day 0) and at post-vaccination days 14, 21, and 180. Seroprotection was defined as a HI titer ≥40. (C) Seroconversion rates at post-vaccination days 14, 21, and 180. Seroconversion was defined as a post-vaccination HI titer ≥40 in subjects with a pre-vaccination titer <10 or a ≥4-fold increase compared with pre-vaccination titer in subjects with a pre-vaccination titer ≥10. (D) Geometric mean titer ratios (GMTRs). The GMTR was the ratio of post-vaccination HI titer vs. the pre-vaccination titer (day 0). Bars indicate means and error bars indicate 95% CI.
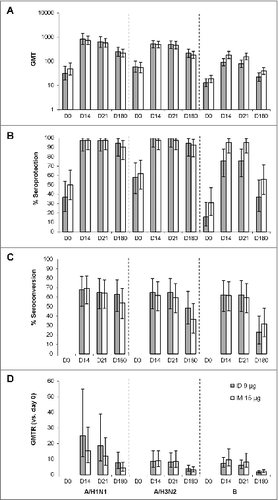
Seroprotection rates were nearly 100% for A/H1N1 and A/H3N2 at days 14 and 21 and >90% at day 180 for both the ID 9 μg and IM 15 μg groups (B). Against the B/Malaysia strain, the seroprotection rate was >70% at day 14 and 21 and >35% at day 180 for both the ID 9 μg and IM 15 μg groups. Seroconversion rates were ≥60% for all three vaccine strains of virus up to day 21 and between 20% and 60% at day 180 (C). Seroprotection rates and seroconversion rates were similar between the ID 9 μg and IM 15 μg groups both before and after vaccination.
The geometric mean of the ratio of the titer at day 14, 21, and 180 vs. the titer at day 0 (GMTR) was similar between the ID 9 μg and IM 15 μg vaccine groups for all three vaccine strains. For all three strains, the mean GMTRs were highest at day 14 to 21 and decreased by approximately half at day 180 (D).
Both vaccines met or exceeded the European Union Committee for Medicinal Products for Human Use (CHMP) criteria for annual influenza vaccines in adults.Citation14
Salivary IgA concentrations
Salivary IgA was used as a surrogate measure of the mucosal immune response.Citation15 According to the 95% CIs, post-vaccination total or virus-specific IgA concentrations (Fig. S1) and the ratio of virus-specific to total IgA concentration () did not change following vaccination.
B cell response
Compared with pre-vaccination (day 0), ID 9 μg and IM 15 μg vaccination increased the number of circulating influenza virus-specific IgG-secreting cells at day 7 to similar extents as measured by enzyme-linked immunospot (ELISPOT) assay (A). However, neither vaccine induced significant increases in the median percentage of memory influenza virus-specific antibody-secreting cells (day 180 vs. day 0) (B).
Figure 3. B cell responses to the ID and IM influenza vaccines (A) Number of circulating influenza virus-specific IgG-secreting cells pre-vaccination (day 0) and 7 d after vaccination. (B) Percent influenza virus-specific IgG-secreting memory B cells. PBMC were treated for 5 d as previously describedCitation37 to generate antibody-secreting B cells. Bars indicate geometric means and error bars indicate interquartile ranges.
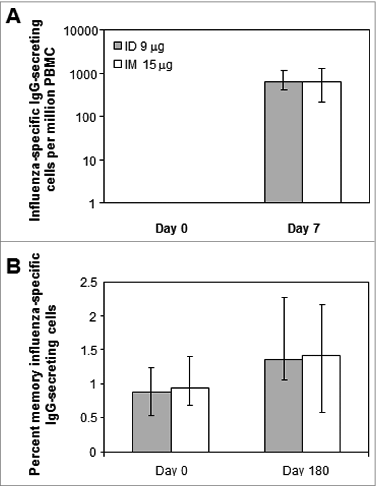
T cell response
Th1 and Th2 cytokine response
HA-stimulated cytokine secretion was greatest for IFN-γ, followed by tumor necrosis factor (TNF)-α and interleukin (IL)-5, whereas little IL-10 was released (). For both the ID 9 μg and IM 15 μg vaccine groups, the median concentrations of all four cytokines increased slightly after vaccination. At day 21, median concentrations of IFN-γ and TNF-α were slightly lower and IL-5 and IL-10 slightly higher for the ID 9 μg than for the IM 15 μg vaccine group. Overall, however, concentrations of each cytokine were within similar ranges for all conditions.
Table 1. Cytokine release by PBMC in response to recombinant A/H1N1 Solomon Island hemagglutinin
CD8− and CD8+ T cell responses
For both CD8− and CD8+ T cells, stimulation in vitro by all three types of virus was greatest for IFN-γ+ cells, followed by TNF-α+ and IL-2+ cells (). However, for both CD8− and CD8+ cells, frequencies and cell numbers were similar between the ID 9 μg and IM 15 μg vaccine groups both before and after vaccination. Frequencies of cytokine double-positive (IFN-γ+IL2+, IFN-γ+TNF-α+, and IL2+TNF-α+) and triple-positive (IFN-γ+IL2+TNF-α+) CD8− and CD8+ T cells were not significantly different between the ID 9 μg and IM 15 μg vaccine groups (data not shown).
Figure 4. Activated CD8+ T cells (CD3+CD8+CD69+) and T helper cells (CD3+CD8−CD69+) in response to the ID and IM influenza vaccines Intracellular cytokine staining for CD3, CD8, CD69, IFN-γ, TNF-α, and IL-2 was performed in PBMC stimulated in vitro for 20–24 h with the indicated strains of influenza virus. Shown are the fractions of IFN-γ+, TNF-α+, and IL-2+ T-helper lymphocytes (CD3+CD8−CD69+; left panel) and CD8+ T cells (CD3+CD8+CD69+; right panel). Bars indicate medians and error bars indicate interquartile ranges.
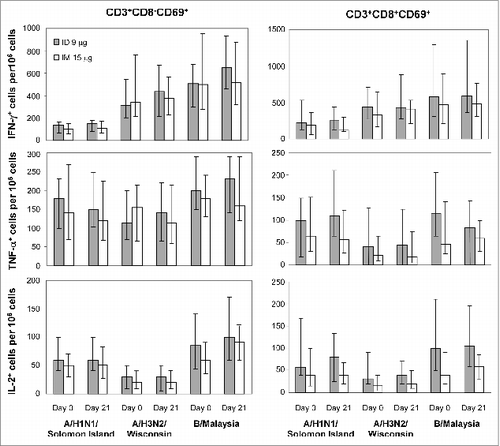
Discussion
This study compared the humoral, cellular, and mucosal immune responses to the 2007/8 Northern hemisphere formulation of the ID trivalent split-virion influenza vaccine, Intanza 9 μg, and the equivalent IM vaccine, Vaxigrip 15 μg. As measured by seroprotection rates, seroconversion rates, and GMTRs, the ID vaccine produced a similar humoral immune response as the IM vaccine for all three vaccine strains of influenza virus (A/H1N1, A/H3N2, and B). Both vaccines exceeded CHMP criteria for annual influenza vaccines in adults.Citation14 Importantly, for all three viral strains and for both vaccines, humoral immunity persisted for at least 180 d. Previous randomized studies have shown similar results, specifically, that, in adults, trivalent split-virion vaccine administered 9 μg ID induces a comparable immune response as 15 μg IM and that immunity persists for at least 12 mo.Citation8,9,16 Therefore, although the ID vaccine is approved at a lower dosage (9 μg) for adults, it provides comparable humoral immunity as the IM vaccine (15 μg).
The current study also showed that the two influenza vaccines equally increased the number of circulating virus-specific IgG-secreting effector B cells. However, the specific memory B cell and Th1/Th2 cytokine responses were not significantly enhanced by either vaccine. We also found that the CD8− (including CD4+) and CD8+ T-cell responses to both the ID and IM vaccines were weak and did not differ before or after vaccination. Admittedly, assays of T cell-mediated immune response have not been standardized, although T cells may help protect against influenza,Citation12 no specific values have been correlated with protection from influenza or influenza-like illness. To help address the concerns about standardization, we took a variety of recommended steps to reduce inter- and intra-assay variability.Citation17
The mucosal immune system is thought to be important in recovery from influenza and in protection against influenza in the respiratory tract.Citation13 Measles vaccine elicits antigen-specific IgA in saliva when administered transcutaneously but not subcutaneously.Citation18 Therefore, although specific IgA titer values have not been correlated with protection from influenza or influenza-like illness, we suspected that influenza vaccination may induce salivary IgA and that there might be differences in IgA responses between the ID and IM vaccines. However, this does not appear to be the case because we did not detect a difference between baseline and post-vaccination normalized salivary IgA levels for the ID 9 μg or the IM 15 μg vaccine, and salivary IgA levels were very low in both cases.
These cellular and humoral results agree with previous studies on the immune responses to the IM vaccine in adults and children.Citation19,20 He et al. also showed that although a live attenuated vaccine (Flumist®, MedImmune) can enhance CD4+ and CD8+ T-cell responses, a trivalent inactivated influenza vaccine (Fluzone®, Sanofi Pasteur) does not.Citation20 The inability of inactivated vaccines to enhance CD4+ T-cell immunity appears to be due to a plateau in the response to influenza antigens before adulthood following multiple exposures to the virus.Citation21 CD8+ cytotoxic T-cell immunity was not enhanced, probably because such a response requires live virus.Citation22 The lack of an increased memory B cell response following vaccination suggests that the subjects had been previously exposed to viral strains with B/T-cell epitopes also conserved in the 2007/8 vaccine strains. Finally, the absence of a mucosal IgA response agrees with previous studies showing that IM influenza vaccines do not increase mucosal levels of soluble IgA,Citation13,15 although they may prime a mucosal immune response to live virus.Citation23
The lack of differences between immune responses to ID and IM vaccination could have been due, in part, to high inter-subject variability combined with relatively small group sizes. Moreover, most of the subjects in this study most likely had been previously exposed to influenza antigens sharing B/T cell epitopes common with the 2007/8 vaccine strains. Several reports have shown that previous influenza vaccination or virus exposure is associated with higher post-vaccination HI titers in childrenCitation24 and young and elderly adults.Citation25,26 In other reports, preexisting HI antibodies have been linked to reduced humoral and effector B cell responses after vaccination.Citation27,28 The relationship between prior immune status and antibody response to influenza may depend, in part, on the viral type or subtype and, overall, on the complex history of priming for each individual. Relatively little is known about the impact of pre-immunity to influenza on vaccine-specific T cells. Boosting of influenza-specific CD4+ T cell responses after influenza vaccination may be affected by prior influenza immune status, although this may depend on the antigenic specificity of the T cells.Citation28,29 We suspect that previous exposure to influenza antigens reduced the ability to detect immunological differences between the ID and IM vaccines in this study, but it should have allowed us to detect large differences between the two vaccines. Detecting differences in immune profiles between the ID and IM influenza vaccines might have been easier in naïve individuals (young children), but the ID device is not appropriate for this age group. Finally, using T cell epitopes or peptides that detect de novo responses, as opposed to recalled responses, might have allowed us to distinguish ID and IM vaccines, but identifying and selecting such epitopes may be difficult.
Conclusions about humoral responses were based on the HI assay, which is considered the reference standard for the evaluation of influenza vaccines.Citation14 Both HI and SRH are recognized by the European Medicines Agency for the assessment of influenza vaccines and provide correlates of protection.Citation14,30 The HI assay is well known to have similar sensitivity for A strains but relatively low sensitivity for antibodies to influenza B strain in human sera,Citation11,31 which was confirmed when the ID vaccine was assessed using both techniques in the elderly.Citation11 Sensitive and specific seroneutralization (SN) tests have been developed and are increasingly used to evaluate influenza vaccines, but they are not yet recognized by the European Medicine Agency for the assessment of influenza vaccines. Although SN assays have been reported to be more sensitive than the HI assay, in particular for heterologous strains, they have not been standardized and tend to be laborious and time-consuming.Citation32 The ID vaccine has been examined using a SN assay, and as with SRH, the overall results have corroborated those from the HI assay.Citation33-35 We do not expect SN or SRH would have yielded information beyond what the HI assay provided, especially in the context of pre-immunity to influenza.
In conclusion, this study showed that, in healthy adults, the 2007/8 formulation of the ID trivalent split-virion vaccine, Intanza 9 μg, and the IM formulation, Vaxigrip 15 μg, protect against influenza by activating equivalent cellular and humoral immune responses. Humoral, cellular, and mucosal responses did not appear different, and both vaccines met or exceeded CHMP criteria for annual influenza vaccines in adults. In both cases, the vaccine's effects appeared to be primarily mediated by induction of a humoral response. Finally, like the IM vaccine, Intanza 9 μg induced a humoral response that persisted for at least 180 d.
Patients and Methods
This trial was a randomized, controlled, single-center, open-label study (EUDRACT no. 2007–002104–18) conducted at the Unité de Recherche Clinique en Immunologie – Lyon Sud (Lyon, France) between September 17, 2007 and May 5, 2008.
Ethics
The trial was conducted in accordance with the Edinburgh revision of the Declaration of Helsinki, Good Clinical Practice guidelines, and European Directive 2001/20/EC and was approved by the local ethics committee. All subjects provided written informed consent before being included in the trial.
Study subjects
Healthy adults 18 to 40 y old were recruited to receive a single injection of trivalent split-virion vaccine by ID (Intanza 9 μg) or IM (Vaxigrip 15 μg) injection. Subjects were excluded if they were participating in another clinical trial in the 4 wk preceding the trial vaccination or during the study period; if they were known or suspected to have an immunodeficiency or be receiving immunosuppressant drugs or treatments; had systemic hypersensitivity to any of the vaccine components or history of a life-threatening reactions to the trial vaccine or to a vaccine components; received blood or blood-derived products in the past 3 mo that might interfere with the assessment of immune response; received any vaccine in the 4 wk preceding the trial vaccination or planned receipt of any vaccine in the 4 wk following the trial vaccination; were seropositive for human immunodeficiency virus, hepatitis B or hepatitis C; had been vaccinated against influenza in the previous 6 mo; had treatments or diseases in the 3 wk preceding the trial considered contraindications for IM vaccination; or had febrile illness or moderate or severe acute illness or infection on the day of vaccination. Women could not be pregnant and had to be using birth control or to not be of child-bearing potential.
Vaccinations
Subjects were randomized (1:1) to receive a single injection of the 2007/8 northern hemisphere formulation of the ID trivalent split-virion influenza vaccine (Intanza 9 μg, Sanofi Pasteur) or the IM trivalent split-virion influenza vaccine (Vaxigrip 15 μg, Sanofi Pasteur), which contain influenza virus strains A/Solomon Islands/3/2006 (H1N1), A/Wisconsin/67/2005 (H3N2), and B/Malaysia/2506/2004. All injections were administered into the deltoid area. Subjects were assigned to the appropriate study group using a scratchable randomization list.
Sample collection
For isolation of PBMC, blood samples were drawn before vaccination (day 0) and on days 7 (±1), 10 (±1), 14 (±1), 21 (±3), and 180 (±7) after vaccination into BD Vacutainer heparin tubes (Becton Dickinson) and PBMC were isolated by centrifugation over a Ficoll gradient, washed, aliquoted, and stored in 10% DMSO under liquid nitrogen. For serum collection, blood samples were also collected before vaccination (day 0) and on days 14 (±1), 21 (±3), and 180 (±7) after vaccination into BD Vacutainer tubes (Becton Dickinson) and serum was collected by centrifugation, aliquoted, and stored at −20°C. Saliva samples were collected before vaccination and on days 7 (±1), 14 (±1), 21 (±3), and 180 (±7) by chewing on a roll-shaped saliva collector (Salivette; Sarstedt, Orsay, France). Saliva samples were centrifuged, aliquoted, and stored at −80°C.
HI assay
HI assays were performed according to the World Health Organization protocol.Citation36 The serum titer was defined as the highest reciprocal dilution producing complete inhibition of hemagglutination. The lower limit of detection was a reciprocal dilution of 10, and the upper limit of detection was a reciprocal dilution of 10,240.
Measurement of IgA concentrations in saliva
Microtiter plates were coated overnight with 1 μg/ml polyclonal goat anti-human IgA antibody (Southern Biotech) in phosphate-buffered saline (PBS) containing 5% bovine serum albumin (BSA). Plates were washed with PBS + 5% BSA and then incubated for 2 h at room temperature with serial dilutions of saliva samples or human IgA (Dade-Behring) in PBS + 5% BSA. Next, plates were incubated for 2 h with 0.5 μg/ml horseradish peroxidase-conjugated polyclonal goat anti-human antibody (Southern Biotech), followed by enzyme-linked immunosorbent assay (ELISA) substrate for colorimetric detection (R&D Systems). Total IgA levels (μg/ml) in samples were determined by comparison with IgA standards. Optical densities were measured using an ELISA plate reader.
Influenza-specific IgA titers (arbitrary units/ml) were measured as described above except that the plates were coated with 1 mg/ml Vaxigrip and IgA was detected with 0.5 μg/ml peroxidase-coupled polyclonal goat anti-human antibody (Southern Biotech) and ELISA Substrate (R&D Systems). The influenza-specific IgA titer from each sample was normalized by the total IgA content to limit variability between samples.
B-cell ELISPOT assay
B-cell ELISPOT assays were conducted using PBMC from 15 randomly selected subjects that had accepted that their samples be used for future testing and who had the highest numbers of PBMC. For these experiments, PBMC were collected pre-vaccination (day 0) and 7 d after vaccination. Before use in ELISPOT assays, PBMC (5 × 105 cells/well) were grown for 5 d in 24-well plates in complemented RPMI (RPMI 1640 + 10% fetal calf serum supplemented with penicillin, L-glutamine, and 50 mM β-mercaptoethanol) supplemented with 1:100 000 pokeweed mitogen (La Jolla Institute) and 1:10 000 fixed Staphylococcus aureus Cowan (Sigma). Nitrocellulose-bottomed 96-well plates were coated with Vaxigrip (1.5 μg/well) or 10 μg/ml anti-human IgG F(ab’)2 (Becton Dickinson) and incubated overnight at 4°C. After washing and blocking with PBS, PBMC (4 × 105, 2 × 105, 1 × 105, or 5 × 104 cells/well) in complemented RPMI were added. After 5 h at 37°C, plates were washed with PBS followed by PBS + 0.05% Tween-20 and then incubated overnight at 20°C for 2 h with 1 μg/ml biotinylated mouse anti-human pan-IgG Fc antibody (Becton Dickinson). Plates were washed with PBS + 0.05% Tween-20 and incubated with 1:1000 peroxidase-conjugated streptavidin (Southern Biotechnology). Plates were washed again with PBS and PBST and then developed with 3-amino-9-ethylcarbazole (Sigma). Spots were counted with an automated spot reader (Microvision). Influenza-specific B cell memory responses were also examined by ELISPOT assays using PBMC collected pre-vaccination (day 0) and 180 d after vaccination that were treated for 5 d to generate antibody-secreting cells.Citation37
Cytometric bead array assay
Cryopreserved PBMC isolated from each subject pre-vaccination (day 0) and 21 d after vaccination (0.2 ml of 106 cells/ml) were thawed and resuspended in AIM V medium (Invitrogen Life Technologies). PBMC were then added to 96-well plates and incubated for 4 d at 37°C with or without 3 μg/ml recombinant H1N1/Solomon Island hemagglutinin protein (Protein Science). The concentrations of IFN-γ, TNF-α, IL-5, and IL-10 were measured using a Th1/Th2 Cytometric Bead Array Kit (BD Biosciences) and a BD FACS Array Bioanalyzer System (BD Biosciences). The lower limits of detection were as follows: IFN-γ, 7.1 pg/ml; TNF-α, 2.8 pg/ml; IL-5, 2.4 pg/ml; IL-10, 2.8 pg/ml.
Intracellular cytokine staining
Cryopreserved PBMC isolated from each subject pre-vaccination (day 0) and 21 d after vaccination were thawed and resuspended in AIM V medium (Invitrogen Life Technologies). PMBC were then added to 96-well microtiter plates (1 × 106 per well) along with 0.5 μg/ml live influenza virus (A/H3N2 Wisconsin/67/2005, A/H1N1 Solomon Islands/3/2006, or B/Malaysia/2506/2004) at 37°C. All samples, except for those collected on day 180, were tested in the same experiment. Lyophilized PBMCs from an influenza-positive donor (Becton Dickinson) were included as a quality control. PBMC from each donor were stimulated in vitro with a positive antigen (staphylococcal enterotoxin B) to confirm that cells responded correctly and consistently throughout the study. After 7 h, 10 μg/ml brefeldin A (Sigma) was added, and after an additional 13 to 17 h (20–24 h total), cells were removed from the wells using 20 mM EDTA and then washed twice with PBS +1% BSA. The cells were fixed and permeabilized with 1X BD Cytofix buffer (BD Biosciences) for 15 min at 4°C. Next, the cells were washed once with BD Perm/Wash buffer (BD Biosciences) and incubated for 30 min at 4°C in the dark with a mixture of APC-H7-conjugated anti-CD3, PerCPCy5.5-conjugated anti-CD8, phycoerythrin-conjugated anti-CD69, allophycocyanin-conjugated anti-IL-2, fluorescein isothiocyanate-conjugated anti- IFN-γ, and phycoerythrin-Cy7-conjugated anti-TNF-α (BD Biosciences). The cells were washed twice with PBS + 1% BSA. To reduce inter-assay variability, a single pre-aliquoted mixture of lyophilized cytokine-specific antibody reagents was used for the entire study. Fluorescence was analyzed using a FACSCanto flow cytometer and BD DIVA software (BD Biosciences). Gating for cell analysis was modified as little as possible between donors. The percentage of IFN-γ-positive, tumor necrosis factor-α (TNF-α)-positive, and interleukin (IL)-2-positive cells was determined in the CD3+CD8+ and CD3+CD8− cell populations. The lower limit of quantification was 0.01% cytokine-producing cells.
Determination of study size
A target size of 40 subjects per group was chosen. Based on a standard deviation of 0.3 for the frequency of CD8− T cells expressing IFN-γ as determined by intracellular staining (our unpublished observations), the power to detect a significant difference between the two groups was 73%.
Statistical analysis
Descriptive statistics were generated using SAS version 9.1 (SAS Institute, Cary, NC). Analyses were performed in all subjects vaccinated. For all assays, values below the lower limit of detection were replaced by one half the lower limit of detection. For HI assays, values above the upper limit of detection were replaced by the upper limit of detection (10,240). Missing values were not replaced. For quantitative endpoints, 95% confidence intervals (CIs) were calculated using a normal approximation after logarithmic transformation. For qualitative endpoints, the 95% CIs were calculated using the Clopper-Pearson exact binomial method.Citation38
Disclosure of Potential Conflicts of Interest
N.N., H.B., and F.B. are employees of Sanofi Pasteur; P.L. was an employee of BD Medical Pharmaceutical Systems during realization of this study; A.R., C.G., B.V., K.R., A.H., and J.-F.N. are/were employees of one or several of the following public institutions in Lyon: INSERM U1111, IFR128, Claude Bernard University, and Hospices Civils.
Supplemental Materials
Supplemental data for this article can be accessed on the publisher's website.
970921_Supplemental_Material.zip
Download Zip (45 KB)Acknowledgments
The authors are grateful to Marie-Jose Quentin-Millet (Sanofi Pasteur) for supporting the present study and Dominique Kaiserlian (INSERM) for helpful advice. The authors also thank the patients and clinical staff at the Centre Hospitalier Lyon-Sud. The authors would also like to thank Francoise Weber (Sanofi Pasteur) for her work as clinical team leader of this clinical trial, Audrey Mamessier, Muriel Thenadey, and Emilie Proust (Sanofi Pasteur) for technical assistance, the staff from the Global Clinical Immunology (Sanofi Pasteur) for assisting with the HI assay, the Unite de Recherche Clinique en Immunologie for patient recruitment, Martine Denis (Sanofi Pasteur) for expert criticism and revision of the manuscript, and Frederick R. Vogel (Sanofi Pasteur) for coordinating the preparation of this manuscript. Medical writing was provided by Dr. Phillip Leventhal (4Clinics, Paris, France) and supported by Sanofi Pasteur.
Funding
The study was part of the Lyon BioPole MICROVAX research program and was funded by the French Ministry of Industry, Grenoble City Metro, Grand Lyon, and Rhône-Alpes region, as well as by Sanofi Pasteur. As the sponsor, Sanofi Pasteur was involved in all stages of the study conduct and analysis. Sanofi Pasteur also took in charge all costs associated with the development and the publishing of the present manuscript. The corresponding author had final responsibility to submit for publication.
References
- Scuffham PA, West PA. Economic evaluation of strategies for the control and management of influenza in Europe. Vaccine 2002; 20:2562-78; PMID:12057614; http://dx.doi.org/10.1016/S0264-410X(02)00154-8
- Blank PR, Schwenkglenks M, Szucs TD. Vaccination coverage rates in eleven European countries during two consecutive influenza seasons. J Infect 2009; 58:446-58; PMID:19446340; http://dx.doi.org/10.1016/j.jinf.2009.04.001
- Nicolas JF, Guy B. Intradermal, epidermal and transcutaneous vaccination: from immunology to clinical practice. Expert Rev Vaccines 2008; 7:1201-14; PMID:18844594; http://dx.doi.org/10.1586/14760584.7.8.1201
- Steinman RM, Pope M. Exploiting dendritic cells to improve vaccine efficacy. J Clin Invest 2002; 109:1519-26; PMID:12070296; http://dx.doi.org/10.1172/JCI0215962
- Le Borgne M, Dubois B, Kaiserlian D. Dendritic cells of mucosa and skin: “recruited for vaccination”. Med Sci (Paris) 2007; 23:819-25; PMID:17937889; http://dx.doi.org/10.1051/medsci/20072310819
- Laurent PE, Bonnet S, Alchas P, Regolini P, Mikszta JA, Pettis R, Harvey NG. Evaluation of the clinical performance of a new intradermal vaccine administration technique and associated delivery system. Vaccine 2007; 25:8833-42; PMID:18023942; http://dx.doi.org/10.1016/j.vaccine.2007.10.020
- European Medicines Agency. Assessment report for Intanza®. London: European Medicines Agency, 2009.
- Beran J, Ambrozaitis A, Laiskonis A, Mickuviene N, Bacart P, Calozet Y, Demanet E, Heijmans S, Van Belle P, Weber F, et al. Intradermal influenza vaccination of healthy adults using a new microinjection system: a 3-year randomised controlled safety and immunogenicity trial. BMC Med 2009; 7:13; PMID:19341446; http://dx.doi.org/10.1186/1741-7015-7-13
- Leroux-Roels I, Vets E, Freese R, Seiberling M, Weber F, Salamand C, Leroux-Roels G. Seasonal influenza vaccine delivered by intradermal microinjection: A randomised controlled safety and immunogenicity trial in adults. Vaccine 2008; 26:6614-9; PMID:18930093; http://dx.doi.org/10.1016/j.vaccine.2008.09.078
- Duggan ST, Plosker GL. Intanza 15 microg intradermal seasonal influenza vaccine: in older adults (aged >or=60 years). Drugs Aging 2010; 27:597-605; PMID:20583853; http://dx.doi.org/10.2165/11203880-000000000-00000
- Van Damme P, Arnou R, Kafeja F, Fiquet A, Richard P, Thomas S, Meghlaoui G, Samson SI, Ledesma E. Evaluation of non-inferiority of intradermal versus adjuvanted seasonal influenza vaccine using two serological techniques: a randomised comparative study. BMC Infect Dis 2010; 10:134; PMID:20504306; http://dx.doi.org/10.1186/1471-2334-10-134
- Thomas PG, Keating R, Hulse-Post DJ, Doherty PC. Cell-mediated protection in influenza infection. Emerg Infect Dis 2006; 12:48-54; PMID:16494717; http://dx.doi.org/10.3201/eid1201.051237
- Brandtzaeg P. Role of mucosal immunity in influenza. Dev Biol (Basel) 2003; 115:39-48; PMID:15088774
- Committee for Proprietary Medicinal Products. Note for guidance on harmonization of requirements for influenza vaccines (CPMP/BWP/214/96). London: European Medicines Agency, 1997.
- Weinberg A, Song LY, Walker R, Allende M, Fenton T, Patterson-Bartlett J, Nachman S, Kemble G, Yi TT, Defechereux P, et al.; IMPAACT P1057 Team. Anti-influenza serum and mucosal antibody responses after administration of live attenuated or inactivated influenza vaccines to HIV-infected children. J Acquir Immune Defic Syndr 2010; 55:189-96; PMID:20581690; http://dx.doi.org/10.1097/QAI.0b013e3181e46308
- Belshe RB, Newman FK, Wilkins K, Graham IL, Babusis E, Ewell M, Frey SE. Comparative immunogenicity of trivalent influenza vaccine administered by intradermal or intramuscular route in healthy adults. Vaccine 2007; 25:6755-63; PMID:17692438; http://dx.doi.org/10.1016/j.vaccine.2007.06.066
- Maecker HT, Rinfret A, D’Souza P, Darden J, Roig E, Landry C, Hayes P, Birungi J, Anzala O, Garcia M, et al. Standardization of cytokine flow cytometry assays. BMC Immunol 2005; 6:13; PMID:15978127; http://dx.doi.org/10.1186/1471-2172-6-13
- Etchart N, Hennino A, Friede M, Dahel K, Dupouy M, Goujon-Henry C, Nicolas JF, Kaiserlian D. Safety and efficacy of transcutaneous vaccination using a patch with the live-attenuated measles vaccine in humans. Vaccine 2007; 25:6891-9; PMID:17764789; http://dx.doi.org/10.1016/j.vaccine.2007.07.014
- Co MD, Orphin L, Cruz J, Pazoles P, Rothman AL, Ennis FA, Terajima M. Discordance between antibody and T cell responses in recipients of trivalent inactivated influenza vaccine. Vaccine 2008; 26:1990-8; PMID:18339461; http://dx.doi.org/10.1016/j.vaccine.2008.02.024
- He XS, Holmes TH, Zhang C, Mahmood K, Kemble GW, Lewis DB, Dekker CL, Greenberg HB, Arvin AM. Cellular immune responses in children and adults receiving inactivated or live attenuated influenza vaccines. J Virol 2006; 80:11756-66; PMID:16971435; http://dx.doi.org/10.1128/JVI.01460-06
- Zeman AM, Holmes TH, Stamatis S, Tu W, He XS, Bouvier N, Kemble G, Greenberg HB, Lewis DB, Arvin AM, et al. Humoral and cellular immune responses in children given annual immunization with trivalent inactivated influenza vaccine. Pediatr Infect Dis J 2007; 26:107-15; PMID:17259871; http://dx.doi.org/10.1097/01.inf.0000253251.03785.9b
- Tamura S, Kurata T. Defense mechanisms against influenza virus infection in the respiratory tract mucosa. Jpn J Infect Dis 2004; 57:236-47; PMID:15623947
- Johnson PR, Feldman S, Thompson JM, Mahoney JD, Wright PF. Immunity to influenza A virus infection in young children: a comparison of natural infection, live cold-adapted vaccine, and inactivated vaccine. J Infect Dis 1986; 154:121-7; PMID:3711685; http://dx.doi.org/10.1093/infdis/154.1.121
- el-Madhun AS, Cox RJ, Søreide A, Olofsson J, Haaheim LR. Systemic and mucosal immune responses in young children and adults after parenteral influenza vaccination. J Infect Dis 1998; 178:933-9; PMID:9806018; http://dx.doi.org/10.1086/515656
- Beyer WE, Palache AM, Sprenger MJ, Hendriksen E, Tukker JJ, Darioli R, van der Water GL, Masurel N, Osterhaus AD. Effects of repeated annual influenza vaccination on vaccine sero-response in young and elderly adults. Vaccine 1996; 14:1331-9; PMID:9004442; http://dx.doi.org/10.1016/S0264-410X(96)00058-8
- Ross TM, Lin CJ, Nowalk MP, Huang HH, Spencer SM, Shay DK, Sambhara S, Sundaram ME, Friedrich T, Sauereisen S, et al. Influence of pre-existing hemagglutination inhibition titers against historical influenza strains on antibody response to inactivated trivalent influenza vaccine in adults 50-80 years of age. Hum Vaccin Immunother 2014; 10:10; PMID:24614078; http://dx.doi.org/10.4161/hv.28313
- Sasaki S, He XS, Holmes TH, Dekker CL, Kemble GW, Arvin AM, Greenberg HB. Influence of prior influenza vaccination on antibody and B-cell responses. PLoS One 2008; 3:e2975; PMID:18714352; http://dx.doi.org/10.1371/journal.pone.0002975
- He XS, Holmes TH, Sasaki S, Jaimes MC, Kemble GW, Dekker CL, Arvin AM, Greenberg HB. Baseline levels of influenza-specific CD4 memory T-cells affect T-cell responses to influenza vaccines. PLoS One 2008; 3:e2574; PMID:18596908; http://dx.doi.org/10.1371/journal.pone.0002574
- Dolfi DV, Mansfield KD, Kurupati RK, Kannan S, Doyle SA, Ertl HC, Schmader KE, Wherry EJ. Vaccine-induced boosting of influenza virus-specific CD4 T cells in younger and aged humans. PLoS One 2013; 8:e77164; PMID:24155927; http://dx.doi.org/10.1371/journal.pone.0077164
- Wood JM, Newman RW, Ploss K. The use of correlates of immunity in European Union licensing of influenza vaccines. Dev Biol (Basel) 2003; 115:9-16; PMID:15088770
- Wood JM, Gaines-Das RE, Taylor J, Chakraverty P. Comparison of influenza serological techniques by international collaborative study. Vaccine 1994; 12:167-74; PMID:8147099; http://dx.doi.org/10.1016/0264-410X(94)90056-6
- Cheng LW, Huang SW, Huang LM, Chang LY, Shao PL, Kiang D, Wang JR. Comparison of neutralizing and hemagglutination-inhibiting antibody responses for evaluating the seasonal influenza vaccine. J Virol Methods 2012; 182:43-9; PMID:22433514; http://dx.doi.org/10.1016/j.jviromet.2012.03.004
- Ansaldi F, Canepa P, Ceravolo A, Valle L, de Florentiis D, Oomen R, Vogel FR, Denis M, Samson SI, Icardi G. Intanza(®) 15 mcg intradermal influenza vaccine elicits cross-reactive antibody responses against heterologous A(H3N2) influenza viruses. Vaccine 2012; 30:2908-13; PMID:22342501; http://dx.doi.org/10.1016/j.vaccine.2012.02.003
- Ansaldi F, Orsi A, de Florentiis D, Parodi V, Rappazzo E, Coppelli M, Durando P, Icardi G. Head-to-head comparison of an intradermal and a virosome influenza vaccine in patients over the age of 60: evaluation of immunogenicity, cross-protection, safety and tolerability. Hum Vaccin Immunother 2013; 9:591-8; PMID:23295262; http://dx.doi.org/10.4161/hv.23240
- Hung IF, Levin Y, To KK, Chan KH, Zhang AJ, Li P, Li C, Xu T, Wong TY, Yuen KY. Dose sparing intradermal trivalent influenza (2010/2011) vaccination overcomes reduced immunogenicity of the 2009 H1N1 strain. Vaccine 2012; 30:6427-35; PMID:22910287; http://dx.doi.org/10.1016/j.vaccine.2012.08.014
- World Health Organization. WHO Manual on Animal Influenza Diagnosis and Surveillance. Geneva: World Health Organization, 2002.
- Crotty S, Aubert RD, Glidewell J, Ahmed R. Tracking human antigen-specific memory B cells: a sensitive and generalized ELISPOT system. J Immunol Methods 2004; 286:111-22; PMID:15087226; http://dx.doi.org/10.1016/j.jim.2003.12.015
- Newcombe RG. Two-sided confidence intervals for the single proportion: comparison of seven methods. Stat Med 1998; 17:857-72; PMID:9595616; http://dx.doi.org/10.1002/(SICI)1097-0258(19980430)17:8<857::AID-SIM777>3.0.CO;2-E