Abstract
Colorectal cancer (CRC) results from the accumulation of both genetic and epigenetic alterations of the genome. However, also the formation of an inflammatory milieu plays a pivotal role in tumor development and progression. Dendritic cells (DCs) play a relevant role in tumor by exerting differential pro-tumorigenic and anti-tumorigenic functions, depending on the local milieu. Quantitative and functional impairments of DCs have been widely observed in several types of cancer, including CRC, representing a tumor-escape mechanism employed by cancer cells to elude host immunosurveillance.
Understanding the interactions between DCs and tumors is important for comprehending the mechanisms of tumor immune surveillance and escape, and provides novel approaches to therapy of cancer. This review summarizes updated information on the role of the DCs in colon cancer development and/or progression.
Abbreviations
CRC | = | Colorectal cancer |
GM-CSF | = | granulocyte macrophage colony stimulating factor |
IL | = | interleukin |
IFN | = | interferon |
TNF | = | tumor necrosis factor |
TGF | = | transforming growth factor |
VEGF | = | vascular endothelial growth factor |
DCs | = | dendritic cells |
mDCs | = | myeloid dendritic cells |
pDCs | = | plasmacytoid dendritic cells |
DCregs | = | regulatory DCs |
TIDCs | = | tumor-infiltrating DCs |
NK | = | natural killer |
MHC | = | major histocompatibility complex |
PAMP | = | pathogen-associated molecular pattern |
PRRs | = | pattern recognition receptors |
TLR | = | toll-like receptor |
Th | = | T helper |
HNSCC | = | head and neck squamous cell carcinoma |
APC | = | antigen presenting cells |
TDLNs | = | draining lymph nodes |
MDSCs | = | myeloid-derived suppressor cells |
HMGB | = | high mobility group box |
CTLA-4 | = | anticytotoxic T-lymphocyte antigen 4 |
PD-1 | = | programmed death 1 |
Introduction
Colorectal cancer (CRC) is one of the most common malignancies encountered in the population living a Western life style with over one million new cases diagnosed worldwide annually.Citation1-3 Rates of this cancer increase with industrialization and urbanization. CRC has been much more common in high income countries but it is now increasing in middle and low-income countries.Citation4
In Europe around 250,000 new colon cases are diagnosed each year, accounting for around 9% of all the malignancies.Citation4 Although surgical resection of the primary tumor is the first choice therapy worldwide, an effective approach for the treatment of metastasis and relapses has not been found yet.Citation2
It is now widely accepted that cancer is a multi-step process resulting from the accumulation of both genetic and epigenetic alterations of the genome.Citation3,5,6 Growing lines of evidence suggest that accumulation of genetic and epigenetic alterations in malignant colonic cells progresses through at least 3 distinct pathways: chromosomal instability (CIN), microsatellite instability (MSI), and CpG island methylator phenotype (CIMP).Citation7 In addition to cancer genome abnormalities, also the formation of an inflammatory milieu plays a pivotal role in tumor development and progression.Citation7-9
In fact, the changes occurring in the tumor microenvironment during cancer progression resemble the process associated with chronic inflammation.Citation10,11 The primary role of the inflammatory response is to restrain and eliminate harmful body aggressors; such is the case of transformed cancer cells and the subsequent recruitment of antitumor immune cells to the cancer occurring tissue.Citation10,11 Thus, the tumor microenvironment is composed not only by tumor cells, but also by stromal and inflammatory cells that are recruited and/or locally induced to proliferate or differentiate by tumor cells or by normal cells “educated” by tumor cells. They communicate both directly through cell-cell contact and indirectly through paracrine signals.Citation12 These signals are predominantly constituted by cytokines and chemokines, key orchestrators of leukocytes trafficking under homeostatic conditions as well as during inflammation and cancer.Citation13-16
The cellular component of the inflammatory microenvironment in the tumor includes the presence of host immune cells comprising monocytes/macrophages, dendritic cells (DCs), mast cells, neutrophils, natural killer cells, and T cells.Citation11,17-19 All these cells are differentially distributed within the tumor, reflecting the diversity in tumor biology and tumor-host interactions.Citation20
The interactions between tumor cells and DCs are complex and have yet to be fully elucidated. Understanding the interactions between DCs and tumors is important for comprehending the mechanisms of tumor immune surveillance and escape and provides novel approaches to therapy of cancer.
This review summarizes updated information on the role of the DCs in colon cancer development and/or progression.
Biology of Dendritic Cells
Over the last few years several studies have focused on dendritic cells, the major antigen-presenting cells, which play a pivotal role in the induction of anti-tumor cytotoxic immune responses and further link the adaptive and innate immune systems.Citation21
Characterization of DC populations in humans is challenging due to their low numbers in circulation (less than 1% of blood mononuclear cells) and limited availability of healthy tissues as opposed to animal models.Citation16
Most findings of DC ontogenesis occurred about 20 y ago when the culture conditions to efficiently generate ex vivo large numbers of dendritic cells from mouse bone marrow were identified. Four years later, the corresponding DC production method from human monocytes was published, using a combination of granulocyte macrophage colony stimulating factor (GM-CSF) and interleukin (IL)-4.Citation22-25
However, whether these observations reflect the in vivo characteristics of peripheral DCs is debatable. Moreover, the lack of DC specific markers has meant previous flow cytometric analysis relied on negative selection using a large panel of antibodies to remove lineage-specific cell populations.Citation26
Peripheral blood dendritic cells comprise a heterogeneous group of cell types derived from haematopoietic precursors. They are frequently classified in 2 broad groups according to their lineage, myeloid dendritic cells (mDCs), often referred as conventional DCs, and plasmacytoid dendritic cells (pDCs),Citation22,25 and identified on the basis of different phenotypic markers and immunological activity.Citation27-29
The characterization of pDC lineage has presented a considerable challenge, as pDC display molecular markers and features of several cell types and can be derived from multiple progenitors. Nevertheless, recent advances have firmly established their common developmental origin and genetic relationship with the dendritic cell lineage.Citation30 The pDCs originate in the bone marrow, from both myeloid and lymphoid precursors, although myeloid derivation is predominant, where a dendritic cell progenitor gives rise to both pDCs and classical mDCs.Citation31-33 The developmental pathways of DCs are summarized in . illustrates the immunophenotypic and functional hallmarks of blood DCs.
Figure 1. DC development. This illustration summarizes the current model of the developmental pathways of both myeloid and plasmacytoid DCs. Dashed lines indicate pathways that are likely but not yet definitively shown to operate in DC development. In humans, equivalents of mouse MDP, CDP, and pre-DC have not been found. Cytokines that are important in each transition are indicated. Abbreviations: MPP, multi potential progenitor; CMP, common myeloid progenitor; CLP, common lymphoid progenitor; MDP, macrophage DC progenitor; CDP, common DC progenitor; pre-DC, circulating DC progenitor; pDC, plasmacytoid DC; mDC, myeloid DC; Mo-DC, monocyte-derived DC.
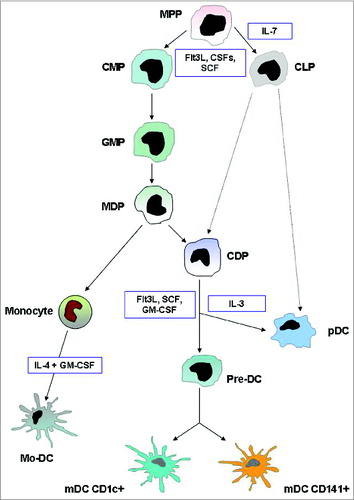
Figure 2. Immunophenotypic and functional hallmarks of DC subsets in blood and lymphoid tissue. (A) Both mDC and pDC cells are effective T-cell stimulators and direct the nature of Th responses, although mDC cells primarily induce Th1 differentiation, whereas pDC cells mainly promote a Th2 response. The archetypical antigen/cytokine of each subset is marked in bold. (B) Key cytokines involved in the DC-mediated polarization of naïve T cells into different T-cell subsets. Mature dendritic cells polarize naive Th0 cells into different Th effector cells through several signals: antigen presentation to the T-cell receptor, co-stimulatory signal and secretion of cytokines. Immature dendritic cells prime Th0 cells to make Treg cells. Abbreviations: pDC, plasmacytoid DC; mDC, myeloid DC; Lin, lineage markers; PRRs, pattern-recognition receptors; TLR, Toll like receptors Th, T helper cell; Treg, regulatory T cell; Ag, antigen, TCR, T-cell receptor; CTLA-4, cytotoxic T-lymphocyte antigen 4.
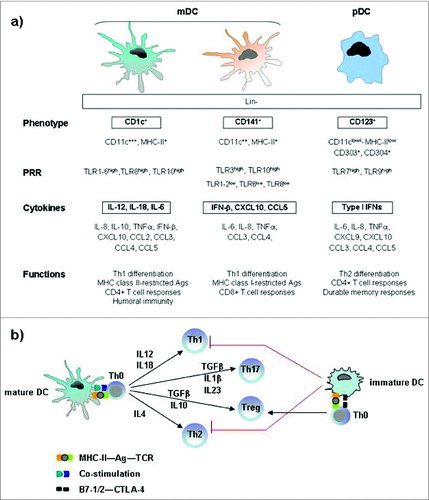
The mDCs express CD11c marker and require GM-CSF for growth and functions such as antigen uptake, T-cell activation and cytokine secretion (IL-6, IL-12 and IL-18). Recently, it has been demonstrated that the mDC subset can be further divided in CD1c+ and CD141+, which show a high level of similarity in protein expression and have also specific functions in the initiation of adaptive immune responses.Citation34 CD1c+ mDCs have been shown to readily stimulate naïve CD4+ T cells and to secrete high amounts of IL-12 in response to toll-like receptor (TLR) ligation, whereas CD141+ DCs do not secrete much IL-12 but they are well equipped to take up dead and necrotic cells for subsequent cross-presentation of derived antigens to CD8+ T cells.Citation34-36
The pDCs express CD123 marker, depend on IL-3 for survival and produce high levels of interferon (IFN)-α,Citation37,38 reflecting their important function in anti-viral immune responses.Citation34,35,39 The released type I IFN by activated pDCs is a pleiotropic cytokine with not only antiviral properties, but it has also been reported to be important for pDC survival, mDC differentiation, mDC-mediated CD4+ and CD8+ T cell-responses, cross presentation, up regulation of co-stimulatory MHC molecules and activation of natural killer (NK), and B cells.Citation34,40-42 Furthermore it has been shown recently that IFN-α possesses also antitumoral activities against several types of cancers, both hematological and solid tumors, by affecting tumor cell survival, proliferation and spreading.Citation43 In addition to type I IFN, pDCs produce other cytokines, including TNF-α, IL-6, and CXCL8,Citation44,45 and inflammatory chemokines such as CXCL9, CXCL10, CCL3, CCL4,and CCL5.Citation33,46,47
The mDC and pDC populations are in close contact in vivo at steady state as well as under inflammatory conditions, and it has been suggested that they act synergistically to induce more potent immuneresponses.Citation34,48,49
DCs in their immature stage are ubiquitously distributed in all peripheral tissues, with a role of sentinels specialized in the uptake and processing of antigen. Upon detection of danger through their pathogen-associated molecular pattern (PAMP) receptors, they mature and migrate to the draining lymph nodes where they present antigens to T cells, generating an antigen-specific response by adaptive immune system.Citation29,50 Both mDCs and pDCs function as APCs following a similar maturation program: they lose immature characteristics, such as phagocytic activity, and acquire several phenotypic and functional features typical of mature DCs, including expression of the co-stimulatory markers CD40, CD80, CD83, and CD86 and the major histocompatibility complex (MHC) class I and II to interact with T cells.Citation29,34,51 However, there are complementary differences especially in the expression of pattern recognition receptors (PRRs) and thus in their response to pathogenic triggers.Citation34 For example, the toll-like receptor (TLR) family is differently expressed in mDCs and pDCs, being TLR7 and 9 selectively expressed in pDCs, and TLR1–6, 8, 10 in mDCs.Citation29,52,53 Both mDC and pDC cells are effective T cell-stimulators.Citation54 They direct the nature of T helper (Th) responses (Th1, Th2, Th17, Treg). It has been demonstrated that mDC cells primarily induce Th1 differentiation, whereas pDC cells mainly promote a Th2 response.Citation53,55
DCs initiate immune responses in a manner that depends on signals they receive from pathogens, surrounding cells and their products.Citation34,56,57 In early studies it was believed that DCs were exclusively immunogenic, actively initiating or up-regulating immune responses.Citation53 More recent reports demonstrated that a perturbation of the homeostatic condition can trigger either an immunogenic (immunostimulatory) or a tolerogenic (immunosuppressive) response, depending on the local circumstances and type of disease.Citation34,53,58 By default, immature pDCs are tolerogenic, whereas activated (mature) pDCs can have both immunogenic and tolerogenic capacities depending on the local environment in which they are activated.Citation34,59,60
Recently new immune regulatory properties of DCs have been identified, so as they are defined regulatory DCs (DCregs). They show a weak capability to activate effector T cells, whereas they induce Treg lymphocytes proliferation and autoreactive T-cells anergy in order to promote immune tolerance.Citation61-63 Under physiological status, DCregs play a pivotal role in terminating inflammatory responses, but it has been shown that also immunogenic DCs can be directed toward the regulatory phenotype by several pathological conditions.Citation63 These findings suggest that DCregs are not a defined DC subset, but rather a functional state that can be acquired by several DC subsets. Over the past years molecules associated with the regulatory function of DCs have been identified, such as CD25, IL-10, TGF-β, IDO and PGE2, but molecular mechanisms involved in DCreg reprogramming are not fully understood.Citation63
Clinical observations showed that DCs possess the ability to migrate directly to a neoplastic site and that the presence of tumor-infiltrating DCs (TIDCs) is associated with the delay of tumor progression and lymphonode metastasis in a variety of solid tumors; particularly the maturation state of DCs represents a potential clinical prognostic factor.Citation58,64-66 Although our views on the potential role of DCs in cancer have expanded remarkably, it is still not fully understood why DCs are unable to induce an efficient immune response. Therefore, understanding the functions of DCs in the tumor microenvironment might represent a rich field of investigation.Citation21
Dendritic Cells in The Cancer Microenvironment
Quantitative and functional impairments of circulating DCs have been widely observed in several types of cancer,Citation38,67-72 and these represent a tumor-escape mechanism employed by cancer cells to elude host immunosurveillance ().Citation73,74
Figure 3. Tumor-altered DC function. Tumor-secreted factors can inhibit DC maturation. Immature DCs, displaying low NF-kB activation, low MHC class II and co-stimulatory molecule expression, are defective antigen presenting cells and induce T cell anergy and exhaustion. Tumor-derived factors can also induce the development of immunosuppressive regulatory DCs, which suppress T cell function through multiple mechanisms. More informations regarding the mechanisms by which tumors alter DC function and suppress host anti-tumor immunity are illustrated in the review of Hargadon.Citation84 Abbreviations: iDC, immature DC; MDC, mature DC; Reg DC, regulatory DC; Treg, regulatory T cell; PD-1L, programmed death 1 ligand.
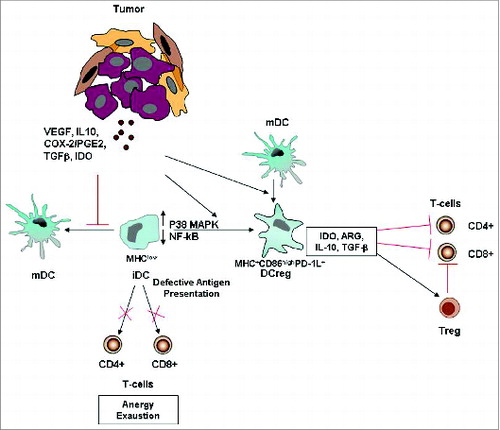
Decreased numbers of mature DCs have been demonstrated in the circulation, tumor bed, and draining lymph nodes, in multiple tumor models.Citation75
In vivo, blood DC numbers have been reported to be altered during the course of various types of hematologic malignancies, in patients with advanced solid tumors such as head and neck squamous cell carcinoma (HNSCC) and in patients with metastatic disease associated with breast cancer and colorectal, gastric, pulmonary, cervical, endometrial and renal cell carcinoma.Citation28,67-72,75-77 The studies analyzing circulating DCs in melanoma patients have reported conflicting data.Citation28,78-80 In a recent studyCitation28 we detected a significant decrease of circulating DCs in melanoma patients, compared to healthy volunteers. This reduction mainly affected pDCs and was ascribable only to the more advanced stage of disease.Citation28
DC subset analysis in patients with breast cancer and HNSCC has revealed that decreases in cell numbers are confined to DCs of the myeloid lineage, in comparison with those of the plasmacytoid or lymphoid lineage.Citation75,81 Minimal DCs are recruited to the tumor bed in patients with renal and prostate cancer, presenting low levels of costimulatory molecules and a decreased capacity to stimulate allogeneic T-cell proliferation.Citation75,82,83
The majority of studies have described soluble factors derived from both tumors and associated cells within the tumor microenvironment that interfere with DC differentiation from precursors, thereby contributing to a loss of stimulatory APC activity in tumor-bearing hosts.Citation84 Tumor-associated DCs show decreased uptake, processing and presentation of antigens, lowered expression of co-stimulatory signal, inefficient motility and migration toward specific chemokines, suppressed endocytic potential and decreased production of IL-12.Citation58,76,85-88
Regardless of the mechanism of inhibition, the loss of APC function associated with suppressing DC differentiation may significantly limit the induction of anti-tumor immune responses and greatly contribute to tumor immune escape.Citation84
A variety of tumor-derived factors that disrupt DC maturation and function have been identified.Citation75 Among cytokines, IL-10 plays important role in DC defects.Citation53 It irreversibly blocks the monocyte-to-DC differentiation, driving the differentiation process toward the macrophage lineage and impair the potent APC function of DC.Citation53,68,89 Beckebaum et al.Citation90 indicated that increased serum levels of IL-10 correlated with numerical deficiency and immature phenotype of circulating DC in patients with hepatocellular carcinoma.Citation68,90 IL-6 and M-CSF have been shown to block the differentiation of CD34+ progenitors into DCs and instead trigger their commitment toward CD14+ monocytes that express little to no MHC and costimulatory molecules and that fail to induce allogenic T cell proliferation in mixed leukocyte reaction (MLR) assays.Citation68,84,91,92
Similar inhibition of CD34+ precursor cell differentiation into DC has been attributed to tumor-derived VEGF.Citation84,93 Moreover, elevated levels of VEGF associate with increased number of immature APC with a suppressive phenotype in the circulation of cancer patients.Citation68 Transforming growth factor (TGF)-β, produced by progressor tumors, has been demonstrated to immobilize dendritic cells within these tumors and inhibit DC migration from tumors to their draining lymph nodes (TDLNs), faciliting metastasis in the affected nodes.Citation94
In addition to secreting cytokines, tumors may also secrete other factors, including gangliosides, prostanoids, and polyamines, that have been demonstrated to affect DC differentiation from progenitors, both in vitro and in vivo.Citation53,68 The gangliosides GD2 and GM3 secreted by human and murine neuroblastoma cell lines have been shown to inhibit differentiation of DC from CD34+ progenitors;Citation95 the gangliosides GM3 and GD3, secreted by human melanomas, have been demonstrated inhibit DC differentiation from monocytic precursors.Citation96
Using both the in vitro and ex-vivo models, Esche et al.Citation97 reported that tumor-derived factors could induce apoptosis in murine and human DCs, and acceleration of DC turnover.Citation58,97,98 Among the above mentioned tumor-derived factors, gangliosides are also known as inducers of DC apoptosis at any step of DC differentiation in a dose-dependent fashion.Citation68,99
In addition to the inhibitory effects of tumor-derived and tumor-associated factors on DC differentiation that preclude the development of cells with APC function, these factors can also promote the recruitment and accumulation of functionally deficient and frequently immature DCs.
It has been demonstrated that certain subsets of immature DCs fail to provide an appropriate costimulatory and cytokine signals to T cells, so tolerance or anergy may develop.Citation58,68 This might induce tolerance through abortive proliferation or anergy of antigen-specific CD4+ and CD8+ T cells in vivo or through the generation of regulatory T cells that prevent immune responses by producing IL-10 and TGF-β.Citation58,68,100,101
The impairment of the differentiation program of DC precursors, can promote also the accumulation of an other subset of cells with immunosuppressive function, i.e. the myeloid-derived suppressor cells (MDSCs). These cells have been detected in various types of cancer, recovered at high levels from both tumors and tumor-draining lymph nodes and found to be associated with a poor prognosis and resistance to therapies.Citation28,102-105 MDSCs are arrested in an immature state and poorly differentiate along the DC or macrophage lineage even in the presence of cytokines or differentiating agents released by tumors and tumor-associated cells.Citation28,84,106,107 They are important immune regulators and play a key role in tumor-induced suppression of T-responses via multiple mechanisms.Citation28,102 It has been reported that they can induce a direct tolerization of anti-tumor T lymphocyte responses and also the development of Foxp3+-regulatory T cells (Tregs).Citation84,103 In addition, it has been shown that MDSCs may also have indirect effects on T cells, by impeding the maturation and T-cell stimulatory capacity of DCs.Citation84,102 MDSCs also promote tumor progression by polarizing helper and cytotoxic T cells toward a type 2 response, through their cross-talk with macrophages by reducing IL-12 macrophage production and by increasing MDSC production of IL-10.Citation108,109 The type 2 responses are less robust in their anti-tumor efficacy.Citation84
Tumors not only suppress DC maturation but they can also induce the development of DCs with regulatory function, that actively display immunosuppressive activity themselves.Citation84 In fact, it has recently demonstrated that the ovarian carcinoma progression is controlled by phenotypic changes in dendritic cells.Citation110
Recently, several studies have shown a wide heterogeneity within DCs with regulatory properties, depending on the signals they receive from the microenvironment and on the immunological response they build up toward them. However they all share some characteristics in common, that are both modulating and inhibiting T-cell activation and inducing Treg proliferation and activity.Citation63 The high degree of plasticity of DCs toward the environmental milieu can determine a complete re-polarization of even fully mature DCs toward a tolerogenic phenotype.Citation58
Moreover, it has been reported that high pDC infiltration can be observed in many types of cancer including melanoma, head and neck cancer, ovarian and prostate cancer, and these infiltrates mostly negatively correlate with patient survival.Citation34,48 pDCs have been documented also in tumor draining, whereas no data are available for pDCs in distant tumor metastasis.Citation48 These pDCs infiltrated in tumor microenvironment are mainly immature, and therefore seem to be predominantly immunosuppressive/tolerogenic.Citation34,43
Conclusively, DCs play a relevant role both in the tumor microenvironment and systemic circulation, exerting differential pro-tumorigenic and anti-tumorigenic functions, which might be due to the plasticity of these cells capable of reacting to and integrating environmental signals.Citation63
Dendritic Cells in Colorectal Cancer
Colorectal cancer has long been considered scarcely immunogenic, but several findings have shown that anti-tumor immune responses might occur in patients.Citation111,112 Similar to other malignancies, CRC antigens evoke DC recruitment, maturation, and cytokine release in order to generate effective Th1-type immune response.Citation65,113 However, CRC cancer cells use diverse strategies to inhibit the local tumor-specific immunity in order to escape attack and prolong cell survival resulting in an immune dysfunction in the tumor microenvironment.Citation113,114 The mechanism of colorectal cancer immune evasion is multifactorial and involves also defects of dendritic cells.
The infiltration rate and the local distribution of DCs in CRC tissue have been largely investigated.Citation65,111,115-117 It was reported that metastasis and tumor stage related with the infiltrating degree of DCs in the tumor tissue.Citation65,118
Recently, Gulubova et al.Citation65 evaluated the association between the presence and maturation status of tumor-infiltrating DCs in different tumor compartments, with some clinical and pathological variables of the patients with colon cancer. They showed that patients with locally advanced tumors (T3–T4) had significantly lower infiltration with CD83+-matured DCs in tumor stroma, and in the invasive margin. The frequency of distant metastases was significantly associated with lower infiltration with CD1a-positive immature DCs in tumor stroma and lower infiltration with CD83-positive DCs in invasive margin.Citation65
According with some previous observations,Citation114,119,120 it has been suggested that this apparent distribution pattern in tumor stroma of CRC might represent a moving of mature DCs from tumor nests to the peripheral lymph nodes for antigen presentation.Citation65
The number of tumor infiltrating dendritic cells (TIDCs) in patients with colorectal cancer, as well as in other malignancies, was negatively correlated with lymph node metastasis, size of tumor, and survival time.Citation65,115,119
The findings concerning DC's numbers correlated to patients’ survival are controversial, sometimes being quite contradictory.Citation65,115,119,121
Dadabayev et al.Citation119 found that the correlation between the degree of tumor infiltration of DCs and the prognosis depends on the level of maturation of these cells. In fact, only high intraepithelial infiltration with immature DCs tended to correlate with a longer disease-free survival, while the presence of mature (i.e., HLA-DR positive) DCs in the stroma showed an opposite effect on patient survival. Similar data have been reported also by Nagorsen et al.Citation115 Sandel et al.Citation121 showed that the number of immature DCs in the tumor did not affect the disease outcome; patients with a relatively higher number of mature (i.e., CD208-positive) infiltrating DCs in the tumor epithelium had a shorter overall survival and patients with higher numbers of immature (i.e., CD1a positive) DCs in the advancing margin of the tumor had a shorter disease-free survival.Citation65,121
As discussed in the previous section, tumors can implement various mechanisms suppressing the activation of DCs in order to escape immune recognition and elimination. Altered immune cellular components and/or dysregulated cytokine levels might be present in the tumor microenvironmentCitation122-124 and even in precancerous lesions such as colorectal adenoma.Citation114,125 Previous studies, reported decreased mature DC density in both human colon cancers and experimental rat colon cancer models.Citation114 Schwaab et al.Citation120 showed that the number of infiltrating mature DCs, in human primary colorectal cancer specimens, was 3 times lower than in normal colonic mucosa normal colonic mucosa and that DC density in metastases was fold6- lower than in colorectal primary tumors. The same Authors reported that the increase in the density of mature DCs was associated with the expression of VEGF.Citation120
In addition to the density changes, multiple factors secreted by the tumor and at a systemic level can modulate also the function of tumor infiltrating DCs.Citation84,114,126 Recently, Michielsen et al.Citation127 found that tumor conditioned-media (TCM), obtained from cultured human colorectal tumor explant tissue, alters DC maturation and function. These Authors suggest that TCM components, such as CCL2, CXCL1, CXCL5 and VEGF may play a role in modulating the inflammatory response through inhibition of IL-12p70 secretion by DCs.Citation127,128
It has been largely demonstrated that upregulation of COX-2, that is currently recognized as an important target for the prevention of CRC,Citation129,130 is associated to increased risk of CRC.Citation114,126,131 Yuan et al.Citation114 show that the DC infiltration pattern is altered along the adenoma-carcinoma sequence and that the COX-2 increase might contribute to the functional defect of DCs in CRC. One potential mechanism evocated about the role of COX-2 in the CRC promotion is the inhibition of host anti-tumor immunity by suppressing DC differentiation, maturation and function through the downstream signal molecule PGE2 and its receptors EP2/EP4.Citation114,132 Apoptosis represents a mechanism of DC elimination in the tumor environment.Citation58
Ishida et al.Citation133 demonstrated that MUC2 mucins purified from the conditioned medium of a colorectal cancer cell line increased the number of apoptotic cells in human monocyte-derived DC cultures. These authors suggested that the interaction between mucins and Siglec-3 expressed on monocytes/DCs may be partially related to this process.Citation133
Moreover, the most important member of the high mobility group box protein family, HMGB1, a multifunctional cytokine secreted by cancer cells, has a role in cancer progression, angiogenesis, invasion, metastasis development and in inducing apoptosis in macrophages and DCs.Citation58
HMGB1 is able to inhibit apoptosis by different pathways;Citation134 its overexpression suppresses caspase-3 and caspase-9 activity, thus, inhibiting significant steps in apoptosis. In colorectal cancer, cytochrome apoptosis inhibitor protein 2 (c-IAP2) levels are related to HMGB1 expression.Citation134,135 Kusume et al.Citation136 demonstrated that CD205-positive intra-tumor DC number decrease in patients with colorectal cancer with HMGB1 over-expression and lymph node metastasis, suggesting that HMGB1 produced by colon cancer cells suppressed nodal DCs.Citation58,134,136
The tumor-induced inhibition of dendritic cells and other cells of the immune system may occur in the tumor microenvironment as well as in the systemic circulation.Citation53,72,74,75 However, few studies have been performed on the expression of DCs in CRC patients at circulating levels.Citation2,72,137,138
An early study of Huang et al.Citation137 demonstrated that circulating DC levels in patients with colorectal cancer were reduced to about 60% of control levels, and complete colorectal cancer removal restored circulating DC levels to the normal range. This significant reduction in the level of circulating DCs was associated with an increased infiltration of Langerhans cells into colorectal mucosa, and it was correlated with a significant increase in the serum level of TGFβ1.
Della Porta et al.Citation138 reported a numerical and functional impairment of peripheral blood DC compartment, related to the stage of the disease and to VEGF levels, suggesting a possible effect of this cytokine in the numerical and functional impairment of DCs in colorectal cancer patients.
Recently, we focused on the quantification of circulating dendritic cells in CRC patients, categorized according to the stage of disease, at both pre- and post-operative time points.Citation72 In line with the above results, we found a significant reduction of the DC number in total and advanced stage-CRC patients compared to healthy controls. This significant reduction was totally recovered after complete tumor resection, suggesting a systemic immunosuppressive effect exerted by the tumor toward circulating DCs. Interestingly, the analysis of DC subset behavior revealed that the reduction in DCs was primarily due to changes in pDCs population.Citation72
Concerning DC subsets, Bellik et al.Citation2 reported that both mDCs and pDCs in CRC patients, with and without metastasis, were significantly reduced compared to healthy subjects. However, they showed that the numbers of both DC subsets were significantly higher in metastatic than in patients without metastasis. Differences between our study and Bellik's one may be attributable to the different methodological approach used, because they isolated PBMCs before the flow cytometric DC counting.Citation72
The observed decrease of circulating DCs may have functional consequences on the production of cytokines and on antigen presentation to T cells. However, data about the phenotype and function of circulating DCs in cancer patients, especially about the pDC subset, are still very limited.Citation48
Interestingly, in our experimental approach we chose a DC gating strategy based on the expression of CD85 k, a DC antigen related to immunosuppressive and tolerogenic functions.Citation72,139 In the same study we showed that CD85 k expression on myeloid DCs was significantly higher in CRC patients compared with controls. This result suggested a more immunosuppressive nature of the myeloid DC subtype in cancer subjects that may contribute to affect the host immune reaction against the tumor.Citation72
Several in vitro experiments have also demonstrated impairments of DC differentiation from CD14+ monocyte precursors, and of DC maturation in cancer patients; both mechanisms lead to dysfunctional DCs that cannot present antigens, thus inducing tolerogenicity.Citation111,140-142 However, these studies showed that there are differences in the quality of the monocyte-derived DCs dependent on the type of cancer, and that, in general, monocytes from cancer patients are less susceptible to cytokine stimulation compared to monocytes from healthy donors.Citation143 Conversely, few studies have been performed on the abilities of CRC patients to generate DCs from circulating monocytes.Citation2,98,111,144
Recently, we showed an impaired in vitro differentiation of CRC patients’ monocytes into immature DCs, compared to healthy subjects.Citation111 Furthermore, the cultured cells were characterized by persistent CD14 expression and higher endocytic activity, reduced ability to present antigens to allogenic T lymphocytes and to stimulate proliferation, together with a significantly altered co-stimulatory molecule expression compared to controls. Accordingly, we observed a markedly immunosuppressive cytokine profile in the patient DCs, characterized by increased IL-10, and reduced IL-12 and TNF-α secretion.Citation111 These results were in agreement with those of Michielsen et al,Citation127 which showed that CRC-conditioned medium negatively influenced DC maturation and IL-12 secretion, while augmented IL-10 secretion. Moreover, these negative effects exerted by the tumor on DC generation and maturation appeared correlate with the disease stage.Citation111
Bellik et al.Citation2 reported an impaired developmental capacity of DCs from monocytes of both surgically-resected and CRC-bearing patients compared with healthy subjects. Furthermore, they showed that chemotherapy significantly affected both circulating DC numbers and monocyte-derived DCs. Kvistborg et al.Citation143 observed that the maturation status of the monocyte-derived DCs correlated with the clinical status of the patients, but however resulting phenotypically and functionally superior to in vivo DCs in the same cancer patients. Thus the authors suggested that monocyte-derived DCs are good candidates for adjuvant cell-based cancer vaccine protocols, after an accurate selection of the cancer patients, based on their immune status.Citation143
Conclusion
This review focus on dendritic cells as they are central players at the interface of the innate and adaptive immunity and play important roles in the establishment and persistence of cancer-induced immunosuppression. During the priming phase of the anti-tumor response, occurring in lymph nodes, DC dysfunction is due to inability to cross-presentation and to inadequate expression of costimulatory ligands. During the effector phase, operating within tumor mass, DC dysfunction involves: inadequate recruitment of activated effector T cells, presence of dominant immune inhibitory mechanisms capable of abrogating T cell effector function (e.g. the inhibitory receptors such as anti-cytotoxic T-lymphocyte antigen 4, CTLA-4, and programmed death 1, PD-1), extrinsic suppressive cells (Tregs, myeloid-derived suppressor cells), metabolic inhibitors (IDO, arginase), and inhibitory cytokines (IL-10, TGF-β).Citation145
These data suggest that there could be potential biomarkers for checkpoint blockade therapy in cancer. Current preclinical studies are combining checkpoint blockade with tyrosine kinase inhibitors, radiation therapy and cancer vaccines ().Citation145
Figure 4. The immune checkpoints CTLA-4 and PD-1/PD-L1. Inhibitory receptors such as anti-cytotoxic T-lymphocyte antigen 4 and programmed death 1 expressed on tumor-specific T cells lead to compromised activation and suppressed effector functions such as proliferation, cytokine secretion, and tumor cell lysis. Modulating these receptors using monoclonal antibodies, an approach termed “immune checkpoint blockade,” has gained momentum as a new approach in cancer immunotherapy. Abbreviations: CTLA-4, cytotoxic T-lymphocyte antigen 4; PD-1, programmed death 1.
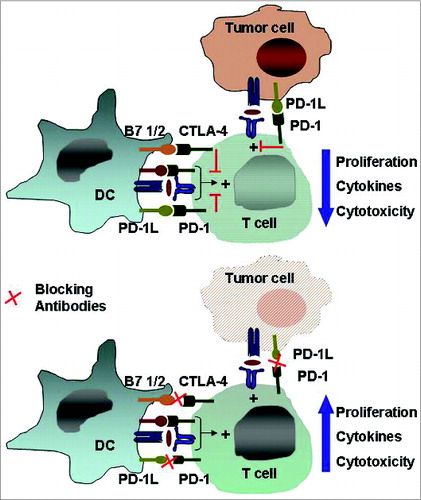
In the CRC, surgical resection is the primary treatment and is potentially curative. However, a significant proportion of patients present with disseminated disease and over half of all patients develop recurrence and die of their disease following surgery.Citation146 Thus, in the management of colorectal cancer alternative therapeutic strategies, such as the application of immunotherapy, are clearly needed.
The fully human anti–PD-1 mAb BMS-936558/MDX-1106/ONO-4538 (nivolumab), has demonstrated antitumor activity in phase 1/1 b studies in CRC, as well renal cell cancer, melanoma and non-small cell lung cancer. The humanized anti–PD-1 antibody MK-3945 (lambrolizumab) has also demonstrated antitumor activity in patients with advanced solid cancers, including CRC, in a phase 1 study.Citation145,147
Dendritic cells are considered as promising candidates in cancer vaccines of CRC patients, to generate host immune responses against tumor antigens, and encouraging results have been obtained.Citation114 However, in spite of available information, many clinical protocols utilizing DC-based vaccines do not consider the fact that DCs administered in patients with cancer might quickly lose their activity in the cancer environment.Citation58 The existence of functionally defective DC in cancer patients strongly emphasizes the rationale of developing DC-based immune therapy to restore proper presentation of tumor associated antigens and T cell activation.Citation143 In fact, taking account of pivotal role of pDCs in ensuring an immune response, by strong IFN-α release and direct cell-cell interactions, Tel et al.Citation39 demonstrated that anti-cancer immune responses may be initiated or boosted by vaccination with autologous tumor antigen loaded pDCs.Citation34,39
Additionally, the DC-Treg interactions, by enhancing tumor-induced immunosuppression, represent a major barrier to successful immunotherapy. Targeting the generation of these 2 suppressive cell populations would be a desiderable goal in chemo-and immunotherapeutic approaches. Therefore, to achieve this objective there is a need to further improve strategies to simultaneously promote the full activation of DC using selective adjuvants such as TLR ligands or cytokines and impair Treg expansion, function and recruitment.Citation148
The presence of TLR ligands as adjuvant in the vaccine strategy has the potential to increase the efficacy of immunization toward a given antigen. TLR activation strategies have been used in both established and experimental vaccines for infectious or non-infectious diseases, as well as for cancer treatment.Citation149 In a recent work, Fang et al,Citation149 demonstrate that danger-associated molecular patterns, released from chemically (oxaliplatin and/or 5-fluorouracil) stressed CRC cells, can induce mouse and human DC activation and maturation via TLR4 and enhance the induction of an anti-tumor T-cell immune response both in vitro and in vivo. Recently, the results of Gao et al,Citation150 indicate that DC vaccine combined with killer cells, generated ex-vivo by cytokines (IL-2, IL-1β, IFN-γ), may improve the disease-free survival in gastric and colorectal cancer.
In conclusion, the knowledge of the mechanisms, aimed to reverse the phenotype of DCs from an immunosuppressive to an immunostimulatory cell type, may be relevant to guide the design of more efficient protocols for cancer immunotherapy and to identify possible candidates for DC-based immunotherapy.
Disclosure of Potential Conflicts of Interest
No potential conflicts of interest were disclosed.
Author Contributions
All listed authors have reviewed and approved the final version of the manuscript. All authors contributed to the work presented in this paper.
References
- Harrison S, Benziger H. The molecular biology of colorectal carcinoma and its implications:A review. Surgeon 2011; 9:200-210; PMID:21672660; http://dx.doi.org/10.1016/j.surge.2011.01.011
- Bellik L, Gerlini G, Parenti A, Ledda F, Pimpinelli N, Neri B, Pantalone D. Role of conventional treatments on circulating and monocyte-derived dendritic cells in colorectal cancer. Clin Immunol 2006; 121:74-80; PMID:16914380; http://dx.doi.org/10.1016/j.clim.2006.06.011
- Failli A, Legitimo A, Migheli F, Coppedè F, Mathers JC, Spisni R, Miccoli P, Migliore L, Consolini R. Efficacy and feasibility of the epithelial cell adhesion molecule (EpCAM) immunomagnetic cell sorter for studies of DNA methylation in colorectal cancer. Int J Mol Sci 2014; 15:44-57; http://dx.doi.org/10.3390/ijms15010044
- Labianca R, Beretta GD, Kildani B, Milesi L, Merlin F, Mosconi S, Pessi MA, Prochilo T, Quadri A, Gatta G, et al. Colon cancer. Crit Rev Oncol Hematol 2010; 74:106-133; PMID:20138539; http://dx.doi.org/10.1016/j.critrevonc.2010.01.010
- Dawson MA, Kouzarides T. Cancer epigenetics:From mechanism to therapy. Cell 2012; 150:12-27; PMID:22770212; http://dx.doi.org/10.1016/j.cell.2012.06.013
- Deschoolmeester V, Baay M, Van Marck E, Weyler J, Vermeulen P, Lardon F, Vermorken JB. Tumor infiltrating lymphocytes:an intriguing player in the survival of colorectal cancer patients. BMC Immunol 2010; 11:19; PMID:20385003; http://dx.doi.org/10.1186/1471-2172-11-19
- Pancione M, Giordano G, Remo A, Febbraro A, Sabatino L, Manfrin E, Ceccarelli M, Colantuoni V. Immune escape mechanisms in colorectal cancer pathogenesis and liver metastasis. J Immunol Res 2014; 2014: Article ID 686879. Eleven pages; http://dx.doi.org/10.1155/2014/686879
- Balkwill F, Charles KA, Mantovani A. Smoldering and polarized inflammation in the initiation and promotion of malignant disease. Cancer Cell 2005; 7:211-217; PMID:15766659; http://dx.doi.org/10.1016/j.ccr.2005.02.013
- Mantovani A, Allavena P, Sica A, Balkwill F. Cancer related inflammation. Nature 2008; 454:436-444; PMID:18650914; http://dx.doi.org/10.1038/nature07205
- Whiteside TL. The tumor microenvironment and its role in promoting tumor growth. Oncogene 2008; 27:5904-5912; PMID:18836471; http://dx.doi.org/10.1038/onc.2008.271
- Krstic J, Santibanez JF. Transforming growth factor-beta and matrix metalloproteinases:Functional interactions in tumor stroma-infiltrating myeloid cells. ScientificWorld J 2014; 2014:521754; http://dx.doi.org/10.1155/2014/521754
- Jain RK. Normalizing tumor microenvironment to treat cancer:bench to bedside to biomarkers. J Clin Oncol 2013; 31:2205-2218; PMID:23669226; http://dx.doi.org/10.1200/JCO.2012.46.3653
- Zlotnik A, Yoshie O. The chemokine superfamily revisited. Immunity 2012; 36:705-716; PMID:22633458; http://dx.doi.org/10.1016/j.immuni.2012.05.008
- Candido J, Hagemann T. Cancer-related inflammation. J Clin Immunol 2013; 33:S79-S84; PMID:23225204; http://dx.doi.org/10.1007/s10875-012-9847-0
- Aldinucci D, Colombatti A. The inflammatory chemokine CCL5 and cancer progression. Mediators Inflamm 2014; 2014: Article ID 292376, 12 pages; http://dx.doi.org/10.1155/2014/292376
- Benencia F, Sprague L, McGinty J, Pate M, Muccioli M. Dendritic cells the tumor microenvironment and the challenges for an effective antitumor vaccination. J Biomed Biotechnol 2012; 2012:425476; PMID:22505809; http://dx.doi.org/10.1155/2012/425476
- Allavena P, Sica A, Solinas G, Porta C, Mantovani A. The inflammatory micro-environment in tumor progression:the role of tumor-associated macrophages. Crit Rev Oncol Hematol 2008; 66:1-9; PMID:17913510; http://dx.doi.org/10.1016/j.critrevonc.2007.07.004
- Ansell SM, Vonderheide RH. Cellular composition of the tumor microenvironment. Am Soc Clin Oncol Educ Book 2013; 2013:91-97; http://dx.doi.org/10.1200/EdBook_AM.2013.33.e91
- Bennett CL, Chakraverty R. Dendritic cells in tissues:in situ stimulation of immunity and immunopathology. Trends Immunol 2012; 33:8-13; PMID:22030236; http://dx.doi.org/10.1016/j.it.2011.09.008
- Galon J, Fridman W-H, Pagès F. The adaptive immunologic microenvironment in colorectal cancer:a novel perspective. Cancer Res 2007; 67:1883-1886; PMID:17332313; http://dx.doi.org/10.1158/0008-5472.CAN-06-4806
- Palucka K, Banchereau J. Cancer immunotherapy via dendritic cells. Nat Rev Cancer 2012; 12:265-277; PMID:22437871; http://dx.doi.org/10.1038/nrc3258
- Escors D. Tumour immunogenicity, antigen presentation, and immunological barriers in cancer immunotherapy. New J Sci 2014; 2014: Article ID 734515, 25 pages; http://dx.doi.org/10.1155/2014/734515
- Inaba K, M. Inaba N, Romani N, Aya H, Deguchi M, Ikehara S, Muramatsu S, Steinman RM. Generation of large numbers of dendritic cells from mouse bone marrow cultures supplemented with granulocyte/macropha.ge colony-stimulating factor. J Exp Med 1992; 176:1693-1702; PMID:1460426; http://dx.doi.org/10.1084/jem.176.6.1693
- Zhou LJ, Tedder TF. CD14+ blood monocytes can differentiate into functionally mature CD83+ dendritic cells. Proc Natl Acad Sci U S A 1996; 93:2588-2592; PMID:8637918; http://dx.doi.org/10.1073/pnas.93.6.2588
- Breckpot K, Escors D. Dendritic cells for active anticancer immunotherapy:targeting activation pathways through genetic modification. Endocr Metab Immune Disord Drug Targets 2009; 9:328-343; PMID:19857199; http://dx.doi.org/10.2174/187153009789839156
- Chowdhury F, Johnson P, Williams AP. Enumeration and phenotypic assessment of human plasmacytoid and myeloid dendritic cells in whole blood. Cytometry A 2010; 77:328-337; PMID:20140969; http://dx.doi.org/10.1002/cyto.a.20872
- Schäkel K. Dendritic cells-why can they help and hurt us. Exp Dermatol 2009; 18:264-273; http://dx.doi.org/10.1111/j.1600-0625.2008.00823.x
- Failli A, Legitimo A, Orsini G, Romanini A, Consolini R. Numerical defect of circulating dendritic cell subsets and defective dendritic cell generation from monocytes of patients with advanced melanoma. Cancer Lett 2013; 337:184-192; PMID:23684927; http://dx.doi.org/10.1016/j.canlet.2013.05.013
- Orsini G, Legitimo A, Failli A, Massei F, Biver P, Consolini R. Enumeration of human peripheral blood dendritic cells throughout the life. Int Immunol 2012; 24:347-356; PMID:22345276; http://dx.doi.org/10.1093/intimm/dxs006
- Reizis B. Regulation of plasmacytoid dendritic cell development. Curr Opin Immunol 2010; 22:206-211; PMID:20144853; http://dx.doi.org/10.1016/j.coi.2010.01.005
- Liu K, Victora GD, Schwickert TA, Guermonprez P, Meredith MM, Yao K, Chu FF, Randolph GJ, Rudensky AY, Nussenzweig M. In vivo analysis of dendritic cell development and homeostasis. Science 2009; 324:392-397; PMID:19286519; http://dx.doi.org/10.1126/science.1171243
- Naik SH, Sathe P, Park HY, Metcalf D, Proietto AI, Dakic A, Carotta S, O’Keeffe M, Bahlo M, Papenfuss A, et al. Development of plasmacytoid and conventional dendritic cell subtypes from single precursor cells derived in vitro and in vivo. Nat Immunol 2007; 8:1217-1226; PMID:17922015; http://dx.doi.org/10.1038/ni1522
- Sozzani S, Vermi W, Del Prete A, Facchetti F. Trafficking properties of plasmacytoid dendritic cells in health and disease. Trends Immunol 2010; 31:270-277; PMID:20579936; http://dx.doi.org/10.1016/j.it.2010.05.004
- Mathan TS, Figdor CG, Buschow SI. Human plasmacytoid dendritic cells:from molecules to intercellular communication network. Front Immunol 2013; 4:372; PMID:24282405; http://dx.doi.org/10.3389/fimmu.2013.00372
- Merad M, Sathe P, Helft J, Miller J, Mortha A. The dendritic cell lineage:ontogeny and function of dendritic cells and their subsets in the steady state and the inflamed setting. Annu Rev Immunol 2013; 31:563-604; PMID:23516985; http://dx.doi.org/10.1146/annurev-immunol-020711-074950
- Crozat K, Guiton R, Guilliams M, Henri S, Baranek T, Schwartz-Cornil I, Malissen B, Dalod M. Comparative genomics as a tool to reveal functional equivalences between human and mouse dendritic cell subsets. Immunol Rev 2010; 234:177-198; PMID:20193019; http://dx.doi.org/10.1111/j.0105-2896.2009.00868.x
- MacDonald KPA, Munster DJ, Clark GJ, Dzionek A, Schimitz J, Hart DJ. Characterization of human blood dendritic cells subsets. Blood 2002; 100:4512-4520; PMID:12393628; http://dx.doi.org/10.1182/blood-2001-11-0097
- Sciarra A, Lichtner M, Autran GA, Mastroianni C, Rossi R, Mengoni F, Cristini C, Gentilucci A, Vullo V, Di Silverio F. Characterization of circulating blood dendritic cell subsets DC123+ (lymphoid) and DC11C +(myeloid) in prostate adenocarcinoma patients. Prostate 2007; 67:1-7; PMID:17075798; http://dx.doi.org/10.1002/pros.20431
- Tel J, Schreibelt G, Sittig SP, Mathan TS, Buschow SI, Cruz LJ, Lambeck AJ, Figdor CG, de Vries IJ. Human plasmacytoid dendritic cells efficiently cross-present exogenous Ags to CD8+ T cells despite lower Ag uptake than myeloid dendritic cell subsets. Blood 2013; 121:459-467; PMID:23212525; http://dx.doi.org/10.1182/blood-2012-06-435644
- LeBon A, Etchart N, Rossmann C, Ashton M, Hou S, Gewert D, Borrow P, Tough DF. Cross-priming of CD8+ T cells stimulated by virus-induced type I interferon. Nat Immunol 2003; 4:1009-1015; PMID:14502286; http://dx.doi.org/10.1038/ni978
- LeBon A, Schiavoni G, D’Agostino G, Gresser I, Belardelli F, Tough DF. Type I interferons potently enhance humoral immunity and can promote isotype switching by stimulating dendritic cells in vivo. Immunity 2001; 14:461-470; PMID:11336691; http://dx.doi.org/10.1016/S1074-7613(01)00126-1
- Montoya M, Schiavoni G, Mattei F, Gresser I, Belardelli F, Borrow P, Tough DF. Type I interferons produced by dendritic cells promote their phenotypic and functional activation. Blood 2002; 99:3263-3271; PMID:11964292; http://dx.doi.org/10.1182/blood.V99.9.3263
- Demoulin S, Herfs M, Delvenne P, Hubert P. Tumor microenvironment converts plasmacytoid dendritic cells into immunosuppressive/tolerogenic cells:insight into the molecular mechanisms. J Leukoc Biol 2013; 93:343-352; PMID:23136258; http://dx.doi.org/10.1189/jlb.0812397
- Liu YJ. IPC:professional type 1 interferon-producing cells and plasmacytoid dendritic cell precursors. Annu Rev Immunol 2005; 23:275-306; PMID:15771572; http://dx.doi.org/10.1146/annurev.immunol.23.021704.115633
- Decalf J, Fernandes S, Longman R, Ahloulay M, Audat F, Lefrerre F, Rice CM, Pol S, Albert ML. Plasmacytoid dendritic cells initiate a complex chemokine and cytokine network and are a viable drug target in chronic HCV patients. J Exp Med 2007; 204:2423-2437; PMID:17893202; http://dx.doi.org/10.1084/jem.20070814
- Swiecki M, Colonna M. Unraveling the functions of plasmacytoid dendritic cells during viral infections, autoimmunity, and tolerance. Immunol Rev 2010; 234:142-162; PMID:20193017; http://dx.doi.org/10.1111/j.0105-2896.2009.00881.x
- Penna G, Vulcano M, Roncari A, Facchetti F, Sozzani S, Adorini L. Cutting edge:differential chemokine production by myeloid and plasmacytoid dendritic cells. J Immunol 2002; 169:6673-6676; PMID:12471096; http://dx.doi.org/10.4049/jimmunol.169.12.6673
- Vermi W, Soncini M, Melocchi L, Sozzani S, Facchetti F. Plasmacytoid dendritic cells and cancer. J Leukoc Biol 2011; 90:681-90; PMID:21730085; http://dx.doi.org/10.1189/jlb.0411190
- Perez-Cabezas B, Naranjo-Gomez M, Ruiz-Riol M, Bastos-Amador P, Fernandez MA, Carmona F, Nuñez F, Pujol-Borrell R, Borràs FE. TLR-activated conventional DCs promote gamma-secretase-mediated conditioning of plasmacytoid DCs. J Leukoc Biol 2012; 92:133-143; PMID:22534476; http://dx.doi.org/10.1189/jlb.0911452
- Charbonneau B, Goode EL, Kalli KR, Knutson KL, Derycke MS. The Immune System in the Pathogenesis of Ovarian Cancer. Crit Rev Immunol 2013; 33:137-164; PMID:23582060; http://dx.doi.org/10.1615/CritRevImmunol.2013006813
- Manh TP, Alexandre Y, Baranek T, Crozat K, Dalod M. Plasmacytoid, conventional, and monocyte-derived dendritic cells undergo a profound and convegent genetic reprogramming during their maturation. Eur J Immunol 2013; 43:1706-1715; PMID:23553052; http://dx.doi.org/10.1002/eji.201243106
- Hornung V, Rothenfusser S, Britsch S, Krug A, Jahrsdörfer B, Giese T, Endres S, Hartmann G. Quantitative expression of toll-like receptor 1-10 mRNA in cellular subsets of human peripheral blood mononuclear cells and sensitivity to CpG oligodeoxynucleotides. J Immunol 2002; 168:4531-4537; PMID:11970999; http://dx.doi.org/10.4049/jimmunol.168.9.4531
- Fricke I, Gabrilovich DI. Dendritic cells and tumor microenvironment:a dangerous liaison. Immunol Invest 2006; 35:459-483; PMID:16916762; http://dx.doi.org/10.1080/08820130600803429
- Lou Y, Liu C, Kim GJ, Liu YJ, Hwu P, Wang G. Plasmacytoid Dendritic Cells Synergize with Myeloid Dendritic Cells in the Induction of Antigen-Specific Antitumor Immune Responses. J Immunol 2007; 178:1534-1541; PMID:17237402; http://dx.doi.org/10.4049/jimmunol.178.3.1534
- Steinman RM. Some interfaces of dendritic cell biology. APMIS 2003; 111:675-697; PMID:12974772; http://dx.doi.org/10.1034/j.1600-0463.2003.11107802.x
- Rissoan MC, Soumelis V, Kadowaki N, Grouard G, Briere F, de Waal Malefyt R, Liu YJ. Reciprocal control of T helper cell and dendritic cell differentiation. Science 1999; 283:1183-1186; PMID:10024247; http://dx.doi.org/10.1126/science.283.5405.1183
- Vieira PL, de JongEC, Wierenga EA, Kapsenberg ML, Kalinski P. Development of Th1-inducing capacity in myeloid dendritic cells requires environmental instruction. J Immunol 2000; 164:4507-4512; PMID:10779751; http://dx.doi.org/10.4049/jimmunol.164.9.4507
- Ma Y, Shurin G, Peiyuan Z, Shurin MR. Dendritic Cells in the Cancer Microenvironment. J Cancer 2013; 4:36-44; PMID:23386903; http://dx.doi.org/10.7150/jca.5046
- Martín-Gayo E, Sierra-Filardi E, Corbí AL, Toribio ML. Plasmacytoid dendritic cells resident in human thymus drive natural Treg cell development. Blood 2010; 115:5366-5375; http://dx.doi.org/10.1182/blood-2009-10-248260
- Hanabuchi S, Ito T, Park WR, Watanabe N, Shaw JL, Roman E, Arima K, Wang YH, Voo KS, Cao W, Liu YJ. Thymic stromal lymphopoietin-activated plasmacytoid dendritic cells induce the generation of FOXP3+ regulatory T cells in human thymus. J Immunol 2010; 184:2999-3007; PMID:20173030; http://dx.doi.org/10.4049/jimmunol.0804106
- Zhang Q, Fujino M, Iwasaki S, Hirano H, Cai S, Kitajima Y, Xu J, Li XK. Generation and characterization of regulatory dendritic cells derived from murine induced pluripotent stem cells. Sci Rep 2014; 4:3979; PMID:24496181
- Steinman RM, Hawiger D, Nussenzweig MC. Tolerogenic dendritic cells. Annu Rev Immunol 2003; 21:685-711; PMID:12615891; http://dx.doi.org/10.1146/annurev.immunol.21.120601.141040
- Schmidt SV, Nino-Castro AC, Schultze JL. Regulatory dendritic cells:there is more than just immune activation. Front Immunol 2012; 3:274; PMID:22969767
- Lijun Z, Xin Z, Danhua S, Xiaoping L, Jianliu W, Huilan W, Lihui W. Tumor-infiltrating dendritic cells may be used as clinicopathologic prognostic factors in endometrial carcinoma. Int J Gynecol Cancer 2012; 22:836-841; PMID:22617481; http://dx.doi.org/10.1097/IGC.0b013e31825401c6
- Gulubova MV, Ananiev JR, Vlaykova TI, Yovchev Y, Tsoneva V, Manolova IM. Role of dendritic cells in progression and clinical outcome of colon cancer. Int J Colorectal Dis 2012; 27:159-169; PMID:22065108; http://dx.doi.org/10.1007/s00384-011-1334-1
- Iwamoto M, Shinohara H, Miyamoto A, Okuzawa M, Mabuchi H, Nohara T, Gon G, Toyoda M, Tanigawa N. Prognostic value of tumor-infiltrating dendritic cells expressing CD83 in human breast carcinomas. Int J Cancer 2003; 104:92-97.
- Della Bella S, Gennaro M, Vaccari M, Ferraris C, Nicola S, Riva A, Clerici M, Greco M, Villa ML. Altered maturation of peripheral blood dendritic cells in patients with breast cancer. Br J Cancer 2003; 89:1463-1472; PMID:14562018; http://dx.doi.org/10.1038/sj.bjc.6601243
- Pinzon-Charry A, Maxwell T, López JA. Dendritic cell dysfunction in cancer:a mechanism for immunosuppression. Immunol Cell Biol 2005; 83:451-461; PMID:16174093; http://dx.doi.org/10.1111/j.1440-1711.2005.01371.x
- Ormandy LA, Farber A, Cantz T, Petrykowska S, Wedemeyer H, Horning M, Lehner F, Manns MP, Korangy F, Greten TF. Direct ex vivo analysis of dendritic cells in patients with hepatocellular carcinoma. World J Gastroenterol 2006; 12:3275-3282; PMID:16718852
- Sakakura K, Chikamatsu K, Takahashi K, Whiteside TL, Furuya N. Maturation of circulating dendritic cells and imbalance of T cell subsets in patients with squamous cell carcinoma of the head and neck. Cancer Immunol Immunother 2006; 55:151-159; PMID:15889251; http://dx.doi.org/10.1007/s00262-005-0697-y
- Yamamoto T, Yanagimoto H, Satoi S, Toyokawa H, Yamao J, Kim S, Terakawa N, Takahashi K, Kwon AH. Circulating myeloid dendritic cells as prognostic factors in patients with pancreatic cancer who have undergone surgical resection. J Surg Res 2012; 173:299-308; PMID:21195425; http://dx.doi.org/10.1016/j.jss.2010.09.027
- Orsini G, Legitimo A. Quantification of Blood Dendritic Cells in Colorectal Cancer Patients During the Course of Disease. Pathol Oncol Res 2013; 20:267-276; http://dx.doi.org/10.1007/s12253-013-9691-4
- Almand B, Clark JI, Nikitina E, Van Beynen J, English NR, Knight SC, Carbone DP, Gabrilovich DI. Increased production of immature myeloid cells in cancer patients:a mechanism of immunosuppression in cancer. J Immunol 2001; 166:678-89; PMID:11123353; http://dx.doi.org/10.4049/jimmunol.166.1.678
- Mazzolini G, Murillo O, Atorrasagasti C, Dubrot J, Tirapu I, Rizzo M, Arina A, Alfaro C, Azpilicueta A, Berasain C, et al. Immunotherapy and immunoescape in colorectal cancer. World J Gastroenterol 2007; 13:5822-5831; PMID:17990348; http://dx.doi.org/10.3748/wjg.v13.i44.5822
- Lenahan C, Avigan D. Dendritic cell defects in patients with cancer:mechanisms and significance. Breast Cancer Res 2006; 8:101; PMID:16469120; http://dx.doi.org/10.1186/bcr1375
- Ratta M, Fagnoni F, Curti A, Vescovini R, Sansoni P, Oliviero B, Fogli M, Ferri E, Della Cuna GR, Tura S, et al. Dendritic cells are functionally defective in multiple myeloma:the role of interleukin-6. Blood. 2002; 100:230-237; PMID:12070032; http://dx.doi.org/10.1182/blood.V100.1.230
- Boissel N, Rousselot P, Raffoux E, Cayuela JM, Maarek O, Charron D, Degos L, Dombret H, Toubert A, Rea D. Defective blood dendritic cells in chronic myeloid leukemia correlate with high plasmatic VEGF and are not normalized by imatinib mesylate. Leukemia 2004; 18:1656-61; PMID:15343347; http://dx.doi.org/10.1038/sj.leu.2403474
- McCarter MD, Baumgartner J, Escobar GA, Richter D, Lewis K, Robinson W, Wilson C, Palmer BE, Gonzalez R. Immunosuppressive dendritic and regulatory T cells are upregulated in melanoma patients. Ann Surg Oncol 2007; 14:2854-2860; PMID:17593331; http://dx.doi.org/10.1245/s10434-007-9488-3
- Charles J, Di Domizio J, Salameire D, Bendriss-Vermare N, Aspord C, Muhammad R, Lefebvre C, Plumas J, Leccia MT, Chaperot L. Characterization of circulating dendritic cells in melanoma:role of CCR6 in plasmacytoid dendritic cell recruitment to the tumor. J Invest Dermatol 2010; 130:1646-1656; PMID:20220766; http://dx.doi.org/10.1038/jid.2010.24
- Hasskamp J, Zapas J, Elias G. Dendritic cells in patients with melanoma. Ann Surg Oncol 2008; 15:1807; PMID:18214620; http://dx.doi.org/10.1245/s10434-007-9784-y
- Hoffmann TK, Muller-Berghaus J, Ferris RJ, Johnson JT, Storkus WJ, Whiteside TL. Alterations in the frequency of dendritic cell subsets in the peripheral circulation of patients with squamous cell carcinomas of the head and neck. Clin Cancer Res 2002; 8:1787-1793; PMID:12060618
- Troy A, Davidson P, Atkinson C, Hart D. Phenotypic characteristics of dendritic cell infiltrate in prostate cancer. J Urol 1998; 160:214-219; PMID:9628653; http://dx.doi.org/10.1016/S0022-5347(01)63093-3
- Troy AJ, Summers KL, Davidson PJ, Atkinson CH, Hart DN. Minimal recruitment and activation of dendritic cells withing renal cell carcinoma. Clin Cancer Res 1998; 4:585-593; PMID:9533525
- Hargadon KM. Tumor-altered dendritic cell function:implications for anti-tumor immunity. Front Immunol 2013; 4:article 192; http://dx.doi.org/10.3389/fimmu.2013.00192
- Aalamian M, Pirtskhalaishvili G, Nunez A, Esche C, Shurin GV, Huland E, Huland H, Shurin MR. Human prostate cancer regulates generation and maturation of monocyte-derived dendritic cells. Prostate 2001; 46:68-75; PMID:11170134; http://dx.doi.org/10.1002/1097-0045(200101)46:1%3c68::AID-PROS1010%3e3.0.CO;2-2
- Brown RD, Pope B, Murray A, Esdale W, Sze DM, Gibson J, Ho PJ, Hart D, Joshua D. Dendritic cells from patients with myeloma are numerically normal but functionally defective as they fail to up-regulate CD80 (B7-1) expression after huCD40LT stimulation because of inhibition by transforming growth factor-beta1 and interleukin-10. Blood 2001; 98:2992-2998; PMID:11698282; http://dx.doi.org/10.1182/blood.V98.10.2992
- Kichler-Lakomy C, Budinsky AC, Wolfram R, Hellan M, Wiltschke C, Brodowicz T, Viernstein H, Zielinski CC. Deficiences in phenotype expression and function of dentritic cells from patients with early breast cancer. Eur J Med Res 2006; 11:7-12; PMID:16504954
- Satthaporn S, Robins A, Vassanasiri W, El-Sheemy M, Jibril JA, Clark D, Valerio D, Eremin O. Dendritic cells are dysfunctional in patients with operable breast cancer. Cancer Immunol Immunother 2004; 53:510-518; PMID:14740176; http://dx.doi.org/10.1007/s00262-003-0485-5
- Allavena P, Piemonti L, Longoni D, Bernasconi S, Stoppacciaro A, Ruco L, Mantovani A. IL-10 prevents the differentiation of monocytes to dendritic cells but promotes their maturation to macrophages. Eur J Immunol 1998; 28:359-369; PMID:9485215; http://dx.doi.org/10.1002/(SICI)1521-4141(199801)28:01%3c359::AID-IMMU359%3e3.0.CO;2-4
- Beckebaum S, Zhang X, Chen X, Yu Z, Frilling A, Dworacki G, Grosse-Wilde H, Broelsch CE, Gerken G, Cicinnati VR. Increased levels of interleukin-10 in serum from patients with hepatocellular carcinoma correlate with profound numerical deficiencies and immature phenotype of circulating dendritic cell subsets. Clin. Cancer Res 2004; 10:7260-7269; PMID:15534100; http://dx.doi.org/10.1158/1078-0432.CCR-04-0872
- Menetrier-Caux C, Montmain G, Dieu MC, Bain C, Favrot MC, Caux C, Blay JY. Inhibition of the differentiation of dendritic cells from CD34(+) progenitors by tumor cells:role of interleukin-6 and macrophage colony-stimulating factor. Blood 1998; 92:4778-4791; PMID:9845545
- Bharadwaj U, Li M, Zhang R, Chen C, Yao Q. Elevated interleukin-6 and G-CSF in human pancreatic cancer cell conditioned medium suppress dendritic cell differentiation and activation. Cancer Res 2007; 67:5479-88; PMID:17545630; http://dx.doi.org/10.1158/0008-5472.CAN-06-3963
- Gabrilovich DI, Chen HL, Girgis KR, Cunningham HT, Meny GM, Nadaf S, Kavanaugh D, Carbone DP. Production of vascular endothelial growth factor by human tumors inhibits the functional maturation of dendritic cells. Nat Med 1996; 2:1096-103; PMID:8837607; http://dx.doi.org/10.1038/nm1096-1096
- Imai K, Minamiya Y, Koyota S, Ito M, Saito H, Sato Y, Motoyama S, Sugiyama T, Ogawa J. Inhibition of dendritic cell migration by transforming growth factor-β1 increases tumor-draining lymph node metastasis. J Exp Clin Cancer Res 2012; 31:3; PMID:22233831; http://dx.doi.org/10.1186/1756-9966-31-3
- Shurin GV, Shurin MR, Bykovskaia S, Shogan J, Lotze MT, Barksdale EM. Neuroblastoma-derived gangliosides inhibit dendritic cell generation and function. Cancer Res 2001; 61:363-369; PMID:11196188
- Péguet-Navarro J, Sportouch M, Popa I, Berthier O, Schmitt D, Portoukalian J. Gangliosides from human melanoma tumors impair dendritic cell differentiation from monocytes and induce their apoptosis. J Immunol 2003; 170:3488-3494; http://dx.doi.org/10.4049/jimmunol.170.7.3488
- Esche C, Lokshin A, Shurin GV, Gastman BR, Rabinowich H, Watkins SC, Lotze MT, Shurin MR. Tumor's other immune targets:dendritic cells. J Leukoc Biol 1999; 66:336-344; PMID:10449178
- Onishi H, Morisaki T, Baba E, Kuga H, Kuroki H, Matsumoto K, Tanaka M, Katano M. Dysfunctional and short-lived subsets in monocyte-derived dendritic cells from patients with advanced cancer. Clin Immunol 2002; 105:286-295; PMID:12498810; http://dx.doi.org/10.1006/clim.2002.5293
- Bennaceur K, Popa I, Chapman JA, Migdal C, Péguet-Navarro J, Touraine J-L, Portoukalian J. Different mechanisms are involved in apoptosis induced by melanoma gangliosides on human monocyte-derived dendritic cells. Glycobiology 2009; 19:576-582; PMID:19240275; http://dx.doi.org/10.1093/glycob/cwp015
- Probst HC, Lagnel J, Kollias G, van den Broek M. Inducible transgenic mice reveal resting dendritic cells as potent inducers of CD8+ T cell tolerance. Immunity 2003; 18:713-720; PMID:12753747; http://dx.doi.org/10.1016/S1074-7613(03)00120-1
- Hawiger D, Inaba K, Dorsett Y, Guo M, Mahnke K, Rivera M, Ravetch JV, Steinman RM, Nussenzweig MC. Dendritic cells induce peripheral T cell unresponsiveness under steady state conditions in vivo. J Exp Med 2001; 194:769-779; PMID:11560993; http://dx.doi.org/10.1084/jem.194.6.769
- Poschke I, Mao Y, Adamson L, Salazar-Onfray F, Masucci G, Kiessling R. Myeloid-derived suppressor cells impair the quality of dendritic cell vaccines. Cancer Immunol Immunother 2012; 61:827-838; PMID:22080405; http://dx.doi.org/10.1007/s00262-011-1143-y
- Ostrand-Rosenberg S, Sinha P. Myeloid-derived suppressor cells:linking inflammation and cancer. J Immunol 2009; 182:4499-4506; PMID:19342621; http://dx.doi.org/10.4049/jimmunol.0802740
- Gabrilovich DI, Ostrand-Rosenberg S, Bronte V. Coordinated regulation of myeloid cells by tumours. Nat Rev Immunol 2012; 12:253-268; PMID:22437938; http://dx.doi.org/10.1038/nri3175
- Mantovani A. The growing diversity and spectrum of action of myeloid-derived suppressor cells. Eur J Immunol 2010; 40:3317-3320; PMID:21110315; http://dx.doi.org/10.1002/eji.201041170
- Obermajer N, Wong JL, Edwards RP, Odunsi K, Moysich K, Kalinski P. PGE(2)-driven induction and maintenance of cancer-associated myeloid-derived suppressor cells. Immunol Invest 2012; 41:635-657; PMID:23017139; http://dx.doi.org/10.3109/08820139.2012.695417
- Jayaraman P, Parikh F, Lopez-Rivera E, Hailemichael Y, Clark A, Ma G, Cannan D, Ramacher M, Kato M, Overwijk WW, et al. Tumor-expressed inducible nitric oxide synthase controls induction of functional myeloid-derived suppressor cells through modulation of vascular endothelial growth factor release. J Immunol 2012; 188:5365-5376; PMID:22529296; http://dx.doi.org/10.4049/jimmunol.1103553
- Sinha P, Clements VK, Bunt SK, Albelda SM, Ostrand-Rosenberg S. Cross-talk between myeloid- derived suppressor cells and macrophages subverts tumor immunity toward a type 2 response. J Immunol 2007; 179:977-983; PMID:17617589; http://dx.doi.org/10.4049/jimmunol.179.2.977
- Sinha P, Parker KH, Horn L, Ostrand-Rosenberg S. Tumor-Induced Myeloid-derived Suppressor Cell Function is Independent of IFNγ and IL-4Rα. Eur J Immunol 2012; 42:2052-2059; PMID:22673957; http://dx.doi.org/10.1002/eji.201142230
- Scarlett UK, Rutkowski MR, Rauwerdink AM, Fields J, Escovar-Fadul X, Baird J, Cubillos-Ruiz JR, Jacobs AC, Gonzalez JL, Weaver J, et al. Ovarian cancer progression is controlled by phenotypic changes in dendritic cells. J Exp Med 2012; 209:495-506; PMID:22351930; http://dx.doi.org/10.1084/jem.20111413
- Orsini G, Legitimo A, Failli A, Ferrari P, Nicolini A, Spisni R, Miccoli P, Consolini R. Defective generation and maturation of dendritic cells from monocytes in colorectal cancer patients during the course of disease. Int J Mol Sci 2013 Nov 7; 14(11):22022-41; http://dx.doi.org/10.3390/ijms141122022
- Dalerba P, Maccalli C, Casati C, Castelli C, Parmiani G. Immunology and immunotherapy of colorectal cancer. Crit Rev Oncol Hematol 2003; 46:33-57; PMID:12672517; http://dx.doi.org/10.1016/S1040-8428(02)00159-2
- Cui G, Yuan A, Goll R, Olsen T, Husebekk A, Vonen B, Florholmen J. Distinct changes of dendritic cell number and IL-12 mRNA level in adjacent mucosa throughout the colorectal adenoma-carcinoma sequence. Cancer Immunol Immunother 2007; 56:1993-2001; PMID:17579859; http://dx.doi.org/10.1007/s00262-007-0345-9
- Yuan A, Steigen SE, Goll R, Vonen B, Husbekk A, Cui G, Florholmen J. Dendritic cell infiltration pattern along the colorectal adenoma-carcinoma sequence. APMIS 2008; 116:445-456; PMID:18754318; http://dx.doi.org/10.1111/j.1600-0463.2008.00879.x
- Nagorsen D, Voigt S, Berg E, Stein H, Thiel E, Loddenkemper C. Tumor-infiltrating macrophages and dendritic cells in human colorectal cancer:relation to local regulatory T cells, systemic T-cell response against tumor-associated antigens and survival. J Transl Med 2007; 5:62; PMID:18047662; http://dx.doi.org/10.1186/1479-5876-5-62
- Ambe K, Mori M, Enjoji M. S-100 protein-positive dendritic cells in colorectal adenocarcinomas. Distribution and relation to the clinical prognosis. Cancer 1989; 63:496-503; PMID:2912528; http://dx.doi.org/10.1002/1097-0142(19890201)63:3%3c496::AID-CNCR2820630318%3e3.0.CO;2-K
- Nakayama Y, Inoue Y, Minagawa N, Katsuki T, Nagashima N, Onitsuka K, Tsurudome Y, Sako T, Hirata K, Nagata N, et al. Relationships between S-100 protein-positive cells and clinicopathological factors in patients with colorectal cancer. Anticancer Res 2003; 23:4423-4426; PMID:14666729
- Xie Z-J, Jia L-M, He Y-C, Gao J-T. Morphological observation of tumor infiltrating immunocytes in human rectal cancer. World J Gastroenterol 2006; 11:1757-1760.
- Dadabayev AR, Sandel MH, Menon AG, Morreau H, Melief CJ, Offringa R, van der Burg SH, Janssen-van Rhijn C, Ensink NG, Tollenaar RA, et al. Dendritic cells in colorectal cancer correlate with other tumor-infiltrating immune cells. Cancer Immunol Immunother 2004; 11:978-986; http://dx.doi.org/10.1007/s00262-004-0548-2
- Schwaab T, Weiss JE, Schned AR, Barth RJ Jr. Dendritic cell infiltration in colon cancer. J Immunother 2001; 24:130-137; http://dx.doi.org/10.1097/00002371-200103000-00007
- Sandel MH, Dadabayev AR, Menon AG, Morreau H, Melief CJ, Offringa R, van der Burg SH, Janssen-van Rhijn CM, Ensink NG, Tollenaar RA, et al. Prognostic value of tumor-infiltrating dendritic cells in colorectal cancer:role of maturation status and intratumoral localization. Clin Cancer Res 2005; 7:2576-2582; http://dx.doi.org/10.1158/1078-0432.CCR-04-1448
- Naito Y, Saito K, Shiiba K, Ohuchi A, Saigenji K, Nagura H, Ohtani H. CD8+ T cells infiltrated within cancer cell nests as a prognostic factor in human colorectal cancer. Cancer Res 1998; 58:3491-3494; PMID:9721846
- Coca S, Perez-Piqueras J, Martinez D, Colmenarejo A, Saez MA, Vallejo C, Martos JA, Moreno M. The prognostic significance of intratumoral natural killer cells in patients with colorectal carcinoma. Cancer 1997; 79:2320-2328; PMID:9191519; http://dx.doi.org/10.1002/(SICI)1097-0142(19970615)79:12%3c2320::AID-CNCR5%3e3.0.CO;2-P
- Conti J, Thomas G. The Role of Tumour Stroma in Colorectal Cancer Invasion and Metastasis. Cancers 2011; 3:2160-2168.
- Banner BF, Sonmez-Alpan E, Yousem SA. An immunophenotypic study of the inflammatory cell populations in colon adenomas and carcinomas. Mod Pathol 1993; 6:295-301; PMID:8346177
- Gabrilovich D. Mechanisms and functional significance of tumour-induced dendritic-cell defects. Nat Rev Immunol 2004; 4:941-52; PMID:15573129; http://dx.doi.org/10.1038/nri1498
- Michielsen AJ, Hogan AE, Marry J, Tosetto M, Cox F, Hyland JM, Sheahan KD, O’Donoghue DP, Mulcahy HE, Ryan EJ, et al. Tumour tissue microenvironment can inhibit dendritic cell maturation in colorectal cancer. PLoS One 2011; 6:e27944; PMID:22125641; http://dx.doi.org/10.1371/journal.pone.0027944
- Michielsen AJ, Noonan S, Martin P, Tosetto M, Marry J, Biniecka M, Maguire AA, Hyland JM, Sheahan KD, O’Donoghue DP, et al. Inhibition of dendritic cell maturation by the tumor microenvironment correlates with the survival of colorectal cancer patients following bevacizumab treatment. Mol Cancer Ther 2012; 11:1829-1837; PMID:22675042; http://dx.doi.org/10.1158/1535-7163.MCT-12-0162
- Wendum D, Masliah J, Trugnan G, Flejou JF. Cyclooxygenase-2 and its role in colorectal cancer development. Virchows Arch 2004; 445:327-333; PMID:15340847; http://dx.doi.org/10.1007/s00428-004-1105-2
- DuBois RN, Smalley WE. Cyclooxygenase, NSAIDs, and colorectal cancer. J Gastroenterol 1996; 31:898-906; PMID:9027660; http://dx.doi.org/10.1007/BF02358623
- Suzuki A, Masuda A, Nagata H, Kameoka S, Kikawada Y, Yamakawa M, Kasajima T. Mature dendritic cells make clusters with T cells in the invasive margin of colorectal carcinoma. J Pathol 2002; 196:37-43; PMID:11748640; http://dx.doi.org/10.1002/path.1018
- Sharma S, Stolina M, Yang SC, Baratelli F, Lin JF, Atianzar K, Luo J, Zhu L, Lin Y, Huang M, Dohadwala M, et al. Tumor cyclooxygenase 2-dependent suppression of dendritic cell function. Clin Cancer Res 2003; 9:961-968; PMID:12631593
- Ishida A, Ohta M, Toda M, Murata T, Usui T, Akita K, Inoue M, Nakada H. Mucin-induced apoptosis of monocyte-derived dendritic cells during maturation. Proteomics 2008; 8:3342-3349; PMID:18690650; http://dx.doi.org/10.1002/pmic.200800039
- Süren D, Yıldırım M, Demirpençe Ö, Kaya V, Alikanoğlu AS, Bülbüller N, Yıldız M, Sezer C. The role of High Mobility Group Box 1 (HMGB1) in colorectal cancer. Med Sci Monit 2014; 20:530-537; PMID:24681824; http://dx.doi.org/10.12659/MSM.890531
- Völp K, Brezniceanu ML, Bösser S, Brabletz T, Kirchner T, Göttel D, Joos S, Zörnig M. Increased expression of high mobility group box 1 (HMGB1) is associated with an elevated level of the anti-apoptotic c-IAP2 protein in human colon carcinomas. Gut 2006; 55:234-242; PMID:16118352; http://dx.doi.org/10.1136/gut.2004.062729
- Kusume A, Sasahira T, Luo Y, Isobe M, Nakagawa N, Tatsumoto N, Fujii K, Ohmori H, Kuniyasu H. Suppression of dendritic cells by HMGB1 is associated with lymph node metastasis of human colon cancer. Pathobiology 2009; 76:155-62; PMID:19571604; http://dx.doi.org/10.1159/000218331
- Huang A, Gilmour JW, Imami N, Amjadi P, Henderson DC, Allen-Mersh TG. Increased serum transforming growth factor-beta1 in human colorectal cancer correlates with reduced circulating dendritic cells and increased colonic Langerhans cell infiltration. Clin Exp Immunol 2003; 134:270-278; PMID:14616787; http://dx.doi.org/10.1046/j.1365-2249.2003.02295.x
- Della Porta M, Danova M, Rigolin GM, Brugnatelli S, Rovati B, Tronconi C, Fraulini C, Russo Rossi A, Riccardi A, Castoldi G. Dendritic cells and Vascular Endothelial Growth Factor in colorectal cancer:correlations with clinicobiological findings. Oncology 2005; 68:276-284; PMID:16015045; http://dx.doi.org/10.1159/000086784
- Vlad G, Chang CC, Colovai AI, Berloco P, Cortesini R, Suciu-Foca N. Immunoglobulin-like transcript 3:A crucial regulator of dendritic cell function. Hum Immunol 2009; 70:340-344; PMID:19275918; http://dx.doi.org/10.1016/j.humimm.2009.03.004
- Roy S, Goswami S, Bose A, Goswami KK, Sarkar K, Chakraborty K, Chakraborty T, Pal S, Haldar A, Basu P, et al. Defective dendritic cell generation from monocytes is a potential reason for poor therapeutic efficacy of interferon α2b (IFNα2b) in cervical cancer. Transl Res 2011; 158:200-213; PMID:21925117; http://dx.doi.org/10.1016/j.trsl.2011.03.003
- Chang LL, Wang SW, Wu IC, Yu FJ, Su YC, Chen YP, Wu DC, Kuo CH, Hung CH. Impaired dendritic cell maturation and IL-10 production following H. pylori stimulation in gastric cancer patients. Appl Microbiol Biotechnol 2012; 96:211-220; PMID:22526791; http://dx.doi.org/10.1007/s00253-012-4034-z
- Ramos RN, Chin LS, Dos Santos AP, Bergami-Santos PC, Laginha F, Barbuto JA. Monocyte-derived dendritic cells from breast cancer patients are biased to induce CD4+CD25+Foxp3+ regulatory T cells. J Leukoc Biol 2012; 92:673-682; PMID:22636320; http://dx.doi.org/10.1189/jlb.0112048
- Kvistborg P, Bechmann CM, Pedersen AW, Toh HC, Claesson MH, Zocca MB. Comparison of monocyte-derived dendritic cells from colorectal cancer patients, non-small-cell-lung-cancer patients and healthy donors. Vaccine 2010; 28:542-547; http://dx.doi.org/10.1016/j.vaccine.2009.09.129
- Hasebe H, Nagayama H, Sato K, Enomoto M, Takeda Y, Takahashi TA, Hasumi K, Eriguchi M. Dysfunctional regulation of the development of monocyte-derived dendritic cells in cancer patients. Biomed Pharmacother 2000; 54:291-298; PMID:10989961; http://dx.doi.org/10.1016/S0753-3322(00)80050-5
- Harris TJ, Drake CG. Primer on tumor immunology and cancer immunotherapy. J Immunother Cancer 2013; 1:12; PMID:24829749; http://dx.doi.org/10.1186/2051-1426-1-12
- Evans MD, Escofet X, Karandikar SS, Stamatakis JD. Outcomes of resection and non-resection strategies in management of patients with advanced colorectal cancer. World J Surg Oncol 2009; 7:28; PMID:19284542; http://dx.doi.org/10.1186/1477-7819-7-28
- McDermott DF, Atkins MB. PD-1 as a potential target in cancer therapy. Cancer Med 2013; 2:662-673; PMID:24403232
- Janikashnili N, Bonnotte B, Katsanis E, Lamornier N. The dendritic cell-regulatory T lymphocyte crosstalk contributes to tumor-induced tolerance. Clin Dev Immunol 2011; 2011:430394; PMID:22110524
- Fang H, Ang B, Xu X, Huang X, Wu Y, Sun Y, Wang W, Li N, Cao X, Wan T. TLR4 is essential for dendritic cell activation and anti-tumor T-cell response enhancement by DAMPs released from chemically stressed cancer cells. Cell Mol Immunol 2014; 11:150-159; PMID:24362470; http://dx.doi.org/10.1038/cmi.2013.59
- Gao D, Li C, Xie X, Zhao P, Wei X, Sun W, Liu HC, Alexandrou AT, Jones J, Zhao R, et al. Autologous tumor lysate-pulsed dendritic cell immunotherapy with cytokine-induced killer cells improves survival in gastric and colorectal cancer patients. PLoS One. 2014; 9:e93886; PMID:24699863; http://dx.doi.org/10.1371/journal.pone.0093886