Abstract
Expression of recombinant vaccine antigens and monoclonal antibodies using plant viral vectors has developed extensively during the past several years. The approach benefits from high yields of recombinant protein obtained within days after transient delivery of viral vectors to leaves of Nicotiana benthamiana, a tobacco relative. Modified viral genomes of both RNA and DNA viruses have been created. Geminiviruses such as bean yellow dwarf virus (BeYDV) have a small, single stranded DNA genome that replicates in the nucleus of an infected plant cell, using the cellular DNA synthesis apparatus and a virus-encoded replication initiator protein (Rep). BeYDV-derived expression vectors contain deletions of the viral genes encoding coat and movement proteins and insertion of an expression cassette for a protein of interest. Delivery of the geminiviral vector to leaf cells via Agrobacterium-mediated delivery produces very high levels of recombinant DNA that can act as a transcription template, yielding high levels of mRNA for the protein of interest. Several vaccine antigens, including Norwalk virus capsid protein and hepatitis B core antigen, were expressed using the BeYDV vector at levels up to 1mg per g of leaf mass. BeYDV replicons can be stacked in the same vector molecule by linking them in tandem, which enables production of multi-subunit proteins like monoclonal antibody (mAb) heavy and light chains. The protective mAb 6D8 against Ebola virus was produced at 0.5mg per g of leaf mass. Multi-replicon vectors could be conveniently used to produce protein complexes, e.g. virus-like particles that require two or more subunits.
Plant Viral Vectors Facilitate Robust Expression
Research involving the use of plants for recombinant protein production has surged in the past 10 years, which is readily demonstrated by the articles and reference lists included in this special issue of Human Vaccines. A major force driving plant-based expression is the development of viral vectors that enable robust and rapid transient expression in plant leaves. Most of the early plant-derived vaccine work used stably transgenic lines, including the first journal publication demonstrating expression of hepatitis B surface antigen in tobacco.Citation1 Integration of transgenes into plant nuclear chromosomal DNA allows stable protein expression and the potential for extreme scale-up by sexual propagation, but yields relatively poor expression, largely due to post-transcriptional silencing via RNA interference (RNAi).Citation2 The development of recombinant plant viral genomesCitation3–Citation5 allowed delivery of replicons into leaves, resulting in robust amplification and generation of target mRNA, without the need for stable integration into the genome. Although some transient expression vectors may still encounter RNAi, the rapid production of mRNA can often quickly overwhelm the plant defenses and generate prodigious amounts of protein before silencing can take effect.
Several types of RNA viruses have been used to create plant expression vectors, including tobacco mosaic virus (TMV), potato virus X (PVX), cowpea mosaic virus (CPMV) and alfalfa mosaic virus (AIMV).Citation3–Citation5 One of the most successful is the magnI-CON system, which uses Agrobacterium tumefaciens (agro-inoculation) to deliver DNA encoding recombinant TMV cDNA into leaves, which upon transcription and transport of RNA to the cytoplasm generates replicating viral genomes that produce very high levels of target gene mRNA.Citation6 However, RNA virus replicons become somewhat cumbersome when more than one gene must be expressed, e.g., heavy and light chains of monoclonal antibodies (mAb). Gleba and coworkers have developed a system that uses co-delivery of two different replicons (TMV and PVX) for production of mAb's, but in the “deconstructed” format it requires co-delivery of five different Agrobacterium lines.Citation7 For the production of virus-like particles (VLP) that require co-expression of three or more genes (e.g., influenza VLP),Citation8 the RNA replicon system becomes unworkable.
Geminivirus Structure and Replication
Several groups have developed recombinant geminivirus genomes to create DNA amplification systems (), which have a large advantage in their ability to replicate multiple different genes simultaneously and independently in the same cells.Citation9 Geminiviruses of the genus Mastrevirus contain a single-stranded circular DNA genome varying in size from 2.5 kilo-bases (kb) to 3.0 kb.Citation10 The genomic DNA is replicated in the nucleus of the host cell by a rolling circle replication mechanism, utilizing double-stranded DNA intermediates. Mastreviruses have monopartite genomes and comprise viruses that infect both monocot plants (wheat dwarf virus, maize steak virus and digitalia steak virus) and dicot plants (tobacco yellow dwarf virus and bean yellow dwarf virus, BeYDV).
The BeYDV genomeCitation11 () contains a long intergenic region (LIR) and short intergenic region (SIR) with four pro-tein-encoding genes lying between them. The movement protein (MP) and the capsid protein (CP) genes occur on the viral-sense (V) strand, while the replication initiator protein (Rep) and RepA protein genes lie on the complementary-sense (C) strand. Alternate splicing of the C transcript yields mRNA's for Rep and RepA. Rep mRNA is generated when a short intron () is spliced, while unspliced mRNA allows translation of the C1 open reading frame to produce RepA. LIR and SIR are the only cis-elements required for replication of the genome. The LIR contains a bi-directional promoter and a stem-loop structure that is essential for initiation of rolling-circle replication. The SIR is the origin of C-strand synthesis and contains transcription termination and polyadenylation signals. Although Rep is required for replication, it can be supplied in trans and thus need not be present in the viral replicon.Citation12
Geminiviruses replicate DNA via rolling-circle mechanism, where Rep mediates nicking and ligating functions.Citation11 Initially, viral single-stranded DNA enters cell nuclei and is converted into the double-stranded DNA replicative form. The replicative form serves as the template for viral transcription as well as the template for further replication.Citation13 Rep nicks the positive sense (V) strand at a specific sequence within the LIR and then covalently binds to 5′ terminus. The 3′-OH terminus is used as the primer for the synthesis of nascent plus strand. DNA synthesis is accomplished by host replication proteins, including DNA polymerases. Completion of the nascent plus strand regenerates the origin of replication, which is nicked by Rep protein again. Rep protein then acts as a terminase to release the displaced plus strand and simultaneously ligates it to create the circular form. In the process, Rep is transferred to the newly created 5′ terminus. Late in the replication cycle single stranded viral genomes are generated for encapsidation. However, in recombinant replicons, the CP gene is deleted and encapsidation does not occur.Citation12
Geminivirus Replicons Enhance Protein Expression
Geminiviral replicon systems are used to increase the expression of foreign proteins in plants. presents published studies that demonstrate expression of various proteins using geminiviral vectors. One of the earliest papers to describe a recombinant geminiviral repliconCitation14 used African cassava mosaic virus (ACMV), which has a bipartite genome. These workers deleted the capsid protein gene in DNA1, and inserted the chloramphenicol acetyl transferase (CAT) gene, showing expression at 80 U per mg soluble leaf protein. Although their intent was to examine the effect of CP on the infection cycle, and not recombinant protein production per se, Stanley's group unequivocally demonstrated replication and expression of the CAT gene and thus, the potential of geminivirus replicons for protein production. Similarly, wheat dwarf virusCitation15 and maize streak virusCitation10 replicons were shown to replicate in plant cells and express selectable markers, although the specific accumulation levels were not reported.
Mor et al.Citation12 using the bean yellow dwarf virus genome provided by John Stanley,Citation11 developed a replicon specifically designed for recombinant protein expression. They deleted the V1 (MP) and V2 (CP) gene (), inserted an expression cassette driven by the cauliflower mosaic virus (CaMV) 35S promoter, a strong constitutive plant promoter. Expression of reporter gene GUS was enhance up to 40-fold in tobacco cells that co-expressed Rep/RepA. These studies also showed that Rep/RepA could be supplied in trans from a separate expression vector, and need not be present in the replicon. Moreover, expression of Rep without RepA generated very little amplification of the replicon, which suggests that RepA has a role in maintaining the nucleus in S phase to facilitate DNA replication. This idea is consistent with observations that WDV RepA binds to maize retinoblastoma-like protein, which controls cell cycle progression.Citation16
A stable transgenic system was created in tobacco NT1 cells and whole potato plants, in which Rep or Rep/RepA expression was controlled by an alcohol inducible promoter.Citation17 A BeYDV replicon similar to that of Mor et al.Citation12 which contained an expression cassette for Norwalk virus capsid protein (NVCP) but lacked the Rep gene, was delivered via a separate transgene chromosomal integration. Amplification of the replicon occurred only upon induction of the Rep/RepA gene cassette, and resulted in up to ten-fold increase in expression of NVCP (up to 1.2% of total soluble protein, TSP), depending on the individual cell line.Citation17
Improved BeYDV Transient Expression Vectors
The tobacco relative Nicotiana benthamiana has proved to be a very useful host for viral pathogenesis as well as recombinant protein expression using viral vectors, due to its ability to support replication of many different viruses.Citation18 Huang et al. used BeYDV replicons to express hepatitis B core antigen (HBcAg) and NVCP in leaves of N. benthamiana. Delivery of replicons by Agrobacterium infiltration of leaves along with pRep110 for expression of Rep/RepA resulted in very high levels of DNA amplification, and expression of HBcAg at 0.8 mg per g leaf fresh weight (mg/gFW), and of NVCP at 0.34 mg/gFW. Maximal expression was obtained after only four days of incubation after agro-inoculation, and was maintained at similar levels to seven days. Both of these antigens assembled VLP, as demonstrated by sucrose gradient sedimentation and electron microscopy. Expression was enhanced substantially by co-delivery of an expression cassette for the p19 protein of tomato bushy stunt virus (TBSV), a potent suppressor of post-transcriptional gene silencing.
Although delivery of the Rep/RepA expression cassette on a separate vector has the potential to control replication by varying the strength of the promoter used, it is more convenient to use a single replicon that includes the Rep/RepA gene in its native configuration as shown in . Thus, Huang et al.Citation9,Citation19 constructed replicons that contained the C1/C2 genes, as shown in . Note that the LIR is duplicated, and flanks the replicon on both sides. The relatively weak C-sense LIR promoter drives transcription of C1/C2, and alternate splicing of the intron produces Rep mRNA, while transcripts that escape splicing generate RepA mRNA. Expression of Rep/RepA proteins then mediates generation of the replicon and its replication (). Interestingly, when C1/C2 genes were present in the replicon, co-delivery of a p19 expression cassette had no discernable effect on expression of HBcAg.
Rybicki and coworkersCitation20 have also utilized replicons that contain the C1/C2 genes from a “mild” strain of BeYDV (BeYDV-m). The replicon is very similar to that described above,Citation19 and produced substantial enhancement of expression over that obtained with a nonreplicating vector for three different genes. The L1 capsid protein of human papillomavirus (HPV-16) accumulated in leaves of N. benthamiana at up to 0.55 mg/gFW, which is in the same range as that obtained for HBcAg and NVCP.Citation19 However, the HPV-16 L1 results were obtained with the co-infiltration of the Nss gene as a suppressor of post-transcriptional silencing.Citation20 The authors did not report a comparison of the BeYDV-m replicon used without the silencing suppressor, so it is not possible to determine its effect with confidence.
Optimized BeYDV Replicon Vectors
Convenient BeYDV-derived single replicon vectors are available for use by the research community. The authors have constructed the plasmids pBYR1 and pBYR2 (), which incorporate the cis-acting LIR and SIR elements, C1/C2 gene for Rep/RepA expression, and one of two expression cassettes that include the CaMV 35S promoter with duplicated enhancer. pBYR1 contains the tobacco etch virus (TEV) 5′ untranslated region (UTR) and the soybean vspB 3′ region with transcription termination and polyadenylation signals. pBYR2 contains the TMV 5′ UTR and the tobacco extensin 3′ region. Both plasmids contain unique restriction sites that lie between the 5′ and 3′ regions in order to facilitate convenient insertion of genes for expression. Another modification replaces the NPTII expression cassette flanking the replicon proximal to the left border sequence (which facilitates selection of stable transgenic plants, but is unnecessary for transient expression) by a cassette for expression of p19 (). Thus, pBYR1p19 and pBYR2p19 enable suppression of post-transcriptional silencing, which in some cases may further enhance expression of the target gene, without the need to co-deliver a second vector. A prudent plan would be to examine expression of a target gene either with or without the p19 cassette, in order to select the optimal system for that particular protein.
Extraction and Purification of VLP Produced with BeYDV Replicon
The success of geminivirus and other virus-based expression systems has made plants one of the most desirable hosts to produce VLP.Citation4,Citation19,Citation21–Citation24 However, challenges exist that must be overcome for plant-derived VLP to become effective clinical materials for preventing, treating and detecting diseases. One such challenge is the need to develop proper methods for delivering plant-produced VLP to patients. While oral ingestion of VLP containing edible plant parts is still one of the options for vaccine delivery, especially for animal vaccines, the regulatory requirements for human vaccines require a defined unit dosage and necessitate extraction and purification of VLP from plants.Citation21,Citation25 Therefore, the successful application and commercial exploitation of plant-derived VLP depends on the availability of selective and scalable methodologies for VLP recovery and purification.
The strategies for extraction and purification of plant-derived VLP are similar to those of other protein biologics, with the common goal of recovering the maximal amount of highly purified target protein with a minimal number of processing steps. However, the unique properties of VLP and host plant tissue may present specific challenges for each of the processing steps. For example, plant extracts are rich in solid debris and contain higher amounts of phenolics and alkaloids than other organisms.Citation26–Citation28 As a result, direct loading of plant extracts onto chromatographic columns often causes resin fouling and poor binding of target protein. Therefore, one or more processing steps using non-chromatographic separation methods is recommended to eliminate these plant specific molecules prior to column chromatography.Citation27 While the relative large size of VLP provides opportunities for separating them from other molecules by centrifugation or filtration methods, the necessity to preserve particle integrity and architecture, and to achieve size uniformity, also presents challenges for each of the processing steps.
The most common purification methods for VLP are based on gradient and/or ultracentrifugation regardless of the origin of the expression systems.Citation19,Citation24,Citation29,Citation30 In these methods, VLP are separated from other molecules based on their unique size and density. The authors have successfully used these methods in isolating and characterizing several plant-derived VLP.Citation19,Citation24 Centrifugation methods, however, have inherent limitations in scalability and time efficiency, and thereby are not optimal for large-scale VLP production.Citation21,Citation31 To improve the scalability and robustness of VLP purification procedures, the authors have developed a scalable purification process for Norwalk VLP based on low pH precipitation and conventional chromatographic steps.Citation21,Citation25 In this procedure, cell debris and ribulose 1,5-bisphosphate carboxylaseoxygenase (RuBisCo), the most abundant plant host protein in leaves, are removed from the extract by precipitation and centrifugation under low pH buffer conditions (pH 5.3). Since column chromatography has been successfully used in large scale cGMP manufacturing of highly purified protein pharmaceuticals,Citation25,Citation32,Citation33 the authors showed that it is effective in producing highly purified (>98%) and fully assembled VLP.Citation21 Compared with the laborious and time-consuming methods based on sucrose or CsCl gradient centrifugation, the chromatography-based method is robust, more scalable, and can shorten the operation time from several days to less than 12 hr (Q. Chen, Manuscript in preparation). This purification scheme has been used to generate Standard Operating Procedures (SOPs) which have been used in a cGMP production of NVCP VLP from N. benthamiana plants for human clinical trials.Citation21
West Nile Virus Chimeric VLP Produced with BeYDV Replicon
The authors' research on development of a vaccine candidate for West Nile virus (WNV) provided another example that demonstrates the utility of geminivirus-based vectors for production of VLP and the effectiveness of chromatographic methods for purifying VLP from plants. WNV is a member of the genus Flavivirus in the Flaviviridae family.Citation34 It is an enveloped virus with a single positive stranded RNA genome and causes infection in the central nervous system of vertebrate animals. Infection in humans can cause meningitis, encephalitis, other severe neurological diseases and death especially in the elderly and immunocompromised population. Since 1999, WNV has escaped from the eastern Hemisphere and spread to the western Hemisphere through New York City.Citation35,Citation36 Surveillance studies by the Centers for Disease Control and Prevention have documented that WNV has become the leading cause of human arboviral infection in the US, with greater than 29,000 cases and 1,200 deaths during the last decade, and many more have been infected and remain undiagnosed.Citation37
Currently, there is no vaccine for WNV licensed for human use. Humoral immunity is considered an essential aspect of protective immunity for WNV.Citation38 Several protective mAb have been identified, most of which are targeted to epitopes on the envelope (E) protein of WNV. For example, Chen and coworkers recently demonstrated the efficacy of a plant-derived mAb (huE16) against the Domain III (DIII) of E protein in protecting mice from lethal WNV infections.Citation39 To develop a vaccine for WNV infection, the authors fused the coding sequence of DIII of WNV E protein to the 3′ end of the hepatitis B core antigen (HBc) gene, aiming to create an HBc-DIII chimeric VLP that displays the DIII epitopes on the surface of the HBc VLP particle. The results indicate that the HBc-DIII fusion protein was produced N. benthamiana plants using a BeYDV-based expression vector like that of . High-level accumulation of this fusion protein was observed within six days post infiltration (). The plant-derived fusion protein was extracted and purified by a low pH precipitation-column chromatography scheme similar to that used for NVCP VLP.Citation21 This protocol was effective not only in separating the fusion protein from other cellular components to yield highly purified target protein, but also in maintaining the structural integrity of the fusion particle to produce assembled VLP with consistent size (). Western blotting showed that a protein of the expected molecular size (∼27 kDa) was detected with either anti-HBc () or anti-DIII antibodies (). These results further demonstrate the robustness of the BeYDV replicon system and the availability of scalable purification schemes, which facilitate the broad application of plants as hosts for the development and production of other VLP pharmaceuticals.
Robust VLP Purification Methods and Considerations for cGMP Compliance
The relative large size of VLP provides opportunities for the development of novel chromatographic matrices with better VLP binding capacity or specificity. In conventional chromatography, the binding between the target molecule and the ligand occurs inside the diffusional pores of resin beads. When membrane is used as a matrix, however, the interaction of the binding sites and the target molecules occurs in convective flow-through pores.Citation40 As a result, membrane chromatography is particularly useful for purification of large molecules with low diffusivities, and therefore, is increasingly explored to replace conventional chromatographic resins for VLP purification.Citation41 Other membrane separation methods developed for non-biological nanoparticles also have potential applications for VLP purification. For example, diafiltration, a membrane-based method, was shown to be an effective way to purify and separate nanoparticles based on their sizes.Citation42 This technique requires simple equipment and has facile scalability, and is particularly useful in eliminating both aggregates and partially assembled nanoparticles from the feed stream. It provides an affordable, convenient means to consolidate purification and size separation of VLP into a single step and to achieve high degree of purity and size uniformity. New technologies such as microarrays have also been used in developing methods for the purification of specific VLP. For example, the authors have developed a system to screen peptide ligands with specific affinity to protein targets in a library of 10,000 randomly generated 20mer peptides immobilized on a microarray. The peptides are immobilized on the microarray in an orientation that mimics their spatial configuration as they are conjugated to an affinity matrix. This ensures that peptides identified by the screening will bind the target protein with equivalent specificity and affinity when coupled to chromatographic resins. This method identified several peptides that exhibit high affinity to NVCP. Column chromatography with these affinity ligands has yielded highly pure NVCP VLP from N. benthamiana plant extract (Chen Q, unpublished results). The rapid ligand discovery process and the economical nature of peptide synthesis will likely allow the use of this technology to identify affinity peptides for the purification of other VLP as well.
The clinical success of plant-produced VLP vaccines will depend on factors other than the availability of robust expression systems and large-scale downstream processing capabilities. It will also require a Quality Management System to ensure compliance with FDA's cGMP regulations. As for other protein biologics, a well-developed manufacturing process for VLP must handle large volumes of preparations, yield highly purified product, preserve the structural integrity and stability of the VLP, reduce the overall cost of production, and ensure the compliance of cGMP regulations. One of the critical considerations for plant-made VLP downstream processing is to take early measures to prevent undesirable contaminants such as bioburden and certain plant host molecules from entering purification feed streams. These measures are essential in ensuring the regulatory compliance of the final product, as well as in simplifying the subsequent purification process. Due to the diversity of VLP architecture, size and epitopes they are designed to display, it is impossible to develop one universal purification scheme that can be applied to all plant-derived VLP. However, effective general purification strategies are being established for adaptation of purifying individual VLP with specific physiochemical properties from a production host plant with unique contaminating molecules. New progress in the development of novel separation materials and technologies will continue to improve in the robustness, cost and cGMP compliance of VLP downstream processes.
Dual Replicon BeYDV Vectors for Co-Expression of Two Separate Genes
Co-expression of two or more genes in separate replicons is readily achieved with BeYDV replicons, since they replicate independently and non-competitively in the same cell.Citation9 Huang et al. showed robust expression of the heavy and light chains of the anti-Ebola virus mAb 6D8,Citation9 using either co-delivery of separate vectors or delivery of a single vector that contained tandemly arranged replicons (). The authors hypothesized that a single LIR placed between the two replicons would serve to facilitate release and amplification of both replicons. This was proved by the accumulation of replicating forms of separate DNA episomes in leaves after agro-inoculation with a single dual replicon vector. The 6D8 mAb was expressed at ∼0.5 mg per g leaf mass, which is the same as that achieved with a different mAb expressed via the magnICON system.Citation7 Although the studies reported by Huang et al.Citation9 utilized co-delivery of a p19 expression vector to suppress silencing, subsequent studies showed that the single vector used without p19 achieved the same results (Chen Q, unpublished results), which indicates that post-transcriptional silencing was not a factor.
Conclusions
Geminivirus-based DNA replicon vectors have been used by plant researchers for at least the past 22 years. Concerted efforts to develop geminiviral replicons for protein production in plants began about 10 years ago, and resulted in systems that are robust and highly competitive with other protein expression systems. VLP expressed with the BeYDV replicon in N. benthamiana leaf and purified by judiciously considered methods are prime candidates for future human vaccines, and could enter clinical trials within the next few years. Moreover, geminivirus-based replicons can be linked in tandem arrays in order to produce two or more proteins at high levels, as shown by the expression of IgG heavy and light chains. Many opportunities for complex protein production using geminiviral vectors exist, including VLP that contain two or more proteins (e.g., influenza hemagglutinin, neuraminidase and matrix M1 proteins), IgM and IgA antibodies, multi-subunit interleukins and modified toxins for use as vaccine adjuvants.
Figures and Tables
Figure 1 Bean yellow dwarf virus genome structure. The genome is circular single-stranded DNA of 2,561 nt in length. The long intergenic region (LIR) contains a bidirectional promoter that drives transcription of the virion sense genes (V1, movement protein; V2, coat protein) and the complimentary sense genes (C1, RepA; C1/C2 with spliced intron, Rep protein). The LIR also contains a stem-loop structure that is the site of nicking by Rep protein. The short intergenic region contains polyadenylation signals for the protein-coding genes.
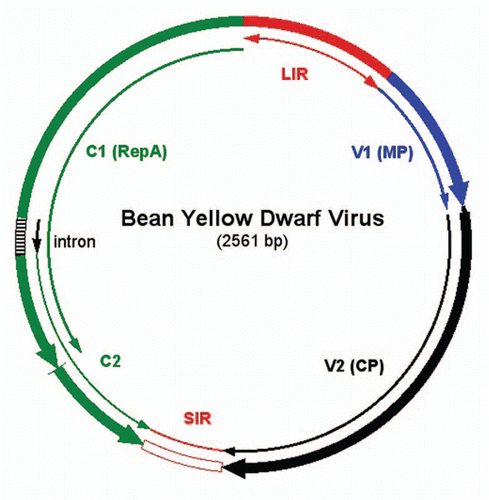
Figure 2 Structure of BeYDV replicon encoding GFP. The T-DNA construct (top) is flanked by left border (LB) and right border (RB) sequences that delineate the DNA to be transferred by Agrobacterium to the plant cells. The replicon is flanked by two LIR elements (red blocks), and the GFP expression cassette is inserted in place of the V1 and V2 genes (), between the one LIR and the SIR (yellow oval). The Rep/RepA (C1/C2) gene is located between the SIR and the other LIR, in its normal position in the BeYDV genome (). Once delivered into plant cells, T-DNA is targeted to the nucleus, where transcription of C1/C2 produces Rep mRNA, which is transported to the cytoplasm and translated to make Rep protein. The Rep protein is targeted back to the nucleus, where it nicks the LIR stem-loops in the T-DNA to release a single-stranded DNA segment. The DNA is then copied to make double-stranded DNA, and rolling circle replication occurs to produce very high copy number of replicons. In double stranded replicative form, the DNA is a template for mRNA transcription and yields GFP mRNA in abundance. 35S/TEV, CaMV 35S promoter linked to the tobacco etch virus 5′UTR; GFP, coding sequence for green fluorescent protein; VSP 3′, soybean vspB 3′ flanking region with transcription termination signals.
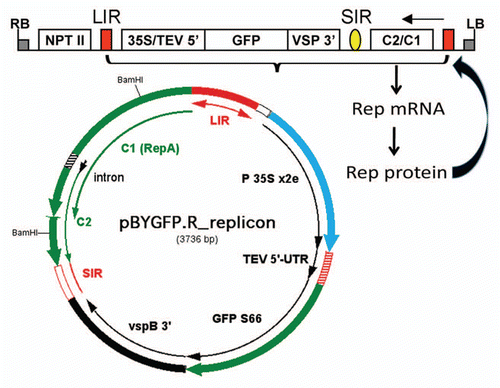
Figure 3 BeYDV replicon vectors available for use. pBYR1 and pBYR2 are T-DNA vectors for use with Agrobacterium DNA delivery, whose structure is similar to that of pBYGFP.RCitation19 (). The GFP coding sequence is replaced by a polylinker with several unique restriction sites (bold font). Both expression cassettes use the CaMV 35S promoter with duplicated enhancer. pBYR1 has the TEV 5′ UTR and vspB 3′ region, while pBYR2 has the TMV 5′ UTR and the extensin (Ext) 3′ region. Both pBYR1 and pBYR2 have been modified to replace the NPTII expression cassette with one encoding the silencing suppressor p19 from TBSV, to produce pBYR1p19 and pBYR2p19.
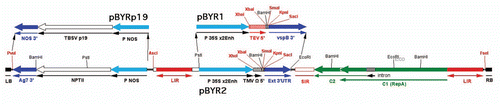
Figure 4 West Nile virus chimeric VLP produced with BeYDV replicon in N. benthamiana plants. (A) Expression of HBc-DIII. Leaves were infiltrated with Agrobacterium transformed with the HBc-DIII construct. Proteins were extracted on days 3 to 7 post Agro-infiltration (DPI) and were analyzed with an ELISA that detects HBcAg.Citation19 Data are mean ± standard error (SE M) of samples from three independent infiltration experiments. (B) Electron microscopy of purified HBc-DIII. HBc-DIII was purified and subject to negative staining with 0.2% aqueous uranyl acetate, and transmission electron microscopy with a Philips CM-12 microscope. (C and D) Western blot analysis of HBc-DIII. Leaf proteins were separated on 12% SDS-PAGE gels under reducing condition and blotted onto PVDF membranes. The membranes were incubated with an anti-HBc antibodyCitation19 (C) or an anti-DIII antibodyCitation39 (D). Lane 1, Protein sample extracted from leaves infiltrated with the HBc-DIII construct; lane 2, Extract from un-infiltrated leaves.
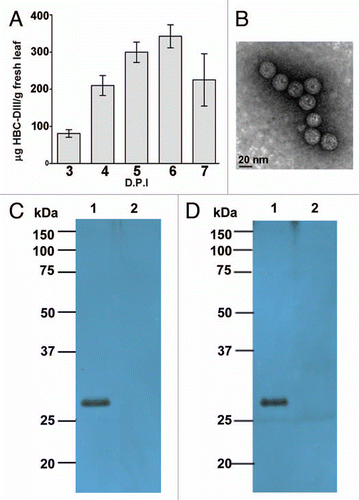
Figure 5 Dual BeYDV replicon vector for co-expression of two genes. The T-DNA construct (top) contains two replicons linked in tandem, with each flanked by an LIR (red blocks). The vector shown is pBY-HL(6D8).R, which drives expression of the heavy and light chains of the anti-Ebola virus mAb 6D8.Citation9 The central LIR functions for both replicons to allow release of two separate single stranded DNA molecules that can enter the rolling circle replication process. The SIR elements are indicated by the yellow ovals. Both replicons are amplified non-competitively to high copy numbers in the nucleus, and generate abundant mRNA's for the heavy and light chains. The pBYK3R replicon (right) contains the C1/C2 gene in its normal position in the viral genome (), and produces Rep mRNA to make Rep protein, which can then act upon both replicons to facilitate their replication. The pBYH2kdel replicon has a large segment of C1/C2 deleted (BamHI fragment, ), since C1/C2 transcription from only one replicon is required. LB and RB, left and right borders of the T-DNA; NPTII, expression cassette for NPTII (facilitates selection of stable transgenic plant lines, but not required for transient expression); 35S/TEV 5′, CaMV 35S promoter linked to tobacco etch virus 5′UTR; H(6D8), coding sequence for mAb 6D8 heavy chain (“H2-SEKDEL” in the replicon below); VSP 3′, soybean vspB 3′ flanking region; 35S/TMV 5′, CaMV 35S promoter linked to TMV 5′UTR; L(6D8), coding sequence for mAb 6D8 light chain (“K chain” in the replicon below); rbcS 3′, tobacco rbcS gene 3′ flanking region; C2/C1, Rep/RepA coding sequence.
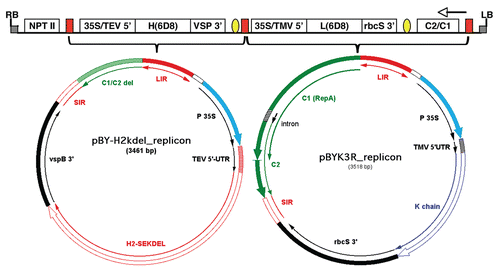
Table 1 Proteins expressed using geminiviral replicons
Acknowledgements
This work was supported by NIH grants U19 AI066332 to H. Mason and U01 AI075549 to Q. Chen.
References
- Mason HS, Lam DMK, Arntzen CJ. Expression of hepatitis B surface antigen in transgenic plants. Proc Natl Acad Sci USA 1992; 89:11745 - 11749
- Voinnet O, Rivas S, Mestre P, Baulcombe D. An enhanced transient expression system in plants based on suppression of gene silencing by the p19 protein of tomato bushy stunt virus. Plant J 2003; 33:949 - 956
- Gleba Y, Klimyuk V, Marillonnet S. Viral vectors for the expression of proteins in plants. Curr Opin Biotechnol 2007; 18:134 - 141
- Lico C, Chen Q, Santi L. Viral vectors for production of recombinant proteins in plants. J Cell Physiol 2008; 216:366 - 377
- Yusibov V, Rabindran S, Commandeur U, Twyman RM, Fischer R. The potential of plant virus vectors for vaccine production. Drugs R D 2006; 7:203 - 217
- Gleba Y, Klimyuk V, Marillonnet S. Magnifection—a new platform for expressing recombinant vaccines in plants. Vaccine 2005; 23:2042 - 2048
- Giritch A, Marillonnet S, Engler C, van Eldik G, Botterman J, Klimyuk V, Gleba Y. Rapid high-yield expression of full-size IgG antibodies in plants coinfected with noncompeting viral vectors. Proc Natl Acad Sci USA 2006; 103:14701 - 14706
- Pushko P, Kort T, Nathan M, Pearce MB, Smith G, Tumpey TM. Recombinant H1N1 virus-like particle vaccine elicits protective immunity in ferrets against the 2009 pandemic H1N1 influenza virus. Vaccine 28:4771 - 4776
- Huang Z, Phoolcharoen W, Lai H, Piensook K, Cardineau G, Zeitlin L, et al. High-level rapid production of full-size monoclonal antibodies in plants by a single-vector DNA replicon system. Biotechnol Bioeng 2010; 106:9 - 17
- Palmer KE, Rybicki EP. The molecular biology of mastreviruses. Adv Virus Res 1998; 50:183 - 234
- Liu L, Davies JW, Stanley J. Mutational analysis of bean yellow dwarf virus, a geminivirus of the genus Mastrevirus that is adapted to dicotyledonous plants. J Gen Virol 1998; 79:2265 - 2274
- Mor TS, Moon YS, Palmer KE, Mason HS. Geminivirus vectors for high-level expression of foreign proteins in plant cells. Biotechnol Bioeng 2003; 81:430 - 437
- Gutierrez C. Geminivirus DNA replication. Cell Mol Life Sci 1999; 56:313 - 329
- Ward A, Etessami P, Stanley J. Expression of a bacterial gene in plants mediated by infectious geminivirus DNA. EMBO J 1988; 7:1583 - 1587
- Matzeit V, Schaefer S, Kammann M, Schalk HJ, Schell J, Gronenborn B. Wheat dwarf virus vectors replicate and express foreign genes in cells of monocotyledonous plants. Plant Cell 1991; 3:247 - 258
- Liu L, Saunders K, Thomas CL, Davies JW, Stanley J. Bean yellow dwarf virus RepA, but not rep, binds to maize retinoblastoma protein and the virus tolerates mutations in the consensus binding motif. Virology 1999; 256:270 - 279
- Zhang X, Mason H. Bean Yellow Dwarf Virus replicons for high-level transgene expression in transgenic plants and cell cultures. Biotechnol Bioeng 2006; 93:271 - 279
- Goodin MM, Zaitlin D, Naidu RA, Lommel SA. Nicotiana benthamiana: its history and future as a model for plant-pathogen interactions. Mol Plant Microbe Interact 2008; 21:1015 - 1026
- Huang Z, Chen Q, Hjelm B, Arntzen C, Mason H. A DNA replicon system for rapid high-level production of virus-like particles in plants. Biotechnol Bioeng 2009; 103:706 - 714
- Regnard GL, Halley-Stott RP, Tanzer FL, Hitzeroth II, Rybicki EP. High level protein expression in plants through the use of a novel autonomously replicating geminivirus shuttle vector. Plant Biotechnol J 2010; 8:38 - 46
- Chen Q. Mou B, Scorza R. Expression and manufacture of pharmaceutical proteins in genetically engineered horticultural plants. Transgenic Horticultural Crops: Challenges and Opportunities 2011; Taylor & Francis
- Chen Q, Tacket CO, Mason H, Mor T, Cardineau GA, Arntzen C. Levine MM. Subunit vaccines produced using plant biotechnology. New Generation Vaccines 2009; New York Informa Healthcare USA, Inc 306 - 315
- Herbst-Kralovetz M, Mason HS, Chen Q. Norwalk virus-like particles as vaccines. Expert Rev Vaccines 2010; 9:299 - 307
- Santi L, Batchelor L, Huang Z, Hjelm B, Kilbourne J, Arntzen CJ, et al. An efficient plant viral expression system generating orally immunogenic Norwalk virus-like particles. Vaccine 2008; 26:1846 - 1854
- Chen Q. Expression and Purification of Pharmaceutical Proteins in Plants Biol Eng 2008; 1:291 - 321
- Bai Y, Glatz CE. Bioprocess considerations for expanded-bed chromatography of crude canola extract: sample preparation and adsorbent reuse. Biotechnol Bioeng 2003; 81:775 - 782
- Menkhaus TJ, Bai Y, Zhang C, Nikolov ZL, Glatz CE. Considerations for the recovery of recombinant proteins from plants. Biotechnol Prog 2004; 20:1001 - 1014
- Valdes R, Gomez L, Padilla S, Brito J, Reyes B, Alvarez T, et al. Large-scale purification of an antibody directed against hepatitis B surface antigen from transgenic tobacco plants. Biochem Biophys Res Commun 2003; 308:94 - 100
- Jiang X, Wang M, Graham DY, Estes MK. Expression, self-assembly and antigenicity of the Norwalk virus capsid protein. J Virol 1992; 66:6527 - 6532
- Venkataram Prasad BV, Hardy ME, Estes MK. Structural studies of recombinant Norwalk capsids. J Infect Dis 2000; 181:317 - 321
- Rolland D, Gauthier M, Dugua JM, Fournier C, Delpech L, Watelet B, et al. Purification of recombinant HBc antigen expressed in Escherichia coli and Pichia pastoris: comparison of size-exclusion chromatography and ultracentrifugation. J Chromatogr B Biomed Sci Appl 2001; 753:51 - 65
- Cook JC, Joyce JG, George HA, Schultz LD, Hurni WM, Jansen KU, et al. Purification of virus-like particles of recombinant human papillomavirus type 11 major capsid protein L1 from Saccharomyces cerevisiae. Protein Expr Purif 1999; 17:477 - 484
- Pattenden LK, Middelberg APJ, Niebert M, Lipin DI. Towards the preparative and large-scale precision manufacture of virus-like particles. Trends Biotechnol 2005; 23:523 - 529
- Davis BS, Chang GJ, Cropp B, Roehrig JT, Martin DA, Mitchell CJ, et al. West Nile virus recombinant DNA vaccine protects mouse and horse from virus challenge and expresses in vitro a noninfectious recombinant antigen that can be used in enzyme-linked immunosorbent assays. J Virol 2001; 75:4040 - 4047
- Hubalek Z, Halouzka J. West Nile fever—a reemerging mosquito-borne viral disease in Europe. Emerg Inf Dis 1999; 5:643 - 650
- Petersen SV, Thiel S, Jensenius JC. The mannan-binding lectin pathway of complement activation: biology and disease association. Mol Immunol 2001; 38:133 - 149
- Diamond MS, Mehlhop E, Oliphant T, Samuel MA. The host immunologic response to West Nile encephalitis virus. Front Biosci 2009; 14:3024 - 3034
- Vaughan K, Greenbaum J, Blythe M, Peters B, Sette A. Meta-analysis of All Immune Epitope Data in the Flavivirus Genus: Inventory of Current Immune Epitope Data Status in the Context of Virus Immunity and Immunopathology. Viral Immunol 2010; 23:259 - 284
- Lai H, Engle M, Fuchs A, Keller T, Johnson S, Gorlatov S, et al. Monoclonal antibody produced in plants efficiently treats West Nile virus infection in mice. Proc Natl Acad Sci USA 2010; 107:2419 - 2424
- Vicente T, Sousa MFQ, Peixoto C, Mota JPB, Alves PM, Carrondo MJT. Anion-exchange membrane chromatography for purification of rotavirus-like particles. J Membrane Sci 2008; 311:270 - 283
- Palomares LA, Ramírez OT. Challenges for the production of virus-like particles in insect cells: The case of rotavirus-like particles. Biochem Engineer J 2009; 45:158 - 167
- Sweeney SF, Woehrle GH, Hutchison JE. Rapid Purification and Size Separation of Gold Nanoparticles via Diafiltration. J Am Chem Soc 2006; 128:3190 - 3197