Abstract
While a few studies have demonstrated the benefit of PEGylation in islet transplantation, most have employed renal subcapsular models and none have performed direct comparisons of islet mass in intraportal islet transplantation using islet magnetic resonance imaging (MRI). In this study, our aim was to demonstrate the benefit of PEGylation in the early post-transplant period of intraportal islet transplantation with a novel algorithm for islet MRI. Islets were PEGylated after ferucarbotran labeling in a rat syngeneic intraportal islet transplantation model followed by comparisons of post-transplant glycemic levels in recipient rats infused with PEGylated (n = 12) and non-PEGylated (n = 13) islets. The total area of hypointense spots and the number of hypointense spots larger than 1.758 mm2 of PEGylated and non-PEGylated islets were quantitatively compared. The total area of hypointense spots (P < 0.05) and the number of hypointense spots larger than 1.758 mm2 (P < 0.05) were higher in the PEGylated islet group 7 and 14 days post translation (DPT). These results translated into better post-transplant outcomes in the PEGylated islet group 28 DPT. In validation experiments, MRI parameters obtained 1, 7, and 14 DPT predicted normoglycemia 4 wk post-transplantation. We directly demonstrated the benefit of islet PEGylation in protection against nonspecific islet destruction in the early post-transplant period of intraportal islet transplantation using a novel algorithm for islet MRI. This novel algorithm could serve as a useful tool to demonstrate such benefit in future clinical trials of islet transplantation using PEGylated islets.
Introduction
Surface camouflage of islets using polyethylene glycol (PEG) has been reported to reduce the need for immunosuppressants in rodent allogeneic islet transplantation.Citation1 Triple PEGylation of islets also enables long-term islet graft survival without immunosuppressants in rodent allogeneic islet transplantation.Citation2 Recently, PEGylation of islets and low-dose treatment with FK506 and MR1 enabled long-term islet graft survival in a rodent xenogeneic islet transplantation model.Citation3
While the results of renal subcapsular islet transplantation in rodents are promising, it does not ensure the benefit in clinical islet transplantation because most of the transplanted islets are destructed by the instant blood-mediated inflammatory reaction (IBMIR) immediately after clinical intraportal islet transplantation.Citation4 To date, little is known about the benefit of PEGylation in intraportal islet transplantation. Although a few studies have demonstrated the efficacy of PEGylation in attenuating IBMIR in intraportal islet transplantation, the results have been shown in terms of recipient glycemic levels, markers of inflammation and coagulation, and/or histologic analysis after sacrifice.Citation5,Citation6 Therefore, the advantages of PEGylation in the early post-transplant period of intraportal islet transplantation have not been demonstrated through real-time determination of functional islet mass before changes in glucose levels become evident. This could be a hurdle for future clinical trials of PEGylated islet transplantation, because the majority of islet injury in clinical islet transplantation takes place before changes in recipient glycemic levels become apparent, and histologic analysis is almost impossible.
Recently, we reported that real-time quantification of functional islet mass can be achieved through magnetic resonance imaging (MRI) of superparamagnetic iron oxide nanoparticle (SPIO)-labeled islets by counting the total area of hypointense spots or the number of hypointense spots larger than pre-determined criterion in magnetic resonance imaging (MRI) of superparamagnetic iron oxide nanoparticles (SPIO)-labeled islets. The algorithm we developed was based on the observation that counting small hypointense spots confounds the quantification of functional islet mass; some of the small hypointense spots may represent non-functioning byproducts of islet transplantation.Citation7 We hypothesized that using this novel algorithm of islet MRI could provide a real-time demonstration of the benefit of PEGylation in the early post-transplant period of intraportal islet transplantation.
We aimed to demonstrate the benefit of PEGylation in the early post-transplant period of intraportal islet transplantation with a novel algorithm of islet MRI. For this purpose, we first compared the MRI parameters between the recipients of PEGylated and non-PEGylated islets, then validated the MRI parameters in our previous study which utilized non-PEGylated isletsCitation7 in euglycemic and hyperglycemic recipients of PEGylated or non-PEGylated islets.
Results
Changes in islet MRI findings and blood glucose levels in control and PEGylated islet groups between 1 d post-transplantation (DPT) and 30 DPT
PEGylation of islets did not affect islet morphology or the glucose-induced insulin secretion index in vitro (). Ex vivo imaging of PEGylated islets labeled with ferucarbotran revealed that most islets were visible in the corresponding islet MR images (). Hypointense spots were visible in in vivo MR images of concurrently PEGylated and ferucarbotran-labeled islets ().
Figure 1. (A) Dithizone stain of untreated (a) and PEGylated (b) islets, and acridine orange and propidium iodide stain of untreated (c) and PEGylated (d) islets. Purple indicates dithizone-stained β cells (a, b). Green indicates acridine orange-stained live cells and red indicates propidium iodide-stained dead cells (c, d). Scale bars indicate 50μm. (B) Glucose-stimulated insulin secretion of control (n = 8) and PEGylated islets (n = 8). (C) Ex vivo imaging of PEGylated islets labeled with ferucarbotran. Islets in 1% agarose gel are in the left panel while corresponding magnetic resonance images are in the right panel. The scale bar indicates 50 μm. (D) Representative MR images of PEGylated (PEG) and non-PEGylated (control) islets. The images were obtained 7 d after islet transplantation. Arrows indicate hypointense spots representing SPIO-labeled islets.
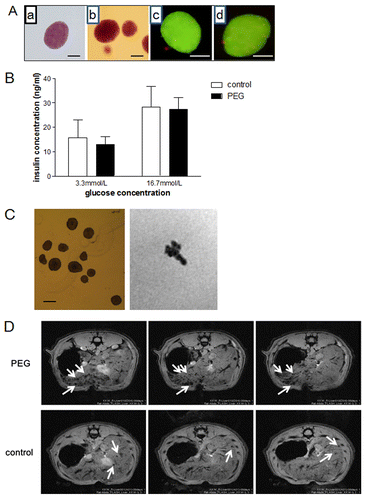
Recipients of the PEGylated islets (PEGylated islet group) had a greater total area of hypointense spots and a greater number of large (> 1.758 mm2) hypointense spots than those of the control islet group 7 and 14 DPT (). There was no difference in the total number of hypointense spots between the groups (data not shown). Blood glucose levels were not significantly different between the PEGylated and control islet groups for up to 2 wk post-transplantation. There was a borderline significant difference in blood glucose levels between the groups 21 and 28 DPT (P = 0.0548 and 0.0605, respectively; ). The PEGylated islet group showed earlier diabetes reversal (P = 0.0186, ): 8% achieved normoglycemia at 7 DPT, 25% at 14 DPT and 67% at 30 DPT. In contrast, only 15% of the control group achieved normoglycemia at 30 DPT.
Figure 2. (A–B) Comparison of the total area of all hypointense spots (A) and the number of large (> 1.758 mm2) hypointense spots (B) between control and PEGylated islet groups 1, 7, and 14 d post-transplantation. There were six animals in the control islet group and eight in the PEGylated islet group on day 1 (animals that showed conglomerated hypointense spots were excluded from the image analysis). *P < 0.05. (C) Comparison of blood glucose levels between control and PEGylated islet groups during 4 wk post-transplantation. (D) The cumulative proportion of recipients that achieved normoglycemia in the PEGylated and control islet groups.
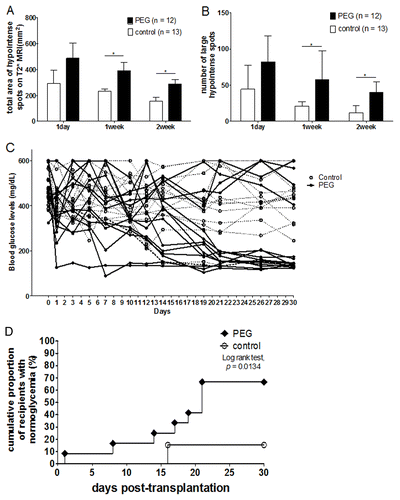
In the histologic analyses of recipient livers (control islets, n = 2; PEGylated islets, n = 2) conducted 1 wk after transplantation, a total of 29 control islets and 36 PEGylated islets were found. All of the islets appeared as hypointense spots in the counterpart MR images. The number of Prussian blue-stained spots was less than 10 spots per islet in most islets (). Only a few islets were co-stained with > 10 prussian blue-stained spots in both groups (n = 5, control islets; n = 4, PEGylated islets; ). The pattern of Prussian blue/insulin co-staining was not different between the control and PEGylation groups. Representative liver histology of recipients is presented in (control group) and 3D (PEGylated islets group).
Figure 3. Histologic analyses of transplanted islets 1 wk after the islet transplantation and the counterpart MR images. (A) An example of insulin-stained islet which was co-stained with more than 10 Prussian blue-stained spots. Low power field histology and its counterpart MR image (left-hand side), and the high power field image of the area indicated by rectangle in the MRI and low power image (right-hand side). This example was from the recipient of control islets. (B) An example of insulin-stained islet which was co-stained with less than 10 Prussian blue-stained spots (right-hand side). Low power field histology and its counterpart MR image (left-hand side), and the high power field image of the area indicated by rectangle in the MRI and low power image (right-hand side). This example was from the recipient of PEGylated islets. (C) Representative high power field images of control islets. (D) Representative high power field images of PEGylated islets. Scale bars indicate 50 μm in each figure (A–D).
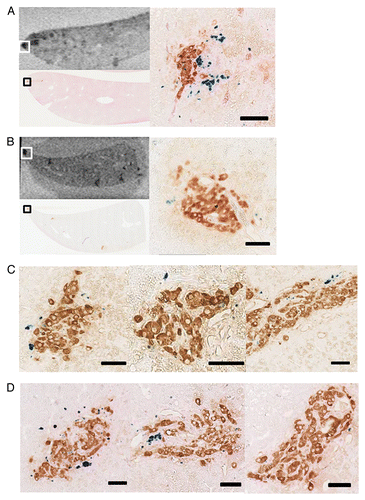
Validation of MRI parameters in euglycemic and hyperglycemic recipients of PEGylated or non-PEGylated islets
Because our previous study used mostly non-PEGylated islets to determine the criterion for large (> 1.758 mm2) hypointense spots,Citation7 we re-validated the predictive value of MRI for PEGylated islets. In the normoglycemia group, which was comprised of eight recipients of PEGylated islets and one recipient of non-PEGylated islets, the total area of hypointense spots and the number of large hypointense spots obtained 1, 7, and 14 DPT were consistently higher than those of the hyperglycemia group (). When combined PEGylated and non-PEGylated islets were analyzed, there were linear associations between the total area of hypointense spots (P = 0.0002, R2 = 0.5003; ) and the number of large hypointense spots (P = 0.0007, R2 = 0.3987; ) obtained at 7 DPT and non-fasting blood glucose levels at 4 wk post-transplantation. Among recipients of PEGylated islets, there was a significant difference between the normoglycemia and hyperglycemia groups in the total area of hypointense spots (P = 0.0040, ) and the number of large hypointense spots (P = 0.0084, ) at 7 DPT.
Figure 4. Validation of MRI parameters in syngeneic islet transplantation. (A–B) Total area of hypointense spots (A) and number of large hypointense spots (B) on T2* MRI 1, 7, and 14 d post-transplantation. There were 6 animals in the hyperglycemia group and 8 in the normoglycemia group 1 DPT. Animals that showed conglomerated hypointense spots were excluded from the image analysis. (C–D) Correlation between the total area of hypointense spots (C) and number of large (> 1.758 mm2) hypointense spots (D) 1 wk post-transplantation and blood glucose levels 4 wk post-transplantation in PEGylated (PEG) and non-PEGylated (control) islets. (E–F) Comparison of the total area of hypointense spots (E) and number of large (> 1.758 mm2) hypointense spots (F) 1 wk post-transplantation between hyperglycemic and euglycemic recipients transplanted with PEGylated islets. *P < 0.05; **P < 0.01; ***P < 0.001.
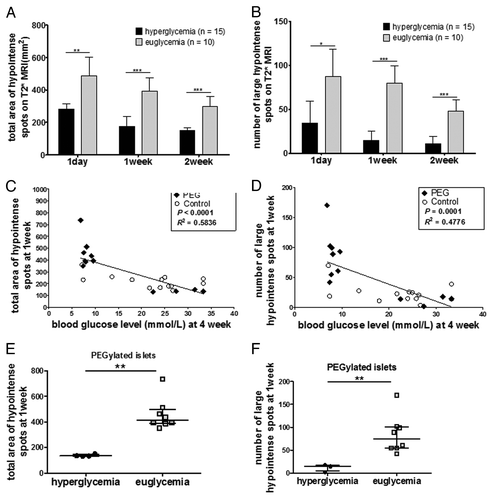
The predictive value of the MRI parameters 1,7, and 14 DPT for classification of recipients to the normoglycemia group, defined by 2 consecutive blood glucose levels < 200 mg/dL by 4 wk post-transplantation, was tested using a receiver-operator characteristics (ROC) analysis. When combined PEGylated and non-PEGylated islets were analyzed, ROC analysis revealed that the total area of hypointense spots and the number of large hypointense spots obtained 1, 7, and 14 DPT significantly predicted classification into the normoglycemia group. The predictive value of the total area of hypointense spots and the number of large hypointense spots was similar in terms of area under the curve. In this analysis, the total area of hypointense spots obtained 7 DPT (sensitivity 90% and specificity 100% at the cut-off value of 304.5 mm2) and the number of large hypointense spots obtained 7 DPT (sensitivity 90% and specificity 100% at the cut-off value of 40.5) had the highest predictive value ().
Table 1. The predictive value of the MRI parameters 1,7, and 14 DPT for classification of recipients to the normoglycemia group*
Discussion
In this study the superiority of PEGylated islets in syngeneic intraportal islet transplantation was directly demonstrated by islet MRI in the early post-transplant period, before glycemic levels of recipients became apparent. In addition, the predictive value of quantitative indicators in islet MRI determined in our previous studyCitation7 was successfully reproduced using PEGylated islets.
Although a few studies have demonstrated the benefit of PEGylation mostly in renal subcapsular islet transplantation,Citation1,Citation3,Citation6 direct comparison of islet mass in intraportal islet transplantation using islet MRI has not been performed because simply counting the number of hypointense spots has failed to correlate with the post-transplant outcomes.Citation8,Citation9 In this study, we successfully reproduced the quantitative association between the novel MRI parameters in the early post-transplant period and the later changes in glycemic levels of recipients.Citation7 While the efficacy and safety of SPIO labeling in terms of islet viability and ex vivo and in vivo insulin secretion have been established,Citation8,Citation10-Citation16 the results of this study provide in vitro and in vivo evidence for the efficacy and safety of concurrent PEGylation and SPIO labeling. Moreover, our technique for conventional PEGylation without further modification after SPIO labeling is easily performed using procedures similar to those used for standard islet culture. This could be advantageous over the alternative strategy of concurrent SPIO labeling and surface camouflage, namely the chemical conjugation of SPIO onto islet surfaces through a PEG linker.Citation17 Because the conventional PEGylation used in this study is widely used to develop drugs for clinical medicineCitation18 and SPIO labeling of islets has been tested in clinical islet transplantation,Citation8,Citation9 the successful combination of both techniques in this study indicates that islet imaging could be a useful tool to follow the kinetics of PEGylated islet graft, provided that substitutes for ferucarbotran become available in clinical medicine in the near future.Citation19
The mechanism of cellular uptake of SPIO in islets is known as fluid-phase endocytosis.Citation17,Citation20,Citation21 Because labeling with ferucarbotran preceded the PEGylation process in this study, it is reasonable to assume that surface modification with PEG did not affect the uptake process of SPIO. To determine if PEGylation could affect the translocation of SPIO from intracellular to extracellular space during the early post-transplant period, we collected histological data 1 wk after transplantation. In this analysis, the pattern of Prussian blue / insulin co-staining was not different between the control and PEGylation groups. Additional findings from this study indicated that PEGylation did not alter the efficacy of SPIO labeling. First, when we compared recipients within the PEGylated islet group, there was significant difference between euglycemic and hyperglycemic recipients in the total area of hypointense spots and the number of large hypointense spots (). Second, the linear association between post-transplant glycemic levels and each parameter in islet MRI was still valid when PEGylated and non-PEGylated islet recipients were analyzed in combination ().
To date, only a few research groups have investigated islet PEGylation using intraportal islet transplantation models; it is not clear whether PEGylation protects against the IBMIR. Recently, Teramura et al. investigated whether surface modification of islets with PEG-lipid or PEG-urokinase provided benefits in a mouse model of syngeneic intraportal islet transplantation.Citation6 PEGylation had a positive impact on insulin levels over a 60 min period after islet transplantation, glucose tolerance 64 DPT, and histochemical analysis 64 DPT.Citation6 Another report showed that PEGylation of islets protected the islet grafts from the IBMIR in terms of platelet adhesion and neutrophil infiltration in a xenogeneic islet transplantation model.Citation5 However, these studies did not provide direct evidence for difference in islet mass during the early post-transplant period. In this study, the differences in islet mass between control and PEGylated islet groups were apparent as early as 1 d after transplantation in islet MRI. However, the differences were not translated into blood glucose levels until later time points due to the time lag caused by islet engraftment, which was similar to the observation in the study by Teramura et al.Citation6
Several limitations of this study should be addressed. First, the results of this study should be reproduced in 1.5- or 3.0-T MR images of islets, although previous studies demonstrated the compatibility of SPIO-labeled islets in clinical MRI.Citation22 Second, the focus of this study was proof-of-concept using a rodent syngeneic intraportal islet transplantation model. Before these methods can be applied to clinical islet transplantation, the results of this study should be examined in large animals using clinically available iron oxide nanoparticles.Citation19 Third, the possibility of islet regeneration after diabetes induction by streptozotocin was not excluded in this study.
In conclusion, islet MRI was used to successfully demonstrate superior post-transplant outcomes with PEGylated islets before changes in glycemia were apparent, providing direct evidence for the benefit of islet PEGylation in protection against nonspecific destruction of islets in early post-transplant period of intraportal islet transplantation. SPIO labeling of islets before PEGylation could serve as a useful tool to demonstrate this benefit in future clinical trials of islet transplantation using PEGylated islets.
Materials and Methods
All of the animal procedures in this study were approved by the Institutional Animal Care and Use Committee of the Samsung Medical Center, Seoul, Republic of Korea.
Pancreatic islet isolation and SPIO nanoparticle labeling
Eight-week-old Lewis rats (Charles River Technology) were used for syngeneic islet transplantation experiments. Diabetes was induced by a single intraperitoneal (IP) injection of streptozotocin (STZ; Sigma) at doses of 65 mg/kg. Rats were regarded as diabetic and designated as recipients when two consecutive blood glucose level readings were higher than 300 mg/dl. Islet transplantation was performed at approximately 1 wk following induction of diabetes with streptozotocin. Islets were isolated using intraductal injection with collagenase P (Roche) and purified using Biocoll (Biochrom). Isolated islets were cultured while free-floating in 10 mL of Opti-MEM (Gibco) in a humidified CO2 incubator at 37 °C and 5% CO2 atmosphere.
Isolated islets were labeled by culturing for 48 h in Opti-MEM (Gibco) supplemented with 5 μl/mL of ferucarbotran (Resovist®, Bayer Schering Pharma AG).Citation21 Preliminary experiments demonstrated superior labeling efficacy when isolated islets were allowed to culture in labeling medium for 48 vs. 24 h.
PEGylation of islet surface
After labeling with ferucarbotran, islets were washed with Hank’s solution. After re-suspension in 10 mL Hank’s solution (pH 7.4), 5 mL of Hank’s solution (pH 7.4) mixed with 7.2 mg methoxy-PEG-CH2COO-N-hydroxysuccinimide ester (mPEG, MW 5000, UNBRIGHT ME-050AS; NOF Co.) was added. The islets were then incubated at 37 °C under 5% CO2 atmosphere for 30 min. After incubation, the PEGylated islets were washed twice in Hank’s solution and islet transplantation was performed immediately after washing to avoid agglomeration of islets. The agglomeration of the PEGylated islets was easily removed by gentle pipetting during the washing procedure.
Islet transplantation
In the rat syngeneic islet transplantation model, ferucarbotran-labeled PEGylated (n = 13) and non-PEGylated (n = 12) rat islets were infused via the portal vein in anesthetized animals. The mass of the transplanted ferucarbotran-labeled islets was 1500 islet equivalent (IE) of islets 150 μm in diameter. IE number was calculated using the Ricordi algorithm by classifying islets according to their diameter.Citation24 The typical size of islets was 100–200 μm in diameter. With one exception, the same batch of islets was divided into two equal portions, half of which was used for experiments in the control group and half for experiments in the PEGylation group. For 4 wk following transplantation, non-fasting blood glucose levels were recorded 3 times a week for the first 2 wk and twice a week for the last 2 wk. The normoglycemia group was defined as those recipients with two consecutive blood glucose levels of < 200 mg/dL by 4 wk post-transplantation. Recipients that did not meet these criteria were classified as hyperglycemic.
In vivo MR imaging of transplanted islets
All rat images were obtained using a 7.0 T MRI System (Bruker-Biospin) equipped with a 20-cm gradient set capable of supplying up to 400 mT/m with a 100-μsec rise-time. MRI was performed at 1 d, 1 wk, and 2 wk after islet transplantation. The detailed imaging protocol of rodent MRI used in this study was previously published elsewhere.Citation7 Briefly, anesthetized rats were fixed in the prone position. Iron-labeled islets appeared as hypointense signals on T2*-weighted MR images. Signal intensities were measured for 20 slices acquired throughout the liver. To avoid transversal projections of the vascular bed, hypointense regions of interest (ROI) were defined as those localized within the liver tissue, that had a round or elliptical shape, and appeared in up to 3 adjacent image slices.Citation22 When conglomerated hypointense spots were present, the animal was excluded from the quantitative analysis of MRI findings. Results were provided as the number of ROIs and the total area of ROIs (mm2). Based on the results of our previous study,Citation7 the total area and the number of large (> 1.758 mm2) hypointense spots can be considered as quantitative indicators of functional islet mass. The definition of large hypointense spots was based on the results of our previous studyCitation7 in which the size of hypointense spots corresponding to the 90th percentile (1.758 mm2) was found to be the optimal cut-off value.
In vitro assessment of islet viability and function
In order to assess islet viability, acridine orange (0.67 μmol/L) and propidium iodide (75 μmol/L) staining were used to simultaneously observe living and nonviable islets. To determine the composition of islets, cultured islets were stained with dithizone (Sigma Chemicals). For glucose stimulatory insulin secretion assays, the islets were incubated for 1 h in Krebs-Ringer bicarbonate buffer (KRBB) supplemented with 0.2% bovine serum albumin (Sigma) and 3.3 mM glucose followed by re-incubation with low (3.3 mM, basal) and high (16.7 mM) glucose for 1 h. The supernatant was collected and the amount of secreted insulin was measured using a rat insulin ELISA kit (Millipore Co.). Glucose-stimulated insulin release was expressed as the stimulation index, calculated as the ratio of stimulated (16.7 mM glucose) to basal (3.3 mM glucose) insulin release.
Concurrent ex-vivo imaging and histologic analysis of the intraportally transplanted islets
One-week after the transplantation of ferucarbotran-labeled syngeneic rat islets, the whole recipient livers (n = 2, control group; n = 2, PEGylated islets group) were sliced with 4mm-thickness immediately after sacrifice. As a result, a total of 56 slices per each group was analyzed. The MRI of each liver slice was obtained immediately after the sacrifice. The closest levels of MRI and histology were matched for the comparison of MRI and histology. Because the thickness of slice in MRI (0.5 mm) was thicker than the thickness of histology slice, MR images contains more hypointense spots than the number of islets in their counterpart histology slice. All of the islets throughout the liver were examined for the presence of hypointense spots in their counterpart MR images. Each slice of the recipient liver was stained with guinea pig anti-swine insulin (1: 500; DAKO) and Prussian blue with iron stain kit (Sigma-Aldrich) according to the manufacturer’s recommendation.
Statistical analysis
Results are expressed as mean ± standard deviation (SD) or median and interquartile range, as appropriate. Continuous variables were compared using Student t-test or Mann–Whitney U test, as appropriate. Correlations were analyzed using Spearman rank test. The value of each MRI parameter in predicting that a recipient will be designated to the normoglycemia group was assessed by receiver-operator characteristics (ROC) analysis. We compared the area under the ROC curve of each parameter, created by plotting the fraction of true positives out of the total actual positives (true positive rate) vs. the fraction of false positives out of the total actual negatives (false positive rate), at various threshold settings. The area under the ROC curve is 0.5 if the parameter has no predictive value at all, and 1.0 if the parameter perfectly predicts the outcome. Optimal cut-off value of each parameter was chosen by Youden index. P values of < 0.05 were considered statistically significant.
Disclosure of Potential Conflicts of Interest
No potential conflicts of interest were disclosed.
Acknowledgments
This work was supported by a National Research Foundation of Korea (NRF) grant funded by the Korean government (MEST) (2012R1A2A2A01013810).
**Author Note: Please cite reference Citation23 in text.
References
- Lee DY, Lee S, Nam JH, Byun Y. Minimization of immunosuppressive therapy after islet transplantation: combined action of heme oxygenase-1 and PEGylation to islet. Am J Transplant 2006; 6:1820 - 8; http://dx.doi.org/10.1111/j.1600-6143.2006.01414.x; PMID: 16780547
- Lee DY, Park SJ, Lee S, Nam JH, Byun Y. Highly poly(ethylene) glycolylated islets improve long-term islet allograft survival without immunosuppressive medication. Tissue Eng 2007; 13:2133 - 41; http://dx.doi.org/10.1089/ten.2006.0009; PMID: 17516853
- Jeong JH, Hong SW, Hong S, Yook S, Jung Y, Park JB, Khue CD, Im BH, Seo J, Lee H, et al. Surface camouflage of pancreatic islets using 6-arm-PEG-catechol in combined therapy with tacrolimus and anti-CD154 monoclonal antibody for xenotransplantation. Biomaterials 2011; 32:7961 - 70; http://dx.doi.org/10.1016/j.biomaterials.2011.06.068; PMID: 21831422
- Korsgren O, Lundgren T, Felldin M, Foss A, Isaksson B, Permert J, Persson NH, Rafael E, Rydén M, Salmela K, et al. Optimising islet engraftment is critical for successful clinical islet transplantation. Diabetologia 2008; 51:227 - 32; http://dx.doi.org/10.1007/s00125-007-0868-9; PMID: 18040664
- Hwang JW, Jung HS, Lee DY. Inhibition of platelet adhesion onto intrahepatically transplanted islets using PEGylation for attenuating instant blood-mediated inflammatory reaction (IBMIR). J Control Release 2011; 152:Suppl 1 e213 - 4; http://dx.doi.org/10.1016/j.jconrel.2011.09.016; PMID: 22195862
- Teramura Y, Iwata H. Improvement of graft survival by surface modification with poly(ethylene glycol)-lipid and urokinase in intraportal islet transplantation. Transplantation 2011; 91:271 - 8; http://dx.doi.org/10.1097/TP.0b013e3182034fa4; PMID: 21344734
- Kim JH, Jin SM, Oh SH, Lee S, Oh BJ, Kim SK, Suh S, Lee JH, Jung HS, Lee MS, et al. Counting small hypointense spots confounds the quantification of functional islet mass based on islet MRI. Am J Transplant 2012; 12:1303 - 12; http://dx.doi.org/10.1111/j.1600-6143.2011.03941.x; PMID: 22299723
- Toso C, Vallee JP, Morel P, Ris F, Demuylder-Mischler S, Lepetit-Coiffe M, Marangon N, Saudek F, James Shapiro AM, Bosco D, et al. Clinical magnetic resonance imaging of pancreatic islet grafts after iron nanoparticle labeling. Am J Transplant 2008; 8:701 - 6; http://dx.doi.org/10.1111/j.1600-6143.2007.02120.x; PMID: 18294167
- Saudek F, Jirák D, Girman P, Herynek V, Dezortová M, Kríz J, Peregrin J, Berková Z, Zacharovová K, Hájek M. Magnetic resonance imaging of pancreatic islets transplanted into the liver in humans. Transplantation 2010; 90:1602 - 6; http://dx.doi.org/10.1097/TP.0b013e3181ffba5e; PMID: 21197715
- Medarova Z, Vallabhajosyula P, Tena A, Evgenov N, Pantazopoulos P, Tchipashvili V, Weir G, Sachs D, Moore A. In vivo imaging of autologous islet grafts in the liver and under the kidney capsule in non-human primates. Transplantation 2009; 87:1659 - 66; http://dx.doi.org/10.1097/TP.0b013e3181a5cbc0; PMID: 19502957
- Marzola P, Longoni B, Szilagyi E, Merigo F, Nicolato E, Fiorini S, Paoli GT, Benati D, Mosca F, Sbarbati A. In vivo visualization of transplanted pancreatic islets by MRI: comparison between in vivo, histological and electron microscopy findings. Contrast Media Mol Imaging 2009; 4:135 - 42; http://dx.doi.org/10.1002/cmmi.274; PMID: 19489041
- Kim HS, Choi Y, Song IC, Moon WK. Magnetic resonance imaging and biological properties of pancreatic islets labeled with iron oxide nanoparticles. NMR Biomed 2009; 22:852 - 6; http://dx.doi.org/10.1002/nbm.1398; PMID: 19489018
- Jirak D, Kriz J, Strzelecki M, Yang J, Hasilo C, White DJ, Foster PJ. Monitoring the survival of islet transplants by MRI using a novel technique for their automated detection and quantification. MAGMA 2009; 22:257 - 65; http://dx.doi.org/10.1007/s10334-009-0172-4; PMID: 19390886
- Ris F, Lepetit-Coiffe M, Meda P, Crowe LA, Toso C, Armanet M, et al. Assessment of human islet labeling with clinical grade iron nanoparticles prior to transplantation for graft monitoring by MRI. Cell Transplant.
- Patil S, Jirák D, Saudek F, Hájek M, Scheffler K. Positive contrast visualization of SPIO-labeled pancreatic islets using echo-dephased steady-state free precession. Eur Radiol 2011; 21:214 - 20; http://dx.doi.org/10.1007/s00330-010-1909-1; PMID: 20683599
- Leoni L, Roman BB. MR imaging of pancreatic islets: tracking isolation, transplantation and function. Curr Pharm Des 2010; 16:1582 - 94; http://dx.doi.org/10.2174/138161210791164171; PMID: 20146663
- Jung MJ, Lee SS, Hwang YH, Jung HS, Hwang JW, Kim MJ, Yoon S, Lee DY. MRI of transplanted surface-labeled pancreatic islets with heparinized superparamagnetic iron oxide nanoparticles. Biomaterials 2011; 32:9391 - 400; http://dx.doi.org/10.1016/j.biomaterials.2011.08.070; PMID: 21903255
- Banerjee SS, Aher N, Patil R, Khandare J. Poly(ethylene glycol)-Prodrug Conjugates: Concept, Design, and Applications. J Drug Deliv 2012; 2012:103973.
- Thu MS, Bryant LH, Coppola T, Jordan EK, Budde MD, Lewis BK, Chaudhry A, Ren J, Varma NR, Arbab AS, et al. Self-assembling nanocomplexes by combining ferumoxytol, heparin and protamine for cell tracking by magnetic resonance imaging. Nat Med 2012; 18:463 - 7; http://dx.doi.org/10.1038/nm.2666; PMID: 22366951
- Lee H, Lee E, Kim K, Jang NK, Jeong YY, Jon S. Antibiofouling polymer-coated superparamagnetic iron oxide nanoparticles as potential magnetic resonance contrast agents for in vivo cancer imaging. J Am Chem Soc 2006; 128:7383 - 9; http://dx.doi.org/10.1021/ja061529k; PMID: 16734494
- Berkova Z, Jirak D, Zacharovova K, Kriz J, Lodererova A, Girman P, Koblas T, Dovolilova E, Vancova M, Hajek M, et al. Labeling of pancreatic islets with iron oxide nanoparticles for in vivo detection with magnetic resonance. Transplantation 2008; 85:155 - 9; http://dx.doi.org/10.1097/01.tp.0000297247.08627.ff; PMID: 18192927
- Malosio ML, Esposito A, Poletti A, Chiaretti S, Piemonti L, Melzi R, Nano R, Tedoldi F, Canu T, Santambrogio P, et al. Improving the procedure for detection of intrahepatic transplanted islets by magnetic resonance imaging. Am J Transplant 2009; 9:2372 - 82; http://dx.doi.org/10.1111/j.1600-6143.2009.02791.x; PMID: 19681816
- Daldrup-Link HE, Rudelius M, Piontek G, Metz S, Bräuer R, Debus G, Corot C, Schlegel J, Link TM, Peschel C, et al. Migration of iron oxide-labeled human hematopoietic progenitor cells in a mouse model: in vivo monitoring with 1.5-T MR imaging equipment. Radiology 2005; 234:197 - 205; http://dx.doi.org/10.1148/radiol.2341031236; PMID: 15618382
- Ricordi C, Gray DW, Hering BJ, Kaufman DB, Warnock GL, Kneteman NM, Lake SP, London NJ, Socci C, Alejandro R, et al. Islet isolation assessment in man and large animals. Acta Diabetol Lat 1990; 27:185 - 95; http://dx.doi.org/10.1007/BF02581331; PMID: 2075782