Abstract
Regular exercise has been shown to improve many complications of Type 1 diabetes mellitus (T1DM) including enhanced glucose tolerance and increased cardiac function. While exercise training has been shown to increase insulin content in pancreatic islets of rats with T1DM, experimental models were severely hyperglycemic and not undergoing insulin treatment. Further, research to date has yet to determine how exercise training alters glucagon content in pancreatic islets. The purpose of the present investigation was to determine the impact of a 10-week aerobic training program on pancreatic islet composition in insulin-treated rats with T1DM. Second, it was determined whether the acute, exercise-mediated reduction in blood glucose experienced in rats with T1DM would become larger in magnitude following aerobic exercise training. Diabetes was induced in male Sprague-Dawley rats by multiple low dose injections of streptozotocin (20mg/kg i.p.) and moderate intensity aerobic exercise training was performed on a motorized treadmill for one hour per day for a total of 10 weeks. Rats with T1DM demonstrated significantly less islet insulin, and significantly more islet glucagon hormone content compared with non-T1DM rats, which did not significantly change following aerobic training. The reduction in blood glucose in response to a single exercise bout was similar across 10 weeks of training. Results also support the view that different subpopulations of islets exist, as small islets (<50 μm diameter) had significantly more insulin and glucagon in rats with and without T1DM.
Introduction
Type 1 diabetes mellitus (T1DM) is characterized by the autoimmune destruction of insulin producing β-cells within pancreatic islets, resulting in the requirement of exogenous insulin in order to limit chronic hyperglycemia and prevent long-term complications.Citation1 Accompanying insulin supplementation is the risk of overcorrection, leading to an increased incidence of severe hypoglycemia.Citation1,Citation2 Further, the lack of tight insulin management in T1DM often leads to the development of insulin insensitivity and increased cardiovascular risk.Citation3,Citation4 Regular exercise has been shown to improve many diabetes-related complications including enhanced glucose tolerance and improved cardiac function.Citation4,Citation5 However, due to a lack of insulin control and heightened incidence of hypoglycemia during and following exercise, many patients with T1DM refrain from exercise.Citation6,Citation7
Insulin and glucagon are the 2 most important hormonal regulators of blood glucose concentrations in response to hypoglycemia, as well as glucose production during moderate intensity aerobic exercise.Citation8-Citation11 While a reduction in islet insulin content and its release from pancreatic islets is well characterized in T1DM, impaired glucagon release from islet α-cells is poorly understood. Shortly after the onset of the disease, secretion of glucagon is absent upon exposure to hypoglycemia, concomitant with an overall decrease in the hepatic response to glucagon.Citation12,Citation13 Interestingly, impaired glucagon function occurs despite postprandial hyperglucagonemia becoming more apparent as the disease progresses.Citation14 Experimentally, α-cell expansion is evident in streptozotocin (STZ)-induced rats with T1DM in comparison to non-T1DM rats.Citation15
It is well recognized that following aerobic training both insulin and glucagon sensitivity are improved.Citation4,Citation16,Citation17 It is unknown if exercise training can improve pancreatic islet hormone content in populations with T1DM. Findings have supported a role for aerobic training in improving insulin content within pancreatic islets of STZ-induced T1DM animals; however, research to date has been completed in severely hyperglycemic animals not treated with exogenous insulin.Citation18,Citation19 This model is not reflective of the exercising population with T1DM, as patients are not likely to partake in exercise without accompanying exogenous insulin treatment. Further, most patients with T1DM display moderate levels of blood glucose even in the presence of insulin supplementation due to the difficulty of maintaining tightly regulated blood glucose concentrations.Citation20 Exercise training has been shown to limit the progression of type 2 diabetes onset by inducing β-cell hyperplasia, stimulating proliferation and suppressing apoptosis through the activation of the insulin/IGF-1 signaling cascade.Citation21 Further, exercise training can stimulate islet growth and survival pathways in non-diabetic rats.Citation22 It has yet to be established if glucagon content in T1DM pancreatic islets is normalized following aerobic training; although differences in glucagon sensitivity have been reported. Drouin et al.Citation16 reported that exercise trained subjects demonstrate an increased hepatic sensitivity to glucagon, while Légaré et al.Citation17 reported an increased density of glucagon receptors in the liver of swim-trained rats. Further work is needed to better understand the impact of aerobic training in the restoration of pancreatic islet morphology, particularly in insulin-treated rats with T1DM.
Given the risks associated with exercise-induced hypoglycemia, studies examining blood glucose concentrations in response to exercise are acute in duration (a single bout of daily exercise).Citation23-Citation25 Our group recently demonstrated that exercise training of various modalities is beneficial in improving insulin sensitivity measures and insulin dosage requirements in rats with T1DM.Citation4 It is unknown if the increase in insulin sensitivity as a result of exercise may increase the risk of exercise-induced hypoglycemia, as exogenous insulin is a contributing factor in hypoglycemia development in response to exercise.Citation26,Citation27 It is unknown how acute reductions in blood glucose as a result of exercise may change following exercise training in populations with T1DM.
Using a model in which rats are moderately hyperglycemic and insulin-treated, the purpose of the present investigation was to: (1) determine the impact of a 10-wk aerobic training program on its ability to normalize pancreatic islet composition; (2) examine how the acute exercise-mediated reduction in blood glucose is altered over the course of exercise training. It was hypothesized that 10 wk of exercise training would lead to restoration of pancreatic insulin and glucagon content in rats with T1DM; however, it is hypothesized that exercise training will cause exercise-induced reductions in blood glucose to increase in magnitude.
Results
Animal weights and blood glucose
All rats gained weight following the completion of the 10-wk aerobic exercise training program (P < 0.05). C weighed significantly more than both D and DE (P < 0.05), while DE weighed significantly less than CE (P < 0.05; ). All rats began the experiment at a similar blood glucose concentration, while at the completion of the aerobic training program blood glucose concentrations of D and DE were significantly higher than both C and CE (P < 0.05). Aerobic exercise did not influence blood glucose concentrations in rats with and without T1DM (P > 0.05). The acute reduction in blood glucose concentration in response to a single bout of aerobic exercise was significantly different between CE and DE (; P < 0.05). DE demonstrated a significant reduction in blood glucose after each exercise bout, while CE demonstrated significant increases in blood glucose compared with pre-exercise (P < 0.05). These acute changes in post-exercise blood glucose were not altered over the course of 10 wk of exercise training in CE or DE rats (P > 0.05).
Table 1. Animal characteristics.
Figure 1. The change in blood glucose concentration from pre to post exercise. The change in blood glucose concentrations in response to exercise was significantly different between non-T1DM exercised rats (CE) and T1DM exercised rats (DE; P < 0.05). A significant increase in blood glucose was evident in response to exercise in CE rats while a significant decrease was evident in DE rats (P < 0.05). The change in blood glucose concentration following exercise was not significantly changed at any point during training in CE or DE rats (P > 0.05). Data are expressed means ± SE for each animal group.
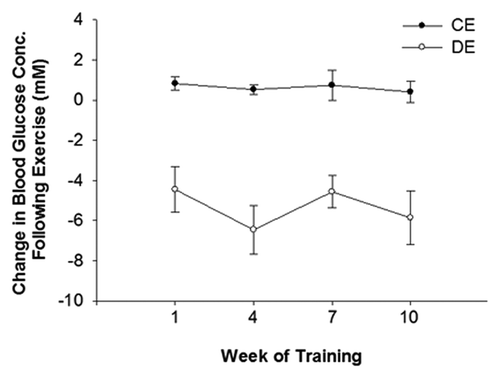
Pancreatic islet insulin and glucagon content
Pancreatic islet insulin content was significantly reduced in rats with T1DM compared to rats without (P < 0.05; ), while islet glucagon content was significantly increased in rats with T1DM compared to rats without (P < 0.05; ). The aerobic exercise program did not significantly alter insulin or glucagon content in rats with or without T1DM (P > 0.05).
Figure 2. Representative images of pancreatic islet insulin staining (A) and insulin quantification (B). Pancreatic islets from both sedentary and exercised rats with T1DM (D and DE) had significantly less insulin staining than sedentary and exercised non-T1DM (C and CE) rats (P < 0.05). (*) indicates a significant difference from C. (#) indicates a significant difference from CE (P < 0.05). Data are expressed as means ± SE for each animal group. (x40 magnification; Bar = 50 µm)
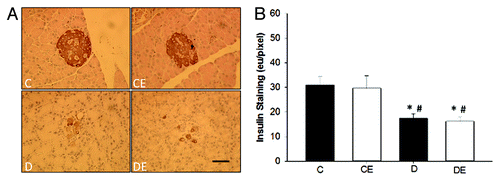
Figure 3. Representative images of pancreatic islet glucagon staining (A) and glucagon quantification (B). Pancreatic islets from both sedentary and exercised rats with T1DM (D and DE) had significantly more glucagon staining than sedentary and exercised non-T1DM (C and CE) rats (P < 0.05). (*) indicates a significant difference from C. (#) indicates a significant difference from CE (P < 0.05). Data are expressed as means ± SE for each animal group. (x40 magnification; Bar = 50 µm)
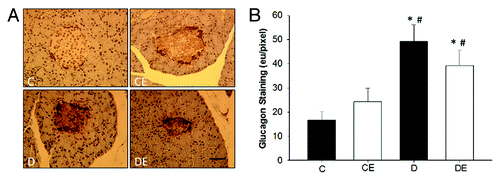
Comparison of pancreatic islets by size
T1DM led to a significant reduction in pancreatic islet size (P < 0.05), independent of aerobic training (P > 0.05; ). There was no significant three-way interaction for islet insulin or glucagon content between T1DM, aerobic training and islet size (P > 0.05). There was a main effect of islet size on hormone content, as smaller islets had significantly more insulin and glucagon than large islets (P < 0.05; ). Large islets had significantly more Ki67 positive cells than small islets based on the three-way ANOVA (P < 0.05; ). Further, there was a main effect of diabetes and exercise (P < 0.05). However, there were no significant interactions for Ki67 positive cells and islet size, diabetes or exercise (P > 0.05).
Figure 4. Pancreatic islet area. Pancreatic islets from both sedentary and exercised rats with T1DM (D and DE) were significantly smaller than sedentary and exercised non-T1DM (C and CE) rats (P < 0.05). (*) indicates a significant difference from C. (#) indicates a significant difference from CE (P < 0.05). Data are expressed as means ± SE for each animal group.
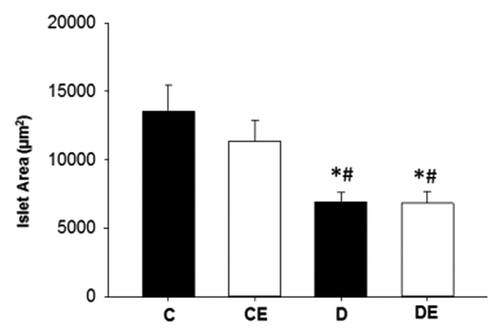
Figure 5. Pancreatic islet insulin (A) and glucagon (B) staining by diameter (small islets < 50 µm). Small islets had significantly more insulin and glucagon staining than larger islets. (*) indicates a significant difference (P < 0.05). Data are expressed as means ± SE for each animal group. (C: non-T1DM sedentary; CE; non-T1DM exercised; D: T1DM sedentary; DE: T1DM exercised)
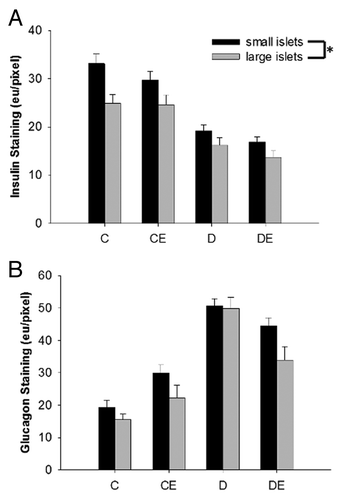
Figure 6. Representative Ki67 stain (A) and number of Ki67 positive cells per islet (B) based on islet diameter (small islets < 50 µm). Large islets had significantly more Ki67 positive cells (P < 0.05). (*) indicates a significant main effect of islet size. Data are expressed as means ± SE for each animal group. (C: non-T1DM sedentary; CE; non-T1DM exercised; D: T1DM sedentary; DE: T1DM exercised). (x40 magnification; Bar = 50µm)
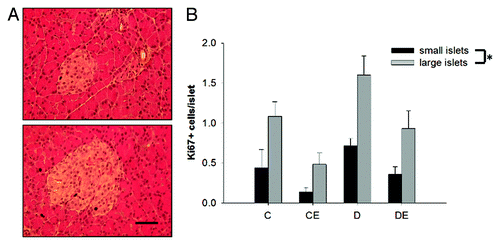
GLUT4 and insulin receptor protein content
Exercise training led to a significant increase in GLUT4 protein content in rats with T1DM (P < 0.05; ). There were no significant differences in insulin receptor protein content across experimental groups (P > 0.05).
Figure 7. GLUT4 protein content in the red vastus muscle (A) and representative blot (B). Insulin receptor protein content in the red vastus muscle (C) and representative blot (D). Exercise training resulted in a significant increase in GLUT4 protein content in rats with T1DM. No change in insulin receptor protein content was evident across experimental groups. (*) indicates a significant difference compared with D. Data are expressed as means ± SE for each animal group. (C: non-T1DM sedentary; CE; non-T1DM exercised; D: T1DM sedentary; DE: T1DM exercised)
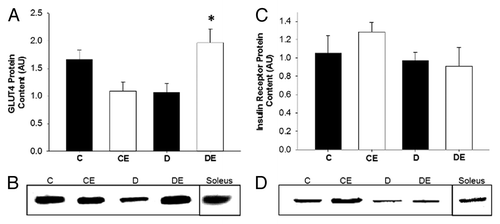
Discussion
While exercise training has been shown to increase insulin sensitivity in patients with T1DM, it is unknown if this is accompanied by increased islet insulin content. Coskun et al.Citation19 determined via immunochemistry that aerobic training has positive effects on pancreatic β-cells during the induction phase of T1DM through STZ injections. Further, it has been reported that following 6 wk of free-wheel training, STZ-induced mice with T1DM demonstrated elevated levels of islet insulin staining, as well as basal insulin release from isolated islets.Citation18 Contrary to these findings, the present investigation found that 10 wk of aerobic training failed to significantly alter insulin content in pancreatic islets. A possible explanation for these discrepancies might be the time at which the exercise regime was initiated. In the study by Coskun et al.,Citation19 treadmill exercise was initiated prior to, and during STZ administration, while free-wheel exercise in the Huang et al.Citation18 study took place just 3 d following STZ administration. Indeed, similar to the current study, Howarth et al.Citation28 found that treadmill exercise initiated one week after the last STZ injection had no effect on the distribution of insulin-positive cells within islets. These findings may suggest that aerobic exercise can have a positive influence on islet insulin content if exercise is initiated early in the course of T1DM development. Further, the role of exercise may be better suited to alleviate β-cell degradation during disease onset, rather than reversal of insulin loss after T1DM has progressed.Citation19
The current study also significantly differs from others with regard to the blood glucose level at which rats with TIDM were maintained. In the present study, both exercise-trained and sedentary rats with T1DM were treated with subcutaneous insulin pellets in order to maintain blood glucose in a moderately hyperglycemic range (9–15 mM). This range is within that typically observed for patients with T1DM treated with conventional insulin therapy.Citation1 Further, individuals with T1DM are typically educated to refrain from exercise when blood glucose concentrations are greater than 14mM, as elevated blood glucose likely implies elevated ketones, which can become detrimental and exacerbated by exercise.Citation29 It is suggested that individuals with T1DM begin an exercise bout when blood glucose concentrations are between 5–13.9mM,Citation29 however, most studies utilize animal models of T1DM in the absence of insulin supplementation and are severely hyperglycemic. In contrary to Coskun et al.,Citation19 in the current investigation rats with T1DM were insulin-treated and had similar blood glucose levels in both sedentary and trained groups. In this respect, exercise training may not have increased islet insulin content in the Coskun et al.Citation19 study, but rather sedentary diabetic rats experienced larger insulin degradation as a result of their higher glycemic level. Indeed, prolonged exposure of isolated islets to high glucose concentrations greatly reduces islet insulin content.Citation30
It is well-recognized that T1DM is associated with an insulin deficiency within pancreatic islets; however, there is still uncertainty whether islet glucagon content may change over the course of diabetes. Some evidence has suggested there is little change in islet α-cells and glucagon content in both genetically-induced Biobreeder (BB) rats and chemically-induced STZ rats,Citation31 while others have shown significant increases in islet glucagon.Citation15,Citation18,Citation28,Citation32 Consistent with the latter studies, we demonstrate that rats with T1DM had significantly more glucagon content per islet than rats without T1DM. The potential role of increased islet glucagon content in exacerbating hyperglycemia has historical context. In 1975, Unger and OrciCitation33 proposed the bihormonal-hypothesis which suggests that chronic hyperglycemia in T1DM is due to an insulin deficiency combined with an excess of circulating glucagon. T1DM is typically associated with postprandial hyperglucagonemia as the disease progresses, which may be reflected by the increased pancreatic glucagon content demonstrated in the present study.Citation14 Elevated levels of glucagon as T1DM progresses has been suggested as a potential therapeutic target in the early course of T1DM.Citation14 As such, the potential impact aerobic training may have in reducing pancreatic islet glucagon content and subsequently, hyperglucagonemia, may prove useful as a therapeutic strategy.
Exercise training has been shown to increase glucagon sensitivity through changes in glucagon receptor density and increased hepatic glucose production in response to a glucagon bolus.Citation16,Citation17 Despite these changes, it is unknown how glucagon content within pancreatic islets may change following exercise training. In the current study the aerobic training program did not alter glucagon content in the pancreatic islets of rats with T1DM. While these results were surprising, they may suggest that islet hormone content is not the primary determinant governing its release and sensitivity; rather, neural or hormonal factors regulating islet hormone release may be of greater importance. This is supported by the fact that extremely low amounts of glucagon producing α-cells are still capable of maintaining glycemic control.Citation34 Further, in comparison to rats without diabetes, STZ-induced T1DM animals exhibit a 2-fold greater glucagon response to epinephrine, which is returned to normal levels following 10 wk of aerobic training.Citation35 Interestingly, this training-mediated alteration in glucagon release was also not explained by islet glucagon or insulin content, and similarly to the current findings, islet hormone content remained unchanged as a result of the training program.Citation35
Exercise-induced reductions in blood glucose occur frequently in individuals with T1DM.Citation6 Similarly, in the present study rats with T1DM frequently experienced significant exercise-induced reductions in blood glucose while rats without T1DM experienced a rise in blood glucose concentrations. The different blood glucose responses to exercise between rats with and without T1DM likely occurred due to the inability of rats with T1DM to inhibit blood insulin concentrations concomitant with increased insulin sensitivity as a result of the exercise.Citation36,Citation37 The normal physiological response to exercise is to decrease the insulin to glucagon ratio, therefore promoting glucose production.Citation11 However, similar to the exercising population with T1DM, rats with T1DM were unable to reduce exogenous insulin in circulation and thus blood glucose levels fell.
A significant portion of individuals with T1DM have difficulty regulating insulin dosage and suffer from insulin resistance. While it is unclear as to the mechanisms by which individuals with T1DM develop insulin resistance, they are disassociated from mechanisms related to Type 2 diabetes mellitus (T2DM).Citation38 As a consequence, insulin overcorrection is an unavoidable contributing factor to most hypoglycemic episodes.Citation2 Further, insulin therapy is also problematic during glucose demanding states whereby circulating insulin levels must be intensely regulated (i.e. exercise).Citation26,Citation27 Exogenous insulin can potentiate the glucose demand of exercise and thus, increase blood glucose clearance. Our laboratory has shown that exercise training, regardless of modality, results in an increase in insulin sensitivity, demonstrated by reduced exogenous insulin requirements, increased glucose clearance during a glucose tolerance test, as well as alterations in GLUT4 protein conent within skeletal muscle.Citation4,Citation5 Exercise has also been shown to increase subcutaneous insulin absorption, which can lead to greater insulin-induced reductions in blood glucose.Citation27 As such, it was hypothesized that concomitant with the expected increase in insulin sensitivity from exercise training, the reduction in blood glucose in response to an exercise bout would become larger in depth, ultimately increasing the frequency of exercise-induced hypoglycemia. Contrary to our hypothesis, the reduction in blood glucose which occurred following an exercise bout remained the same throughout 10 wk of training. This exercise-induced reduction in blood glucose remained the same despite elevations in GLUT4 being evident following exercise training in rats with T1DM. The increase in GLUT4 protein content is likely even larger than shown in the current study, as translocation of GLUT4 to the cell membrane is transient and is typically greatly reduced by 48 h post-exercise.Citation39 A possible explanation for this finding is that increases in glucagon sensitivity likely occurred concurrently with increased insulin sensitivity. Indeed, it has been shown that aerobic training can increase the response of gluconeogenesis and glycogenolysis to glucagon.Citation16,Citation40 This expected increase likely attenuated significant reductions in blood glucose, as glucagon itself accounts for more than 60% of glucose production during exercise.Citation11,Citation41 In summary, these results and the findings of others suggest that regulation of blood glucose post-exercise is multi-faceted and that several factors must be considered when attempting to improve the metabolic response to exercise in a patient with T1DM.
While aerobic training was unable to alter pancreatic islet hormone content in rats with T1DM, evidence exists which suggests that pancreatic islets respond differently based on their size.Citation42-Citation44 Early reports suggested that the bulk of islet endocrine function came from larger islets while small islets had little functional importance.Citation45 However, to date this view has been challenged by evidence suggesting that small islets contain more insulin, are superior in insulin secretion, and have better transplantation outcomes compared with large islets.Citation42-Citation44 The majority of this work cites larger increases in C-peptide and insulin secretion from small islets in isolated islet preparations; therefore, it was important to explore different islet subpopulations in an in vivo setting.Citation42-Citation44 Results from the present study support the view that small islets (<50 µm in diameter) from rats with and without T1DM have significantly more insulin content than larger islets. Huang et al.Citation42 also observed in vivo through immunochemistry that small islets have more insulin content than larger islets in rats without T1DM; however, until now this observation had not been demonstrated in T1DM pancreatic islets. Less information is available as to the distribution of glucagon with respect to islet size. MacGregor et al.Citation44 observed that glucagon content within small and large islets was distributed similarly between both subpopulations. However, to our knowledge, this is the first experiment to demonstrate that glucagon content is higher in small islets of rats with and without T1DM. Ultimately, the differences in the amount of insulin and glucagon content within different sizes of pancreatic islets supports the view that different subpopulations of islets exist. Further, this suggests that the significant increase in glucagon content within the pancreatic islets of rats with T1DM may exist due the presence of more small islets than large. In order to determine if the increase in islet hormone content in small islets occurred as a result of increased cell proliferation the number of Ki67 positive cells in different cell populations were examined. While Ki67 positive cells were significantly higher in large islets, cell proliferation was likely occurring at the same rate, as proliferating cells within most islets were minimal. Despite cell proliferation not occurring at a high rate, islets may be undergoing dedifferentiation or hypertrophy. Indeed, others have shown that small islets consistently had a higher cell density when compared with larger islets.Citation42 Further work is required to determine the implications of islet size on both insulin and glucagon release, although it does not appear that aerobic training is capable of increasing islet hormone content in small or large islets. To date, studies examining alterations in islet hormone content following aerobic training were conducted in T1DM animals without insulin supplementation, an environment not representative of human populations with T1DM. Using a conventional insulin therapy model, the present investigation determined whether 10 wk of aerobic training could restore the pancreatic islet hormone composition of rats with T1DM to similar levels as rats without T1DM. It is concluded that 10 wk of exercise training was unable to improve insulin and glucagon content in T1DM pancreatic islets. Further, the current study supports the view that different islet subpopulations, based on size, may exist. Although no islet composition changes were evident following exercise training, the magnitude of exercise-induced glucose reductions remained constant across the 10 wk.
Materials and Methods
Ethics approval and animals
This study was approved by the Research Ethics Board of the University of Western Ontario in accordance with the guidelines of the Canadian Council on Animal Care. Eight-week old, male Sprague-Dawley rats were obtained from Charles River Laboratories, housed in standard rat cages and maintained on a 12-h light/dark cycle at a constant temperature and humidity. Rats were allowed access to standard rat chow and water ad libitum. Twenty-two rats were randomly assigned to either non-T1DM sedentary control (C; n = 5), non-T1DM exercised control (CE; n = 5), T1DM sedentary (D; n = 6), T1DM exercised (DE; n = 6).
Diabetes induction
A multiple low-dose STZ-induced T1DM model was employed. T1DM was induced by intraperitoneal injection of STZ (20mg/kg; Sigma-Aldrich) dissolved in citrate buffer (0.1 M, pH 4.5) on 5 consecutive days. This multiple low-dose model of T1DM has been established to elicit immune-mediated destruction of pancreatic β-cells similar to the normal pathogenesis of a patient with T1DM.Citation5,Citation46 All injections were sterile, filtered (0.2 µm), and occurred within 5 min of STZ being dissolved in the citrate buffer. Diabetes was confirmed following 2 consecutive blood glucose concentrations of >18mM. Following T1DM confirmation, an insulin pellet (1 pellet; 2U insulin/day; Linplant, Linshin Canada Inc.) was implanted subcutaneously in the abdomen. Blood glucose concentrations were closely monitored throughout the study (see below) and insulin pellets were adjusted to maintain blood glucose within a moderate glycemic range (9–15mM).
Exercise protocol
Animals were gradually introduced to the exercise training program with familiarization to the treadmill on two separate occasions (15m/min, 2% grade for 15 min). Subsequently, treadmill running occurred for one hour each day at a 2% grade, 5 d a week for a total of 10 wk. The 10 wk of treadmill training consisted of 17m/min (week 1), 24m/min (week 2), and subsequently 27m/min for the completion of the study (week 3–10). Treadmill running at 27m/min represents approximately 70–80% of a STZ-induced diabetic rat’s VO2 max.Citation47 Continuous running was encouraged by compressed air or tactile stimulus when rats reached the back of the treadmill and broke a photoelectric beam.
Blood glucose concentrations
Non-fasting blood glucose concentrations were obtained from the saphenous vein at the beginning and following exercise training using a Freestyle Lite Blood Glucose Monitoring System (Abbott Diabetes Inc.). Blood glucose concentrations were also recorded prior to, and immediately following an acute bout of treadmill training, during weeks 1, 4, 7 and 10 of the aerobic training period.
Tissue collection and Immunohistochemistry
Pancreatic tissue was extracted from animals 4 d following the last exercise bout. Pancreata were fixed in 10% normal buffered formalin for 72 h at 4 °C and placed in ethanol prior to tissue processing and paraffinization. Paraffin embedded tissue was sectioned at 5 μm and mounted on positively charged slides (Fischer Scientific Superfrost Plus #12-550-15).
Immunohistochemical staining was conducted using the avidin-biotin complex technique. Paraffin embedded pancreata were deparaffinized and hydrated in xylene and graded ethanol solutions. Following deparaffinization, pancreata were subjected to heat-induced epitope retrieval (antigen retrieval buffer: 10mM sodium citrate buffer, pH 6, 0.05% Tween-20). Slides were then quenched in a 3% hydrogen peroxide solution for 20 min, washed in phosphate buffered saline (PBS) for 5 min and blocked in 10% goat serum overnight at 4 °C. All antibodies were diluted with 1% goat serum and incubated for one hour at room temperature: insulin (1:100; Dako: A0564), glucagon (1:4000; abcam: k79bB10) and Ki67 (1:100; abcam: ab16667). Corresponding biotinylated secondary antibodies were diluted in 1% goat serum (1:200, Vector Laboratories Inc.) and incubated for 1 h at room temperature. Sections were then treated with Vectastain solution (Vectastain ABC Elite Kit, PK-6100 Vector Laboratories Inc.) for 1 h at room temperature prior to being exposed to diaminobenzidine (DAB; Vector Laboratories, SK-4100) for 7 min. Tissues were then counterstained with hematoxylin (Sigma-Alderich hematoxylin solution: GHS-3).
Immunohistochemistry quantification
Insulin and glucagon within pancreatic islets were examined by measuring the cumulative signal strength of images, a quantitative immunochemistry technique established by Matkowskyj and colleagues.Citation48,Citation49 Approximately 30 islets from each animal were visualized using a Zeiss Axiovert S100 inverted microscope in combination with a Sony PowerHAD (DXC950–3CCD color) camera. For each islet analyzed, two serial secretions of pancreata within 5 µm were used, with only one section being exposed to primary antibody. In order to account for possible visual differences in exocrine tissue between stained and unstained images, and to make backgrounds more homogenous, each islet was placed on a black background. A black pixel is represented by a zero in each of the 3 (red, blue, green) color channels. All islets were cropped using Photoshop (Adobe Photoshop CS6), and consistent with Matkowskyj and colleagues,Citation48,Citation49 the amount of ‘energy units (eu)’ from the image of a non-stained islet was subtracted from the eu of the same stained islet using Matlab (Mathworks, Version R2009a). Differences in islet size were accounted for by dividing eu by the number of pixels making up each individual islet. Ki67 positive cells within pancreatic islets were counted by two observers blind to experimental conditions. Islet area and diameter was determined for each individual cropped islet with the mean islet diameter in the current study being ~50 µm. Small islets were classified as having a diameter of 50 µm or less while large islets had a diameter of greater than 50 µm.
Western blotting
Red vastus muscle (100 mg) was homogenized in a 1:10 ratio of homogenizing buffer (100 mmol/L NaCl, 50 mmol/L Tris base, 0.1 mmol/L EDTA, 0.1 mmol/L EGTA and 1% Triton X-100, adjusted to pH ~7.5%, 1% phosphatase inhibitor and 1% protease inhibitor) and protein concentrations were determined using the Bradford protein method.Citation50 Homogenates were mixed with equal volumes of sample buffer (0.5 M Tris base, 13% glycerol, 0.5% SDS, 13% β-mercaptoethanol and bromophenol blue) and separated according to their molecular weight on 10% acrylamide separating gel and 4% acrylamide stacking gel. Each gel contained a molecular weight marker (Bio-Rad: catalog no. 161-0373) to determine the molecular weight of the proteins as well as a soleus muscle, which served as a positive control to permit the standardization of each band across the various gels. After electrophoresis, proteins were transferred to nitrocellulose membranes and blocked in 5% milk blotto (BioRad 170-6404) in Tris-buffered saline (TBS; 10 mmol/L Tris, 100 mmol/L NaCl, 0.1% Tween-20, pH 7.5) for 2 h. Membranes were incubated with primary antibodies specific to GLUT4 (rabbit mAb anti GLUT4, Cell Signaling 2213, 1:1000) and insulin receptor (rabbit mAb anti insulin receptor β, Cell Signaling 3025, 1:1000) in TTBS (0.1% Tween 20 and TBS) with 2% milk blotto. Membranes were then washed and incubated for 1 h with horseradish peroxidase-conjugated secondary antibody specific for rabbit IgG (goat anti-rabbit IgG-HRP conjugate, BioRad, 1:5000) diluted in TTBS with 2% non-fat dry milk. Following 3 washes in TTBS for 10 min membranes were developed using chemiluminescent substrate (BioRad Western C Enhanced Chemiluminescent Kit, 170-5070). Protein bands were captured using a BioRad Chemidoc XRS imager and optical densities were quantified using BioRad Quantity One software (#170-9600 Bio-Rad). Optical densities were normalized to the soleus muscle positive control on each blot.
Statistical analysis
Body weight, blood glucose concentration, GLUT4 and insulin receptor protein content, islet hormone content (insulin and glucagon) and islet area were compared using a two-way ANOVA. Blood glucose concentrations in response to aerobic exercise were analyzed using a two-way repeated measures ANOVA. Insulin and glucagon content, as well as Ki67 positive cells based on islet size were compared using a three-way ANOVA. A Tukey post hoc test was performed with a significance level set at P < 0.05. All data are expressed as mean ± SE and statistical analysis was performed using SigmaPlot 11 statistical software.
Abbreviations: | ||
ANOVA | = | analysis of variance |
BB | = | biobreeder |
C | = | non-T1DM sedentary control |
CE | = | non-T1DM exercised control |
DAB | = | 3,3′-diaminobenzidine |
DI | = | T1DM sedentary |
DIE | = | T1DM exercised |
eu | = | energy units |
GLUT4 | = | glucose transporter 4 |
IGF-1 | = | insulin-like growth factor-1 |
IR | = | insulin receptor |
PBS | = | phosphate buffered saline |
SE | = | standard error |
STZ | = | streptozotocin |
T1DM | = | Type 1 diabetes mellitus |
T2DM | = | Type 2 diabetes mellitus |
Disclosure of Potential Conflicts of Interest
No potential conflicts of interest were disclosed.
Financial Support
This study was supported by Canadian Institute of Health Research Grant (CCT-83029).
Author Contributions
M.W.M. contributed to data analysis, interpretation of the data, writing and revision of manuscript; M.R.M. contributed to data analysis, interpretation of the data, revision of manuscript; K.E.H. contributed to data analysis; E.G.N. contributed to the design and implementation of the project, interpretation of the data, and revision of manuscript; C.W.J.M. contributed to design and implementation of the project, interpretation of the data, writing and revision of manuscript.
References
- The Diabetes Control and Complications Trial Research Group. The effect of intensive treatment of diabetes on the development and progression of long-term complications in insulin-dependent diabetes mellitus. N Engl J Med 1993; 329:977 - 86; http://dx.doi.org/10.1056/NEJM199309303291401; PMID: 8366922
- The Diabetes Control and Complications Trial Research Group. Hypoglycemia in the Diabetes Control and Complications Trial. Diabetes 1997; 46:271 - 86; http://dx.doi.org/10.2337/diab.46.2.271; PMID: 9000705
- Khaw KT, Wareham N, Bingham S, Luben R, Welch A, Day N. Association of hemoglobin A1c with cardiovascular disease and mortality in adults: the European prospective investigation into cancer in Norfolk. Ann Intern Med 2004; 141:413 - 20; http://dx.doi.org/10.7326/0003-4819-141-6-200409210-00006; PMID: 15381514
- Hall KE, McDonald MW, Grisé KN, Campos OA, Noble EG, Melling CW. The role of resistance and aerobic exercise training on insulin sensitivity measures in STZ-induced Type 1 diabetic rodents. Metabolism 2013; 62:1485 - 94; http://dx.doi.org/10.1016/j.metabol.2013.05.012; PMID: 23810201
- Melling CWJ, Grisé KN, Hasilo CP, Fier B, Milne KJ, Karmazyn M, Noble EG. A model of poorly controlled type 1 Diabetes Mellitus and its treatment with aerobic exercise training. Diabetes Metab 2013; 39:226 - 35; http://dx.doi.org/10.1016/j.diabet.2013.02.004; PMID: 23522732
- Bhatia V, Wolfsdorf JI. Severe hypoglycemia in youth with insulin-dependent diabetes mellitus: frequency and causative factors. Pediatrics 1991; 88:1187 - 93; PMID: 1956736
- McMahon SK, Ferreira LD, Ratnam N, Davey RJ, Youngs LM, Davis EA, Fournier PA, Jones TW. Glucose requirements to maintain euglycemia after moderate-intensity afternoon exercise in adolescents with type 1 diabetes are increased in a biphasic manner. J Clin Endocrinol Metab 2007; 92:963 - 8; http://dx.doi.org/10.1210/jc.2006-2263; PMID: 17118993
- Marliss EB, Vranic M. Intense exercise has unique effects on both insulin release and its roles in glucoregulation: implications for diabetes. Diabetes 2002; 51:Suppl 1 S271 - 83; http://dx.doi.org/10.2337/diabetes.51.2007.S271; PMID: 11815492
- Cherrington AD, Williams PE, Shulman GI, Lacy WW. Differential time course of glucagon’s effect on glycogenolysis and gluconeogenesis in the conscious dog. Diabetes 1981; 30:180 - 7; http://dx.doi.org/10.2337/diab.30.3.180; PMID: 6110598
- Cryer PE, Gerich JE. Relevance of glucose counterregulatory systems to patients with diabetes: critical roles of glucagon and epinephrine. Diabetes Care 1983; 6:95 - 9; http://dx.doi.org/10.2337/diacare.6.1.95; PMID: 6341018
- Wasserman DH, Cherrington AD. Hepatic fuel metabolism during muscular work: role and regulation. Am J Physiol 1991; 260:E811 - 24; PMID: 2058658
- Gerich JE, Langlois M, Noacco C, Karam JH, Forsham PH. Lack of glucagon response to hypoglycemia in diabetes: evidence for an intrinsic pancreatic alpha cell defect. Science 1973; 182:171 - 3; http://dx.doi.org/10.1126/science.182.4108.171; PMID: 4581053
- Orskov L, Alberti KGMM, Mengel A, Møller N, Pedersen O, Rasmussen O, Seefeldt T, Schmitz O. Decreased hepatic glucagon responses in type 1 (insulin-dependent) diabetes mellitus. Diabetologia 1991; 34:521 - 6; http://dx.doi.org/10.1007/BF00403290; PMID: 1916058
- Brown RJ, Sinaii N, Rother KI. Too much glucagon, too little insulin: time course of pancreatic islet dysfunction in new-onset type 1 diabetes. Diabetes Care 2008; 31:1403 - 4; http://dx.doi.org/10.2337/dc08-0575; PMID: 18594062
- Li Z, Karlsson FA, Sandler S. Islet loss and alpha cell expansion in type 1 diabetes induced by multiple low-dose streptozotocin administration in mice. J Endocrinol 2000; 165:93 - 9; http://dx.doi.org/10.1677/joe.0.1650093; PMID: 10750039
- Drouin R, Lavoie C, Bourque J, Ducros F, Poisson D, Chiasson JL. Increased hepatic glucose production response to glucagon in trained subjects. Am J Physiol 1998; 274:E23 - 8; PMID: 9458743
- Légaré A, Drouin R, Milot M, Massicotte D, Péronnet F, Massicotte G, Lavoie C. Increased density of glucagon receptors in liver from endurance-trained rats. Am J Physiol Endocrinol Metab 2001; 280:E193 - 6; PMID: 11120674
- Huang H-H, Farmer K, Windscheffel J, Yost K, Power M, Wright DE, Stehno-Bittel L. Exercise increases insulin content and basal secretion in pancreatic islets in type 1 diabetic mice. Exp Diabetes Res 2011; 2011:481427; http://dx.doi.org/10.1155/2011/481427; PMID: 21912535
- Coskun O, Ocakci A, Bayraktaroglu T, Kanter M. Exercise training prevents and protects streptozotocin-induced oxidative stress and beta-cell damage in rat pancreas. Tohoku J Exp Med 2004; 203:145 - 54; http://dx.doi.org/10.1620/tjem.203.145; PMID: 15240923
- The DCCT Research Group. Epidemiology of severe hypoglycemia in the diabetes control and complications trial. Am J Med 1991; 90:450 - 9; http://dx.doi.org/10.1016/0002-9343(91)80085-Z; PMID: 2012085
- Choi SB, Jang JS, Hong SM, Jun DW, Park S. Exercise and dexamethasone oppositely modulate beta-cell function and survival via independent pathways in 90% pancreatectomized rats. J Endocrinol 2006; 190:471 - 82; http://dx.doi.org/10.1677/joe.1.06400; PMID: 16899580
- Calegari VC, Abrantes JL, Silveira LR, Paula FM, Costa JM Jr., Rafacho A, Velloso LA, Carneiro EM, Bosqueiro JR, Boschero AC, et al. Endurance training stimulates growth and survival pathways and the redox balance in rat pancreatic islets. J Appl Physiol (1985) 2012; 112:711 - 8; http://dx.doi.org/10.1152/japplphysiol.00318.2011; PMID: 22174407
- Iscoe KE, Riddell MC. Continuous moderate-intensity exercise with or without intermittent high-intensity work: effects on acute and late glycaemia in athletes with Type 1 diabetes mellitus. Diabet Med 2011; 28:824 - 32; http://dx.doi.org/10.1111/j.1464-5491.2011.03274.x; PMID: 21388440
- Yardley JE, Kenny GP, Perkins BA, Riddell MC, Balaa N, Malcolm J, Boulay P, Khandwala F, Sigal RJ. Resistance versus aerobic exercise: acute effects on glycemia in type 1 diabetes. Diabetes Care 2013; 36:537 - 42; http://dx.doi.org/10.2337/dc12-0963; PMID: 23172972
- Adams OP. The impact of brief high-intensity exercise on blood glucose levels. Diabetes Metab Syndr Obes 2013; 6:113 - 22; http://dx.doi.org/10.2147/DMSO.S29222; PMID: 23467903
- Briscoe VJ, Tate DB, Davis SN. Type 1 diabetes: exercise and hypoglycemia. Appl Physiol Nutr Metab 2007; 32:576 - 82; http://dx.doi.org/10.1139/H07-025; PMID: 17510699
- Dandona P, Hooke D, Bell J. Exercise and insulin absorption from subcutaneous tissue. Br Med J 1978; 1:479 - 80; http://dx.doi.org/10.1136/bmj.1.6111.479-a; PMID: 626843
- Howarth FC, Marzouqi FMA, Al Saeedi AMS, Hameed RS, Adeghate E. The effect of a heavy exercise program on the distribution of pancreatic hormones in the streptozotocin-induced diabetic rat. JOP 2009; 10:485 - 91; PMID: 19734622
- Riddell MC, Perkins BA. Type 1 diabetes and vigorous exercise: applications of exercise physiology to patient management. Can J Diabetes 2006; 30:63 - 71; http://dx.doi.org/10.1016/S1499-2671(06)01010-0
- Eizirik DL, Korbutt GS, Hellerström C. Prolonged exposure of human pancreatic islets to high glucose concentrations in vitro impairs the beta-cell function. J Clin Invest 1992; 90:1263 - 8; http://dx.doi.org/10.1172/JCI115989; PMID: 1401063
- Papaccio G, Mezzogiorno V. Morphological aspects of glucagon and somatostatin islet cells in diabetic bio breeding and low-dose streptozocin-treated Wistar rats. Pancreas 1989; 4:289 - 94; http://dx.doi.org/10.1097/00006676-198906000-00003; PMID: 2567512
- Rastogi KS, Cooper RL, Shi ZQ, Vranic M. Quantitative measurement of islet glucagon response to hypoglycemia by confocal fluorescence imaging in diabetic rats: effects of phlorizin treatment. Endocrine 1997; 7:367 - 75; http://dx.doi.org/10.1007/BF02801332; PMID: 9657075
- Unger RH, Orci L. The essential role of glucagon in the pathogenesis of diabetes mellitus. Lancet 1975; 1:14 - 6; http://dx.doi.org/10.1016/S0140-6736(75)92375-2; PMID: 46337
- Thorel F, Damond N, Chera S, Wiederkehr A, Thorens B, Meda P, Wollheim CB, Herrera PL. Normal glucagon signaling and β-cell function after near-total α-cell ablation in adult mice. Diabetes 2011; 60:2872 - 82; http://dx.doi.org/10.2337/db11-0876; PMID: 21926270
- Nadeau A, Rousseau-Migneron S, Tancrède G, Jobidon C, Trudel D. Diminished glucagon response to epinephrine in physically trained diabetic rats. Diabetes 1985; 34:1278 - 82; http://dx.doi.org/10.2337/diab.34.12.1278; PMID: 3905462
- Younk LM, Mikeladze M, Tate D, Davis SN. Exercise-related hypoglycemia in diabetes mellitus. Expert Rev Endocrinol Metab 2011; 6:93 - 108; http://dx.doi.org/10.1586/eem.10.78; PMID: 21339838
- Slentz CA, Gulve EA, Rodnick KJ, Henriksen EJ, Youn JH, Holloszy JO. Glucose transporters and maximal transport are increased in endurance-trained rat soleus. J Appl Physiol (1985) 1992; 73:486 - 92; PMID: 1399970
- Bergman BC, Howard D, Schauer IE, Maahs DM, Snell-Bergeon JK, Eckel RH, Perreault L, Rewers M. Features of hepatic and skeletal muscle insulin resistance unique to type 1 diabetes. J Clin Endocrinol Metab 2012; 97:1663 - 72; http://dx.doi.org/10.1210/jc.2011-3172; PMID: 22362823
- Etgen GJ Jr., Brozinick JT Jr., Kang HY, Ivy JL. Effects of exercise training on skeletal muscle glucose uptake and transport. Am J Physiol 1993; 264:C727 - 33; PMID: 8460676
- Drouin R, Robert G, Milot M, Massicotte D, Péronnet F, Lavoie C. Swim training increases glucose output from liver perfused in situ with glucagon in fed and fasted rats. Metabolism 2004; 53:1027 - 31; http://dx.doi.org/10.1016/j.metabol.2004.03.010; PMID: 15281013
- Wasserman DH, Lacy DB, Bracy DP. Relationship between arterial and portal vein immunoreactive glucagon during exercise. J Appl Physiol (1985) 1993; 75:724 - 9; PMID: 8226475
- Huang H-H, Novikova L, Williams SJ, Smirnova IV, Stehno-Bittel L. Low insulin content of large islet population is present in situ and in isolated islets. Islets 2011; 3:6 - 13; http://dx.doi.org/10.4161/isl.3.1.14132; PMID: 21325888
- Lehmann R, Zuellig RA, Kugelmeier P, Baenninger PB, Moritz W, Perren A, Clavien P-A, Weber M, Spinas GA. Superiority of small islets in human islet transplantation. Diabetes 2007; 56:594 - 603; http://dx.doi.org/10.2337/db06-0779; PMID: 17327426
- MacGregor RR, Williams SJ, Tong PY, Kover K, Moore WV, Stehno-Bittel L. Small rat islets are superior to large islets in in vitro function and in transplantation outcomes. Am J Physiol Endocrinol Metab 2006; 290:E771 - 9; http://dx.doi.org/10.1152/ajpendo.00097.2005; PMID: 16303846
- Kaihoh T, Masuda T, Sasano N, Takahashi T. The size and number of Langerhans islets correlated with their endocrine function: a morphometry on immunostained serial sections of adult human pancreases. Tohoku J Exp Med 1986; 149:1 - 10; http://dx.doi.org/10.1620/tjem.149.1; PMID: 3526638
- O’Brien BA, Harmon BV, Cameron DP, Allan DJ. Beta-cell apoptosis is responsible for the development of IDDM in the multiple low-dose streptozotocin model. J Pathol 1996; 178:176 - 81; http://dx.doi.org/10.1002/(SICI)1096-9896(199602)178:2<176::AID-PATH433>3.0.CO;2-8; PMID: 8683386
- Rodrigues B, Figueroa DM, Mostarda CT, Heeren MV, Irigoyen M-C, De Angelis K. Maximal exercise test is a useful method for physical capacity and oxygen consumption determination in streptozotocin-diabetic rats. Cardiovasc Diabetol 2007; 6:38; http://dx.doi.org/10.1186/1475-2840-6-38; PMID: 18078520
- Matkowskyj KA, Schonfeld D, Benya RV. Quantitative immunohistochemistry by measuring cumulative signal strength using commercially available software photoshop and matlab. J Histochem Cytochem 2000; 48:303 - 12; http://dx.doi.org/10.1177/002215540004800216; PMID: 10639497
- Matkowskyj KA, Cox R, Jensen RT, Benya RV. Quantitative immunohistochemistry by measuring cumulative signal strength accurately measures receptor number. J Histochem Cytochem 2003; 51:205 - 14; http://dx.doi.org/10.1177/002215540305100209; PMID: 12533529
- Bradford MM. A rapid and sensitive method for the quantitation of microgram quantities of protein utilizing the principle of protein-dye binding. Anal Biochem 1976; 72:248 - 54; http://dx.doi.org/10.1016/0003-2697(76)90527-3; PMID: 942051