Abstract
Understanding the mechanisms that regulate dynamic localization of a protein within a cell can provide critical insight to its functional molecular interactions. Signal transducers and activators of transcription (STATs) play essential roles in development, proliferation, and immune defense. However the consequences of STAT hyperactivity can predispose to diseases including autoimmunity and cancer. To function as transcription factors STATs must gain access to the nucleus, and knowledge of the mechanisms that regulate STAT nuclear trafficking can provide a means to control STAT action. This review presents a synopsis of some of the studies that address the nuclear dynamics of the STAT proteins. Evidence suggests that not all STATs are the same. Nuclear import of STAT1 and STAT4 appears linked to their tyrosine phosphorylation and the formation of parallel dimers via reciprocal phosphotyrosine and Src homology 2 domain interactions. This dimer arrangement generates a conformational nuclear localization signal. STAT2 is imported continually to the nucleus in an unphosphorylated state due to its association with IRF9, but the dominant nuclear export signal of STAT2 shuttles the complex back to the cytoplasm. Following STAT2 tyrosine phosphorylation, it can form dimers with STAT1 to affect nuclear import as the trimeric complex (ISGF3). Distinctly, STAT3, STAT5, and STAT6 are continually imported to the nucleus independent of tyrosine phosphorylation. Mutational studies indicate the nuclear localization signals in these STATs require the conformational structure of their coiled-coil domains. Increases in STAT nuclear accumulation following cytokine stimulation appear coordinate with their ability to bind DNA.
Introduction
Eukaryotic chromosomes are sequestered within the nuclear envelope where processes of DNA replication and transcription are precisely regulated.Citation1,Citation2 In addition to intimate association with chromatin, the nuclear envelope discriminates access of proteins and RNAs across its borders.Citation3 Once activated by tyrosine phosphorylation in response to diverse cytokine signals in the cytoplasm, STATs need to gain entrance to the nucleus to bind specific DNA targets and function as transcription factors.Citation4-Citation7 Evidence indicates that even unphosphorylated STATs can play a role in nuclear gene expression.Citation8 The induction of specific genes by the seven mammalian STATs results in discreet and critical biological effects on cellular differentiation, proliferation, and immunity. Either the loss of STAT activity or uncontrolled constitutive STAT activity can have devastating effects.Citation9-Citation13 One of the mechanisms regulating STAT nuclear function is the means by which STAT proteins enter and exit the nucleus. Understanding the distinguishing qualities of STAT nuclear trafficking may provide knowledge necessary to selectively enable or disable STAT signaling in health and disease.
Nuclear Traffic
Although the nuclear envelope serves to separate nucleus from cytoplasm, the two compartments retain close communication. The nuclear envelope is comprised of an inner and an outer nuclear membrane, the latter of which is contiguous with the endoplasmic reticulum of the cytoplasm.Citation1,Citation2,Citation14 The inner and outer nuclear membranes are connected by structures measuring about 100–150 nm in diameter known as nuclear pore complexes (NPCs) that span the nuclear envelope. It is the NPCs that allow passage of molecules between cytoplasm and nucleus.Citation15-Citation17 The NPC is a cylindrical complex with octagonal symmetry comprised of multiple copies of ~30 proteins, designated nucleoporins (Nups). A subset of Nups contain phenylalanine–glycine (FG) repeats and line the channel of the NPC; these FG-Nups are responsible for controlling the permeability of the channel. Molecules smaller than 40–60 kDa can passively diffuse through the NPC; however, larger molecules, such as the STATs, usually require facilitated transport. Facilitated transport is mediated by specific transport receptors that recognize cargo to be transported, and also interact with the FG-Nups of the NPC, disrupting the permeability barrier.Citation18-Citation21
Signal sequences in proteins determine their ability to be recognized by transport receptors and thereby transit the NPC.Citation22,Citation23 For nuclear import, large proteins must possess sequences or structures that serve as nuclear localization signals (NLSs). These signals can either function constitutively or conditionally based on signal masking, protein conformation, or protein modification. The best characterized NLSs consist of either a monopartite or bipartite stretch of basic amino acids rich in lysine and arginine. Similarly, proteins destined for export from the nucleus must also have an element called a nuclear export signal (NES) recognized by distinct receptors. A commonly described NES is hydrophobic and rich in leucine residues.
Transport receptors, primarily in the karyopherin-β family, mediate transit of cargo proteins through the NPC.Citation22-Citation26 Those that mediate import are often referred to as importins, and those that mediate export, as exportins. Their structure is composed of tandem HEAT motifs of two antiparallel α-helices and they can bind cargo directly, or indirectly using adaptor proteins such as importin-αs that interact directly with cargo. The karyopherin-βs have the ability to bind FG-Nups and GTP-bound Ran. Binding to Ran-GTP causes a conformational change in the karyopherin-β allowing either release of cargo (importin) or binding to cargo (exportin). The direction of transport is determined by a gradient of Ran-GTP, which is at high levels in the nucleus due to the presence of a Ran-guanine nucleotide exchange factor, and at low levels in the cytoplasm due to a Ran GTPase activating protein. When importin-β complexes enter the nucleus, importin-β binds Ran-GTP and releases its cargo. Conversely, exportins in the nucleus bind Ran-GTP in order to form complexes with cargo, and following entrance to the cytoplasm, they release cargo with GTP hydrolysis.
Nuclear import of proteins with a classical NLS is commonly mediated by an importin-α:importin-β1 heterodimer.Citation22,Citation27 Importin-α serves as an adaptor molecule by binding the NLS of cargo, and binding importin-β1 at its N-terminus.Citation28 There are six characterized human importin-αs that share sequence identity and similar structural features: an N-terminal importin-β1 binding domain followed by 10 tandem armadillo (ARM) repeats, each ARM consisting of three α-helices. Although some NLSs can be recognized by all of the importin-α proteins, evidence indicates distinct NLSs are recognized by specific importin-αs.Citation29
The Crm1 exportin is the karyopherin-β chaperone for nuclear export of proteins with short leucine-rich NESs.Citation30 Crm1 recognizes NES cargo in the nucleus in a ternary complex with Ran-GTP, and following transit through the NPC and Ran-GTP hydrolysis, it delivers cargo to the cytoplasm. A membrane-permeable antibiotic Leptomycin B (LMB) has been very useful in identifying the role of Crm1 in the export of many proteins.Citation31 LMB covalently modifies Crm1 at a cysteine residue so that export cargo cannot dock within this domain.
Seeing It with Green Fluorescent Protein
Immunofluorescence traditionally has been a method of choice to visualize the location of proteins within the cell. However, fixation and permeabilization can distort structural integrity, and cross-reaction of antibodies can lead to misinterpretation of results. The discovery and implementation of green fluorescent protein (GFP) as a genetic tag in conjunction with confocal microscopy has dramatically advanced our ability to visualize life.Citation32-Citation35 The GFP protein from the Aequorea victoria jellyfish is comprised of 238 aa that form a barrel structure with 11 β-sheets surrounding a central chromophore. Although GFP can form weak dimers, it can be engineered into monomers with a single aa change (A206K). Variants of GFP optimized for brightness, such as enhanced GFP (EGFP), or selected for different spectral qualities, have augmented approaches to molecular imaging. Following excitation, proteins tagged with GFP can be visualized directly in fixed or live cells with fluorescence microscopy using appropriate optical filters. Since GFP is a relatively large tag (~27 kDa), it is imperative that the tagged protein maintains biological function. In our studies with STAT proteins, a GFP tag located at the C-terminus does not interfere with tyrosine phosphorylation or DNA binding, whereas a GFP tag at the N-terminus can inhibit cytokine-mediated tyrosine phosphorylation.
STAT1: Localization Linked to Tyrosine Phosphorylation and DNA Binding
STAT1 is the founding member of the STAT family and is activated by tyrosine phosphorylation in response to all interferons (IFNs). It has a domain structure similar to other STATs with an N-terminus, a coiled-coil domain, a DNA binding domain (DBD), a Src homology 2 (SH2) domain, a tyrosine that is phosphorylated in response to cytokine, and a C-terminus that facilitates transcriptional induction ().Citation4,Citation5,Citation7 Following IFN binding to cell surface receptors, Janus kinases (JAKs) associated with receptor subunits are activated and phosphorylate the receptor on specific tyrosine residues. This leads to the recruitment of STAT1 via its SH2 domain to the proximity of the JAKs.Citation36 Tyrosine phosphorylation of STAT1 by JAKs promotes its ability to bind specific DNA targets.
Figure 1. Nuclear trafficking of STAT1 linked to tyrosine phosphorylation and DNA binding. Top: Linear diagram of STAT1 domains: coiled-coil (CC) domain, DNA-binding domain (DBD), Src homology domain 2 (SH2), and tyrosine 701 phosphorylated by Janus kinases (JAK) (pY). Bottom: U-STAT1 exists primarily as an anti-parallel dimer (bottom left). Blue image represents STAT1 with circle at N-terminus. Following tyrosine phosphorylation by Janus kinases (JAK), reciprocal pY and SH2 domain interactions between monomers generate parallel dimers (top left). STAT1 parallel dimers are recognized by importin-α5:importin-β1 and are imported to the nucleus. In the nucleus Ran-GTP binds importin-β1 and the complex releases STAT1 cargo (top right). STAT1 phosphorylated dimers bind DNA targets in responsive genes. Protein tyrosine phosphatases (PTPase) and PIAS proteins contribute to STAT1 dissociation from DNA, and the NES in the STAT1 DBD is recognized by the Crm1 exportin to effect nuclear export.
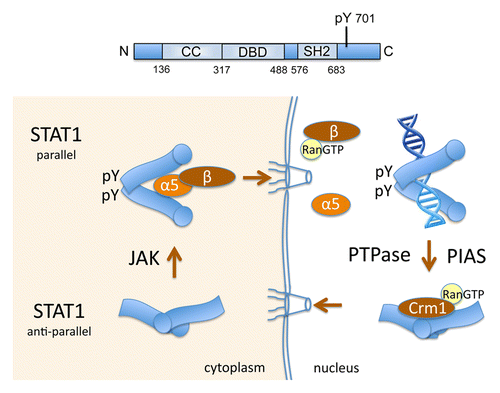
The crystal structure of tyrosine phosphorylated STAT1 bound to DNA has been solved.Citation37 It reveals a homodimer in which each monomer is associated with a half-site of the γIFN activated site (GAS), and the dimer is stabilized by reciprocal SH2 domain-phosphotyrosine 701 interactions between monomers. This conformation is now referred to as a “parallel” STAT dimer. The crystal structure of unphosphorylated STAT1 (U-STAT1) was also determined, and this too identified a homodimer, however with a distinct head-to-head orientation of each monomer.Citation38 This conformation is referred to as “anti-parallel” with the SH2 domains of each monomer at opposite extremities of the dimerCitation39 (). Tyrosine phosphorylation appears to stabilize the parallel form and promote specific DNA binding.
The use of GFP to tag STAT1 (STAT1-GFP) and follow its dynamic redistribution in the cell was first demonstrated in 1999.Citation40 STAT1-GFP localized primarily in the cytoplasm, and nuclear accumulation appeared within minutes after IFN-γ addition. However nuclear accumulation of STAT1-GFP was transient, and STAT1-GFP disappeared from the nucleus within hours after IFN. Protein synthesis and JAK inhibitors did not alter the relocalization of STAT1 to the cytoplasm, indicating that the long-lived STAT1 protein was actively exported and “recycled”.
STAT1: Export from the Nucleus
The transcriptional response to IFN is transient, with STAT1 disappearing from the nucleus within hours. Results indicate that the decrease of nuclear STAT1 is not a consequence of protein degradation, but correlates with its tyrosine dephosphorylation.Citation41,Citation42 An inhibitor of Crm1 exportin, LMB, inhibits export of nuclear STAT1-GFP, but does not delay STAT1 dephosphorylation.Citation43 A nuclear phosphatase has been subsequently identified that dephosphorylates STAT1: TC45, the nuclear isoform of the T-cell protein tyrosine phosphatase (TC-PTP).Citation44
The nuclear export of STAT1 is primarily mediated by the Crm1 exportin. Crm1 was shown to bind U-STAT1 in vitro dependent on a sequence in the DNA binding domain (DBD).Citation43 Crm1 binds to a consensus nuclear export signal (NES) containing a hydrophobic sequence 400–409 aa (LAAEFRHLQL) within the DBD of STAT1. The location of this STAT1 NES within the DBD suggested that the NES was masked when STAT1 was bound to DNA, but accessible to Crm1 when nuclear STAT1 was not bound to DNA. To test this hypothesis, the behavior of a DNA binding mutation in STAT1-GFP was evaluated. The STAT1-GFP DBD mutant was tyrosine phosphorylated in response to IFN, but a microscopic snapshot image did not show STAT1-GFP fluorescence in the nucleus. Following treatment with both IFN and LMB, the STAT1 DBD mutant was found to accumulate in the nucleus. These results indicate the DBD mutant was imported to the nucleus, but effectively exported. It supports the premise that the NES is masked when tyrosine phosphorylated STAT1 is bound to DNA. Other studies that repositioned the NES in the full-length STAT1-GFP protein verified this NES function.Citation43 Following release of STAT1 from DNA by normal equilibrium or as a consequence of tyrosine dephosphorylation or association with a protein inhibitor of activated STAT (PIAS),Citation45 the STAT1 NES appears to become accessible to Crm1 recognition, and STAT1 is actively exported from the nucleus (). There may be other factors that contribute to STAT1 export, such as the existence of a non-Crm1 mediated NES that remains to be identified, nevertheless, the nuclear export of STAT1 correlates with its dephosphorylation and release from DNA.Citation43,Citation46
Along with the use of GFP-tagged proteins, confocal microscopy has advanced visualization of proteins with increased resolution, laser efficiency, and time-lapse imaging.Citation47 The advantage of the confocal microscope is the pinhole aperture in front of the object plane that eliminates out-of-focus images and allows high image resolution. With photobleaching and live cell imaging, microscopy has provided insight to the dynamics of STAT mobility. Continuous photoactivation of GFP will eventually destroy its fluorescence; however, this property can be used with various photobleaching techniques to follow the mobility of proteins in living cells. Fluorescence recovery after photobleaching (FRAP) is a method to bleach the GFP-tagged protein in a targeted area of the cell, and subsequently follow the recovery of fluorescence due to movement of unbleached GFP-tagged proteins into that area. The rate of recovery is proportional to the rate of movement of new molecules into the bleached area. Fluorescence loss in photobleaching (FLIP) uses repetitive high intensity pulses of light in a small area of the cell to continuously bleach any GFP-tagged proteins that enter this targeted area. Fluorescence loss is monitored in a second area of the cell. Loss of fluorescence in the second unbleached area indicates GFP-proteins have moved out of the second unbleached area into the laser path of the small bleached area. This technique can be used to measure movement of GFP tagged molecules from one area to another. Photobleaching techniques were used to visualize STAT1-GFP dynamic movements in the cell before and after IFN stimulation.Citation48 Cytoplasmic FLIP of STAT1-GFP in a small area resulted in entire loss of cytoplasmic fluorescence within seconds with or without IFN treatment indicating the very rapid movement of cytoplasmic STAT1-GFP. FLIP and FRAP in the nucleus also demonstrated rapid random movement of STAT1-GFP with no apparent immobile fraction. IFN signaling mediated by STAT1 was not dependent on intact cytoskeleton as it occurred in the presence of cytochalasin D or nocodazole, which respectively prevent actin polymerization and disrupt microtubules.
STAT1: Nuclear Import
Nuclear accumulation of tyrosine phosphorylated STAT1 occurs within minutes of IFN treatment.Citation49 Insight to the conditional regulated nuclear import of STAT1 was provided when tyrosine-phosphorylated STAT1, but not U-STAT1, was found to be recognized specifically by the importin-α5 adaptor ().Citation50 By microinjection of inhibitory antibodies to importin-α5 or importin-β1, and by expression of a dominant negative form of importin-α5, the importin-α5:importin-β1 heterodimer was shown to be responsible for the conditional nuclear import of tyrosine-phosphorylated STAT1. A responsible transport receptor was thereby identified for nuclear import of tyrosine phosphorylated STAT1. However, the conditional STAT1 NLS still remains to be fully characterized.
U-STAT1 in an antiparallel dimer conformation is not recognized by importin-α5, and does not have an identified NLS that can confer nuclear import to a heterologous protein. Inhibition of STAT1 nuclear export by LMB does not lead to U-STAT1 nuclear accumulation.Citation43 Tyrosine phosphorylation and the parallel dimer of STAT1 results in the gain of a conformational NLS. To identify amino acids necessary for nuclear import, mutational analyses were performed. Since in many nuclear proteins there is a close spatial relationship between the NLS and DNA binding domain,Citation51,Citation52 mutations in the STAT1 DBD were evaluated. Mutation of a single leucine 407 residue was found to block STAT1-GFP nuclear import.Citation53 This L407A STAT1 mutant was still tyrosine phosphorylated in response to IFN and could bind target DNA, but it could not bind importin-α5. It should be noted that these experiments were performed in U3A cells that lack endogenous STAT1,Citation54 since wt STAT1 can partially rescue nuclear import of the L407A mutant. Following import of tyrosine phosphorylated STAT1 to the nucleus, specific DNA targets effectively compete for STAT1 binding and apparently displace importin-α5.Citation53,Citation55 Intriguingly the leucine 407 critical for nuclear import is not a basic residue as found in conventional NLSs, and it is located within the NES of STAT1. Mutation of other residues in this region, lysine 410 and 413, was reported to block STAT1 nuclear import, however these mutations inhibit STAT1 DNA binding.Citation55,Citation56 Inhibition of DNA binding confounds interpretation of static images due to nuclear export, and although these residues are basic, they do not behave as a conventional NLS. Understanding the interface between tyrosine phosphorylated STAT1 and importin-α5 may await a co-crystal structure. A non-canonical model of STAT1 nuclear import has also been proposed that is dependent on IFN ligand and receptor internalization.Citation57 Following IFN binding to the receptor, an activated complex of IFN ligand/IFN receptor/JAK/STAT is endocytosed, and a classical NLS in the IFN ligand is proposed to be responsible for transporting the complex into the nucleus.Citation58
The nuclear import of STAT1 may not be restricted to tyrosine phosphorylated dimers. Detectable levels of U-STAT1 have also been demonstrated in the nucleus.Citation56,Citation59 Cell transfection experiments with a mutation of the STAT1 tyrosine 701 phosphorylation site demonstrated nuclear immunofluorescence of U-STAT1.Citation56 This study also described STAT1 association with the interferon regulatory factor 1 (IRF1), and it remains to be determined if the constitutive NLS of IRF1 contributes to STAT1 nuclear presence. Cell microinjection experiments with STAT1 antibodies or with STAT1 recombinant proteins have also indicated U-STAT1 can enter the nucleus.Citation56,Citation60 It was proposed that U-STAT1 interacts directly with the NPC independent of transport receptors since in vitro binding of STAT1 to a subset of Nups was demonstrated.Citation60 The means by which U-STAT1 may enter the nucleus remains to be completely understood.
Results indicate nuclear import of tyrosine phosphorylated STAT1 depends on a parallel dimer conformation with reciprocal phosphotyrosine-SH2 domain interactions between monomers (). This conformation of the phosphorylated dimer is recognized by the importin-α5:importin-β1 complex and transported through the NPC. In the nucleus, the transport complex dissociates and STAT1 binds to DNA. Following dephosphorylation, or release from DNA by other means, the NES within the DBD of STAT1 becomes accessible and is recognized by a Crm1-RanGTP complex for nuclear export. This elegant mechanism conditionally regulates STAT1 nuclear import and nuclear export coordinate with its ability to bind DNA. The conditional access to the nucleus was initially expected to be similar for all the STAT proteins. However, it now appears that nuclear trafficking of other STAT proteins is regulated distinctly.
STAT2: The IRF9 Connection
Cell imaging of endogenous unphosphorylated STAT2 (U-STAT2) clearly shows complete cytoplasmic localization of the protein.Citation61,Citation62 However, addition of the Crm1 inhibitor LMB leads to a dramatic nuclear accumulation of STAT2. This finding demonstrates U-STAT2 is imported to the nucleus and continually shuttles between nucleus and cytoplasm.
STAT2 differs from other members of the STAT family in that it associates constitutively with a non-STAT protein, the interferon regulatory factor 9 (IRF9).Citation63,Citation64 This was demonstrated by co-immunoprecipitation and by protein–protein interactions in vitro. Analysis of STAT2 deletions and STAT1-STAT2 chimeras identified a region within the coiled-coil domain of STAT2 (125–230aa) to be necessary for binding IRF9.Citation64 IRF9 is a member of the family of IRFs that share homology in their N-terminal DNA-binding domain.Citation65 IRF9 contains a bipartite basic NLS within its DBD, and DNA-binding is not required for its nuclear accumulation.Citation66 Association with STAT2 is mediated by a region within the carboxyl terminus of IRF9, and the constitutive nuclear import of U-STAT2 is dependent on its association with IRF9.Citation61
The nuclear import of U-STAT2 that is apparent with the addition of LMB does not occur in U2A cells lacking IRF9, and it does not occur with a deletion mutant of STAT2 that cannot bind IRF9.Citation61,Citation67 Since the steady-state location of U-STAT2 is in the cytoplasm, STAT2 must have a functional and dominant NES. Deletion analyses of STAT2-GFP identified a strong hydrophobic NES located within the C-terminus of STAT2. Therefore U-STAT2, continually bound to IRF9, is imported to the nucleus via IRF9 recognition by an importin-α:importin-β1 complex; however, a dominant NES in the C-terminus of STAT2 returns the STAT2-IRF9 complex back to the cytoplasm ().Citation61. U-STAT2-IRF9 continually shuttles in and out of the nucleus, but the U-STAT2 NES leads to prominence in the cytoplasm.
Figure 2. Nucleocytoplasmic transport of STAT2. Green image represents STAT2 with circle at N-terminus. U-STAT2 is bound to the IRF9 transcription factor and the complex is continually imported to the nucleus due to the constitutive NLS of IRF9 that is recognized by importin-α:importin-β1 (top left). In the nucleus, the Crm1-mediated NES in the carboxyl terminus of STAT2 effects dominant nuclear export of the complex (right). Following tyrosine phosphorylation by Janus kinases (JAK) in the cytoplasm, STAT2 dimerizes with tyrosine phosphorylated STAT1 to form the trimeric interferon stimulated gene factor 3 (ISGF3) that is imported to the nucleus and binds DNA (bottom left).
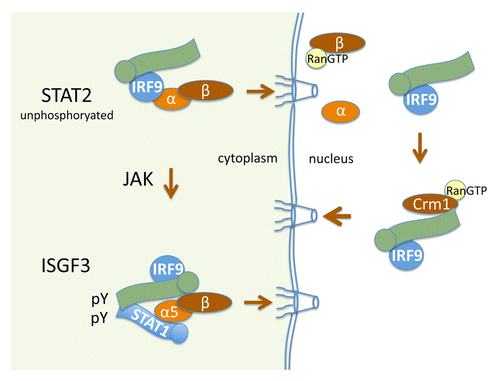
The cytoplasmic localization of STAT2 dramatically changes following type I or III IFN stimulation. IFNs stimulate the tyrosine phosphorylation of both STAT1 and STAT2, promoting a heterodimer mediated by phosphotyrosine and SH2-domains of STAT1 and STAT2 ().Citation5,Citation7 Since STAT2 is bound to IRF9, a multimeric complex of STAT1:STAT2:IRF9 is formed known as IFN stimulated gene factor 3 (ISGF3). Tyrosine-phosphorylated STAT2 within the ISGF3 complex rapidly accumulates in the nucleus due to its dimeric conformation with tyrosine phosphorylated STAT1 and formation of a conditional NLS recognized by the importin-α5:importin-β1 transporter.Citation61,Citation68 In the nucleus the ISGF3 complex binds specific DNA targets, designated IFN stimulated response elements (ISRE), to elicit gene induction. Following dephosphorylation, the NES of STAT2 leads to its return to the cytoplasm. STAT2 nuclear trafficking is thereby regulated both prior to and subsequent to tyrosine phosphorylation.
STAT4: Induced Nuclear Import
STAT4 is distinct from other STATs for the reason that its expression is restricted to spermatozoa, myeloid cells, and T lymphocytes.Citation69-Citation71 STAT4 was first demonstrated to be activated by tyrosine phosphorylation in response to interleukin-12 (IL-12) treatment of T cells.Citation72,Citation73 Most studies have focused on its role in T helper cell function,Citation74 and research on STAT4 nuclear transport is limited. Reports that exist indicate STAT4 nuclear import is dependent on the parallel conformation of tyrosine phosphorylated dimers, similar to STAT1.
Immunofluoresence studies demonstrate U-STAT4 is cytoplasmic, and STAT4 appears in the nucleus only following tyrosine phosphorylation.Citation75-Citation77 A study directed at identifying domains of STAT4 required for nuclear import evaluated the localization of STAT4-GFP.Citation78 STAT4-GFP accumulated in the nucleus only after cells were stimulated with IL-12. Deletion mutations determined a region of the DBD (395–416 aa) was needed for STAT4-GFP nuclear import. However, deletion of this domain may disrupt STAT4 conformation and prevent tyrosine phosphorylation, or prevent nuclear accumulation due to DNA binding. Clearly additional studies are needed to understand the regulation of STAT4 nuclear trafficking.
STAT3/STAT5/STAT6: Nuclear Import Independent of Tyrosine Phosphorylation
It was initially presumed that nuclear import of the STAT family would be dependent on tyrosine phosphorylation. However, STAT3, STAT5, and STAT6 factors are continually imported to the nucleus independent of tyrosine phosphorylation. Tyrosine phosphorylation may transiently increase their nuclear accumulation, but this appears to be a result of sequestration by DNA binding, or heterodimerization with other phosphorylated STATs. The NLS of these STATs appears to require the conformation of the coiled-coil domain.
STAT3
Microscopic visualization of endogenous STAT3 by immunofluorescence or by ectopically expressed STAT3-GFP clearly shows the nuclear presence of unphosphorylated STAT3 (U-STAT3).Citation79-Citation82 Nuclear import of U-STAT3 is evident with a double mutation that replaces both the phosphorylated tyrosine (Y705F) and a critical arginine in the SH2 domain (R609A), preventing the stable parallel dimer formation.Citation79 Therefore STAT3 nuclear import is independent of the tyrosine phosphorylated dimer. Structural evidence has even emerged that both the STAT3 tyrosine phosphorylated parallel dimer and the U-STAT3 anti-parallel dimer can directly bind DNA.Citation83,Citation84 The coiled-coil region of STAT3 appears to be required for constitutive NLS function.Citation79,Citation82,Citation85 Small deletions within the STAT3 coiled-coil domain can abrogate nuclear import. However these small deleted regions cannot confer nuclear import to a heterologous protein, and a much larger conformational NLS including the coiled-coil domain is needed.
U-STAT3 fragments containing the coiled-coil domain were shown to bind to the importin-α3 and importin-α6 adapters.Citation79 Since importin-α6 expression is limited to the testis, importin-α3 appears the primary NLS adaptor in most cells.Citation29 The specific binding to importin-α3 can be demonstrated for both U-STAT3 and tyrosine phosphorylated STAT3. In addition, knockdown of endogenous importin-α3 by siRNA inhibits the nuclear appearance of STAT3-GFP and leads to its aggregation outside the nuclear membrane.Citation79 In support of importin-α/importin-β mediated import, knockdown of importin-β1 with siRNA inhibits STAT3 nuclear import, and expression of a constitutive Ran mutant Q69L that remains in a GTP-bound state disrupts STAT3 nuclear import kinetics.Citation80
Clearly additional studies on STAT3 import are warranted. The tyrosine phosphorylated form of STAT3 also has been demonstrated to bind importin-α5 and -α7.Citation86 This indicates the parallel dimer of STAT3 could change its binding specificity to importins. Tyrosine phosphorylated STAT3 and STAT5 have also been reported to require the MgcRacGAP protein as an adaptor for nuclear import.Citation87 However, expression of the dominant negative N17Rac1 did not appear to effect STAT3 nuclear import.Citation80 MgcRacGAP was also reported to be necessary for tyrosine phosphorylation of STATs.
The continual nuclear import of U-STAT3 can be followed with live cell imaging by tagging STAT3 with a photoactivatable GFP (PA-GFP).Citation80 Within minutes after photoactivation of U-STAT3-PA-GFP in the cytoplasm, nuclear accumulation is apparent reaching equilibrium by 10 min. In addition, live cell imaging with nuclear FRAP of STAT3-GFP was used to evaluate the import kinetics in serum-starved cells (U-STAT3) or cytokine stimulated cells. The kinetics of nuclear fluorescence recovery were similar for U-STAT3 or tyrosine phosphorylated STAT3 in various cells tested.
STAT3 does not remain in the nucleus, but it continuously shuttles between nuclear and cytoplasmic compartments.Citation85,Citation88 In fact, the effect of tyrosine phosphorylation has been reported to increase the rate of STAT3 nucleoplasmic shuttling.Citation89 Nuclear export can be demonstrated with live cell imaging and photobleaching techniques such as cytoplasmic FLIP.Citation80 In this assay a continuous laser bleaches STAT3-GFP in the cytoplasm, and the decrease of nuclear STAT3-GFP indicates export from the nucleus into the path of the laser. By analyzing fragments of STAT3 and their cellular localization, multiple Crm1 dependent NES elements were identified.Citation90 However, LMB only delays nuclear export, and therefore STAT3 must have an additional non-Crm1 NES.Citation79
STAT5
Two of the STAT genes, Stat5a and Stat5b, encode proteins that are more than 95% identical.Citation91,Citation92 The crystal structure of unphosphorylated STAT5A has been solved, and similar to U-STAT1, U-STAT5A appears to exist as an anti-parallel homodimer.Citation93 Following STAT5A tyrosine 694 phosphorylation,Citation94 reciprocal SH2 domain and phosphotyrosine interactions promote a parallel conformation that gains the ability to bind specific DNA targets ().
Figure 3. STAT5 nuclear import independent of tyrosine phosphorylation. Orange image represents STAT5 with circle at N-terminus. U-STAT5 forms an anti-parallel dimer (bottom left) and is recognized by importin-α3:importin-β1 for continuous import to the nucleus. The coiled-coil domain functions as an unconventional NLS both before and following tyrosine phosphorylation of STAT5 by Janus kinases (JAK) (top left). Nuclear export of STAT5 is mediated by both Crm1-dependent and Crm1-independent NESs (right).
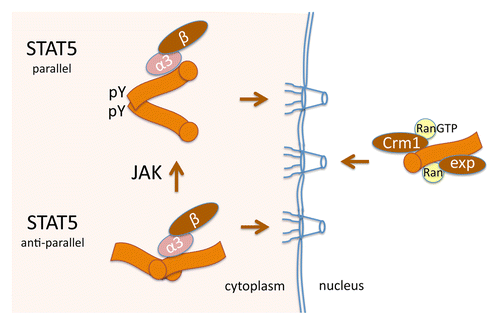
Studies with LMB provided the first indication that U-STAT5B shuttled between nucleus and cytoplasm.Citation95 Nuclear accumulation occurred with STAT5B-GFP independent of cytokine and tyrosine phosphorylation. N-terminal mutations of STAT5B-GFP that removed part of the coiled-coil domain abrogated the nuclear import.
Live cell imaging of STAT5A-GFP with photobleaching techniques demonstrated continuous shuttling of STAT5A in and out of the nucleus.Citation96 Nuclear FRAP of STAT5A was used to follow kinetics of U-STAT5A nuclear import. Complete recovery of U-STAT5A in the nucleus required more than an hour, in comparison to the rapid equilibrium within 3 min of the small diffusible GFP. Tyrosine phosphorylation of STAT5A in response to growth hormone did increase STAT5A nuclear accumulation. By evaluating STAT5A with a DNA-binding mutation, it was shown that this increase in nuclear accumulation required DNA binding. Comparative nuclear FLIP to bleach a very small region within the nucleus of cells showed that tyrosine phosphorylated STAT5A-GFP had dramatically slowed nuclear movement. Therefore nuclear accumulation of tyrosine phosphorylated STAT5A apparently results from retention due to DNA binding.
A small deletion within the coiled-coil domain of STAT5A was shown to abrogate nuclear import of otherwise full-length STAT5A;Citation96 however, a peptide corresponding to this region is not sufficient to function as an NLS.Citation97 The coiled-coil domain of STAT5 is composed of four α-helices.Citation93 Evaluation of each of these helices tagged with GFP either alone or in combination demonstrated that all four α-helices were required for effective nuclear import.Citation97 Although mutation of specific residues in the coiled-coil domain could abrogate nuclear import, STAT5A import is dependent on an unconventional NLS that functions within the conformation of an extensive coiled-coil domain. This domain was used in protein-protein interaction studies, and similar to STAT3, STAT5A binds to the importin-α3 adaptor and STAT5A nuclear import is inhibited with siRNA to importin-α3. These results indicate that importin-α3:importin-β1 is a primary transport receptor for STAT5A import.
Following import to the nucleus, STAT5 is recycled back to the cytoplasm. Live cell imaging with cytoplasmic FLIP was used to follow the kinetics of STAT5A-GFP nuclear export in the presence or absence of the Crm1 inhibitor LMB.Citation97 LMB was found to reduce the kinetics of STAT5A nuclear export, but it did not eliminate its nuclear export. Analysis of fragments of STAT5A capable of mediating nuclear export identified a Crm1-dependent NES in STAT5A N-terminus, and a Crm1-independent NES within the DBD of STAT5A. It remains to be determined if the Crm1-independent NES is accessible when STAT5A is bound to DNA.
STAT6
Nuclear trafficking of the STAT6 transcription factor shares many similarities with STAT3 and STAT5. The nuclear import of STAT6-GFP was evaluated by live cell imaging with nuclear FRAP, and it revealed similar import kinetics of U-STAT6 and tyrosine phosphorylated STAT6.Citation98 STAT6 tyrosine phosphorylation is stimulated by the cytokines interleukin-4 (IL-4) and IL-13, and following cytokine stimulation there is a noted accumulation of STAT6 in the nucleus. This nuclear accumulation is not due to a change in nuclear import, but is due to a decrease in nuclear export. A DNA-binding mutant of STAT6 is transported into the nucleus with a similar rate as wt STAT6, but it does not accumulate. Cytoplasmic FLIP of STAT6-GFP to measure nuclear export in live cells showed a dramatic decrease in nuclear export following IL-4 treatment.Citation98 In addition, FLIP of a very small region within the nucleus of cells treated with IL-4 demonstrated very slowed movement of wt STAT6 but not a DNA-binding mutant of STAT6.
To identify amino acids responsible for the continual nuclear import of STAT6, the cellular localization of STAT6 fragments tagged with GFP was evaluated.Citation98 As for STAT3 and STAT5, a region within the coiled-coil domain of STAT6 was shown to be required for nuclear import, although the precise aa are distinct. Small deletions within full-length STAT6 abrogate nuclear import, but peptides corresponding to the deletions cannot function as an independent NLS. A large conformational NLS exists appears to include the STAT6 coiled-coil domain. Similar to STAT3 and STAT5, STAT6 can bind importin-α3 in vitro and nuclear import of STAT6 appears to be mediated by the importin-α3:importin-β1 transport complex.
Dynamic movement of STAT3, STAT5, and STAT6 has been examined with live cell imaging and photobleaching techniques and shows their continual nuclear import independent of tyrosine phosphorylation. The region of these STAT proteins that mediates nuclear import appears to be an unconventional NLS that requires the coiled-coil domain. The specific residues that contribute to the NLS in each STAT appear to be distinct.Citation79,Citation97,Citation98 For this reason it may be possible to develop specific discriminating inhibitors that can block nuclear import of these individual STATs.
Perspectives
The STATs were first identified for their ability to transmit signals from the plasma membrane to target genes in the nucleus. In addition to gene induction, there now is evidence that STATs can also exert non-genomic functions.Citation99-Citation101 However their importance in regulation of nuclear transcription is well established. IFNs activate primarily STAT1 and STAT2, and are known to promote antiviral and inflammatory responses. Viruses have evolved many strategies to block STAT activation, and one mode of viral offense is to block STAT nuclear import in order to evade cellular defense genes.Citation102,Citation103 Although the domain structures of the STATs are conserved, they are activated in response to different cytokines and growth factors. It appears that nuclear import of STAT1, STAT2, and STAT4 is regulated by conditional tyrosine phosphorylation. This is distinct from STAT3, STAT5, and STAT6 that are imported to the nucleus independent of tyrosine phosphorylation, and whose persistent activation is causally linked to various cancers. Whether the physiological effects of the STATs have evolved with their regulated nuclear import remains an intriguing speculation.
Disclosure of Potential Conflicts of Interest
No potential conflicts of interest were disclosed.
Acknowledgments
Many thanks to past and present members of the lab for their insight, enthusiasm, and dedication. Particular thanks to HaYoun Shin and Dr Hui-Chen Foreman for contributions to the manuscript. This work was supported by grants from NIH and a Carol Baldwin Cancer Research Award to NC Reich.
References
- Hetzer MW. The nuclear envelope. Cold Spring Harb Perspect Biol 2010; 2:a000539; http://dx.doi.org/10.1101/cshperspect.a000539; PMID: 20300205
- Wilson KL, Berk JM. The nuclear envelope at a glance. J Cell Sci 2010; 123:1973 - 8; http://dx.doi.org/10.1242/jcs.019042; PMID: 20519579
- Rout MP, Aitchison JD. The nuclear pore complex as a transport machine. J Biol Chem 2001; 276:16593 - 6; http://dx.doi.org/10.1074/jbc.R100015200; PMID: 11283009
- Levy DE, Darnell JE Jr.. Stats: transcriptional control and biological impact. Nat Rev Mol Cell Biol 2002; 3:651 - 62; http://dx.doi.org/10.1038/nrm909; PMID: 12209125
- Schindler C, Levy DE, Decker T. JAK-STAT signaling: from interferons to cytokines. J Biol Chem 2007; 282:20059 - 63; http://dx.doi.org/10.1074/jbc.R700016200; PMID: 17502367
- Reich NC, Liu L. Tracking STAT nuclear traffic. Nat Rev Immunol 2006; 6:602 - 12; http://dx.doi.org/10.1038/nri1885; PMID: 16868551
- Stark GR, Darnell JE Jr.. The JAK-STAT pathway at twenty. Immunity 2012; 36:503 - 14; http://dx.doi.org/10.1016/j.immuni.2012.03.013; PMID: 22520844
- Cheon H, Yang J, Stark GR. The functions of signal transducers and activators of transcriptions 1 and 3 as cytokine-inducible proteins. J Interferon Cytokine Res 2011; 31:33 - 40; http://dx.doi.org/10.1089/jir.2010.0100; PMID: 21166594
- Bromberg JF, Wrzeszczynska MH, Devgan G, Zhao Y, Pestell RG, Albanese C, Darnell JE Jr.. Stat3 as an oncogene. Cell 1999; 98:295 - 303; http://dx.doi.org/10.1016/S0092-8674(00)81959-5; PMID: 10458605
- Akira S. Functional roles of STAT family proteins: lessons from knockout mice. Stem Cells 1999; 17:138 - 46; http://dx.doi.org/10.1002/stem.170138; PMID: 10342556
- O’Shea JJ, Holland SM, Staudt LM. JAKs and STATs in immunity, immunodeficiency, and cancer. N Engl J Med 2013; 368:161 - 70; http://dx.doi.org/10.1056/NEJMra1202117; PMID: 23301733
- Yu H, Pardoll D, Jove R. STATs in cancer inflammation and immunity: a leading role for STAT3. Nat Rev Cancer 2009; 9:798 - 809; http://dx.doi.org/10.1038/nrc2734; PMID: 19851315
- Casanova JL, Holland SM, Notarangelo LD. Inborn errors of human JAKs and STATs. Immunity 2012; 36:515 - 28; http://dx.doi.org/10.1016/j.immuni.2012.03.016; PMID: 22520845
- Walters AD, Bommakanti A, Cohen-Fix O. Shaping the nucleus: factors and forces. J Cell Biochem 2012; 113:2813 - 21; http://dx.doi.org/10.1002/jcb.24178; PMID: 22566057
- Aitchison JD, Rout MP. The yeast nuclear pore complex and transport through it. Genetics 2012; 190:855 - 83; http://dx.doi.org/10.1534/genetics.111.127803; PMID: 22419078
- Raices M, D’Angelo MA. Nuclear pore complex composition: a new regulator of tissue-specific and developmental functions. Nat Rev Mol Cell Biol 2012; 13:687 - 99; http://dx.doi.org/10.1038/nrm3461; PMID: 23090414
- Wente SR, Rout MP. The nuclear pore complex and nuclear transport. Cold Spring Harb Perspect Biol 2010; 2:a000562; http://dx.doi.org/10.1101/cshperspect.a000562; PMID: 20630994
- Chook YM, Blobel G. Karyopherins and nuclear import. Curr Opin Struct Biol 2001; 11:703 - 15; http://dx.doi.org/10.1016/S0959-440X(01)00264-0; PMID: 11751052
- Hülsmann BB, Labokha AA, Görlich D. The permeability of reconstituted nuclear pores provides direct evidence for the selective phase model. Cell 2012; 150:738 - 51; http://dx.doi.org/10.1016/j.cell.2012.07.019; PMID: 22901806
- Peters R. Translocation through the nuclear pore complex: selectivity and speed by reduction-of-dimensionality. Traffic 2005; 6:421 - 7; http://dx.doi.org/10.1111/j.1600-0854.2005.00287.x; PMID: 15813752
- Rout MP, Aitchison JD, Magnasco MO, Chait BT. Virtual gating and nuclear transport: the hole picture. Trends Cell Biol 2003; 13:622 - 8; http://dx.doi.org/10.1016/j.tcb.2003.10.007; PMID: 14624840
- Cook A, Bono F, Jinek M, Conti E. Structural biology of nucleocytoplasmic transport. Annu Rev Biochem 2007; 76:647 - 71; http://dx.doi.org/10.1146/annurev.biochem.76.052705.161529; PMID: 17506639
- Macara IG. Transport into and out of the nucleus. Microbiol Mol Biol Rev 2001; 65:570 - 94; http://dx.doi.org/10.1128/MMBR.65.4.570-594.2001; PMID: 11729264
- Pemberton LF, Paschal BM. Mechanisms of receptor-mediated nuclear import and nuclear export. Traffic 2005; 6:187 - 98; http://dx.doi.org/10.1111/j.1600-0854.2005.00270.x; PMID: 15702987
- Mattaj IW, Englmeier L. Nucleocytoplasmic transport: the soluble phase. Annu Rev Biochem 1998; 67:265 - 306; http://dx.doi.org/10.1146/annurev.biochem.67.1.265; PMID: 9759490
- Görlich D, Kutay U. Transport between the cell nucleus and the cytoplasm. Annu Rev Cell Dev Biol 1999; 15:607 - 60; http://dx.doi.org/10.1146/annurev.cellbio.15.1.607; PMID: 10611974
- Stewart M. Molecular mechanism of the nuclear protein import cycle. Nat Rev Mol Cell Biol 2007; 8:195 - 208; http://dx.doi.org/10.1038/nrm2114; PMID: 17287812
- Goldfarb DS, Corbett AH, Mason DA, Harreman MT, Adam SA. Importin alpha: a multipurpose nuclear-transport receptor. Trends Cell Biol 2004; 14:505 - 14; http://dx.doi.org/10.1016/j.tcb.2004.07.016; PMID: 15350979
- Köhler M, Speck C, Christiansen M, Bischoff FR, Prehn S, Haller H, Görlich D, Hartmann E. Evidence for distinct substrate specificities of importin alpha family members in nuclear protein import. Mol Cell Biol 1999; 19:7782 - 91; PMID: 10523667
- Fornerod M, Ohno M, Yoshida M, Mattaj IW. CRM1 is an export receptor for leucine-rich nuclear export signals. [see comments] Cell 1997; 90:1051 - 60; http://dx.doi.org/10.1016/S0092-8674(00)80371-2; PMID: 9323133
- Kudo N, Wolff B, Sekimoto T, Schreiner EP, Yoneda Y, Yanagida M, Horinouchi S, Yoshida M. Leptomycin B inhibition of signal-mediated nuclear export by direct binding to CRM1. Exp Cell Res 1998; 242:540 - 7; http://dx.doi.org/10.1006/excr.1998.4136; PMID: 9683540
- Tsien RY. The green fluorescent protein. Annu Rev Biochem 1998; 67:509 - 44; http://dx.doi.org/10.1146/annurev.biochem.67.1.509; PMID: 9759496
- Chudakov DM, Matz MV, Lukyanov S, Lukyanov KA. Fluorescent proteins and their applications in imaging living cells and tissues. Physiol Rev 2010; 90:1103 - 63; http://dx.doi.org/10.1152/physrev.00038.2009; PMID: 20664080
- Wang Y, Shyy JY, Chien S. Fluorescence proteins, live-cell imaging, and mechanobiology: seeing is believing. Annu Rev Biomed Eng 2008; 10:1 - 38; http://dx.doi.org/10.1146/annurev.bioeng.010308.161731; PMID: 18647110
- Shaner NC, Steinbach PA, Tsien RY. A guide to choosing fluorescent proteins. Nat Methods 2005; 2:905 - 9; http://dx.doi.org/10.1038/nmeth819; PMID: 16299475
- Bach EA, Aguet M, Schreiber RD. The IFN gamma receptor: a paradigm for cytokine receptor signaling. Annu Rev Immunol 1997; 15:563 - 91; http://dx.doi.org/10.1146/annurev.immunol.15.1.563; PMID: 9143700
- Chen X, Vinkemeier U, Zhao Y, Jeruzalmi D, Darnell JE Jr., Kuriyan J. Crystal structure of a tyrosine phosphorylated STAT-1 dimer bound to DNA. Cell 1998; 93:827 - 39; http://dx.doi.org/10.1016/S0092-8674(00)81443-9; PMID: 9630226
- Mao X, Ren Z, Parker GN, Sondermann H, Pastorello MA, Wang W, McMurray JS, Demeler B, Darnell JE Jr., Chen X. Structural bases of unphosphorylated STAT1 association and receptor binding. Mol Cell 2005; 17:761 - 71; http://dx.doi.org/10.1016/j.molcel.2005.02.021; PMID: 15780933
- Zhong M, Henriksen MA, Takeuchi K, Schaefer O, Liu B, ten Hoeve J, Ren Z, Mao X, Chen X, Shuai K, et al. Implications of an antiparallel dimeric structure of nonphosphorylated STAT1 for the activation-inactivation cycle. Proc Natl Acad Sci U S A 2005; 102:3966 - 71; http://dx.doi.org/10.1073/pnas.0501063102; PMID: 15753310
- Köster M, Hauser H. Dynamic redistribution of STAT1 protein in IFN signaling visualized by GFP fusion proteins. Eur J Biochem 1999; 260:137 - 44; http://dx.doi.org/10.1046/j.1432-1327.1999.00149.x; PMID: 10091593
- David M, Grimley PM, Finbloom DS, Larner AC. A nuclear tyrosine phosphatase downregulates interferon-induced gene expression. Mol Cell Biol 1993; 13:7515 - 21; PMID: 8246969
- Haspel RL, Salditt-Georgieff M, Darnell JE Jr.. The rapid inactivation of nuclear tyrosine phosphorylated Stat1 depends upon a protein tyrosine phosphatase. EMBO J 1996; 15:6262 - 8; PMID: 8947049
- McBride KM, McDonald C, Reich NC. Nuclear export signal located within theDNA-binding domain of the STAT1transcription factor. EMBO J 2000; 19:6196 - 206; http://dx.doi.org/10.1093/emboj/19.22.6196; PMID: 11080165
- ten Hoeve J, de Jesus Ibarra-Sanchez M, Fu Y, Zhu W, Tremblay M, David M, Shuai K. Identification of a nuclear Stat1 protein tyrosine phosphatase. Mol Cell Biol 2002; 22:5662 - 8; http://dx.doi.org/10.1128/MCB.22.16.5662-5668.2002; PMID: 12138178
- Shuai K, Liu B. Regulation of gene-activation pathways by PIAS proteins in the immune system. Nat Rev Immunol 2005; 5:593 - 605; http://dx.doi.org/10.1038/nri1667; PMID: 16056253
- Meyer T, Marg A, Lemke P, Wiesner B, Vinkemeier U. DNA binding controls inactivation and nuclear accumulation of the transcription factor Stat1. Genes Dev 2003; 17:1992 - 2005; http://dx.doi.org/10.1101/gad.268003; PMID: 12923054
- Smith CL. Basic confocal microscopy. Current protocols in molecular biology / edited by Frederick M Ausubel [et al 2008; Chapter 14:Unit 14 1.
- Lillemeier BF, Köster M, Kerr IM. STAT1 from the cell membrane to the DNA. EMBO J 2001; 20:2508 - 17; http://dx.doi.org/10.1093/emboj/20.10.2508; PMID: 11350940
- Schindler C, Shuai K, Prezioso VR, Darnell JE Jr.. Interferon-dependent tyrosine phosphorylation of a latent cytoplasmic transcription factor. Science 1992; 257:809 - 13; http://dx.doi.org/10.1126/science.1496401; PMID: 1496401
- Sekimoto T, Imamoto N, Nakajima K, Hirano T, Yoneda Y. Extracellular signal-dependent nuclear import of Stat1 is mediated by nuclear pore-targeting complex formation with NPI-1, but not Rch1. EMBO J 1997; 16:7067 - 77; http://dx.doi.org/10.1093/emboj/16.23.7067; PMID: 9384585
- LaCasse EC, Lefebvre YA. Nuclear localization signals overlap DNA- or RNA-binding domains in nucleic acid-binding proteins. Nucleic Acids Res 1995; 23:1647 - 56; http://dx.doi.org/10.1093/nar/23.10.1647; PMID: 7540284
- Cokol M, Nair R, Rost B. Finding nuclear localization signals. EMBO Rep 2000; 1:411 - 5; http://dx.doi.org/10.1093/embo-reports/kvd092; PMID: 11258480
- McBride KM, Banninger G, McDonald C, Reich NC. Regulated nuclear import of the STAT1 transcription factor by direct binding of importin-alpha. EMBO J 2002; 21:1754 - 63; http://dx.doi.org/10.1093/emboj/21.7.1754; PMID: 11927559
- McKendry R, John J, Flavell D, Müller M, Kerr IM, Stark GR. High-frequency mutagenesis of human cells and characterization of a mutant unresponsive to both alpha and gamma interferons. Proc Natl Acad Sci U S A 1991; 88:11455 - 9; http://dx.doi.org/10.1073/pnas.88.24.11455; PMID: 1837150
- Fagerlund R, Mélen K, Kinnunen L, Julkunen I. Arginine/lysine-rich nuclear localization signals mediate interactions between dimeric STATs and importin alpha 5. J Biol Chem 2002; 277:30072 - 8; http://dx.doi.org/10.1074/jbc.M202943200; PMID: 12048190
- Meyer T, Begitt A, Lödige I, van Rossum M, Vinkemeier U. Constitutive and IFN-gamma-induced nuclear import of STAT1 proceed through independent pathways. EMBO J 2002; 21:344 - 54; http://dx.doi.org/10.1093/emboj/21.3.344; PMID: 11823427
- Johnson HM, Subramaniam PS, Olsnes S, Jans DA. Trafficking and signaling pathways of nuclear localizing protein ligands and their receptors. Bioessays 2004; 26:993 - 1004; http://dx.doi.org/10.1002/bies.20086; PMID: 15351969
- Johnson HM, Noon-Song EN, Dabelic R, Ahmed CM. IFN signaling: how a non-canonical model led to the development of IFN mimetics. Front Immunol 2013; 4:202; http://dx.doi.org/10.3389/fimmu.2013.00202; PMID: 23898330
- Chatterjee-Kishore M, Wright KL, Ting JP, Stark GR. How Stat1 mediates constitutive gene expression: a complex of unphosphorylated Stat1 and IRF1 supports transcription of the LMP2 gene. EMBO J 2000; 19:4111 - 22; http://dx.doi.org/10.1093/emboj/19.15.4111; PMID: 10921891
- Marg A, Shan Y, Meyer T, Meissner T, Brandenburg M, Vinkemeier U. Nucleocytoplasmic shuttling by nucleoporins Nup153 and Nup214 and CRM1-dependent nuclear export control the subcellular distribution of latent Stat1. J Cell Biol 2004; 165:823 - 33; http://dx.doi.org/10.1083/jcb.200403057; PMID: 15210729
- Banninger G, Reich NC. STAT2 nuclear trafficking. J Biol Chem 2004; 279:39199 - 206; http://dx.doi.org/10.1074/jbc.M400815200; PMID: 15175343
- Frahm T, Hauser H, Köster M. IFN-type-I-mediated signaling is regulated by modulation of STAT2 nuclear export. J Cell Sci 2006; 119:1092 - 104; http://dx.doi.org/10.1242/jcs.02822; PMID: 16507591
- Horvath CM, Stark GR, Kerr IM, Darnell JE Jr.. Interactions between STAT and non-STAT proteins in the interferon-stimulated gene factor 3 transcription complex. Mol Cell Biol 1996; 16:6957 - 64; PMID: 8943351
- Martinez-Moczygemba M, Gutch MJ, French DL, Reich NC. Distinct STAT structure promotes interaction of STAT2 with the p48 subunit of the interferon-alpha-stimulated transcription factor ISGF3. J Biol Chem 1997; 272:20070 - 6; http://dx.doi.org/10.1074/jbc.272.32.20070; PMID: 9242679
- Tamura T, Yanai H, Savitsky D, Taniguchi T. The IRF family transcription factors in immunity and oncogenesis. Annu Rev Immunol 2008; 26:535 - 84; http://dx.doi.org/10.1146/annurev.immunol.26.021607.090400; PMID: 18303999
- Lau JF, Parisien JP, Horvath CM. Interferon regulatory factor subcellular localization is determined by a bipartite nuclear localization signal in the DNA-binding domain and interaction with cytoplasmic retention factors. Proc Natl Acad Sci U S A 2000; 97:7278 - 83; http://dx.doi.org/10.1073/pnas.97.13.7278; PMID: 10860992
- Pellegrini S, John J, Shearer M, Kerr IM, Stark GR. Use of a selectable marker regulated by alpha interferon to obtain mutations in the signaling pathway. Mol Cell Biol 1989; 9:4605 - 12; PMID: 2513475
- Melen K, Fagerlund R, Franke J, Kohler M, Kinnunen L, Julkunen I. Importin alpha nuclear localization signal binding sites for STAT1, STAT2, and influenza A virus nucleoprotein. J Biol Chem 2003; 278:28193 - 200; http://dx.doi.org/10.1074/jbc.M303571200; PMID: 12740372
- Yamamoto K, Quelle FW, Thierfelder WE, Kreider BL, Gilbert DJ, Jenkins NA, Copeland NG, Silvennoinen O, Ihle JN. Stat4, a novel gamma interferon activation site-binding protein expressed in early myeloid differentiation. Mol Cell Biol 1994; 14:4342 - 9; PMID: 8007943
- Zhong Z, Wen Z, Darnell JE Jr.. Stat3 and Stat4: members of the family of signal transducers and activators of transcription. Proc Natl Acad Sci U S A 1994; 91:4806 - 10; http://dx.doi.org/10.1073/pnas.91.11.4806; PMID: 7545930
- Herrada G, Wolgemuth DJ. The mouse transcription factor Stat4 is expressed in haploid male germ cells and is present in the perinuclear theca of spermatozoa. J Cell Sci 1997; 110:1543 - 53; PMID: 9247188
- Bacon CM, Petricoin EF 3rd, Ortaldo JR, Rees RC, Larner AC, Johnston JA, O’Shea JJ. Interleukin 12 induces tyrosine phosphorylation and activation of STAT4 in human lymphocytes. Proc Natl Acad Sci U S A 1995; 92:7307 - 11; http://dx.doi.org/10.1073/pnas.92.16.7307; PMID: 7638186
- Jacobson NG, Szabo SJ, Weber-Nordt RM, Zhong Z, Schreiber RD, Darnell JE Jr., Murphy KM. Interleukin 12 signaling in T helper type 1 (Th1) cells involves tyrosine phosphorylation of signal transducer and activator of transcription (Stat)3 and Stat4. J Exp Med 1995; 181:1755 - 62; http://dx.doi.org/10.1084/jem.181.5.1755; PMID: 7722452
- Watford WT, Hissong BD, Bream JH, Kanno Y, Muul L, O’Shea JJ. Signaling by IL-12 and IL-23 and the immunoregulatory roles of STAT4. Immunol Rev 2004; 202:139 - 56; http://dx.doi.org/10.1111/j.0105-2896.2004.00211.x; PMID: 15546391
- Dumler I, Kopmann A, Wagner K, Mayboroda OA, Jerke U, Dietz R, Haller H, Gulba DC. Urokinase induces activation and formation of Stat4 and Stat1-Stat2 complexes in human vascular smooth muscle cells. J Biol Chem 1999; 274:24059 - 65; http://dx.doi.org/10.1074/jbc.274.34.24059; PMID: 10446176
- Berenson LS, Gavrieli M, Farrar JD, Murphy TL, Murphy KM. Distinct characteristics of murine STAT4 activation in response to IL-12 and IFN-alpha. J Immunol 2006; 177:5195 - 203; PMID: 17015705
- Toyoda H, Ido M, Hayashi T, Gabazza EC, Suzuki K, Bu J, Tanaka S, Nakano T, Kamiya H, Chipeta J, et al. Impairment of IL-12-dependent STAT4 nuclear translocation in a patient with recurrent Mycobacterium avium infection. J Immunol 2004; 172:3905 - 12; PMID: 15004198
- Huang Yu-Mei WY-P. Li Xuan-An, Yuan Yuan, Luo Qi-Zhi, LIi Ming. Amino acids 395–416 in DNA binding domain of STAT4 is involved in IL-12-induced nuclear import of STAT4. Acta Phys Sin 2012; 64:372 - 8
- Liu L, McBride KM, Reich NC. STAT3 nuclear import is independent of tyrosine phosphorylation and mediated by importin-alpha3. Proc Natl Acad Sci U S A 2005; 102:8150 - 5; http://dx.doi.org/10.1073/pnas.0501643102; PMID: 15919823
- Cimica V, Chen HC, Iyer JK, Reich NC. Dynamics of the STAT3 transcription factor: nuclear import dependent on Ran and importin-β1. PLoS One 2011; 6:e20188; http://dx.doi.org/10.1371/journal.pone.0020188; PMID: 21625522
- Herrmann A, Sommer U, Pranada AL, Giese B, Küster A, Haan S, Becker W, Heinrich PC, Müller-Newen G. STAT3 is enriched in nuclear bodies. J Cell Sci 2004; 117:339 - 49; http://dx.doi.org/10.1242/jcs.00833; PMID: 14657276
- Ma J, Zhang T, Novotny-Diermayr V, Tan AL, Cao X. A novel sequence in the coiled-coil domain of Stat3 essential for its nuclear translocation. J Biol Chem 2003; 278:29252 - 60; http://dx.doi.org/10.1074/jbc.M304196200; PMID: 12746441
- Becker S, Groner B, Müller CW. Three-dimensional structure of the Stat3beta homodimer bound to DNA. Nature 1998; 394:145 - 51; http://dx.doi.org/10.1038/28101; PMID: 9671298
- Nkansah E, Shah R, Collie GW, Parkinson GN, Palmer J, Rahman KM, Bui TT, Drake AF, Husby J, Neidle S, et al. Observation of unphosphorylated STAT3 core protein binding to target dsDNA by PEMSA and X-ray crystallography. FEBS Lett 2013; 587:833 - 9; http://dx.doi.org/10.1016/j.febslet.2013.01.065; PMID: 23434585
- Pranada AL, Metz S, Herrmann A, Heinrich PC, Müller-Newen G. Real time analysis of STAT3 nucleocytoplasmic shuttling. J Biol Chem 2004; 279:15114 - 23; http://dx.doi.org/10.1074/jbc.M312530200; PMID: 14701810
- Ma J, Cao X. Regulation of Stat3 nuclear import by importin alpha5 and importin alpha7 via two different functional sequence elements. Cell Signal 2006; 18:1117 - 26; http://dx.doi.org/10.1016/j.cellsig.2005.06.016; PMID: 16298512
- Kawashima T, Bao YC, Minoshima Y, Nomura Y, Hatori T, Hori T, Fukagawa T, Fukada T, Takahashi N, Nosaka T, et al. A Rac GTPase-activating protein, MgcRacGAP, is a nuclear localizing signal-containing nuclear chaperone in the activation of STAT transcription factors. Mol Cell Biol 2009; 29:1796 - 813; http://dx.doi.org/10.1128/MCB.01423-08; PMID: 19158271
- Cimica V, Reich NC. Nuclear trafficking of STAT proteins visualized by live cell imaging. Methods Mol Biol 2013; 967:189 - 202; http://dx.doi.org/10.1007/978-1-62703-242-1_14; PMID: 23296731
- Herrmann A, Vogt M, Mönnigmann M, Clahsen T, Sommer U, Haan S, Poli V, Heinrich PC, Müller-Newen G. Nucleocytoplasmic shuttling of persistently activated STAT3. J Cell Sci 2007; 120:3249 - 61; http://dx.doi.org/10.1242/jcs.03482; PMID: 17726064
- Bhattacharya S, Schindler C. Regulation of Stat3 nuclear export. J Clin Invest 2003; 111:553 - 9; PMID: 12588893
- Liu X, Robinson GW, Gouilleux F, Groner B, Hennighausen L. Cloning and expression of Stat5 and an additional homologue (Stat5b) involved in prolactin signal transduction in mouse mammary tissue. Proc Natl Acad Sci U S A 1995; 92:8831 - 5; http://dx.doi.org/10.1073/pnas.92.19.8831; PMID: 7568026
- Mui AL, Wakao H, Harada N, O’Farrell AM, Miyajima A. Interleukin-3, granulocyte-macrophage colony-stimulating factor, and interleukin-5 transduce signals through two forms of STAT5. J Leukoc Biol 1995; 57:799 - 803; PMID: 7539031
- Neculai D, Neculai AM, Verrier S, Straub K, Klumpp K, Pfitzner E, Becker S. Structure of the unphosphorylated STAT5A dimer. J Biol Chem 2005; 280:40782 - 7; http://dx.doi.org/10.1074/jbc.M507682200; PMID: 16192273
- Gouilleux F, Wakao H, Mundt M, Groner B. Prolactin induces phosphorylation of Tyr694 of Stat5 (MGF), a prerequisite for DNA binding and induction of transcription. EMBO J 1994; 13:4361 - 9; PMID: 7925280
- Zeng R, Aoki Y, Yoshida M, Arai K, Watanabe S. Stat5B shuttles between cytoplasm and nucleus in a cytokine-dependent and -independent manner. J Immunol 2002; 168:4567 - 75; PMID: 11971004
- Iyer J, Reich NC. Constitutive nuclear import of latent and activated STAT5A by its coiled-coil domain. FASEB J 2008; 22:391 - 400; http://dx.doi.org/10.1096/fj.07-8965com; PMID: 17846080
- Shin HY, Reich NC. Dynamic trafficking of STAT5 depends on an unconventional nuclear localization signal. J Cell Sci 2013; 126:3333 - 43; http://dx.doi.org/10.1242/jcs.123042; PMID: 23704351
- Chen HC, Reich NC. Live cell imaging reveals continuous STAT6 nuclear trafficking. J Immunol 2010; 185:64 - 70; http://dx.doi.org/10.4049/jimmunol.0903323; PMID: 20498360
- Gough DJ, Corlett A, Schlessinger K, Wegrzyn J, Larner AC, Levy DE. Mitochondrial STAT3 supports Ras-dependent oncogenic transformation. Science 2009; 324:1713 - 6; http://dx.doi.org/10.1126/science.1171721; PMID: 19556508
- Wegrzyn J, Potla R, Chwae YJ, Sepuri NB, Zhang Q, Koeck T, Derecka M, Szczepanek K, Szelag M, Gornicka A, et al. Function of mitochondrial Stat3 in cellular respiration. Science 2009; 323:793 - 7; http://dx.doi.org/10.1126/science.1164551; PMID: 19131594
- Verma NK, Dourlat J, Davies AM, Long A, Liu WQ, Garbay C, Kelleher D, Volkov Y. STAT3-stathmin interactions control microtubule dynamics in migrating T-cells. J Biol Chem 2009; 284:12349 - 62; http://dx.doi.org/10.1074/jbc.M807761200; PMID: 19251695
- Reid SP, Valmas C, Martinez O, Sanchez FM, Basler CF. Ebola virus VP24 proteins inhibit the interaction of NPI-1 subfamily karyopherin alpha proteins with activated STAT1. J Virol 2007; 81:13469 - 77; http://dx.doi.org/10.1128/JVI.01097-07; PMID: 17928350
- Palosaari H, Parisien JP, Rodriguez JJ, Ulane CM, Horvath CM. STAT protein interference and suppression of cytokine signal transduction by measles virus V protein. J Virol 2003; 77:7635 - 44; http://dx.doi.org/10.1128/JVI.77.13.7635-7644.2003; PMID: 12805463