Abstract
Isolating high-affinity antibodies against native tumor antigens on the cell surface is not straightforward using standard hybridoma procedures. Here, we describe a combination method of synthetic peptide immunization and high-throughput flow cytometry screening to efficiently isolate hybridomas for cell binding. Using this method, we identified high-affinity monoclonal antibodies specific for the native form of glypcian-3 (GPC3), a target heterogeneously expressed in hepatocellular carcinoma (HCC) and other cancers. We isolated a panel of monoclonal antibodies (YP6, YP7, YP8, YP9 and YP9.1) for cell surface binding. The antibodies were used to characterize GPC3 protein expression in human liver cancer cell lines and tissues by flow cytometry, immunoblotting and immunohistochemistry. The best antibody (YP7) bound cell surface-associated GPC3 with equilibrium dissociation constant, KD = 0.3 nmol/L and was highly specific for HCC, not normal tissues or other forms of primary liver cancers (such as cholangiocarcinoma). Interestingly, the new antibody was highly sensitive in that it detected GPC3 in low expression ovarian clear cell carcinoma and melanoma cells. The YP7 antibody exhibited significant HCC xenograft tumor growth inhibition in nude mice. These results describe an improved method for producing high-affinity monoclonal antibodies to cell surface tumor antigens and represent a general approach to isolate therapeutic antibodies against cancer. The new high-affinity antibodies described here have significant potential for GPC3-expressing cancer diagnostics and therapy.
Introduction
Liver cancer is the fifth most common malignant tumor worldwide.Citation1 Hepatocellular carcinoma (HCC) is the most common form. Cholangiocarcinoma (CCA) is another major form of primary liver cancer. Although surgical resection offers a standard method for treatment of the disease, only a small portion of patients are eligible for the procedure. Liver cancer does not respond to most chemotherapy drugs. There is a critical need for novel immunotherapy such as antibody therapy. In view of this, it has been suggested that glypican-3 (GPC3) represents an attractive target for liver cancer therapy because it is highly expressed in HCC.Citation2,Citation3 The GPC3 gene encodes a 70-kDa precursor core protein that can be cleaved by furin to generate a 40-kDa amino (N) terminal protein and a 30-kDa membrane-bound carboxyl (C) terminal protein.Citation4,Citation5 The C-terminus is attached to the cell membrane by a glycosylphosphatidylinositol (GPI) anchor. GPC3 has been suggested as a target for antibody and cell-based immunotherapies.Citation6-Citation10 However, GPC3 expression is highly heterogeneous in HCC and other cancers, e.g., ovarian clear cell carcinoma, melanoma.Citation11 Ideal therapeutic monoclonal antibodies (mAbs) should eliminate tumor cells expressing low levels of target antigen. Research in the area has been hampered by the lack of high-affinity mAbs that could be used to detect low expression of GPC3 in tumor cells for cancer therapy and diagnostics.
Filmus and colleagues developed the widely-used 1G12 mAb specific for the C-terminus of GPC3 and established an ELISA method to detect serum GPC3 in HCC patients.Citation2 On the other hand, Hippo et al. developed mAbs specific for the N-terminus of GPC3.Citation12 While both studies detected soluble GPC3 protein in HCC culture supernatant or in the circulating blood of cancer patients, it is not clear whether the N-terminal or C-terminal subunit represents the soluble GPC3 format.Citation3 Our unpublished work (and that of others) indicates that concentrations of serum GPC3 are generally not high in patients and that none of the readily available mAbs can be used to measure serum GPC3 due most likely to low affinity. Thus, new high-affinity antibodies for GPC3 may be useful to analyze and measure serum GPC3 in patients and to study liver cancer.
Here, we describe a panel of novel antibodies that bind with high affinity to cell surface and soluble GPC3 proteins. A high-throughput flow cytometry method using the Guava EasyCyte system was developed for primary screening of a large number of mAbs recognizing the native form of GPC3 on the cell surface. The best mAb may be a very promising candidate for liver cancer diagnostics and therapy.
Results
Design of the peptide as an immunogen for mouse immunization
To design a peptide for immunization, we analyzed the primary structure of the GPC3 protein because a 3D structure is not available. The mature GPC3 protein on the cell surface is modified by heparan sulfate chains and attached to the cell membrane by a GPI anchor (). The GPC3 gene is located on human X chromosome (Xq26) and transcribed and alternatively spliced into four mRNA isoforms.Citation4 All isoforms share the same C-terminal subunit while their N-terminal subunits are slightly different. Their distribution and functional significance have not been established. The most common one (Isoform 2, GenBank Accession Nos.: NP_004475 and JQ943686) encodes a 70 kDa core protein with 580 amino acids.Citation1,Citation4 Three variants have been identified in GenBank that encode alternatively spliced forms termed Isoform 1 (NP_001158089), Isoform 3 (NP_001158090) and Isoform 4 (NP_001158091).
Figure 1. Discovery of high-affinity monoclonal antibodies to GPC3. (A) Schematic structure of GPC3. The human GPC3 gene encodes a 70 kDa precursor protein of 580 amino acids. In the mature form at the cell membrane, a GPI anchor replaces the C-terminal signal (predicted cleavage site: S560). The core protein is held together by cysteine residues forming disulfide bridges (green). Heparan sulfate (HS) chains (orange) attached to the polypeptide core. Immunized peptide corresponding to the C-terminal end of matured GPC3 (Residues: 511–560). (B) High-throughput flow cytometry screening. Representative histograms obtained with positive (top panel) and negative supernatant (bottom panel). Each hybridoma was tested for GPC3-specific mAb production by a simultaneous binding assay incorporating a 1:1 mixture of antigen-positive A431/G1 cells and antigen-negative A431 cells in flow cytomtery analysis. (C) Flow cytometry analysis of five selected mouse hybridoma clones (YP6, YP7, YP8, YP9 and YP9.1) (solid lines) and 1G12, a control anti-GPC3 mAb (dotted lines, in the middle) using A431/G1 and Hep3B. Filled peak: isotype control. (D) ELISA analysis of five hybridoma clones on recombinant GPC3 proteins. GPC3: recombinant GPC3-human Fc; GPC3ΔHS: recombinant GPC3 without HS; control: BSA. 1G12: a commercial anti-GPC3 mAb. (E) western blot analysis of YP7 and YP9. Thirty μg of whole cell lysates of HepG2, Hep3B, A431/G1 (A431.GPC3+) and A431 was loaded and probed with each hybridoma supernatant.
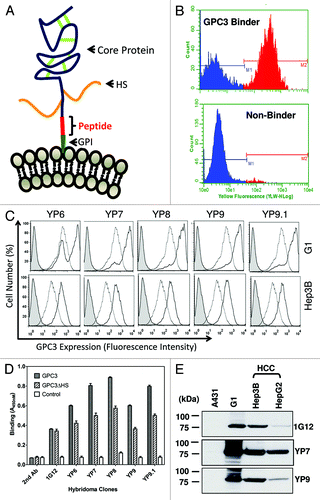
The C-terminal subunit of GPC3 is approximately 30 kDa in length. Based on protein sequence using big-PI Predictor (http://mendel.imp.ac.at/sat/gpi/gpi_server.html), we predicted serine 560 as a cleavage site for GPI.Citation5 We synthesized a 50-mer peptide (residues 511 – 560) corresponding to the C-terminal end of cell-surface GPC3 allowing for recognition of each isoform. An additional cysteine residue was introduced to the N-terminus of the peptide. Therefore, mAbs raised against this peptide presumably bind the C-terminal end of membrane-bound GPC3.
Screening of monoclonal antibodies to cell surface-associated GPC3
Five BALB/c mice were immunized three times and boosted with peptide-KLH conjugate. Serum antibody titer to native conformation of GPC3 on the cell surface was measured by flow cytometry using GPC3-expressing cells as described in Materials and Methods. Antibody titers for all mice were > 10,000 after three immunizations, observed as non-overlapped stained cell peaks at a 1:10,000 dilution of sera along with peaks of non-stained cells. Spleen cell fusions were conducted with the two best mice.
To perform primary screening of ten 96-well plates of mAbs that recognize cell surface-associated GPC3 protein, we developed a flow cytometry-based assay in which a mixture of GPC3-negative cells (A431) and GPC3-positive cells (G1 or A431/G1, A431 stably transfected with GPC3) were used as the antigen to be tested for each supernatant sample (). The use of this rapid screening method made it possible to screen 960 clones on the Guava EasyCyte flow cytometer within a 24-h period. Clones with binding ratios (G1/A431) of 15 or more were selected for flow cytometry analysis using HepG2 cells. Twelve clones were subcloned by limited dilution. Among them, five (YP6, YP7, YP8, YP9 and YP9.1) were recovered and isotyped as IgG1 (γ1κ). It should be noted that mAbs with the highest binding signals for the peptide in a standard ELISA have very weak or no binding to cell-surface associated GPC3 (data not shown). Taken together, the new method successfully isolated mAbs with high binding affinity for cell surface GPC3.
Affinity and specificity of GPC3 mAbs on cells
The five selected mAbs (YP6, YP7, YP8, YP9 and YP9.1) were analyzed for binding using four cell lines (A431, A431/G1, HepG2 and Hep3B) at 1 μg/ml. As shown in , all bound to two GPC3-expressing cell lines (A431/G1 and Hep3B). Conversely, none bound to the GPC3-negative A431 cell line (data not shown), suggesting that it is GPC3-specific and that binding for cell surface GPC3 was generally higher than 1G12. We then tested for reactivity with recombinant GPC3 and GPC3ΔHS (a mutant without heparan sulfate) proteins in ELISA. Interestingly, we saw stronger binding signals on wild-type GPC3 compared with GPC3ΔHS, the GPC3 core protein alone, while 1G12 bound both similarly (). This result suggests that these new mAbs recognize the native form of GPC3 protein with heparan sulfate on the cell surface.
shows a western blot analysis indicating strong immunoreactivity of YP7 and YP9 with recombinant GPC3 proteins expressed in the A431/G1 cell line and no reactivity with other cellular proteins in the A431 cell line lysate. These mAbs also reacted with endogenous GPC3 proteins in HCC cell lines to various extents. Among those tested (including 1G12), YP7 showed strongest reactivity with GPC3 proteins in both HCC cell lines (HepG2 and Hep3B) and was selected for further analyses using a panel of HCC and CCA cell lines.
As shown in , YP7 binds all HCC cell lines (HepG2, Hep3B, Huh-4 and Huh-7) in flow cytometry analysis. In contrast, YP7 did not react with the SK-Hep-1 cell line (data not shown), originally reported as a HCC cell line but recently redefined as a non-HCC cell line.Citation13 also shows that YP7 did not bind any of the CCA lines tested (HuCCT1, KMBC, KMCH and Mz-ChA-1). The high binding affinity of YP7, subnanomolar (KD = 0.3 nM), was determined with the native form of GPC3 protein expressed on cells (). Six glypicans (GPC1–6) have been identified in mammals sharing a characteristic structure.Citation4 To evaluate whether YP7 is cross-reactive with other glypicans, we tested GPC1, GPC5, and GPC3 via ELISA, and found that YP7 bound GPC3, not GPC1 or GPC5 as shown in .
Figure 2. Characterization of YP7 binding properties. (A) Flow cytometry analysis using a panel of endogenous GPC3–expressed human cell lines. Four HCC (HepG2, Hep3B, Huh4, Huh7), four CCA (HuCCT1, KMBC, KMCH and Mz-ChA-1), one ovarian CCC (TOV-21G) and one melanoma (UACC-62) cell lines were shown. Filled peak: isotype control. (B) Binding affinity measurement of YP7 against cell surface-associated GPC3. MFI: mean fluorescence intensity. The Scatchard plot and the KD value were calculated using Prism. (C) Binding of YP7 on recombinant GPC1, GPC3 and GPC5 proteins.
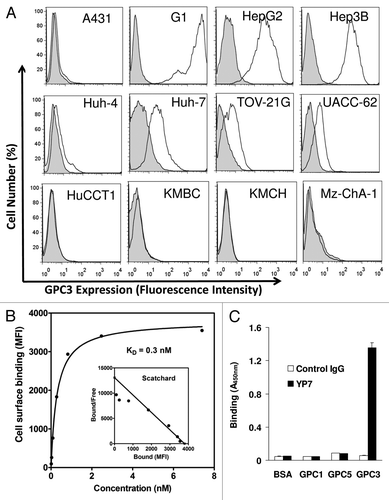
To evaluate the binding specificity of YP7 on liver cancer cells, we performed immunoblotting on a panel of HCC and CCA cells (). YP7 showed strong GPC3 signals in five (HepG2, Hep3B, Huh-1, Huh-4 and Huh-7) out of five (100%) HCC cell lines. SK-Hep-1 was GPC3-negative. No GPC3 protein was detected in any of the four CCA cell lines (KMCH, KMBC, Mz-ChA-1 and Oz). The reactivity profile of YP7 in western blotting matched well with flow cytometry data (), suggesting that YP7 specifically and equally detects endogenous GPC3 proteins. Interestingly, YP7 detected GPC3 expressed in TOV-21G, an ovarian clear cell carcinoma (CCC), while 1G12 did not clearly detect it (). The difficulty of 1G12 has been previously reported and attributed to the very low expression level of GPC3 in TOV-21G cells,Citation11 thus depicting the usefulness of a high affinity mAb over 1G12. Together, our results demonstrate that YP7 has excellent binding affinity and specificity for the native form of GPC3 in HCC and other GPC3-expressing cancers (e.g., ovarian CCC), and is able to detect endogenous GPC3 at a very low expression level.
Figure 3. GPC3 protein expression in liver cancer. (A) GPC3 expression in liver cancer cell lines detected in western blotting. HCC: hepatocellular carcinoma; CCA: cholangiocarcinoma. 1G12: a commercial GPC3 mAb. Mz: Mz-ChA-1; SK: SK-Hep-1. (B) western blots of ovarian clear cell carcinoma (CCC). TOV-21G: ovarian CCC. (C) Soluble GPC3 protein immunoprecipitated from culture supernatant and analyzed by western blotting. YP7 was used to pull down soluble GPC3 protein in culture supernatant. H: mouse IgG heavy chain; L: mouse IgG light chain. C-terminus: C-terminal subunit (~30 kDa). IP: immunoprecipitation. (D) GPC3 expression analyzed by western blotting in nine HCC and nine CCA primary liver tumor tissues. (E) Immunohistochemistry of GPC3 in HCC tissues. Circles identify tumor nests (a, c) with or without distinct GPC3 positivity at a low magnification. Arrows identify tumor (b, d) with or without GPC3 positivity at a higher magnification. a, c: 100X. b and d: a higher magnification (400X) of a and c, respectively.
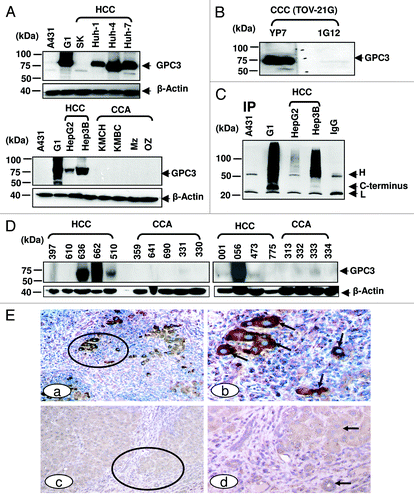
Detection of soluble and tissue GPC3 proteins by YP7
Previous studies showed the existence of soluble GPC3 protein in culture supernatant and patient serum.Citation2,Citation12 To investigate its diagnostic potential, we used YP7 to pull down and probe soluble protein in culture supernatant from four cell lines (A431, A431/G1, HepG2, and Hep3B) (). In A431/G1 and Hep3B pull down proteins, soluble GPC3 proteins were detected as smeared bands indicating a wide range of high molecular weight proteins corresponding to GPC3 proteins modified with varying numbers of heparan sulfate chains.Citation2,Citation3,Citation12 Interestingly, in addition to GPC3 with heparan sulfate (smear), a 30 kDa band was detected in both culture supernatants, indicating that the C-terminal subunit alone was present in soluble GPC3 proteins.
To evaluate YP7 for immunohistochemical applications, nine HCC and nine CCA patient tissues were used for western blotting.Citation13 High GPC3 protein expression was found in four out of nine (44%) HCC tissues as bands with an expected size of ~70kDa. GPC3 expression was not found in any of the nine (0%) CCA tissues ().
Previous studies showed GPC3 expression in other cancers such as ovarian clear cell carcinoma, melanoma and non-small lung adenocarcinoma, although the expression profiling was generally not convincing.Citation4 We tested YP7 on all of the GPC3-positive cell lines in the NCI-60 set based on public microarray data (http://dtp.nci.nih.gov/mtweb/search.jsp) () and found that GPC3 protein expression in non-HCC cancer cells was consistent, cell surface expression in non-HCC cells was in general lower than that of HCC cells. Interestingly, YP7 bound UACC-62, a human melanoma cell line. GPC3 has been suggested as a potential therapeutic target for melanoma.Citation1,Citation4 However, a cell line model for GPC3-expressing melanoma has not been established and GPC3-targeted antibody therapy against melanoma has not been investigated due partly to the lack of a good cell model and a high-affinity anti-GPC3 mAb. Our data indicate that UACC-62 may be a useful model to study GPC3 in melanoma and that YP may be a suitable antibody candidate for melanoma therapy.
Table 1. Characterization of GPC3 expression in non-HCC in the NCI-60 set
We also examined the binding of YP7 on human liver tissues by immunohistochemistry (IHC). As shown in , invasive HCC showed distinct membrane or cytoplasmic expression of GPC3, whereas normal cells lacked distinct immunostaining (). Our observation supports the belief that GPC3 stained by YP7 may be a suitable marker for IHC of liver tumors, particularly HCC. Together, western blot analysis of HCC cells and tissues shows that YP7 is highly specific and sensitive for endogenous GPC3 proteins in both cell-surface form and soluble (shed) form, suggesting potential as a diagnostic agent to evaluate serum GPC3 protein and GPC3 expression in HCC tissues.
Antitumor activity of YP7
To explore YP7 as a potential cancer therapeutic, we examined tumor growth inhibition in HCC mice xenografts by using HepG2 cells to establish a nude mice model (). When tumors reached an average volume of 100 mm3 on day 7, mice were administered 5 mg/kg of YP7 twice a week for two weeks. YP7 significantly inhibited the growth of the HepG2 tumor as measured with nearly constant tumor volume values during the two-week period, whereas tumors inoculated to non-treated mice continued to rapidly grow during this period. Therefore, YP7 exhibited strong antitumor activity against HepG2 xenografts in mice, although the precise mechanism of growth inhibition needs to be examined in future studies.
Figure 4. Anti-tumor activity of YP7 in the HCC xenograft model. (A) BALB/c nu/nu mice s.c. inoculated with HepG2 cells. When tumors reached an average volume of 100 mm3, mice were administered 5 mg/kg of YP7 twice a week for two and a half weeks. Arrow: YP7 injection. *p < 0.05 (B) Representative mice bearing HCC tumors in treated (YP7) and untreated (vehicle) groups.
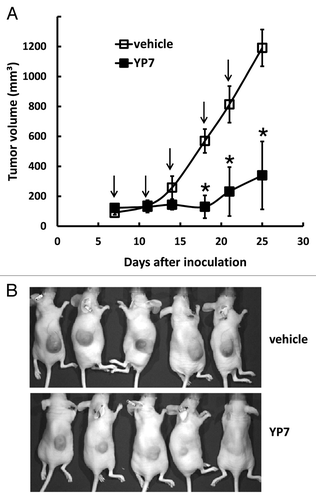
Discussion
In the present study, we generated high-affinity mAbs to GPC3 that were particularly specific for GPC3-expressing tumor cells and tissues. Interestingly, YP7 detected low expression levels of GPC3 in HCC (e.g., HepG2 and Huh-4), ovarian clear cell carcinoma (e.g., TOV-21G), and melanoma cells (e.g., UACC-62). Our results show that it may be a very promising candidate for cancer diagnostics and therapy targeting GPC3-expressing tumor cells.
Hybridoma technology for mAb production has been well-established during the past 37 y,Citation14 with 11 mAbs approved for cancer therapy since the debut of rituximab.Citation15 However, a major obstacle in high-throughput development of therapeutic antibodies involves the feasibility of screening hundreds to thousands of hybridoma supernatants within a short time frame (24–48 h).Citation16 While an ELISA is a suitable method for isolating mAbs specific for the peptide backbone or partially denatured protein antigen, it is not always practical for picking up mAbs that recognize the native form of antigens on cancer cells.Citation17 To assess antibody reactivity with intact cell-associated antigens, a flow cytometry method is ideal, although traditional instruments are not designed for high-throughput assays. In light of this, we used the Guava EasyCyte system, a flow cytometer with 96-well plates and an autosampler during initial screenings. A potential drawback for cell-based screening includes artifacts due to non-desired mAbs reacting with non-target cellular components displayed by cells. We excluded such clones by simultaneously evaluating reactivity with GPC3-negative and GPC3-positive cells. It is conceivable that the method described here can be applied to isolate other cell-surface antigens in cancer cells.
In the present study, we found that YP7 was able to pull down native soluble GPC3 from HCC culture supernatant, indicating that YP7 could be used as a novel reagent to detect serum GPC3 in cancer patients. To explore this application in cancer diagnostics, we will need two different mAbs to establish a sensitive method such as sandwich ELISA. To this end, we will isolate a high-affinity anti-GPC3 mAb recognizing an epitope different from the YP7 site.
We demonstrated that the YP7 antibody exhibited significant HCC xenograft tumor growth inhibition in nude mice. To investigate whether YP7 had any inhibitory effect on HCC cell proliferation, we tested YP7 in three HCC cell lines (Hep3B, HepG2 and Huh-7) in vitro by WST-8 assay and found that YP7 did not inhibit cell proliferation in concentrations ranging from 0.1 to 2.5 μM (data not shown). We assume that the anti-tumor efficacy we found in mice treated with YP7 may be partly due to its Fc-mediated antibody-dependent cellular cytotoxicity (ADCC) and complement-dependent cytotoxicity (CDC). To explore its therapeutic potential, we are humanizing the YP7 antibody. We will analyze ADCC and CDC activities using the humanized version of YP7.
We believe that these new mAbs have significant potential for GPC3-expressing cancer treatment and diagnosis. Humanization and development of antibody toxin/drug conjugates may develop them for cancer therapy.Citation18,Citation19 Immunoconjugates such as immunotoxins generally require very high-affinity Fv for toxin delivery because patients are treated with considerably smaller doses and fewer cycles than whole IgG antibodies alone to avoid toxin-mediated side effects.Citation19 Furthermore, the antibody Fvs can be applied in chimeric antigen receptor (CAR)-T cell and antibody Fv-based gene therapy. Our mAbs may also have utility in diagnostics such as measurement of serum GPC3, IHC for cancer detection and treatment follow-up, in vivo tumor imaging, and isolation of circulating tumor cells.
Materials and Methods
Cell lines
Six human HCC cell lines (SK-Hep1, HepG2, Hep3B, Huh-1, Huh-4, and Huh-7) were obtained from Xin-wei Wang at the National Cancer Institute (NCI), Bethesda, Maryland. Five human CCA lines (HuCCT1, OZ, Mz-ChA-1, KMBC, and KMCH) were obtained from Gregory J. Gores from the Mayo Clinic, Rochester, Minnesota. TOV-21G (human ovarian clear cell carcinoma cell line) was obtained from the American Type Culture Collection, Manassas, Virginia. A431/G1 is a transfected A431 human epithelial carcinoma cell line produced in our lab that stably expresses human GPC3. The cell lines were cultured as described.Citation13
Monoclonal antibody production
Mouse hybridoma technology included peptide synthesis, mice immunization, spleen cell fusion, hybridoma selection and expansion, and supernatant screening using peptide or protein.Citation20 In brief, a peptide consisting of 50 residues, DGMIKVKNQLRFLAELAYDLDVDDAPGNSQQATPKDNEISTFHNLGNVHS, was synthesized (GenScript USA, Piscataway, NJ) and conjugated to a carrier protein, keyhole limpet hemocyanin (KLH), by maleimide chemistry via a cysteine residue introduced at the N-terminal. Five BALB/c mice were immunized, followed by test bleeds after second and third immunizations, with two fusions performed on mice showing the highest antibody titers (> 10,000 on GPC3-expressing cancer cells). Hybridoma cells were screened via flow cytometry (see below). The most positive five clones were selected for subcloning, determined with immunoglobulin (Ig) isotype, and further expanded. The YP7 clone demonstrating the highest affinity and most specific binding was chosen for purification via ascites production in BALB/c mice and protein A purification.Citation20
High-throughput flow cytometry
Cells were incubated with mouse hybridoma supernatant containing 1 μg/mL of each mAb. Binding was detected with goat anti-mouse IgG conjugated with phycoerythrin (PE) (Sigma-Aldrich, St. Louis, MO). The fluorescence associated with live cells was measured using a FACSCalibur (BD Biosciences, San Jose, CA). For screening of hybridomas, a 1:1 mixture of A431 and A431/G1 cells (10,000 cells per well in 96-well plates) were incubated with a 1:5 dilution of mouse hybridoma supernatant. Binding was detected with goat anti-mouse IgG conjugated with PE (Sigma-Aldrich, St. Louis, MO). Fluorescence was measured using Guava EasyCyte Plus instrument equipped with a built-in autosampler (Millipore, Billerica, MA). We collected 5000 events per sample for analysis. Each peak, either the M1 gate (antigen negative) or the M2 gate (antigen positive), included approximately 2500 events. Due to the fact that we selected only live cells for analysis to avoid potential non-specific autofluorecence from dead cells and our screening of ten 96-well plates took over ten hours, actual live cell counts in each peak (as shown in ) were less than 2500. In some cases, the two peaks were not equal. The smaller peak for the M1 gate in the histogram of the GPC3 binder () indicated relatively low cell viability of GPC3 negative cell population in that sample. However, we found that cell viability did not affect our selection which was based only on live cells. Geomean values for A431/G1 and A431 were measured simultaneously for individual clones using Guava Express Pro Software. Clones with binding ratios of 15 or more were considered specific to cell-associated GPC3 protein and selected for further flow cytometry using HepG2 cells.
The Scatchard plot and the KD value were calculated using Prism (GraphPad Software Inc., San Diego, CA) as previously described.Citation21
Enzyme-linked Immunosorbent Assay (ELISA)
Nunc MaxiSorp 96-well flat-bottom plates were incubated overnight with 1 μg/ml of wild-type or mutant GPC3 (GPC3ΔHS) protein in PBS at 4°C followed by blocking with SuperBlock buffer (Thermo Fisher Scientific Inc., Rockford, IL). Recombinant GPC3 proteins were made,Citation22 and recombinant GPC1 and GPC5 proteins were purchased (R&D Systems, Minneapolis, MN). Mouse sera were diluted 1:5 in PBST buffer (0.05% Tween 20) and incubated in the same plate for 1 h at room temperature. To detect bound antibodies, a 1:10,000 dilution of goat anti-mouse IgG conjugated with horseradish peroxidase (HRP, Invitrogen, Carlsbad, CA) was added. Assays were developed as described.Citation21
Immunoprecipitation and western blot analysis
Supernatants of cultured cells were incubated with 1 μg/mL of purified mouse hybridoma antibody or a mouse monoclonal IgG control rotated overnight at 4°C. Fresh protein A beads (Roche, Mannheim, Germany) were washed in advance with immunoprecipitation assay buffer containing 2% SDS and protease inhibitors (“Complete Mini-EDTA Free” protease inhibitor tablet, Roche, Mannheim, Germany) three times. Beads were incubated with sample mixtures and rotated for 2 h at 4°C, washed three times, incubated with a solution containing loading buffer and immunoprecipitation assay buffer, and reduced at 99°C for 5 min. Supernatants were separated by SDS-PAGE followed by western blot using a standard method.Citation13
Frozen and fixed liver tumor samples were acquired from the Cooperative Human Tissue Network (Charlottesville, VA).Citation13 western blots were performed.
Immunohistochemistry
Formalin-fixed and paraffin-embedded tissue sections were subjected to immunohistochemical staining using 6 μg/mL of YP7 following our previously published protocol.Citation23 Immunostaining was performed as previously described with YP7 and an isotype control mouse IgG (Southern Biotech, Birmingham, AL). Immunostained sections were independently evaluated by two investigators.
Animal testing
All mice were housed and treated under the protocol approved by the Institutional Animal Care and Use Committee at the National Institutes of Health (NIH). We performed two rounds of in vivo passaging for the HepG2 cell line before animal testing. Ten million cells were suspended in 200 μl of PBS and inoculated subcutaneously into 4 to 6 week-old female BALB/c nu/nu nude mice (NCI-Frederick, Frederick, MD). When the tumor volume reached approximately 100 mm3 (V = ab2/2, where a and b represent tumor length and width, respectively), mice were intravenously injected with 5 mg/kg of YP7 twice a week for two and a half weeks.
Abbreviations: | ||
HCC | = | hepatocellular carcinoma |
CCA | = | cholangiocarcinoma |
GPC | = | glypican |
GPI | = | glycosylphosphatidylinositol |
mAb | = | monoclonal antibody |
NCI | = | National Cancer Institute |
Ig | = | immunoglobulin |
PE | = | phycoerythrin |
HRP | = | horseradish peroxidase |
ELISA | = | enzyme-linked immunosorbent assay |
KLH | = | keyhole limpet hemocyanin |
HS | = | heparan sulfate |
MFI | = | mean fluorescence intensity |
IP | = | immunoprecipitation |
IHC | = | immunohistochemistry |
CAR | = | chimeric antigen receptor |
Acknowledgments
This study was supported by the Intramural Research Program of the National Institutes of Health, National Cancer Institute (NCI), Center for Cancer Research. Satoshi Nagata was supported by a NIH COBRE grant (1P20RR024219–01A2). We thank our colleagues Heungnam Kim for establishing the A431/G1 cell line, Mingqian Feng and Ruoqi Wang for producing recombinant GPC3 and GPC3ΔHS proteins, and the NIH Fellows Editorial Board for editorial assistance.
Disclosure of Potential Conflicts of Interest
No potential conflicts of interest were disclosed.
References
- Ho M. Advances in liver cancer antibody therapies: a focus on glypican-3 and mesothelin. BioDrugs 2011; 25:275 - 84; http://dx.doi.org/10.2165/11595360-000000000-00000; PMID: 21942912
- Capurro M, Wanless IR, Sherman M, Deboer G, Shi W, Miyoshi E, et al. Glypican-3: a novel serum and histochemical marker for hepatocellular carcinoma. Gastroenterology 2003; 125:89 - 97; http://dx.doi.org/10.1016/S0016-5085(03)00689-9; PMID: 12851874
- Capurro M, Filmus J. Glypican-3 as a serum marker for hepatocellular carcinoma. Cancer Res 2005; 65:372 - , author reply 372-3; PMID: 15665316
- Ho M, Kim H. Glypican-3: a new target for cancer immunotherapy. Eur J Cancer 2011; 47:333 - 8; http://dx.doi.org/10.1016/j.ejca.2010.10.024; PMID: 21112773
- Allegretta M, Filmus J. Therapeutic potential of targeting glypican-3 in hepatocellular carcinoma. Anticancer Agents Med Chem 2011; 11:543 - 8; PMID: 21554204
- Ishiguro T, Sugimoto M, Kinoshita Y, Miyazaki Y, Nakano K, Tsunoda H, et al. Anti-glypican 3 antibody as a potential antitumor agent for human liver cancer. Cancer Res 2008; 68:9832 - 8; http://dx.doi.org/10.1158/0008-5472.CAN-08-1973; PMID: 19047163
- Nakano K, Orita T, Nezu J, Yoshino T, Ohizumi I, Sugimoto M, et al. Anti-glypican 3 antibodies cause ADCC against human hepatocellular carcinoma cells. Biochem Biophys Res Commun 2009; 378:279 - 84; http://dx.doi.org/10.1016/j.bbrc.2008.11.033; PMID: 19022220
- Nakano K, Ishiguro T, Konishi H, Tanaka M, Sugimoto M, Sugo I, et al. Generation of a humanized anti-glypican 3 antibody by CDR grafting and stability optimization. Anticancer Drugs 2010; 21:907 - 16; http://dx.doi.org/10.1097/CAD.0b013e32833f5d68; PMID: 20847643
- Nakatsura T, Komori H, Kubo T, Yoshitake Y, Senju S, Katagiri T, et al. Mouse homologue of a novel human oncofetal antigen, glypican-3, evokes T-cell-mediated tumor rejection without autoimmune reactions in mice. Clin Cancer Res 2004; 10:8630 - 40; http://dx.doi.org/10.1158/1078-0432.CCR-04-1177; PMID: 15623647
- Komori H, Nakatsura T, Senju S, Yoshitake Y, Motomura Y, Ikuta Y, et al. Identification of HLA-A2- or HLA-A24-restricted CTL epitopes possibly useful for glypican-3-specific immunotherapy of hepatocellular carcinoma. Clin Cancer Res 2006; 12:2689 - 97; http://dx.doi.org/10.1158/1078-0432.CCR-05-2267; PMID: 16675560
- Suzuki S, Yoshikawa T, Hirosawa T, Shibata K, Kikkawa F, Akatsuka Y, et al. Glypican-3 could be an effective target for immunotherapy combined with chemotherapy against ovarian clear cell carcinoma. Cancer Sci 2011; 102:1622 - 9; http://dx.doi.org/10.1111/j.1349-7006.2011.02003.x; PMID: 21668581
- Hippo Y, Watanabe K, Watanabe A, Midorikawa Y, Yamamoto S, Ihara S, et al. Identification of soluble NH2-terminal fragment of glypican-3 as a serological marker for early-stage hepatocellular carcinoma. Cancer Res 2004; 64:2418 - 23; http://dx.doi.org/10.1158/0008-5472.CAN-03-2191; PMID: 15059894
- Yu L, Feng M, Kim H, Phung Y, Kleiner DE, Gores GJ, et al. Mesothelin as a potential therapeutic target in human cholangiocarcinoma. J Cancer 2010; 1:141 - 9; http://dx.doi.org/10.7150/jca.1.141; PMID: 20922056
- Köhler G, Milstein C. Continuous cultures of fused cells secreting antibody of predefined specificity. Nature 1975; 256:495 - 7; http://dx.doi.org/10.1038/256495a0; PMID: 1172191
- Shuptrine CW, Surana R, Weiner LM. Monoclonal antibodies for the treatment of cancer. Semin Cancer Biol 2012; 22:3 - 13; http://dx.doi.org/10.1016/j.semcancer.2011.12.009; PMID: 22245472
- Chiarella P, Fazio VM. Mouse monoclonal antibodies in biological research: strategies for high-throughput production. Biotechnol Lett 2008; 30:1303 - 10; http://dx.doi.org/10.1007/s10529-008-9706-5; PMID: 18418716
- Yokoyama WM, Christensen M, Santos GD, Miller D. Production of monoclonal antibodies. Curr Protoc Immunol 2006; Chapter 2:2 - , 5; PMID: 18432969
- Getts DR, Getts MT, McCarthy DP, Chastain EM, Miller SD. Have we overestimated the benefit of human(ized) antibodies?. MAbs 2010; 2:682 - 94; http://dx.doi.org/10.4161/mabs.2.6.13601; PMID: 20935511
- Pastan I, Hassan R, Fitzgerald DJ, Kreitman RJ. Immunotoxin therapy of cancer. Nat Rev Cancer 2006; 6:559 - 65; http://dx.doi.org/10.1038/nrc1891; PMID: 16794638
- Harlow E, Lane D. Antibodies: a laboratory manual, Cold Spring Harbor, NY; Cold Spring Harbor Laboratory, 1988.
- Ho M, Kreitman RJ, Onda M, Pastan I. In vitro antibody evolution targeting germline hot spots to increase activity of an anti-CD22 immunotoxin. J Biol Chem 2005; 280:607 - 17; PMID: 15491997
- Feng M, Kim H, Phung Y, Ho M. Recombinant soluble glypican 3 protein inhibits the growth of hepatocellular carcinoma in vitro. Int J Cancer 2011; 128:2246 - 7; http://dx.doi.org/10.1002/ijc.25549; PMID: 20617511
- Man YG, Tavassoli FA. A simple epitope retrieval method without the use of microwave oven or enzyme digestion. Appl Immunohistochem 1996; 4:139 - 41