Abstract
Characterization of aggregation profiles of monoclonal antibodies (mAb) is gaining importance because an increasing number of mAb-based therapeutics are entering clinical studies and gaining marketing approval. To develop a successful formulation, it is imperative to identify the critical biochemical properties of each potential mAb drug candidate. We investigated the conformational change and aggregation of a human IgG1 using external dye-binding experiments with fluorescence spectroscopy and compared the aggregation profiles obtained to the results of size-exclusion chromatography. We show that using an appropriate dye at selected mAb concentration, unfolding or aggregation can be studied. In addition, dye-binding experiments may be used as conventional assays to study therapeutic mAb stability.
Monoclonal antibodies (mAbs) have emerged as a novel class of protein drugs and are utilized for a variety of mostly incurable and debilitating diseases such as cancer and rheumatoid arthritis.Citation1–Citation4 For treatment of chronic diseases, it is desirable for these drugs to be administered subcutaneously, in which case high protein concentrations (>100 mg/mL) are generally needed.Citation5,Citation6 Protein-based drugs containing mAbs must contain minimum amounts of aggregation and fragmentation and conserve their structural integrity during storage because degraded or aggregated protein may induce immunogenicity or reduce efficacy. Currently, size-exclusion chromatography-high performance liquid chromatography (SEC-HPLC) is the most commonly used method to characterize mAb aggregation profiles;Citation7 however it is time consuming, expensive and requires expertise. SEC-HPLC cannot be used to obtain accurate biophysical profiles of mAbs at high concentrations because dilution during the experiment might lead to reversible aggregation. Furthermore, the potential interaction of aggregates with surfaces, e.g., needle, tubing, column, will lead to the loss of sample and thus an inaccurate analysis.Citation8,Citation9 Additional drawbacks of the technique are that different conformations such as partially unfolded monomers also cannot be distinguished by SEC-HPLC and large aggregates may be totally excluded during the injection into the column.
External dye binding assays have been used to characterize protein stability and aggregation,Citation10–Citation12 and studies involving biopharmaceuticals have been reported recently, e.g., for thermostability screeningCitation10 and detection of aggregation.Citation11–Citation14 These methods are not limited by protein quantity and are more sensitive because they are fluorescence-based. We studied the accelerated unfolding of an IgG1 mAb with the hydrophobic dye 1-anilino-8-naphthale-nesulfonate (ANS), and its accelerated aggregation with aggregate specific Thioflavin T (ThT). We have also conducted accelerated aggregation studies with SEC-HPLC7 and compared the findings to the ThT binding results. We hypothesize that key structures formed during mAb aggregation can be probed selectively by the appropriate dyes () with specific mAb concentrations.
Conformational Change of mAb
Depending on the stress level, irreversible or partial unfolding of mAbs can occur. Unfolded proteins can be formed at high temperatures, thereby exposing hydrophobic patches that are usually buried in the native protein. Each mAb domain has different stability;Citation15 nevertheless, for simplicity, we hypothesize that there is one unfolding species. We monitored conformational changes of the mAb as a function of temperature with the change of ANS fluorescence at low protein concentrations. The fluorescence of ANS first increased, and then shifted to lower wavelengths upon binding to the protein. The fluorescence change is directly proportional to the degree of protein unfolding.Citation16–Citation19
The calculated equilibrium ANS binding constant to the native mAb is KANS = 0.0025 ± 0.0017 M−1 by the double reciprocal method ( and Sup. Fig. 1).Citation20 This binding constant is about 10-fold less than the binding constants for proteins in the “molten globule” state,Citation17 indicating the structural stability of the mAb. It is also more than those found for smaller proteins in size,Citation21,Citation22 showing good correlation with the mAb size.
We also measured kinetic rates of the conformational change of monomer at the elevated temperatures () with an empirical sigmoid function fit from the ANS fluorescence change (see Supporting Information) (). The mAb was incubated at elevated temperatures (63–70°C) at 0.2 mg/mL concentrations up to 6 h and 20 µM ANS was added immediately after the incubation, kept in ice water for 2 h. The aggregate levels in these biopharmaceuticals are generally very low during long-term storage conditions even at high protein concentrations. We tested the aggregate levels at different mAb concentrations and temperatures with SEC and reported the results elsewhere in reference Citation7. At concentrations below 5 mg/mL, we did not observe aggregate formation during incubation at elevated temperatures (Sup. Fig. 2) because of the high stability of these proteins. Hence, in order to detect aggregate formation, we used very high mAb concentrations (e.g., 150 mg/mL). Nevertheless, the observed aggregate levels almost never exceeded a few percents since we stressed the mAb gently and always used incubation temperatures 10–15°C below the melting temperature of the Fab domain. Lastly, ANS can also bind to aggregates as well as partially unfolded monomers, albeit with different affinity. However, since we do not observe aggregate formation at 0.2 mg/mL concentration (Sup. Fig. 2), we hypothesize that ANS probes mAb unfolding preferentially by interacting with the partially unfolded protein either via exposed hydrophobic surfaces or via electrostatic interactions or both.
Aggregation of mAb
The second step shown in depicts the aggregate formation. Aggregates were not separated in dye-binding experiments; one type of aggregate was assumed to represent the soluble irreversible aggregates. The second step in thus includes all the protein aggregates, referred to as “aggregated mAb.” We probed the aggregation step by ThT binding and the data was also analyzed with sigmoid equation (Supporting Information). ThT recognizes certain features of the amyloid-type protein aggregates, e.g., β-sheets and the surfaces formed by tyrosine residues and between the protofilaments, with nonspecific interactions.Citation23 ThT goes through ground-state spectral changes that influence the absorbance spectrum, but not the emission spectrum when it binds to aggregates. Furthermore, aggregate formation is detected by the increase in the fluorescence intensity. It is also known that local secondary structure of the protein is predominantly preserved in these aggregates, while the tertiary structure is lost.Citation24,Citation25
When incubated at 150 mg/mL, the SEC-HPLC data indicated that the mAb existed primarily either in the monomer or aggregate form (Sup. Fig. 3). Since the native mAb structure is extensively β-sheet, residual ThT binding to monomer mAb was also observed. However, the binding rate constant was 0.069 min−1 when mAb was incubated at concentration of 0.2 mg/mL at 68°C and 0.667 min−1 when incubated at 150 mg/mL, suggesting that ThT binds more favorably to aggregates compared with monomers. The unbound ThT is also not considerably fluorescent compared to bound ThT. Moreover, the level of protein aggregation was relatively excessive; we assumed that ThT fluorescence change mainly represented the aggregation kinetics (). ThT binding experiments were conducted via aggregating the protein first at 150 mg/mL mAb concentration, and then diluting the sample to the total final concentration of 0.2 mg/mL prior to ThT addition. We recently showed that the mAb aggregates were irreversible, had different sizes, and mainly contained partially unfolded protein.Citation7 Therefore, once the mAb forms aggregates at 150 mg/mL, by dilution only the amount of protein (aggregate or monomer) available in the solution is reduced. Lastly, aggregates resemble amyloids in terms of containing rich beta motifs. We hypothesize that this is probably the main reason why ThT binds to mAb aggregates with high affinity.
The Arrhenius plot shows that the activation energy for protein unfolding calculated by the ANS binding is ∼610 kJ/mol (). This relatively high activation energy required for the unfolding suggests that the mAb is very stable in its native form, which correlated well with having a TmFab of 80°C (DSC data not shown). Furthermore, the calculated activation energy for the observed protein aggregation with ThT binding is ∼540 kJ/mol, which is equivalent (within experimental error) to the energy required for the conformational change calculated from the ANS binding experiments. It is also significant to note that multidomain proteins such as mAbs usually aggregate with the non-Arrhenius kinetics over a wide temperature range.Citation7,Citation26 However, over small temperature ranges, even the non-Arrhenius kinetics could be modeled well with Arrhenius kinetics.Citation27 Therefore, Arrhenius kinetics is applicable for the short temperature ramp from 63–70°C for the dye-binding experiments. shows that the activation energy of the protein aggregation recovered by the ThT binding method is in excellent agreement with the values calculated from the SEC-HPLC experiments. Similar activation energies indicate that at the high incubation temperatures, mAb unfolding governs aggregation ().
In conclusion, we demonstrated that external dye-binding assays are rapid, sensitive, require minimal mAb amounts, and thus, easily adapted for therapeutic mAb unfolding and aggregation studies. ANS binding results indicate that the kinetics of the mAb unfolding is similar to that of other globular proteins, but with relatively high stability. Furthermore, the ThT binding illustrates that mAb aggregation adopts a similar aggregation profile to that of amyloidal proteins, where a considerable increase in ThT fluorescence is observed. Due to the same solution conditions and sample preparations, we believe that the aggregates formed are identical in the ThT binding and the SEC experiments. Having said that, it is quite challenging to deduce a detailed aggregation mechanism with ThT binding. Hence, we presented only a simple aggregation schematic to indicate that we probed unfolding with ANS and aggregate formation with ThT, owing largely to the strict control of mAb concentrations during incubation at elevated temperatures. Nevertheless, since ThT binds to mAb aggregates with high affinity, we hypothesize that these aggregates resemble amyloids. Dye-binding assays could also be used for the candidate ranking to find the most stable mAb among potential therapeutic candidates. In addition, these assays could could be used in formulation ranking (Kayser et al. unpublished results), where the conformation and stability of the same mAb can differ significantly in each formulation. Dye-binding studies reveal more specific information about the structure and stability of the protein compared to chromatographic methods. Dye-binding assays could also be employed to elucidate the unfolding and aggregation behavior of biological protein drugs other than mAbs.
Disclosure of Potential Conflicts of Interest
No potential conflicts of interest were disclosed.
Figures and Tables
Figure 1 Key structures of the mAb probed by fluorescent dyes. N and U are native and unfolded monomers, respectively. “n” reactive monomers form aggregates.
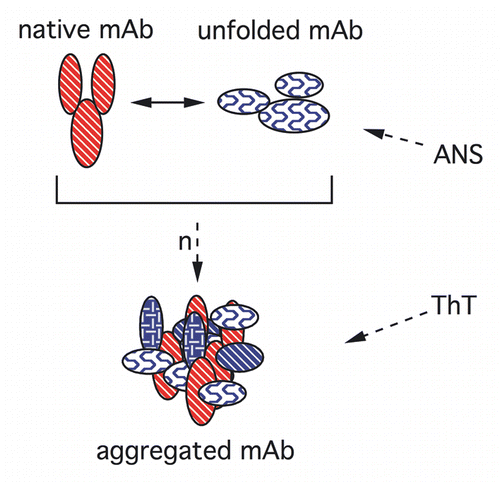
Figure 2 Binding of ANS to the mAb. (A) ANS binding equilibrium to native mAb with increasing dye concentration. Inset: Double reciprocal representation.Citation20 (B) Temperature-dependence of mAb unfolding studied with ANS binding. Dye binding rates were determined from these data as described in the text and shown in .
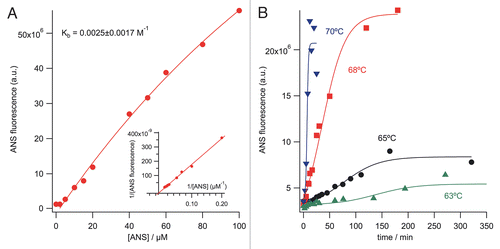
Figure 3 Arrhenius plots for unfolding and aggregate formation of the mAb as a function of temperature (63–70°C). Rate constants were calculated with the first-order reaction for both of the proteins' unfolding and aggregation with the empirical sigmoid fit function. SEC-HPLC results (55–68°C) were calculated using the second-order reaction rate. Data fit was performed using Arrhenius equation (ln kobs = ln A − Ea/RT). The activation energies for the mAb unfolding and aggregation from the ANS and ThT binding and SEC-HPLC experiments are 610, 544 and 549 kJ/mol, respectively.
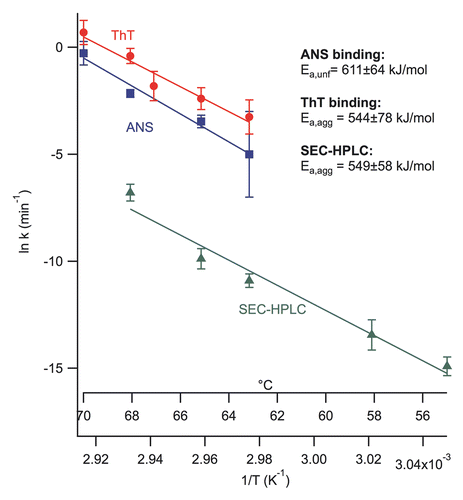
Table 1 Observed unfolding and aggregation rate constants of the mAb by the dye-binding studies
Additional material
Download Zip (598.8 KB)Acknowledgments
We thank Mehmet Sen (Immune Disease Institute) for valuable discussions and Novartis for financial support.
References
- Qu Z, Griffiths GL, Wegener WA, Chang CH, Govindan SV, Horak ID, et al. Development of humanized antibodies as cancer therapeutics. Methods 2005; 36:84 - 95; PMID: 15848077; http://dx.doi.org/10.1016/j.ymeth.2005.01.008
- Carter PJ. Potent antibody therapeutics by design. Nat Rev Immunol 2006; 6:343 - 357; http://dx.doi.org/10.1038/nri1837
- Hale G. Therapeutic antibodies—Delivering the promise?. Adv Drug Deliv Rev 2006; 58:633 - 639; PMID: 16822575; http://dx.doi.org/10.1016/j.addr.2006.03.010
- Mather JP. Engineered antibody therapeutics. Adv Drug Deliv Rev 2006; 58:631 - 632; PMID: 16857292; http://dx.doi.org/10.1016/j.addr.2006.05.001
- Wang W, Singh S, Zeng DL, King K, Nema S. Antibody structure, instability and formulation. J Pharm Sci 2007; 96:1 - 26; PMID: 16998873; http://dx.doi.org/10.1002/jps.20727
- Daugherty AL, Mrsny RJ. Formulation and delivery issues for monoclonal antibody therapeutics. Adv Drug Deliv Rev 2006; 58:686 - 706; PMID: 16839640; http://dx.doi.org/10.1016/j.addr.2006.03.011
- Kayser V, Chennamsetty N, Voynov V, Helk B, Forrer K, Trout BL. Evaluation of a non-Arrhenius model for therapeutic monoclonal antibody aggregation. J Pharm Sci 2011; 100:2526 - 2542; PMID: 21268027; http://dx.doi.org/10.1002/jps.22493
- Bee JS, Davis M, Freund E, Carpenter JF, Randolph TW. Aggregation of a monoclonal antibody induced by adsorption to stainless steel. Biotechnol Bioeng 2009; 105:121 - 129; PMID: 19725039; http://dx.doi.org/10.1002/bit.22525
- Carpenter JF, Randolph TW, Jiskoot W, Crommelin DJA, Middaugh CR, Winter G. Potential inaccurate quantitation and sizing of protein aggregates by size exclusion chromatography: Essential need to use orthogonal methods to assure the quality of therapeutic protein products. J Pharm Sci 2010; 99:2200 - 2208; PMID: 19918982; http://dx.doi.org/10.1002/jps.21989
- Stryer L. The interaction of a naphthalene dye with apomyoglobin and apohemoglobin: A fluorescent probe of non-polar binding sites. J Mol Biol 1965; 13:482 - 495; PMID: 5867031; http://dx.doi.org/10.1016/S0022-2836(6aev5)80111-5
- Semisotnov GV, Rodionova NA, Razguly OI, Uversky VN, Gripas' AF, Gilmanshin RI. Study of the “molten globule” intermediate state in protein folding by a hydrophobic fluorescent probe. Biopolymers 1991; 31:119 - 128; PMID: 2025683; http://dx.doi.org/10.1002/bip.360310111
- Levine HI. Thioflavine T interaction with synthetic Alzheimer's disease β-amyloid peptides: Detection of amyloid aggregation in solution. Protein Sci 1993; 2:404 - 410; PMID: 8453378; http://dx.doi.org/10.1002/pro.5560020312
- He F, Hogan S, Latypov RF, Narhi LO, Razinkov VI. High throughput thermostability screening of monoclonal antibody formulations. J Pharm Sci 2010; 99:1707 - 1720; PMID: 19780136; http://dx.doi.org/10.1002/jps.21955
- Demeule B, Lawrence MJ, Drake AF, Gurny R, Arvinte T. Characterization of protein aggregation: The case of a therapeutic immunoglobulin. Biochim Biophys Acta 2007; 1774:146 - 153; PMID: 17142116; http://dx.doi.org/10.1016/j.bbapap.2006.10.010
- Hawe A, Friess W, Sutter M, Jiskoot W. Online fluorescent dye detection method for the characterization of immunoglobulin G aggregation by size exclusion chromatography and asymmetrical flow field flow fractionation. Anal Biochem 2008; 378:115 - 122; PMID: 18455994; http://dx.doi.org/10.1016/j.ab.2008.03.050
- He F, Phan DH, Hogan S, Bailey R, Becker GW, Narhi LO, et al. Detection of IgG aggregation by a high throughput method based on extrinsic fluorescence. J Pharm Sci 2010; 99:2598 - 2608; PMID: 20039384; http://dx.doi.org/10.1002/jps.22036
- Demeule B, Palais C, Machaidze G, Gurny R, Arvinte T. New methods allowing the detection of protein aggregates: A case study on trastuzumab. mAbs 2009; 1:142 - 150; http://dx.doi.org/10.4161/mabs.1.2.7632
- Daniel E, Weber G. Cooperative effects in binding by bovine serum albumin. I. The binding of 1-Anilino-8-naphthalenesulfonate. Fluorimetric titrations. Biochemistry 1966; 5:1893 - 1900; http://dx.doi.org/10.1021/bi00870a016
- Guijarro JI, Sunde M, Jones JA, Campbell ID, Dobson CM. Amyloid fibril formation by an SH3 domain. Proc Natl Acad Sci 1998; 95:4224 - 4228; PMID: 9539718
- Van Nuland NAJ, Meijberg W, Warner J, Forge V, Scheek RM, Robillard GT, et al. Slow cooperative folding of a small globular protein HPr. Biochemistry 1998; 37:622 - 637; PMID: 9425085; http://dx.doi.org/10.1021/bi9717946
- Chang Ls, Wen Ey, Hung Jj, Chang Cc. Energy transfer from tryptophan residues of proteins to 8-anilinonaphthalene-1-sulfonate. Protein J 1994; 13:635 - 640; PMID: 7702745; http://dx.doi.org/10.1007/BF01890462
- Pedersen S, Nesgaard L, Baptista RP, Melo EP, Kristensen SR, Otzen DE. pH-dependent aggregation of cutinase is efficiently suppressed by 1,8-ANS. Biopolymers 2006; 83:619 - 629; PMID: 16964599; http://dx.doi.org/10.1002/bip.20598
- Biancalana M, Makabe K, Koide A, Koide S. Molecular mechanism of Thioflavin-T binding to the surface of β-rich peptide self-assemblies. J Mol Biol 2009; 385:1052 - 1063; PMID: 19038267; http://dx.doi.org/10.1016/j.jmb.2008.11.006
- Khurana R, Coleman C, Ionescu-Zanetti C, Carter SA, Krishna V, Grover RK, et al. Mechanism of thioflavin T binding to amyloid fibrils. J Struct Biol 2005; 151:229 - 238; PMID: 16125973; http://dx.doi.org/10.1016/j.jsb.2005.06.006
- LeVine H, Ronald W. Quantification of β-sheet amyloid fibril structures with thioflavin T. Methods Enzymol 1999; 309:274 - 284; PMID: 10507030; http://dx.doi.org/10.1016/S0076-6879(99)09020-5
- Roberts CJ. Kinetics of irreversible protein aggregation: Analysis of extended Lumry-Eyring models and implications for predicting protein shelf life. J Phys Chem B 2003; 107:1194 - 1207; http://dx.doi.org/10.1021/jp026827s
- Murphy RM, Tsai AM. Misbehaving proteins: protein (mis)folding, aggregation and stability 2006; New York Springer Science + Business Media, LLC