Abstract
Protein biopharmaceuticals, such as monoclonal antibodies (mAbs) are widely used for the prevention and treatment of various diseases. The complex and lengthy upstream and downstream production methods of the antibodies make them susceptible to physical and chemical modifications. Several IgG1 immunoglobulins are used as medical agents for the treatment of colon, breast, and head and neck cancers, and at least four to eight isoforms exist in the products. The regulatory agencies understand the complex nature of the antibody molecules and allow the manufactures to set their own specifications for lot release, provided the safety and efficacy of the products are established in animal models prior to clinical trials. During the manufacture of a mAb product, we observed lot-to-lot variability in the isoform content and, although the variability is within the set specifications for lot release, made attempts to gain mechanistic insight by isolating and characterizing the individual isoforms. Matrix-assisted laser desorption/ionization (MALDI) and liquid chromatography (LC)/mass spectrometry (MS)/MS analyses of the isolated isoforms indicate that this variability is caused by sialic acid content, as well as truncation of C-terminal lysine of the individual isoforms. Sialidase and carboxypeptidase treatment of the product confirm the observations made by MALDI and LC/MS/MS.
Monoclonal antibodies (mAbs) are used as medical agents to treat a variety of diseases including cancer, cardiovascular diseases and blood disorders.Citation1–Citation3 Although a few IgG2 (e.g., panitumumab, denosumab) and IgG4 antibody molecules are in the market, most of the approved products are IgG1 molecules. IgG1 antibodies are glycoproteins with a conserved N-glycosylation site at Asn 297. Glycosylation influences the biological functions, such as antibody dependent cell-mediated cytotoxicity (ADCC) and complement dependent cytotoxicity (CDC) of the antibodies. The oligosaccharides present in the IgG1 molecules are heterogeneous due to the presence of various sugar residues, including sialic acid, galactose, N-acetylglucasmine and fucose residues. Molecular alterations in antibodies can take place at every stage of manufacturing: upstream and downstream processing, formulation and storage. These alterations can take place enzymatically or non-enzymatically and may produce charge or size heterogeneity. Deamidation, proteolytic fragmentation, oxidation, disulfide bond shuffling and glycosylation are the most common modifications that occur during the production of protein therapeutics.Citation4–Citation7 These modifications can reduce the biological activity and may induce immunogenicity in patients. Hence, the regulatory agencies require a comprehensive characterization of the structural integrity, purity and stability of the protein therapeutics.Citation8
To date, eight chimeric, humanized and human IgG1 mAbs have been approved in the United States, Europe, as well as other countries, for the treatment of several types of cancers.Citation9–Citation12 One such molecule produced at ImClone has two N-glycosylation sites and at least six to eight isoforms with isoelectric points (pIs) between 7.9–8.9 are present in this product. Although techniques such as ion exchange chromatography (IEX) and capillary isoelectic focusing (IEF) are available for the separation and characterization of charge varients,Citation13,Citation14 we were not successful in separating the individual isoforms with these techniques from the IgG1 product used in this investigation. The peaks from IEX showed the presence of multiple bands on IEF. Hence, an alternative approach was used to isolate each isoform of this IgG1 product, and we demonstrated the involvement of sialic acid and C-terminal lysine as the root causes for lot-to-lot variation observed during the production of this molecule. The method is fast and very effective in separating isoforms with a difference in the pI values < 0.1.
Results
Variability of isoforms of IgG1 product due to process change.
The IEF pattern of different lots of the product produced using two different processes and different locations are shown in and . Irrespective of the process or the manufacturing location, six to eight isoforms are present in most of the lots with pI's between 7.9–8.9; however, the relative abundance of each isoform showed some variability. Hence, we made attempts to isolate and characterize each isoform of the product to determine the root cause of this charge heterogeneity. The N-linked glycan content of each isoform was compared to study the underlying cause of heterogeneity.
MALDI analysis of released glycan from IgG1 isoforms.
The matrix-assisted laser desorption/ionization (MALDI) analysis () of the IgG1 product indicates that most of the N-linked glycans were complex, biantennary glycans with core fucosylation. Small amounts of triantennary and high-man-nose glycans were also observed. A significant proportion of the N-glycans contain terminal α-gal and N-glycolyl neuraminic acid (NGNA) residues. shows N-linked oligosaccharide MALDI profile for each band. Basically, each band contains G0, G1 and G2 with core fucosylation. The oligosaccharides with terminal α-gal and NGNA residues play a significant role in the migration of the protein in IEF gel. Band 1 contained neutral oligosaccharide, mainly G2F-2α-gal; Band 7 and 8 contained mostly sialylated oligosaccharides with low amount of α-gal species. The relative abundances of oligosaccharides residues with terminal α-gal and NGNA vs. G0F, which was set as the internal reference for each band calculation (% relative abundance = oligosaccharide MS area/G0F MS area), are shown in . The species with different glycans are enriched differently in each band compared with the total pool of oligosaccharides. From the MALDI analysis of the released glycans from each isoform, it is evident that isoforms 2–7 contain sialyated oligosaccharides and isoform 7 contains fully sialylated oligosaccharides and less α-galactosylated oligosaccharides.
Sialidase treatment of isoform 7.
To better understand the affect of the variation observed in IEF, characterization studies were performed to determine the species present in IEF isoform 7. To determine if isoform 7 contained sialylated species, the mAb product was treated with sialidase and analyzed by IEF. As shown in , isoform 7 was not present in the sample of the product treated sialidase, demonstrating presence of terminal sialic acid.
C-terminal lysine.
The C-terminal lysine may also contribute to charge heterogeneity. To determine the presence of C-terminal lysine in isoform 7, the mAb product was treated with carboxy-peptidase and analyzed by IEF. The carboxypeptidase-treated sample showed the presence of isoform 7 (data not shown); however, isoforms 1 and 2 were no longer observed. This result indicates that isoforms 1 and 2 contain species with C-terminal lysine.
Peptide map of the isoforms of the IgG1 product.
The individual isoforms of the IgG1 product were reduced and alkylated, subjected to trypsin digestion and the peptides extracted from the membrane using 1% Zwittergent 3–16 at 37°C for 2 h. These samples were subjected to liquid chromatography (LC)/mass spectrometry (MS)/MS analysis; the peptide maps of each isoform are presented in . The C-terminal lysine containing peptide and truncated C-terminal form were identified by MS/MS data. From the analysis, the percent of lysine of isoforms 1–8 were determined to be 80.2, 54.3, 21.0, 14.3, 8.5, 5.4, 5.1 and 5.7, respectively, indicating lysine truncation in isoforms 5–8. This also confirmed the result from that both isoforms 1 and 2 have significant amounts of C-terminal lysine.
Biological activity of the IgG1 product.
The biological activities of all the lots used in this investigation are presented in . Although variation in the number of isoforms was observed, no significant difference in the biological activity was observed between these lots. This result indicates that the isoform variability did not affect the potency of the molecule.
Discussion
Due to the complexity of the upstream and downstream processing conditions used during mAb manufacturing, maintenance of the structural integrity of the molecule is a challenge. C-terminal processing of the lysine or arginine residues, variation in the glycosylation pattern, protein folding, disulfide bond formation and chemical modification of amino acid chains of the peptide backbone are known to cause structural heterogeneity.Citation6 Cell culture conditions have been known to cause post-translational modifications, such as deterioration of the N-glycosylation of Chinese hamster ovary cell-derived interferon gammaCitation15 and sialic acid composition of gamma interferon.Citation16 Patel et al.Citation17 reported that murine IgG exhibits varying sialic acid content depending on the production conditions. Fang et al.Citation18 documented that fermentation conditions have the capability to affect the ratio of the isoforms of HumanDREG-55. Apart from the cell culture conditions, formulations and storage conditions may also contribute to heterogeneity of the antibodies. Deamidation is shown to be one type of chemical degradation that occurs during production and storage of protein therapeutics.Citation6 Kroon et al.Citation19 demonstrated that two susceptible deamidation sites exist in Orthoclone OKT3. One of these sites was found to be in the constant region of the light chain, indicating that the kappa light chain is susceptible to deamidation at this site. Deamidation of the Asn at neutral or alkaline pH is facilitated if the subsequent residue is small, such as Ser or Gly.Citation20,Citation21 Perkins et al.Citation22 also made a similar observation. Additional factors such as three dimensional structure and exposure to solvent may also influence the rate of deamidation. It is known that the succinimide intermediate spontaneously hydrolyzes to isoAsp or Asp at an approximate ratio of 3:1.Citation23–Citation25 The isoAsp can distort the confirmation of the protein,Citation17 which may result in charge heterogeneity.Citation26 Charge variation can also occur due to the enzymatic removal of a C-terminal lysine from either one or both heavy chains. It is frequently observed in mAbs with up to three charge variants resulting from the removal of terminal lysine.Citation27 The extent of C-terminal lysine removal depends of the endogenous carboxypeptidase activity of the host cell line, and that peptidase level may vary from batch-to-batch fermentation conditions. Another common form of IgG heterogeneity involves cysteine residues, such as oxidation status, disulfide linkage or decomposition.Citation28,Citation29 Pristatsky et al.Citation30 provided evidence for the presence of trisulfide bonds in a human IgG2 mAb and speculated that dissolved hydrogen sulfide generated during the fermentation process might have contributed to the formation of trisulfide bonds.
The carbohydrate moiety of IgGs also exhibit heterogeneity in sugar content and structure. A negatively charged sialic acid molecule typically caps the end of the carbohydrate chain, and as a consequence of the variable nature of sialic acid content, isoforms with differences in charge can be formed.Citation31 Catlin et al.Citation32 reported that the number of sialic acid molecules influenced the pI of rHuEPO.
Antibody products produced in murine cell lines have several oligosaccharide structures.Citation33 The IEF of the product used in this investigation displayed banding patterns with a narrow pI range (<0.1 pH units) with different densities, which indicated protein heterogeneity. It is necessary for pharmaceutical manufacturers to define and characterize the pattern of protein heterogeneity and assure lot-to-lot consistency for the review by regulatory agencies.Citation8 Hence, we attempted to isolate and characterize the isoforms to identify the difference between the various subsets. Separation of the IgG1 isoforms poses a major challenge because the differences in pI are <0.1 pH units. We took a novel but simple approach to isolate the isoforms by performing the IEF on the flatbed surface, followed by blotting the isoforms on PVDF membrane. Using this approach, we generated enough material to perform peptide mapping and characterize the oligosaccharides. Our study revealed that the sialic acid content of the isoforms vary with each form, and the peptide map analysis of the isoforms indicated that the C-terminal lysine content also contributed to the differences in the migration pattern. Sialidase and carboxypeptidase treatments of the IgG1 product demonstrated the involvement of C-terminal lysine and sialic acid in charge heterogeneity of this molecule. Based on the results presented in this investigation, we propose that variation in the sialic acid content, as well as the differences in the C-terminal lysine content, contributed to variation in the IEF pattern in the product. The biological activity of all lots used in the investigation indicates no significant differences, which suggests that the variability in the isoform content of the IgG1 product may not result in drastic modification of the molecule.
There are numerous reports implicating the involvement of deamidation as one of the origins of charge heterogeneity; in most cases, loss of biological activity and shift in the pIs of the isoforms to the acidic region were reported. In this investigation, we did not observe loss of biological activity or a drastic shift in the pI's of the isoforms to the acidic region. However, it should be pointed out that after sialidase and carboxy peptidase treatments, three charged varients were still present in this molecule (). We isolated these varients and performed further characterization using LC/MS/MS. Our studies indicated that the isoforms remaining after sialidase and carboxy peptidase digestion had different degrees of deamidation on the heavy chain T34 (PENNY peptide) with a similar glycosylation pattern.
Apart from the inhibition of tumor cell proliferation, some IgG1 molecules are also capable of lysing cells through antibody-mediated celluar cytotoxicity (ADCC),Citation34 which is triggered upon binding of lymphocyte receptors to the Fc region of the antibody. It is well-documented that fucosylation of the IgG1 affects ADCC activity; however, the role of sialic acid in effector function is under debate.Citation35–Citation38 Antibody galactosylation has been observed to affect complement activation, but it has no impact on ADCC.Citation39 The affect of glycosylation on the ADCC activity of the mAb product discussed here was not within the scope of this report, but may be investigated in the future.
It is interesting to note that variability of the isoform pattern was observed irrespective of the process and location changes. During the production of antibodies, ranges for all processing conditions are generally used. For example, the fermentation conditions may not have an absolute limit for pH, stripping method, dissolved oxygen content, harvest time or the raw materials. It is likely that the minor changes (within the set acceptance range) in the upstream processing and other production conditions might have contributed to modification of glycosylation pattern and truncation of C-terminal lysine of the IgG1 product, thereby resulting in charge heterogeneity. Regulatory agencies understand the complex nature of antibodies and allow manufactures to set their own specifications for lot quality control.Citation40 However, the safety and efficacy of the released lots must be established in animal models prior to the initiation of clinical trials. Apart from this, it is also expected that the mechanism of action and the stability of the molecule will be understood. As required by the regulatory guidelines, the lots used in this investigation were tested in animal models and showed no significant differences in safety and efficacy.
Materials and Methods
The IgG1 mAb product used in this investigation was manufactured at ImClone Systems (Branchburg, NJ). Peptide N-glycosidase was purchased from Prozyme. Sodium cyanoborohydride, anthracitic acid (2-AA), 2,5-dihydroxybenzoic acid, 5-methoxysalicylic acid were obtained from Sigma-Aldrich.
Isolation of isoforms using isoelectric focusing.
IEF of the IgG1 product was performed using Multiphor™ Electrophoresis (GE Healthcare, Piscataway, NJ). Briefly, 10 µL (20 µg) of the desalted samples were applied on the IEF agarose pH 3–10 gels (Lonza, Wakersville, MD) and focused for 1 h 15 min at 1,000 V and 15 W. The anode and cathode solutions were 0.5 M acetic acid and 0.5 M NaOH, respectively. The separated isoforms were then transferred to a polyvinylidene fluoride (PVDF) membrane by press blotting for 15 min, stained with Coomassie blue and the bands were excised using a razor blade. Each band was cut from the PVDF membrane as closely as possible to minimize overlap between the bands, divided into a number of smaller pieces washed and destained in 500 µL 50% methanol overnight. The membrane pieces were then dehydrated for further analysis.
N-glycan release and anthranilic acid labeling.
N-linked oligosaccharides for each band were released by treatment with PNGase F for approximately 18 h at 37°C. The released N-glycans were labeled with 2-AA and sodium cyanoborohydride in methanol/acetic acid for 1.5 h at 80°C. The labeled glycans were purified by SPE cartridge.
MALDI analysis.
Small aliquots of the reductively-aminated N-glycans were dialyzed for 45 min using an MF-Millipore membrane filter (0.025 µm pore, 47 mm dia), floating on water. The dialyzed sample was dried in a Speed-Vac, redissolved in a small amount of water, and mixed with a solution of 2,5-dihydroxybenzoic acid (9 g/L) and 5-methoxysalicylic acid (1 g/L) dissolved in water/acetonitrile (50:50). The mixture was dried onto a MALDI target and analyzed using an Applied Biosystems DE-Pro mass spectrometer operated in the linear/negative-ion mode. Oligosaccharides were assigned based on the observed mass-to-charge ratio.
Biological activity of the IgG1 product.
The biological activity of the IgG1 product was monitored by plating target cells expressing specific receptor for the IgG1 in 96-well plate followed by the addition of IgG1 at concentrations ranging from 7.5 nM to 0.01 nM. The cells treated with the antibody were incubated for 72 h at 37°C/7% CO2. The cell growth was measured by the uptake and cleavage of yellow 3-(4,5-dimethyl-2-yl)-5-(3-carboxymethoxyphenyl)-2-(4-sulfophenyl)-2H-tetrazolium (MTS) to purple formazan crystals. The biological activity was reported as the ratio of percent growth inhibition using ED50 values of the test sample to the reference standard.
Trypsin PVDF membrane peptide mapping.
The bands were cut from PVDF membrane and wet by 100% MeOH before de-stained by water/acetonitrile (50:50). The bands were reduced with 0.02 M DTT in 0.05 M Tris pH 7.5 at 37°C for an hour followed by alkylation with 0.1 M iodoacetimide at room temperature in dark for 30 min. The digestion was performed using trypsin (5 ng/ml) in 0.05 M Tris buffer pH 7.5 with 1% Zwittergent 3–16 (Calbiochem, San Diego, CA) at 37°C for 2 h. The digested peptides were extracted using water/acetonitrile (60:40) with 0.1% trifluoroacetic acid, and the resulting solution was dried down before analysis by LC-MS/MS. The resultant peptides were resolved using a C18 reverse phase column (Zorbax C18 300SB, 300 Å, 5 µm, 4.6 × 150 mm) using an Agilent HPLC 1100 series (Agilent, Wilmington, DE) interfaced to a LCQ Deca ion trap mass spectrometer (Thermo Scientific). The digested sample was eluted with a gradient from 98% solvent A (0.1% TFA) to 40% solvent B (100% acetonitrile, 0.085% TFA) in 95 min at a flow rate of 0.5 ml/min. The scale of X axis of all the chromatograms is tailored to maximize the intensity of the C-terminal peptides.
Disclosure of Potential Conflicts of Interest
No potential conflicts of interest were disclosed.
Figures and Tables
Figure 1 Comparison of the isoforms of different lots of IgG1 product produced using Process 1 and 2. (A and J) Markers; (B) Process 1, Lot 1; (C) Process 1, Lot 2; (D) Process 2, Lot 1; (E) Process 2, Lot 2; (F) Lot 1, Location 1; (G) Lot 2, Location 2; (H) Lot 3, Location 1; (I) Lot 4, Location 2.
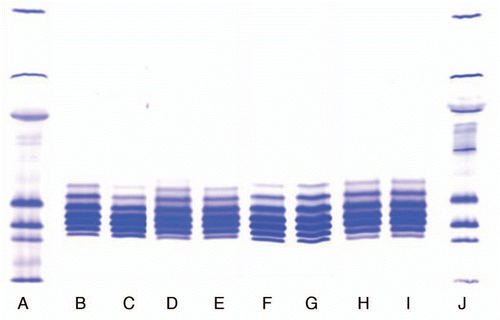
Figure 2 Comparison of isoforms of the IgG1 product prepared with Process 3 in two locations. (F) Marker; (G) Lot 1, Location 1; (H) Lot 2, Location 2; (I) Lot 3, Location 1; (J) Lot 4, Location 4.
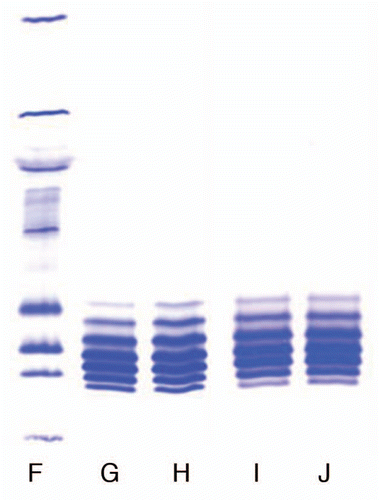
Figure 3 Negative-ion MALDI spectrum of 2-AA N-linked glycans released from IgG1 product RS by PNGase F. Sugar residues are GlcNAc (blue square), Fuc (yellow triangle), Man (green circle), Gal (pink circle) and NGNA (black diamond).
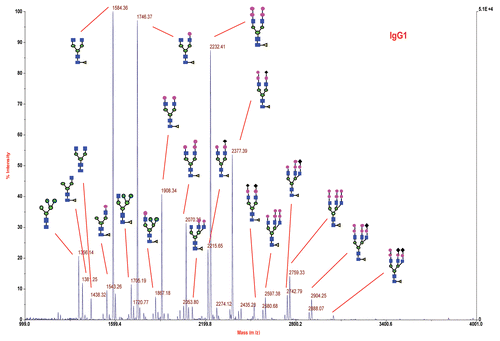
Figure 5 Effect of sialidase and carboxypeptidase treatment on the IgG1 product. The Isoforms 1 and 2 are not present in the sialidase and carboxy peptidase treated sample but they are present in sialidase treated sample. Isoforms 6, 7, 8 are not present in sialidase treated sample indicating that they contain sialic acid.
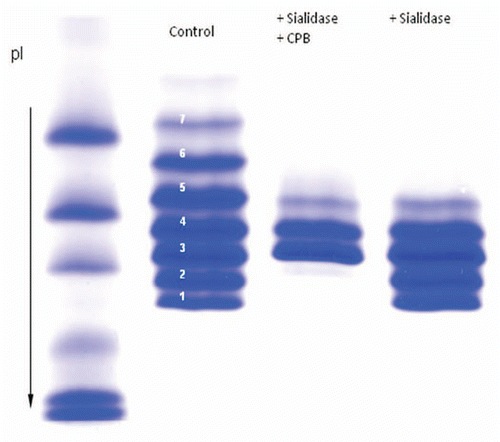
Figure 6 LC/MS/MS peptide map of IgG1 isoforms. The percent of Lysine of the isoforms 1–8 were determined to be 80.2, 54.3, 21.0, 14.3, 8.5, 5.4, 5.1 and 5.7, respectively indicating lysine truncation in isoforms 5–8.
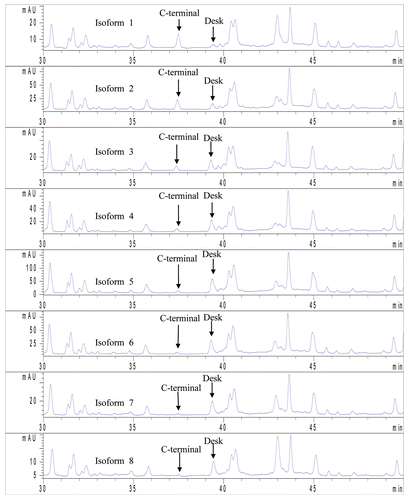
Table 1 Relative abundance of oligosaccharides verse G0F for each band
Table 2 Biological activity of the IgG1 product
Acknowledgments
The authors would like to thank Ms. Meinuo Li and Dr. Liangjie Tang for preparing sialidase-carboxypeptidase treated monoclonal antibody product.
References
- Brekke OH, Sandlie I. Therapeutic antibodies for human diseases at the dawn of the twenty-first century. Nat Rev Drug Discov 2003; 2:52 - 62; PMID: 12509759; http://dx.doi.org/10.1038/nrd984
- Bebbington C, Yarranton G. Antibodies for the treatment of bacterial infections: current experience and future prospects. Curr Opin Biotechnol 2008; 19:613 - 619; PMID: 19000762; http://dx.doi.org/10.1016/j.cop-bio.2008.10.002
- Aires da Silva F, Corte-Real S, Goncalves J. Recombinant antibodies as therapeutic agents: pathways for modeling new biodrugs. BioDrugs 2008; 22:301 - 314; PMID: 18778112
- Jefferis R, Lund J, Mizutani H, Nakagawa H, Kawazoe Y, Arata Y, et al. A comparative study of N-linked oligosaccharide structures of human IgG subclass proteins. Biochem J 1990; 268:529 - 537; PMID: 2363690
- Jefferis R, Lund J. Glycosylation of antibody molecules. Structural and functional significance. Chem Immunol 1997; 65:111 - 128; PMID: 9018875; http://dx.doi.org/10.1159/000319352
- Manning MC, Patel K, Borchardt R. Stability of protein pharmaceuticals. Pharm Res 1989; 6:903 - 918; PMID: 2687836; http://dx.doi.org/10.1023/A:1015929109894
- Volkin DB, Mach H, Middaugh CR. Degradative covalent reactions important to protein stability. Mol Biotechnol 1997; 8:105 - 122; PMID: 9406181
- United States Food and Drug Administration. Points to consider in the manufacture and testing of monoclonal antibody products for human use 1997; 2 28 US department of Health and Human services, FDA Center for Biologics Evaluation and Research
- Reichert JM. Antibody-based therapeutics to watch in 2011. MAbs 2011; 3:76 - 99; PMID: 21051951; http://dx.doi.org/10.4161/mabs.3.1.13895
- Reichert JM. Metrics for antibody development. MAbs 2010; 2:695 - 700; PMID: 20930555; http://dx.doi.org/10.4161/mabs.2.6.13603
- Lansigan F, Foss FM. Current and emerging treatment strategies for cutaneous T-cell lymphoma. Drugs 2010; 70:273 - 286; PMID: 20166766; http://dx.doi.org/10.2165/11532190
- Eggermont AM, Robert C. New drugs in melanoma: It's whole new world. Eur J Cancer 2011; 47:2150 - 2157; PMID: 21802280; http://dx.doi.org/10.1016/j.ejca.2011.06.052
- Vlasak J, Ionescu R. Heterogeneity of monoclonal antibodies revealed by charge sensitive methods. Curr Pharm Biotechnol 2008; 9:468 - 481; PMID: 19075686; http://dx.doi.org/10.2174/138920108786786402
- Meert CD, Brady LJ, Guo A, Balland A. Characterization of antibody charge heterogeneity resolved by preparative immobilized pH gradients. Anal Chem 2010; 82:3510 - 3518; PMID: 20364842; http://dx.doi.org/10.1021/ac902408r
- Hooker AD, Goldman MH, Markham MH, James DC, Ison AP, Bull AT, et al. N-glycans of recombinant human interferon-gamma change during batch culture of Chinese hamster ovary cells. Biotechnol Bioeng 1995; 48:639 - 648; PMID: 18623533; http://dx.doi.org/10.1002/bit.260480612
- Gu X, Xie L, Harmon BJ, Wang DI. Influence of Primatone RL supplementation on sialylation of recombinant human interferon-gamma produced by Chinese hamster ovary cell culture using serum-free media. Biotechnol Bioeng 1997; 56:353 - 360; PMID: 18642238; http://dx.doi.org/10.1002/(SICI)1097-0290(19971120)56:4353::AID-BIT13.0.CO;2-N
- Patel TP, Parkeh RB, Moellering BJ, Prior CP. Different culture methods lead to differences in glycosylation of a murine IgG monoclonal antibody. Biochem J 1992; 285:839 - 845; PMID: 1497622
- Fang Y, Dumont L, Larsen B. Real time isoform analysis by two dimensional chromatography of a monoclonal antibody during bioreactor fermentations. J Chromatogr A 1998; 816:39 - 47; PMID: 9741099; http://dx.doi.org/10.1016/S0021-9673(98)00032-6
- Kroon DJ, Baldwin-Ferro A, Lalan L. Identification of sites of degradation in a therapeutic monoclonal antibody by peptide mapping. Pharm Res 1992; 9:1386 - 1393; PMID: 1475223; http://dx.doi.org/10.1023/A:1015894409623
- Bornstein P, Balian G. Hirs CHW, Serge N, Timasheff N. Cleavage at Asn-Gly bonds with hydroxylamine. Methods in Enzymol. Vol XLVIII, Enzyme Structure (Part E) 1997; New York Academic Press
- Stephenson RC, Clarke S. Succimide formation from aspartyl and asparaginal peptides as a model for spontaneous degradation of proteins. J Biol Chem 1989; 264:6164 - 6170; PMID: 2703484
- Perkins M, Theiler R, Lunte S, Jeschke M. Determination of the origin of charge heterogeneity in a murine monoclonal antibody. Pharm Res 2000; 17:1110 - 1117; PMID: 11087044; http://dx.doi.org/10.1023/A:1026461830617
- Clarke S. Propensity for spontaneous succinimide formation from aspartyl and asparaginyl residues in cellular proteins. Int J Pept Protein Res 1987; 30:808 - 821; PMID: 3440704; http://dx.doi.org/10.1111/j.1399-3011.1987.tb03390.x
- Kossiakoff AA. Tertiary structure is a principal determinant to protein deamidation. Science 1988; 240:191 - 194; PMID: 3353715; http://dx.doi.org/10.1126/science.3353715
- Tyler-Cross R, Schirch V. Effects of amino acid sequence, buffers and ionic strength on the rate and mechanism of deamidation of asparagines residues in small peptides. J Biol Chem 1991; 266:22549 - 22556; PMID: 1939272
- Zhang W, Czuprryn M. Analysis of isoaspartate in a recombinant monoclonal antibody and its charge isoforms. J Pharm Biomed Anal 2003; 30:1479 - 1490; PMID: 12467919; http://dx.doi.org/10.1016/S0731-7085(02)00479-X
- Harris RJ. Processing of C-terminal lysine and arginine residues of proteins isolated from mammalian cell culture. J Chromatogr A 1995; 705:129 - 134; PMID: 7620566; http://dx.doi.org/10.1016/0021-9673(94)01255-D
- Tous GI, Wei Z, Feng J, Bilbulin S, Bowen S, Smith J, et al. Characterization of a novel modification to monoclonal antibodies: thioether cross-link of heavy and light chains. Anal Chem 2005; 77:2675 - 2682; PMID: 15859580; http://dx.doi.org/10.1021/ac0500582
- Cohen SL, Price C, Vlasak J. β-elimination and peptide bond hydrolysis: two distinct mechanisms of human IgG1 hinge fragmentation upon storage. J Am Chem Soc 2007; 129:6976 - 6977; PMID: 17500521; http://dx.doi.org/10.1021/ja0705994
- Pristatsky P, Cohen SL, Krantz D, Acevedo J, Ionescu R, Vlasak J. Evidence for trisulfide bonds in a recombinant vaient of human IgG2 monoclonal antibody. Anal Chem 2009; 81:6148 - 6155; PMID: 19591437; http://dx.doi.org/10.1021/ac9006254
- Rush RS, Derby PL, Smith DM, Merry C, Rogers G, Rhode MF. Micro-hertogeneity of erythropoietin carbohydrate structure. Anal Chem 1995; 67:1442 - 1452; PMID: 7741215; http://dx.doi.org/10.1021/ac00104a022
- Catlin DH, Breidbach A, Elliot S, Glaspy J. Comparison of isoelectric patterns of Darbepoeetin Alfa, recombinant erythropoietin and endogeneous erythropoietin from human urine. Clin Chem 2002; 11:2057 - 2059
- Qian J, Liu T, Yang L, Daus A, Crowley R, Zhou Q. Structural characterization of N-linked oligosaccharides on monoclonal antibody cetuximab by the combination of orthogonal matrix-assisted lase desorption/ionization hybrid quadrupole-quadrupole time-of-fight tandem mass spectrometry and sequential enzymatic digestion. Anal Biochem 2007; 364:8 - 18; PMID: 17362871; http://dx.doi.org/10.1016/j.ab.2007.01.023
- Natsume A, Niwa R, Satoh M. Improving effector functions of antibodies for cancer treatment: Enhancing ADCC and CDC. Drug Des Devel Ther 2009; 3:7 - 16; PMID: 19920917
- Peipp M, Lammerts JJ, van Bueren L, Schneider-Merck TJ, Bleeker WWK, Dechant M, et al. Antibody fucosylation differently impacts cytotoxicity mediated by NK and PMN effector cells. Blood 2008; 112:2390 - 2399; PMID: 18566325; http://dx.doi.org/10.1182/blood-2008-03-144600
- Kaneko Y, Nimmerijahn F, Ravetch JV. Anti inflammatory activity of immunoglobulin G resulting from Fc sialyation. Science 2006; 313:670 - 673; PMID: 16888140; http://dx.doi.org/10.1126/science.1129594
- Scallon BJ, Tam SH, McCarthy SG, Cai AN, Raju TS. Higher levels of sialyated Fc glycans in immunoglobulin G molecules can adversely impact functionality. Mol Immunol 2007; 44:1524 - 1534; PMID: 17045339; http://dx.doi.org/10.1016/j.molimm.2006.09.005
- Naso MF, Tam SH, Scallon BJ, Raju TS. Engineering host cell lines to reduce terminal sialyation of secreted antibodies. MAbs 2010; 2:519 - 527; PMID: 20716959; http://dx.doi.org/10.4161/mabs.2.5.13078
- Shinkawa T, Nakamura K, Yamane N, Shoji-Hosaka E, Kanda Y, Sakurada M, et al. The absence of fucose but not the presence of galactose or bisecting N-acetylglucosamine of human IgG1 complex-type oligosaccharides shows the critical role of enhancing antibody dependent cellular cytotoxicity. J Biol Chem 2003; 278:3466 - 3473; PMID: 12427744; http://dx.doi.org/10.1074/jbc.M210665200
- Weinberg W, Fraizer-Jesson MR, Wu WJ, Weir A, Hartsough M, Keegan P, et al. Development and regulation of monoclonal antibody products: Challenges and opportunities. Cancer Metastasis Rev 2005; 24:569 - 584; PMID: 16408162; http://dx.doi.org/10.1007/s10555-005-6196-y