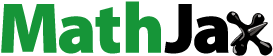
Abstract
Subcutaneous (SC) delivery is a common route of administration for therapeutic monoclonal antibodies (mAbs) with pharmacokinetic (PK)/pharmacodynamic (PD) properties requiring long-term or frequent drug administration. An ideal in vivo preclinical model for predicting human PK following SC administration may be one in which the skin and overall physiological characteristics are similar to that of humans. In this study, the PK properties of a series of therapeutic mAbs following intravenous (IV) and SC administration in Göttingen minipigs were compared with data obtained previously from humans. The present studies demonstrated: (1) minipig is predictive of human linear clearance; (2) the SC bioavailabilities in minipigs are weakly correlated with those in human; (3) minipig mAb SC absorption rates are generally higher than those in human and (4) the SC bioavailability appears to correlate with systemic clearance in minipigs. Given the important role of the neonatal Fc-receptor (FcRn) in the PK of mAbs, the in vitro binding affinities of these IgGs against porcine, human and cynomolgus monkey FcRn were tested. The result showed comparable FcRn binding affinities across species. Further, mAbs with higher isoelectric point tended to have faster systemic clearance and lower SC bioavailability in both minipig and human. Taken together, these data lend increased support for the use of the minipig as an alternative predictive model for human IV and SC PK of mAbs.
Introduction
The primary advantages of monoclonal antibodies (mAbs) as therapeutic molecules are their target specificity and their prolonged serum persistence. During the development of mAb therapeutics, the selection of a relevant preclinical animal model is essential for the prediction of the human pharmacokinetic (PK) profile, as well as for assessing overall safety and exposure-response relationships prior to clinical studies.Citation1–Citation3 Moreover, a predictive preclinical PK model is also important for understanding the impact of various molecular properties on the in vivo behavior of mAbs, thus enabling optimization of therapeutic candidates in early development.
Therapeutic mAbs are typically administered via intravenous (IV) infusion or subcutaneous (SC) injection. While the PK properties of mAbs following IV administration are well predicted by studies in non-human primates,Citation4–Citation6 a reliable animal model to predict the PK of mAbs following SC administration has not been clearly established.Citation7 Relatively little is known about the mechanism of SC absorption of mAbs in different species. The major parameters that affect this process are thought to include the role of lymph and blood capillaries in systemic absorption, cross-species differences in hypodermis morphology and physiology, drug formulation, stability of the molecule, the site of injection, the depth of injection, as well as the molecular properties of the mAbs themselves.Citation8–Citation11 Published data suggest that animal models are not necessarily reliable predictors of antibody SC PK in humans as there is often no apparent relationship in SC bioavailability between humans and animals.Citation7 Indeed, for larger biotherapeutics, absolute SC bioavailability has been reported to be higher in cynomolgus monkeys than in humans, while for biotherapeutics with molecular weights <40 kDa no clear relationship was obvious between human and animal data, both in rodents and cynomolgus monkeys.Citation7 Given these discrepancies, it is believed that the lack of predictability could be attributed to differences in hypodermis structure and physiology between humans and rodents or non-human primates.Citation12
With the increasing ethical concerns regarding the use of primates in non-clinical testing, attention has been increasingly focused on the potential use of minipigs as non-rodent alternatives for pharmaceutical testing.Citation13–Citation17 As such, minipigs are becoming more frequently used for toxicological and PK studies of small molecules.Citation18–Citation20 Recently, minipigs have been used to test the immunogenicity of therapeutic proteins;Citation21,Citation22 however, limited data exist for the pharmacokinetics of macromolecules in minipigs.Citation23–Citation25 In particular, to the best of our knowledge, there are no studies that describe the PK of mAbs in minipigs. The combined results from previous studies have demonstrated similarities between pig/minipig and human skin and lymph architectureCitation26,Citation27 that are likely key contributors to SC absorption and bioavailability of macromolecules. In addition, the thickness of the epidermis and the stratum corneum as well as the lipid composition of the stratum corneum is similar between human and pigs, which has led to the frequent use of the pig/minipig model for dermal administration.Citation28 The pig also has a tight link between dermis and underlying muscle, which is similar to the human situation, and these features have been attributed to the arrangement of elastic fibers in the hypodermis/subcutis, the target for SC administration.Citation29 The structure of the hypodermis in pigs differs from that in furred animals like rodents and cynomolgus monkeys, which have less abundant elastic fiber, and thus looser skin.Citation30 Similar to humans, the dermis of pigs is connected to the deep fascia via a fibrous network in the hypodermis.Citation29 Finally, pigs/minipigs have an easily accessible SC space with a tissue thickness similar to humans, making this species suitable to mimic the human situation for SC administration of proteins in preclinical studies. Because of these similarities, it is hypothesized that the PK of macromolecules in minipigs following SC administration may better resemble the SC PK in humans than other commonly used laboratory animals.
A robust preclinical model for predicting human PK of mAbs is also desirable to study the effects of various molecular properties of mAbs on their in vivo behaviors. Advances in antibody engineering technologies have enabled the development of a diverse class of humanized and human antibodies consisting of IgGs with such altered properties as different molecular weights, domain architectures, glycosylation patterns, electrical charge, subclasses and interactions with Fc receptors or target molecules.Citation31 As a result, the impact of these properties on the PK and distribution properties of IgGs is of keen interest. In particular, it has been well established that the binding of the IgG Fc domain to the neonatal Fc receptor (FcRn) plays a principal role in the long serum persistence of IgG by salvaging it from a default catabolic pathway in the vascular endothelium and bone-marrow derived cells.Citation32–Citation37 Hence, altering the binding affinity of IgG to FcRn can have a significant impact on serum IgG half-life, particularly in the case where affinity is reduced.Citation38 In addition, previous studies also indicate that nonspecific electrostatic interactions caused by differences in cell membrane surface charge and antibody charge can affect the tissue distribution and pharmacokinetics of mAbs.Citation39 For instance, modification of the isoelectric point of an antibody of approximately one pI unit or more can result in significant differences in its PK properties.Citation39–Citation41 Therefore, for successful non-clinical PK evaluation of mAbs, understanding the various characteristics of the mAb, such as pI, specific and non-specific binding, and FcRn affinity, can be helpful.
In this study, the PK of a series of therapeutic mAbs following IV and SC administration was assessed in Göttingen minipigs. The tested mAbs are active against soluble or membrane-bound targets with indications in oncology, inflammatory diseases or metabolic diseases. The derived PK parameters were compared with the known PK properties of these mAbs in humans. In addition, given that binding to FcRn plays an important role in the disposition and serum half-life of mAbs,Citation32,Citation37 the binding affinity of these antibodies to human, pig and cynomolgus monkey FcRn was compared. Further, the impact of various characteristics of these mAbs, including FcRn binding, isoelectric point (pI) and in vitro blood cell and plasma protein binding, on their PK properties were evaluated. The goal of this study is to pave the way for further evaluation of minipig as a potential translatable model for human PK of mAbs.
Results
Pharmacokinetic study in minipigs after intravenous and subcutaneous administration.
The pharmacokinetic properties of eight therapeutic human IgG antibodies were evaluated in Göttingen minipigs after IV and SC administration. The PK of one additional mAb (mAb8) was tested after IV injection only. Profiles of the mean serum/plasma mAb concentration vs. time after a single IV or SC administration for each mAb are presented in . The estimated PK parameters from a compartmental analysis of both IV and SC data are summarized in .
Following IV injection, the serum/plasma concentration of most molecules tested exhibited a biphasic PK profile typical for mAbs, with a relatively rapid distribution phase, slow elimination phase and log-linear terminal phase with no signs of nonlinear pharmacokinetics. An exception is adalimumab, whose PK appeared to be affected by anti-therapeutic antibodies (ATA). In addition, mAb7 showed a slight trend of nonlinearity in the terminal phase after IV injection. The elimination half-lives of the various mAbs were relatively long, ranging from 6.9 to 26 d. The central volume of distribution (Vc) ranged from 36 to 62 mL/kg, consistent with the expected range of plasma volumes in animals.Citation42 The systemic clearance (CL) was slow, ranging from 2.5 to 11 mL/day/kg for most molecules, except for mAb2 which had an unusually fast CL of 36 mL/day/kg.
Following SC injection, the antibodies were slowly absorbed into the systemic circulation with a median time to maximum concentration (Tmax) between 1 and 4 d and with estimated rates of absorption ranging from 0.32 to 4.6 d−1. With the exception of adalimumab, whose PK appears to be affected by anti-therapeutic antibodies (ATA), the elimination profiles for SC and IV administration were nearly parallel for all mAbs tested, suggesting similar systemic elimination kinetics following SC or IV injections of these mAbs. The estimated systemic bioavailability (assessed as drug fraction absorbed by compartmental modeling) following SC injection was variable among different molecules, ranging from 36 to 98%.
Comparison of minipig and human pharmacokinetic properties.
Allometric scaling of clearance. In order to examine whether minipigs can be used to predict the clearance of mAbs in human, the correlation between the clearance rates of mAbs observed in this study and those previously obtained in human studies was examined using interspecies scaling. The results are tabulated in . The calculated allometric scaling exponent (w) ranged from 0.75 to 1.17, with an arithmetic mean value of 0.98 and a standard deviation of 0.16, using data from the eight mAbs in the present study. MAb2 was excluded from the calculations due to an abnormally fast clearance in minipigs, albeit normal clearance in humans. Further, analysis of linear correlation between the CL observed in minipigs and humans showed a positive correlation between the two (r2 = 0.69) ().
Comparison of SC bioavailability and absorption rate. The available human SC bioavailability/fraction absorbed (F) and rate of absorption (Ka) data for these mAbs are summarized in . The correlation between human and minipig bioavailability is shown in . There is a weak correlation between minipig and human SC bioavailability (r2 = 0.32) (). In general, molecules with higher bioavailability in minipig tend to have higher bioavailability in human, although a few molecules showed higher bioavailability in minipigs than in humans. Out of the six mAbs with both human and minipig SC bioavailability data, one mAb had similar bioavailability in human and minipig (mAb4), one had lower bioavailability in minipig (mAb5) and the remaining four had higher bioavailability in minipig. Notably, three mAbs with the highest bioavailability in minipig (mAb1, mAb5 and adalimumab) also had the highest bioavailability in human. The SC rate of absorption is higher in minipigs than that in humans for all molecules ( and ).
Correlation between systemic clearance and bioavailability. Interestingly, there is an apparent correlation (r2 = 0.82) between the systemic clearance and bioavailability (after excluding mAb2 which had an unusual PK in minipig) such that as clearance increases, the bioavailability tends to decrease (). However, from the limited data available, no obvious correlation was observed in human ().
Effect of FcRn binding, pIs and blood/plasma protein binding on pharmacokinetics.
To compare the FcRn binding properties across species and to determine if differences in FcRn binding affinity could account for observed differences in PK parameters, we evaluated the binding affinity of each IgG to recombinantly-expressed human, pig and cynomolgus monkey FcRn via surface plasmon resonance (BIAcore). Purified FcRn was injected over immobilized IgGs, and the equilibrium dissociation constant (KD) for each interaction was calculated using a simple 1:1 binding model (). Values for KD are reported in nM and standard errors appear in parentheses for each value. No significant cross-species variability in the IgG-FcRn binding affinity was observed for any of the IgGs tested. In addition, binding across different IgGs was fairly consistent for a given species of FcRn. No apparent correlation was found between FcRn binding affinity and the clearance or SC bioavailability of the various mAbs in minipig or human (data not shown).
To assess the potential impact of mAb pI on PK properties, the pI values for all mAbs were obtained either experimentally by IEF or from the literature and are shown in . Interestingly, a clear trend was observed: mAbs with higher pI values (>∼9.0) appeared to have faster clearance (after excluding mAb2 with unusually fast clearance in minipig) and lower bioavailability in both human and minipig, with the exception of mAb5 ().
To examine the effect of blood and/or plasma protein binding on PK behavior of the mAbs, in vitro binding studies were conducted in minipig whole blood. The results demonstrate that none of the mAbs bound to blood cells () or plasma proteins from minipig, as determined by size-exclusion HPLC ().
Discussion
Characterization of serum clearance, SC bioavailability and systemic absorption of mAbs in relevant animal models is crucial for designing improved formulations or drug delivery systems, as well as for the interpretation of exposure-response relationships and development of useful PK models with predictive value.Citation8 Preclinical experimentation remains an essential component of antibody PK testing, but there is no requirement to solely rely on traditional animal species.Citation43 As established models, rodents and monkeys are generally the default animal species of choice to characterize mAb PK. However, there are known differences in the skin architecture and physiology between humans and rodents or non-human primates,Citation12,Citation30 and the predictability of these species for human SC PK of macromolecules has been poor.Citation7 The minipig is a potentially better predicative animal model given its similarity in skin physiology to human.Citation26–Citation29 To date, there has been no published data on the PK of mAbs in minipig, and no systematic study to compare the applicability of minipigs and non-human primates as translatable preclinical models for this class of biotherapeutics.Citation44 Our present study was conducted to fill this gap.
Cross-reactivity of the respective mAb to its target has to be considered when conducting PK studies in animals, since target-mediated disposition can play a relevant role in the PK of mAbs in vivo. The cross-reactivity of the tested mAbs in minipigs was not studied, but it was not expected in this non-primate species. Also the appearance of the concentration-time curves gave no indication for a nonlinear pharmacokinetics, as they showed a log-linear elimination phase after IV administration. Thus, any potentially existing target-mediated CL pathway was saturated at the doses used in our study. An exception to this was mAb7, for which a nonlinear pharmacokinetics was evident and considered in the compartmental PK model. Thus, except for mAb7, minipig data reflect linear CL. In order to ensure an appropriate comparison between minipig and human CL data, human CL data at doses that saturated the target-mediated CL were used in the analysis for mAbs with relevant target-mediated CL in humans. The results from this study showed that, in general, the CL of mAbs in minipig is predictive of that in human, with an estimated allometric scaling exponent of 0.98 ( and ) in the absence of relevant contributions from target mediated drug-disposition to the overall clearance. The inter-species scaling of the CL of mAbs have been evaluated in previous studies in reference Citation4, Citation5 and Citation45. These studies suggested that cynomolgus monkeys can be successfully used to predict human CL of therapeutic antibodies using an allometric scaling exponent of 0.85. The data suggest that the minipig may be used as an alternative animal model to project human CL for mAbs. It is of note, however, that these projections address only the linear clearance processes, but not target-mediated, nonlinear clearance processes, which depends on the cross-reactivity of the mAb to its target in the minipig species. Allometric scaling of nonlinear CL, however, is hampered by the potential interspecies differences in target expression, turnover and affinity and has not yet been successful.Citation4
Conversely, a weak correlation observed between the SC bioavailability of mAbs in minipig and human was observed (), although it should be noted that the number of molecules available for this analysis was quite limited. Out of the six mAbs tested, one had similar bioavailability in minipig and human, one had higher bioavailability in human and four had higher bioavailability in minipig. Notably, three mAbs with the highest bioavailability in minipig (mAb1, mAb5 and adalimumab) also had the highest bioavailability in human. Also, in the correlation of SC data from minipigs and humans, the potential role of target-mediated disposition needs to be considered. However, the chosen compartmental PK assessment and PK parameters for description of SC absorption (fraction absorbed and absorption rate constant) are independent of systemic target-mediated disposition processes. Thus, these parameters are still useful when assessed in a non-responder species, unless there is relevant target expression in the hypodermis or draining lymph vessels. The latter may lead to a relevant target-mediated ‘first-pass clearance’ during the absorption phase, which would reduce the fraction absorbed.
Recently published work by McDonald et al. described the SC bioavailability of therapeutic proteins between different species and showed that four out of the five marketed monoclonal antibodies reviewed had higher bioavailability in cynomolgus monkeys than in humans, with adalimumab having markedly higher bioavailability (96%) in cynomolgus monkeys.Citation7 Only one of the five had similar bioavailability in both species.Citation7 Of the mAbs discussed in this report, our in-house data suggest that two mAbs (mAb2 and mAb3) had higher SC bioavailability in cynomolgus monkeys (85% and 74%, respectively, unpublished data) than in humans. For these three antibodies (adalimumab, mAb2 and mAb3), the SC bioavailabilities measured in minipigs are closer to those obtained in humans than those obtained in cynomolgus monkeys. Hence, the results presented here provide initial evidence to suggest that minipig may be a better predictive model for human SC bioavailability of mAbs than cynomolgus monkeys although additional data are needed to confirm this. Interestingly, a clear relationship was found between clearance and SC bioavailability in minipigs when mAb2 is excluded due to its unusually fast clearance (). This relationship suggests that the same molecular characteristics or pharmacokinetic processes may determine systemic clearance and SC absorption, the latter being reflected in the SC bioavailability.
Generally, the rate of absorption following SC administration was about 2- to 5-fold higher for most monoclonal antibodies in minipigs than in humans ( and ). This is consistent with previous studies demonstrating that the SC rate of absorption of recombinant human erythropoietin across species scales inversely with body weight.Citation46 Although the exact cause for the faster absorption in minipigs is not known, differences in mAb transport through the extracellular matrix of the SC space prior to lymphatic uptakeCitation12 or differences in the lymphatic transportCitation8–Citation10 may be involved. Nevertheless, it is unlikely that transport of the antibodies through lymphatic vessels is the rate-limiting step for SC absorption because the residence time in both the macro- and micro-lymphatic systems is estimated to be on the order ∼1 h,Citation47–Citation51 which is much shorter than the time scale of SC absorption of mAbs (on the order of days).
The serum clearance, SC bioavailability and rate of absorption of therapeutic mAbs could be affected by many factors, including, but not limited to, nonspecific binding, development of immunogenicity, target-mediated disposition, affinity to FcRn, pI, the site of SC injection and injection depth.Citation7 In this study, an unusually fast clearance was observed for mAb2 in minipig despite normal clearance in human and cynomolgus monkey (data not shown). While the exact cause of the fast clearance of this mAb in the minipig is unknown, it is potentially due to nonspecific binding in minipig tissues causing fast elimination of the antibody, given its clean blood/plasma protein binding profiles ( and B). The fast clearance of antibodies due to off-target binding has been previously reported in both rodents and cynomolgus monkeys.Citation52,Citation53 The unexpected fast clearance of mAb2 in our study is likely a non-generalizable phenomenon specific to this particular antibody, although further monitoring of mAb PK in minipig is warranted. In addition, the PK profiles of adalimumab in minipigs indicate the presence of ATAs, consistent with findings reported in reference Citation21. The effect of ATA on PK of mAbs may be addressed by excluding the periods of accelerated clearance due to ATAs from the PK assessment prior to scaling, as the ATA formation against a human mAb in a laboratory animal bears no relevance to the human situation.Citation54 Further, target-mediated disposition can also play a major role in the clearance of mAbs in vivo. In such cases, a binding species would be more appropriate for studying the target-mediated clearance of mAbs, whereas non-binding species (such as minipig) can still be useful to investigate the linear portion of the clearance pathway. These confounding factors should be considered when using minipig as a predictive model for evaluating human clearance of mAbs.
Since FcRn protects circulating IgG from systemic elimination by recycling it away from the default catabolic pathway in vascular endothelial cells and bone marrow-derived cells,Citation32,Citation37 altering the binding affinity of IgG to FcRn can have a significant impact on the PK of monoclonal antibodies.Citation38,Citation55 In the study reported here, we characterized the in vitro IgG-FcRn interaction using recombinantly expressed human, pig and cynomolgus monkey FcRn in order to systematically investigate the role of FcRn in regulating the serum PK of IgG across these three species. Given the sequence identity between human and pig FcRn (∼76%) and the presence of canonical intracellular trafficking motifs in the pig FcRn cytoplasmic tail (di-leucine and WXX. motifs),Citation56 it is hypothesized that the binding, trafficking and IgG salvage behavior could be similar between the pig and human/cynomolgus monkey FcRn. Indeed, our current results indicate that, as expected, the binding affinities of the human IgG molecules tested are similar across these three species (), thus providing additional rationale for the use of minipigs and cynomolgus monkeys as a translatable species for mAb PK. Notably, to our knowledge, this is the first controlled study to test the affinities of clinically used therapeutic mAbs to FcRn in a comparative PK study across humans, cynomolgus monkeys and pigs.
In addition to the similar binding properties of these IgGs to FcRn, no apparent correlation was observed between the FcRn binding affinity and PK of the mAbs in minipigs or human. This is consistent with recent findings that 3- to 4-fold differences in FcRn-binding affinity arising from mutations in the Fc region of a single human IgG did not result in significant PK differences in cynomolgus monkeys.Citation34 This lack of a correlation suggests that small differences in FcRn binding may not be the major driver for the differences in the PK of mAbs in this case, and that unexpected differences in PK among antibodies with identical constant regions may be due to non-FcRn-dependent mechanisms, such as non-specific or target-mediated clearance.Citation41
We next sought to determine if the PK differences in the mAbs may be due to differences in their pI values since changes in isoelectric point have been shown to affect the PK behaviors of intact antibodies.Citation39–Citation41 The pI values of the mAbs used in this study varied moderately, ranging from 6.1 to 9.4 (). Interestingly, a clear trend was observed for most mAbs tested (with the exception of mAb5), with higher values pI (greater than ∼9.0) tending to be associated with faster systemic clearance rates and lower SC bioavailabilities in both humans and minipig (). This trend is consistent with previous findings showing that increases in the pI of antibodies resulted in increased blood clearance and decreased half-life in vivo due to the nonspecific electrostatic interactions between the anionic cell membrane surface and the antibody.Citation39–Citation41 While these previous observations were primarily made in rodents, our study confirmed these findings in minipigs and humans, utilizing a set of antibodies with different specificities and frameworks. In addition, we demonstrated that pI not only affects systemic clearance, but also potentially SC bioavailability. It is worth noting that in vitro binding studies showed no evidence of protein or cell binding in the minipig plasma or whole blood, respectively, for any of the antibodies tested (), suggesting that the postulated nonspecific interactions driven by mAb pI may take place primarily in tissue, or that the affinity of these interactions may be below the assay detection limit. Taken together, the current study suggests that the characteristics/processes governing systemic clearance and SC bioavailability of the mAbs tested herein are less likely to be dependent upon FcRn, plasma protein or blood cell binding and are possibly related to the electric charge of antibodies and its effect on electrostatic interactions with negatively charged cell surfaces.Citation39 On the other hand, the lack of a clear correlation between human clearance and SC bioavailability may be due to other factors contributing to the clearance or absorption processes, such as target-mediated disposition.
To our knowledge, this study represents the first comprehensive evaluation of the minipig as a potential translational model for human IV and SC PK of therapeutic monoclonal antibodies. The results support the minipig as an alternative, less expensive and sufficiently predictive model over other commonly used species (e.g., cynomolgus monkeys) for evaluation of linear clearance rates and SC bioavailability of mAbs. This is further supported by the similar binding properties of human and pig FcRn for the mAbs tested herein. Moreover, both the systemic clearance and SC bioavailability of most antibodies tested appear to correlate with their pI values, suggesting the nonspecific electrostatic interactions may play a role in both processes in minipigs and humans. Taken together, this study serves as a starting point for the evaluation of the minipig as a potential alternative or better translatable model to predict IV and SC PK of mAbs in humans.
Materials and Methods
Antibodies.
This study used recombinant IgG mAbs that were produced at Genentech or Hoffmann-La Roche (with the exception of adalimumab, which was produced by Abbott Pharmaceuticals and obtained from commercial sources). All non-disclosed mAbs except one were humanized IgGs, while adalimumab is a human IgG. The non-disclosed mAbs were of either IgG1 or stabilized IgG4 subtype, with a molecular weight around 150 kDa. Antibodies were expressed in Chinese hamster ovary cells and were purified using Protein A affinity chromatography followed by size exclusion chromatography. All materials and reagents used for this study were formulated and ensured to be pyrogen-free, either by limulus amebocyte lysate test or material certification. Concentration was measured for all the mAb formulations, which are specified in .
Animals.
Female and male Göttingen minipigs were purchased from Ellegaard Göttingen Minipigs A/S, Dalmose, Denmark or Marshall BioResources, North Rose, NY. Animals were examined and weighed on the day following receipt, and were allowed to acclimate to the laboratory environment for 15–20 d prior to the first day of dosing. Prior to study initiation, animals were also trained repeatedly to be accustomed to the handling procedures for dosing and blood sampling. While on study, animals were housed individually in swine cages or housed jointly in the respective dose group. Housing and care were as specified in the applicable USA-, Danish and UK-regulations.
Intravenous and subcutaneous pharmacokinetic studies in minipigs.
The PK studies of all nine IgGs were conducted in Göttingen minipig across four contract research organizations (CRO): Pipeline Biotech, Trige, Denmark; Charles Rivers Labs, Ohio, USA; Covance Laboratories, Harrogate, UK; and LAB Research, Lille Skensved, Denmark. Before each study began, the animals were quarantined and acclimated for at least 7 d. During this period, they were weighed, physically examined by a staff veterinarian and determined to be healthy at the beginning of each study. Protocols were reviewed and approved by the Institutional Animal Care and Use Committee at each CRO. Minipigs were assigned into groups of three to five animals each, with similar average body weight in the SC and IV groups for the respective test substance. Animals received a single IV bolus dose of test article either via jugular cannula or via a catheter in the ear vein. SC dosing was done in the scapular (20 g needle) or inguinal area (27 g needle) (). Blood samples were collected from the femoral or jugular vein for each animal at pre-dose and multiple time points up to 28 d post-dose. Following the final blood collection on Day 28, all surviving animals were either returned to the stock population of the respective laboratory or euthanized by sodium pentobarbital injection followed by exsanguination and discarded.
Samples collection and processing.
For preparation of serum samples, PK blood samples were collected into serum separator tubes (with clot activator) or into plain tubes. Samples were allowed to clot at room temperature for at least 20 min, but no longer than 1 h. The clotted samples were maintained at room temperature until centrifuged, commencing within 1 h after the collection time, at a relative centrifugal force of 2,000x g for 10 min in a refrigerated centrifuge set to maintain 4°C or, alternatively, at 3,500x g for 10 min at room temperature. The serum was separated from each of the blood samples within 20 min after centrifugation and transferred into two approximately equal aliquots of 0.5 mL each. Samples were held on dry ice until stored in a freezer set to maintain −60°C to −80°C. In the studies with adalimumab, mAb5 and mAb8, plasma was prepared using EDTA or heparin as anticoagulants.
Analysis of serum/plasma samples.
Serum or plasma samples were assayed for mAbs 1 through 7 concentrations using enzyme linked immunosorbent assays (ELISA), where each analyte was captured using a recombinant human protein specific for that analyte. MAb8 and adalimumab were analyzed with a generic human IgG ELISA using an anti-human Fc antibody for both capture and detection. Minimum quantifiable concentrations for each assay were: mAb1, 65 ng/mL; mAb2, 280 ng/mL; mAb3, 81 ng/mL; mAb4, 81 ng/mL; mAb5, 391 ng/mL; mAb6, 500 ng/mL; mAb7, 20 ng/mL; mAb8, 12.5 ng/mL; and adalimumab, 12.5 ng/mL.
Pharmacokinetic data analysis.
The serum mAb concentration-time data for all mAbs following IV or SC administration were analyzed by compartmental PK analysis using nonlinear mixed effects modeling. A two-compartmental model with first-order absorption and elimination kinetics was used to simultaneously fit the concentration-time profiles from individual animals following a single IV or SC injection for most antibodies. For mAb7, an additional nonlinear elimination term was included. The structural model is composed of two compartments with linear clearance from the first compartment (“central compartment”) and linear inter-compartment exchange. Additionally, first-order absorption from an absorption site compartment was assumed in the case of SC administration. The structure model is demonstrated in:
Eq. 1
Eq. 1
where Aabs is the amount of the drug in the absorption site depot, Cc and Cp are the drug concentrations in the “central” and “peripheral” compartments, respectively. The structural model parameters included clearance (CL), volume of distribution of the central compartment (Vc) and peripheral compartment (Vp), inter-compartmental clearance (Q), first-order rate of absorption (Ka) and bioavailability or fraction absorbed (F) for subcutaneous administration. In the case of mAb7, the linear clearance term was substituted by the sum of a linear and a saturable (nonlinear) term as follows:
Eq. 2
Eq. 2
Inter-individual differences of parameters were modeled by log-normal distribution. Proportional and additive error models were used for the residual errors of the observed concentration data. For mAb1–4 the modeling was performed using NONMEM (version VI; UCSF; San Francisco, CA), using the FOCE method. For mAb5–8 and adalimumab, the nonlinear mixed effect modeling software MONOLIX 3.1 Citation57 was used running on MATLAB 7.9.0.529 (R2009b) (The MathWorks, Inc., Natick, MA USA), with the structural model implemented in MLXTRAN. The observed time to maximum concentration (Tmax) values were obtained directly from the observed data using WinNonlin Pro (version 5.0.1; Pharsight Corporation; Mountain View, CA USA) or ToxKin 3.5 (Entimo, Berlin, Germany).
Inter-species scaling of CL.
The clearance data in minipigs (CLminipigs) were extrapolated to clearance in humans (CLhumans) using the allometric equation:
Eq. 3
Eq. 3
where w is the scaling exponent for clearance. Based on the observed mean CLminipigs and CLhumans and the average body weights of the minipigs in the respective studies and the typical body weight of humans (70 kg), w was calculated for each antibody using EquationEq. 3
Eq. 3
Eq. 3 . Human CL data at doses that saturated the target-mediated CL were used in the analysis for mAbs with relevant target-mediated CL in humans.
Cloning of human, pig and cynomolgus monkey FcRn.
The coding regions of the cynomolgus monkey, human and pig FcRn α-chain ectodomain and the cognate full-length β2-microglobulin light chain (β2m) genes were generated by gene synthesis (Blue Heron, USA). The coding regions of FcRn and recombinant β2m were subcloned into a previously described pRK mammalian cell expression vector.Citation58 For expression and purification of FcRn constructs, human embryonic kidney cells 293 were transfected using FUGENE (Roche) according to the manufacturer's protocol. After 24 h of incubation with transfection complexes, cells were switched to serum-free PSO4 medium (Genentech; 1 g/L Pluronic F-68, 5.5 g/L combination nonselect medium (Life Technologies), 4.3 g/L glucose, 1.22 g/L sodium bicarbonate, 0.1 g/L gentamicin sulfate (pH 7.1); 350 milliosmolar) supplemented with 5 mg/L recombinant bovine insulin and trace elements and grown for 7 d. Cells were collected by centrifugation and soluble FcRn was purified from the culture supernatants by pH-dependent binding to human IgG-Sepharose (Amersham). Briefly, supernatants were acidified to pH 5.8 with 50 mM MES and flowed over a 4 mL hIgG-Sepharose column at ∼1.5 mL/min. After washing with >10 column volumes of wash buffer (20 mM MES, 150 mM NaCl, pH 5.8), bound FcRn was eluted with 20 mM HEPES, 150 mM NaCl, pH 8.0. Eluted FcRn was concentrated and further purified by size exclusion chromatography on a Superdex 200 column (Amersham) with PBS pH 6.0 as the running buffer. Fractions containing monomeric FcRn were pooled, and the concentration was determined on a Nanodrop 8000 spectrometer (Thermo Scientific) using the species-specific mass extinction coefficient at 280 nm.
Affinity measurements binding to human, pig and cynomolgus monkey FcRn.
Binding kinetics and affinity studies were performed on purified FcRn by surface plasmon resonance (SPR) using a Biacore T-100™ instrument (GE Healthcare, Piscataway, NJ). All experiments were performed at 25°C. IgGs (5–10 µg/mL) were immobilized onto three of the individual flow cells (FC) of a Series S CM5 sensor chip (GE Healthcare), using a standard amine coupling procedure according to the manufacturer's protocol, with FC1 serving as the reference flow cell. The immobilization levels were approximately 1,000 response units (RU) per flow cell. Eight serial 3-fold dilutions of each FcRn (10 µM-1.5 nM) were prepared in running buffer (25 mM MES, 25 mM HEPES, pH 5.8, 150 mM NaCl, 0.05% Tween-20) and were injected for 60 sec at a flow rate of 50 µL/minute followed by a dissociation phase of 30 sec. Surfaces were regenerated between cycles by a single injection of running buffer at pH 8.0 (30 sec at 50 µL/min). Raw sensogram data were reduced and referenced using the Scrubber II software package (BioLogic Software, Campbell, Australia) and fit to a simple 1:1 binding model under equilibrium conditions.
In vitro blood binding studies.
All antibodies were radioiodinated using the indirect iodogen addition method as previously described in reference Citation59. The radiolabeled proteins were purified using NAP5™ columns pre-equilibrated in PBS. The specific activities of the antibodies were in the range of 11.5 to 15.5 µCi/µg. Radioiodinated antibodies were spiked into Göttingen minipig whole blood (Bioreclamation LLC, Hicksville, NY) followed by gentle mixing. Three 0.5 mL aliquots of blood were removed and incubated for 1 h at 37°C. The aliquots were centrifuged at 12,800x g for 5 min at 4°C to separate the plasma samples from the cell pellets, after which the cell pellets were washed with 0.5 mL cold PBS. All samples were counted for radioactivity on a gamma counter (Wallac 1480 Wizard 3” EC&G Wallac; Turku, Finland).
The plasma samples were subsequently analyzed by size-exclusion high performance liquid chromatography (HPLC). Size-exclusion HPLC separation was performed on a Phenomenex™ BioSep-SEC-S 3000, 300 × 7.8 mm, 5 µm column. The mobile phase was PBS and the flow rate was 0.5 mL/min (isocratic) for 30 min. The ChemStation analog-to-digital converter was set to 25,000 units/mV, peak width 2 sec, slit 4 nM (Agilent Technologies). Iodine-125 was detected with a raytest Ramona 90 in line with a standard Agilent 1100 HPLC module system. The plasma samples were diluted 1:1 with PBS prior to loading on the column.
pI determination by isoelectric focusing (IEF).
The pIs of native mAbs except mAb5 and adalimumab were determined by imaged capillary isoelectric focusing (iCIEF) using an iCE280 analyzer (ProteinSimple, Toronto, Canada). Solutions of anolyte, catholyte and pI markers were purchased from ProteinSimple. The pI of mAb5 was determined by conventional gel IEF on Immobiline™ Dry Strip pH 6–11 gel (18 cm: GE Healthcare Bio-Sciences).
Disclosure of Potential Conflicts of Interest
All authors are employees of Genentech, Inc., a member of the Roche Group or F. Hoffmann-La Roche and are Roche stockholders.
Abbreviations
Aabs | = | amount of the drug in the absorption site depot |
ATA | = | anti-therapeutic antibodies |
F | = | bioavailability/fraction absorbed |
β2m | = | β2-microglobulin |
CL | = | clearance |
CLhumans | = | clearance in humans |
CLminipigs | = | clearance in minipig |
Cc | = | drug concentration in the central compartment |
Cp | = | drug concentrations in the peripheral compartment |
CRO | = | contract research organizations |
ELISA | = | enzyme linked immunosorbent assay |
FC | = | flow cell |
FcRn | = | neonatal Fc receptor |
HPLC | = | high performance liquid chromatography |
IEF | = | isoelectric focusing |
IV | = | intravenous |
Q | = | inter-compartmental clearance |
pI | = | isoelectric point |
Ka | = | rate of absorption |
KD | = | equilibrium dissociation constant |
mAb | = | monoclonal antibody |
PK | = | pharmacokinetic |
PD | = | pharmacodynamic |
RU | = | response unit |
SC | = | subcutaneous |
SPR | = | surface plasmon resonance |
Vc | = | volume of distribution of the central compartment |
Vp | = | volume of distribution of the peripheral compartment |
w | = | scaling exponent for clearance |
Figures and Tables
Figure 1 Dose-normalized plasma or serum concentration-time curves following intravenous (closed symbols) or subcutaneous (open symbols) administration to Göttingen minipigs of (A) mAb1, (B) mAb2, (C) mAb3, (D) mAb4, (E) mAb5, (F) mAb6, (G) mAb7, (H) adalimumab and (I) mAb8 (intravenous dosing only) (mean ± SD).
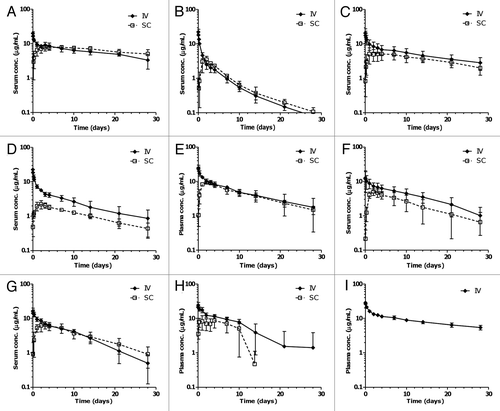
Figure 2 Correlation between minipig and human (A) clearance and (B) subcutaneous bioavailability following intravenous and subcutaneous administration of various monoclonal antibodies. Circles represent the reported mean parameter values while error bars represent the standard errors of the estimate; solid lines represent the linear regression lines (with r2 values displayed), and dashed lines represent the hypothetical line where the minipig and human values are identical. Note: in cases when more than one bioavailability value was reported, the average of all the reported values was used for the correlation analysis. For (A), mAb2 is excluded.
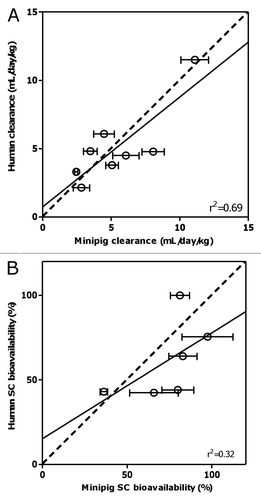
Figure 3 Correlation between clearance and subcutaneous bioavailability of the various mAbs in (A) minipig and (B) human. Black dots represent the reported mean parameter values while error bars represent the standard errors of estimate. For (A), mAb2 is excluded; solid line represents the linear regression line (with r2 value displayed).
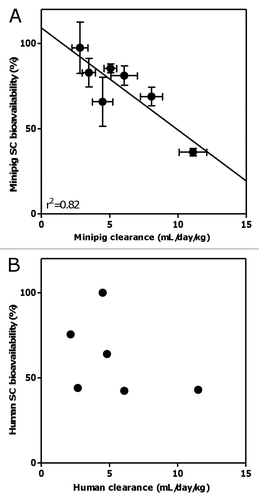
Figure 4 Correlation between pI and clearance in minipig (A) and human (B), and SC bioavailability in minipig (C) and human (D) of various monoclonal antibodies. Solid dots represent the reported mean parameter values while vertical error bars represent the standard errors of estimate. For (A), mAb2 is excluded. Grey dots represent mAb5 (outlier) whereas black dots represent all other mAbs.
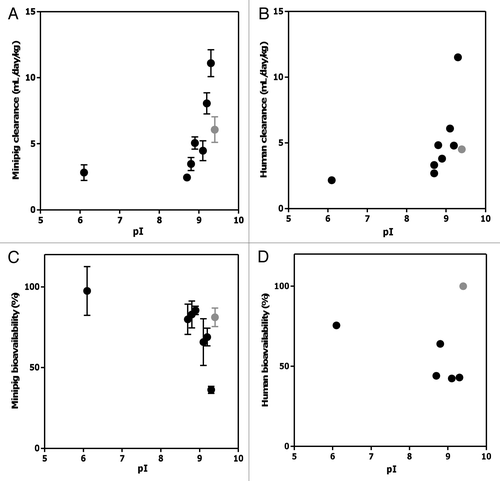
Figure 5 (A) Percent radioactivity remaining in the cell pellets following a 1 h incubation at 37°C of radioiodinated antibodies in minipig whole blood. (B) Size-exclusion HPLC radiochromatograms of [125I]-antibodies in plasma isolated from minipig whole blood following 1 h incubation.
![Figure 5 (A) Percent radioactivity remaining in the cell pellets following a 1 h incubation at 37°C of radioiodinated antibodies in minipig whole blood. (B) Size-exclusion HPLC radiochromatograms of [125I]-antibodies in plasma isolated from minipig whole blood following 1 h incubation.](/cms/asset/3cdcff8b-daae-4d18-8a9c-41c522c18c53/kmab_a_10919387_f0005.gif)
Table 1 Minipig studies: Pharmacokinetic parameters after intravenous and subcutaneous dosing for different monoclonal antibodies (mAbs)
Table 2 Allometric scaling of clearance for various monoclonal antibodies from minipigs to humans
Table 3 Human rate of absorption and bioavailability after subcutaneous dosing for different monoclonal antibodies
Table 4 Isoelectric points (pI) and in vitro FcRn binding affinities (KD) at pH 6 (mean and standard error)
Table 5 Experimental conditions of in vivo pharmacokinetic studies in Göttingen minipigs
Acknowledgments
This study was supported financially by Genentech, Inc., a member of the Roche Group and F. Hoffmann-La Roche. We would like to thank Wayne Kung, Carolina Chou and Joseph Meyer for coordinating the minipig PK studies, Isabelle Bauer Dauphin, Ulla Grauschopf, Michael Adler, Sreedhara Alavattam, Jamie Moore, Mechelle Carnine, Osi Esue and Srikanth Chary for providing the dosing formulations, Sirj Goswami for carrying out part of the pharmacokinetic studies, David Michels for conducting the pI measurements, Zhenling Yao, Victor Yip and Crystal Zhang for their helpful discussions. We also would like to express appreciation to the key personnel involved in conducting the studies across the four contract research organizations (Pipeline Biotech, Trige, Denmark; Charles Rivers Labs, Ohio, USA; Covance Laboratories, Harrogate, UK; and LAB Research, Lille Skensved, Denmark).
References
- Green MD, Hartsough M. The Role of Pharmacokinetics and Pharmacodynamics in Selecting a Relevant Species. Preclinical Safety Evaluation of Biopharmaceuticals 2007; John Wiley & Sons, Inc. 277 - 291
- Mould DR, Green B. Pharmacokinetics and pharmacodynamics of monoclonal antibodies: concepts and lessons for drug development. BioDrugs 2010; 24:23 - 39; PMID: 20055530; http://dx.doi.org/10.2165/11530560-000000000-00000
- Chapman K, Pullen N, Graham M, Ragan I. Preclinical safety testing of monoclonal antibodies: the significance of species relevance. Nat Rev Drug Discov 2007; 6:120 - 126; PMID: 17268483; http://dx.doi.org/10.1038/nrd2242
- Deng R, Iyer S, Theil FP, Mortensen DL, Fielder PJ, Prabhu S. Projecting human pharmacokinetics of therapeutic antibodies from nonclinical data: what have we learned?. MAbs 2011; 3:61 - 66; PMID: 20962582; http://dx.doi.org/10.4161/mabs.3.1.13799
- Ling J, Zhou H, Jiao Q, Davis HM. Interspecies scaling of therapeutic monoclonal antibodies: initial look. J Clin Pharmacol 2009; 49:1382 - 1402; PMID: 19837907; http://dx.doi.org/10.1177/0091270009337134
- Oitate M, Masubuchi N, Ito T, Yabe Y, Karibe T, Aoki T, et al. Prediction of human pharmacokinetics of therapeutic monoclonal antibodies from simple allometry of monkey data. Drug Metab Pharmacokinet 2011; 26:423 - 430; PMID: 21606605; http://dx.doi.org/10.2133/dmpk.DMPK-11-RG-011
- McDonald TA, Zepeda ML, Tomlinson MJ, Bee WH, Ivens IA. Subcutaneous administration of biotherapeutics: current experience in animal models. Curr Opin Mol Ther 2010; 12:461 - 470; PMID: 20677097
- McLennan DN, Porter CJH, Charman SA. Subcutaneous drug delivery and the role of the lymphatics. Drug Discov Today Technol 2005; 2:89 - 96; http://dx.doi.org/10.1016/j.ddtec.2005.05.006
- Porter CJ, Charman WN. Transport and absorption of drugs via the lymphatic system. Adv Drug Deliv Rev 2001; 50:1 - 2; PMID: 11489330; http://dx.doi.org/10.1016/S0169-409X(01)00146-6
- Porter CJ, Charman SA. Lymphatic transport of proteins after subcutaneous administration. J Pharm Sci 2000; 89:297 - 310; PMID: 10707011; http://dx.doi.org/10.1002/(SICI)1520-6017(200003)89:3<297::AID-JPS2>3.0.CO
- Beshyah SA, Anyaoku V, Niththyananthan R, Sharp P, Johnston DG. The effect of subcutaneous injection site on absorption of human growth hormone: abdomen versus thigh. Clin Endocrinol (Oxf) 1991; 35:409 - 412; PMID: 1814654; http://dx.doi.org/10.1111/j.1365-2265.1991.tb03557.x
- Frost GI. Recombinant human hyaluronidase (rHuPH20): an enabling platform for subcutaneous drug and fluid administration. Expert Opin Drug Deliv 2007; 4:427 - 440; PMID: 17683255; http://dx.doi.org/10.1517/17425247.4.4.427
- Forster R, Bode G, Ellegaard L, van der Laan JW. Steering Group of the RETHINK Project. The RETHINK project—minipigs as models for the toxicity testing of new medicines and chemicals: an impact assessment. J Pharmacol Toxicol Methods 2010; 62:158 - 159; PMID: 20685395; http://dx.doi.org/10.1016/j.vascn.2010.05.003
- Dolgin E. Minipig, minipig, let me in. Nat Med 2010; 16:1349; PMID: 21135823; http://dx.doi.org/10.1038/nm1210-1349a
- Bronaugh RL, Stewart RF, Congdon ER. Methods for in vitro percutaneous absorption studies. II. Animal models for human skin. Toxicol Appl Pharmacol 1982; 62:481 - 488; PMID: 7071863; http://dx.doi.org/10.1016/0041-008X(82)90149-1
- Washer G, Makin A. Minipigs as an alternative non-rodent species in toxicology and safety studies 2008; Seattle Society of Toxicology
- Ellegaard L, Cunningham A, Edwards S, Grand N, Nevalainen T, Prescott M, et al. Steering Group of the RETHINK Project. Welfare of the minipig with special reference to use in regulatory toxicology studies. J Pharmacol Toxicol Methods 2010; 62:167 - 183; PMID: 20621655; http://dx.doi.org/10.1016/j.vascn.2010.05.006
- Qvist MH, Hoeck U, Kreilgaard B, Madsen F, Frokjaer S. Evaluation of Göttingen minipig skin for transdermal in vitro permeation studies. Eur J Pharm Sci 2000; 11:59 - 68; PMID: 10913754; http://dx.doi.org/10.1016/S0928-0987(00)00091-9
- Xing QF, Lin S, Chien YW. Transdermal testosterone delivery in castrated Yucatan minipigs: pharmacokinetics and metabolism. J Control Release 1998; 52:89 - 98; PMID: 9685939; http://dx.doi.org/10.1016/S0168-3659(97)00190-9
- Nobilis M, Kopecký J, Kvetina J, Svoboda Z, Pour M, Kunes J, et al. Comparative biotransformation and disposition studies of nabumetone in humans and minipigs using high-performance liquid chromatography with ultraviolet, fluorescence and mass spectrometric detection. J Pharm Biomed Anal 2003; 32:641 - 656; PMID: 12899954; http://dx.doi.org/10.1016/S0731-7085(03)00171-7
- van Mierlo G, Cnubben NLA, Wouters D, Wolbink GJ, Gandsrup NC, et al. Immunogenicity testing of Adalimumab and Infliximab in Göttingen Minipigs. Society of Toxicology 2011; Washington DC Society of Toxicology
- Penninks A, Wolthoorn J, Cnubben N, Gandsrup NC, van Mierlo G. Immunogenicity testing of Kineret in Göttingen minipigs. Society of Toxicology 2011; Washington DC Society of Toxicology
- Lin S, Chen LL, Chien YW. Comparative pharmacokinetic and pharmacodynamic studies of human insulin and analogues in chronic diabetic Yucatan minipigs. J Pharmacol Exp Ther 1998; 286:959 - 966; PMID: 9694956
- Harvey AJ, Kaestner SA, Sutter DE, Harvey NG, Mikszta JA, Pettis RJ. Microneedle-based intradermal delivery enables rapid lymphatic uptake and distribution of protein drugs. Pharm Res 2011; 28:107 - 116; PMID: 20354765; http://dx.doi.org/10.1007/s11095-010-0123-9
- Chen SA, Sawchuk RJ, Brundage RC, Horvath C, Mendenhall HV, Gunther RA, et al. Plasma and lymph pharmacokinetics of recombinant human interleukin-2 and polyethylene glycol-modified interleukin-2 in pigs. J Pharmacol Exp Ther 2000; 293:248 - 259; PMID: 10734176
- Jacobs A. Use of nontraditional animals for evaluation of pharmaceutical products. Expert Opin Drug Metab Toxicol 2006; 2:345 - 349; PMID: 16863438; http://dx.doi.org/10.1517/17425255.2.3.345
- Sharma R, Wang W, Rasmussen JC, Joshi A, Houston JP, Adams KE, et al. Quantitative imaging of lymph function. Am J Physiol Heart Circ Physiol 2007; 292:3109 - 3118; PMID: 17307997; http://dx.doi.org/10.1152/ajpheart.01223.2006
- Hammond SA, Tsonis C, Sellins K, Rushlow K, Scharton-Kersten T, Colditz I, et al. Transcutaneous immunization of domestic animals: opportunities and challenges. Adv Drug Deliv Rev 2000; 43:45 - 55; PMID: 10967220; http://dx.doi.org/10.1016/S0169-409X(00)00076-4
- Rose EH, Vistnes LM, Ksander GA. The panniculus carnosus in the domestic pig. Plast Reconstr Surg 1977; 59:94 - 97; PMID: 831245; http://dx.doi.org/10.1097/00006534-197701000-00017
- Kawamata S, Ozawa J, Hashimoto M, Kurose T, Shinohara H. Structure of the rat subcutaneous connective tissue in relation to its sliding mechanism. Arch Histol Cytol 2003; 66:273 - 279; PMID: 14527168; http://dx.doi.org/10.1679/aohc.66.273
- Sidhu SS. Full-length antibodies on display. Nat Biotechnol 2007; 25:537 - 538; PMID: 17483836; http://dx.doi.org/10.1038/nbt0507-537
- Roopenian DC, Akilesh S. FcRn: the neonatal Fc receptor comes of age. Nat Rev Immunol 2007; 7:715 - 725; PMID: 17703228; http://dx.doi.org/10.1038/nri2155
- Kim JK, Tsen MF, Ghetie V, Ward ES. Catabolism of the murine IgG1 molecule: evidence that both CH2-CH3 domain interfaces are required for persistence of IgG1 in the circulation of mice. Scand J Immunol 1994; 40:457 - 465; PMID: 7939418; http://dx.doi.org/10.1111/j.1365-3083.1994.tb03488.x
- Yeung YA, Leabman MK, Marvin JS, Qiu J, Adams CW, Lien S, et al. Engineering human IgG1 affinity to human neonatal Fc receptor: impact of affinity improvement on pharmacokinetics in primates. J Immunol 2009; 182:7663 - 7671; PMID: 19494290; http://dx.doi.org/10.4049/jimmunol.0804182
- Akilesh S, Christianson GJ, Roopenian DC, Shaw AS. Neonatal FcR expression in bone marrow-derived cells functions to protect serum IgG from catabolism. J Immunol 2007; 179:4580 - 4588; PMID: 17878355
- Suzuki T, Ishii-Watabe A, Tada M, Kobayashi T, Kanayasu-Toyoda T, Kawanishi T, et al. Importance of neonatal FcR in regulating the serum half-life of therapeutic proteins containing the Fc domain of human IgG1: a comparative study of the affinity of monoclonal antibodies and Fc-fusion proteins to human neonatal FcR. J Immunol 2010; 184:1968 - 1976; PMID: 20083659; http://dx.doi.org/10.4049/jimmunol.0903296
- Tabrizi MA, Tseng CM, Roskos LK. Elimination mechanisms of therapeutic monoclonal antibodies. Drug Discov Today 2006; 11:81 - 88; PMID: 16478695; http://dx.doi.org/10.1016/S1359-6446(05)03638-X
- Petkova SB, Akilesh S, Sproule TJ, Christianson GJ, Al Khabbaz H, Brown AC, et al. Enhanced half-life of genetically engineered human IgG1 antibodies in a humanized FcRn mouse model: potential application in humorally mediated autoimmune disease. Int Immunol 2006; 18:1759 - 1769; PMID: 17077181; http://dx.doi.org/10.1093/intimm/dxl110
- Boswell CA, Tesar DB, Mukhyala K, Theil FP, Fielder PJ, Khawli LA. Effects of charge on antibody tissue distribution and pharmacokinetics. Bioconjug Chem 2010; 21:2153 - 2163; PMID: 21053952; http://dx.doi.org/10.1021/bc100261d
- Khawli LA, Mizokami MM, Sharifi J, Hu P, Epstein AL. Pharmacokinetic characteristics and biodistribution of radioiodinated chimeric TNT-1, -2 and -3 monoclonal antibodies after chemical modification with biotin. Cancer Biother Radiopharm 2002; 17:359 - 370; PMID: 12396700; http://dx.doi.org/10.1089/108497802760363150
- Igawa T, Tsunoda H, Tachibana T, Maeda A, Mimoto F, Moriyama C, et al. Reduced elimination of IgG antibodies by engineering the variable region. Protein Eng Des Sel 2010; 23:385 - 392; PMID: 20159773; http://dx.doi.org/10.1093/protein/gzq009
- Davies B, Morris T. Physiological parameters in laboratory animals and humans. Pharm Res 1993; 10:1093 - 1095; PMID: 8378254; http://dx.doi.org/10.1023/A:1018943613122
- Simianer H, K”hn F. Genetic management of the G”ttingen Minipig population. J Pharmacol Toxicol Methods 2010; 62:221 - 226; PMID: 20570747; http://dx.doi.org/10.1016/j.vascn.2010.05.004
- van der Laan JW, Brightwell J, McAnulty P, Ratky J, Stark C, Steering Group of the RETHINK Project. Regulatory acceptability of the minipig in the development of pharmaceuticals, chemicals and other products. J Pharmacol Toxicol Methods 2010; 62:184 - 195; PMID: 20601024; http://dx.doi.org/10.1016/j.vascn.2010.05.005
- Wang W, Prueksaritanont T. Prediction of human clearance of therapeutic proteins: simple allometric scaling method revisited. Biopharm Drug Dispos 2010; 31:253 - 263; PMID: 20437464
- Woo S, Jusko WJ. Interspecies comparisons of pharmacokinetics and pharmacodynamics of recombinant human erythropoietin. Drug Metab Dispos 2007; 35:1672 - 1678; PMID: 17576810; http://dx.doi.org/10.1124/dmd.107.015248
- Leu AJ, Berk DA, Yuan F, Jain RK. Flow velocity in the superficial lymphatic network of the mouse tail. Am J Physiol 1994; 267:1507 - 1513; PMID: 7943396
- Berk DA, Swartz MA, Leu AJ, Jain RK. Transport in lymphatic capillaries. II. Microscopic velocity measurement with fluorescence photobleaching. Am J Physiol 1996; 270:330 - 337; PMID: 8769769
- Modi S, Stanton AW, Mortimer PS, Levick JR. Clinical assessment of human lymph flow using removal rate constants of interstitial macromolecules: a critical review of lymphoscintigraphy. Lymphat Res Biol 2007; 5:183 - 202; PMID: 18035937; http://dx.doi.org/10.1089/lrb.2007.5306
- Modi S, Stanton AW, Svensson WE, Peters AM, Mortimer PS, Levick JR. Human lymphatic pumping measured in healthy and lymphoedematous arms by lymphatic congestion lymphoscintigraphy. J Physiol 2007; 583:271 - 285; PMID: 17569739; http://dx.doi.org/10.1113/jphysiol.2007.130401
- Fischer M, Franzeck UK, Herrig I, Costanzo U, Wen S, Schiesser M, et al. Flow velocity of single lymphatic capillaries in human skin. Am J Physiol 1996; 270:358 - 363; PMID: 8769772
- Vugmeyster Y, Szklut P, Wensel D, Ross J, Xu X, Awwad M, et al. Complex pharmacokinetics of a humanized antibody against human amyloid beta peptide, anti-abeta Ab2, in nonclinical species. Pharm Res 2011; 28:1696 - 1706; PMID: 21424161; http://dx.doi.org/10.1007/s11095-011-0405-x
- Bumbaca D, Wong A, Drake E, Reyes AE 2nd, Lin BC, Stephan JP, et al. Highly specific off-target binding identified and eliminated during the humanization of an antibody against FGF receptor 4. MAbs 2011; 3:376 - 386; PMID: 21540647; http://dx.doi.org/10.4161/mabs.3.4.15786
- Richter WF, Gallati H, Schiller CD. Animal pharmacokinetics of the tumor necrosis factor receptor-immunoglobulin fusion protein lenercept and their extrapolation to humans. Drug Metab Dispos 1999; 27:21 - 25; PMID: 9884305
- Ghetie V, Popov S, Borvak J, Radu C, Matesoi D, Medesan C, et al. Increasing the serum persistence of an IgG fragment by random mutagenesis. Nat Biotechnol 1997; 15:637 - 640; PMID: 9219265; http://dx.doi.org/10.1038/nbt0797-637
- Zhao Y, Kacskovics I, Zhao Z, Hammarström L. Presence of the di-leucine motif in the cytoplasmic tail of the pig FcRn alpha chain. Vet Immunol Immunopathol 2003; 96:229 - 233; PMID: 14592735; http://dx.doi.org/10.1016/j.vetimm.2003.08.006
- Lavielle M, Mesa H, Chatel K. MONOLIX 3.1 2009; INRIA http://software.monolix.org/sdoms/software/
- Eaton DL, Wood WI, Eaton D, Hass PE, Hollingshead P, Wion K, et al. Construction and characterization of an active factor VIII variant lacking the central one-third of the molecule. Biochemistry 1986; 25:8343 - 8347; PMID: 3030393; http://dx.doi.org/10.1021/bi00374a001
- Chizzonite R, Truitt T, Podlaski FJ, Wolitzky AG, Quinn PM, Nunes P, et al. IL-12: monoclonal antibodies specific for the 40-kDa subunit block receptor binding and biologic activity on activated human lymphoblasts. J Immunol 1991; 147:1548 - 1556; PMID: 1715362
- den Broeder A, van de Putte L, Rau R, Schattenkirchner M, Van Riel P, Sander O, et al. A single dose, placebo controlled study of the fully human anti-tumor necrosis factor-alpha antibody adalimumab (D2E7) in patients with rheumatoid arthritis. J Rheumatol 2002; 29:2288 - 2298; PMID: 12415583
- Santora LC, Krull IS, Grant K. Characterization of recombinant human monoclonal tissue necrosis factor-alpha antibody using cation-exchange HPLC and capillary isoelectric focusing. Anal Biochem 1999; 275:98 - 108; PMID: 10542114; http://dx.doi.org/10.1006/abio.1999.4275