Abstract
During neurogenesis, multiple regulatory networks integrate extracellular and intracellular signals to ensure proper final numbers for each neuronal subtype at the end of the developmental process. The Activin/TGF-β signaling cascade is one of the main players of neurogenesis in vertebrates, balancing proliferation and differentiation of neural stem cells by regulating gene expression via the R-Smads transcription factors. Despite their equivalent upstream activation mechanism, Smad2 and Smad3 functions can be redundant or opposite depending on the particular context of the cell. We demonstrate that R-Smad simultaneously cooperate and antagonize in the regulation of gene expression in the context of vertebrate neurogenesis. We propose a model where synergism and antagonism appear as a consequence of the competition between Smad2 and Smad3 to form the different transcriptionally active heterotrimers with Smad4. This dual interplay of R-Smads significantly modulates the role of TGF-β pathway in the balance between proliferation and differentiation in the developing vertebrate spinal cord.
Transforming growth factor β (TGF-β) superfamily is one of the main classes of multi-tasking secreted proteins that drives animal development.Citation1 There are 2 branches in this family: bone morphogenic proteins (BMP) and Nodal/Activin/TGF-β, which orchestrate several key processes during embryogenesis.Citation2 Acting through Smad transcription factors, they regulate a variety of cellular functions including cell proliferation, growth arrest, differentiation and migration. The canonical TGF-β pathway begins with the binding of TGF-β ligands to different isoforms of type II and I Ser/Thr kinase receptors at the cell membrane.Citation3 The ligand promotes receptor dimerization, enabling the phosphorylation and activation of the receptor type I by the type II. Specifically, activated Nodal/Activin/TGF-β receptor complexes recruit and phosphorylate Smad2 and Smad3 proteins, which oligomerize with Smad4 to form transcriptionally active heterotrimers. These active complexes translocate into the nucleus where they regulate gene expression ().Citation4-Citation6
Figure 1. Scheme of the TGF-β pathway. (A) (1) Dimers of soluble Activin or TGF-β ligands bind their specific Ser/Thr kinase receptor type II, presented by the co-receptor betaglycan. (2) The complex oligomerizes and phosphorylates receptor type I and finally forms the tetrameric active ligand-receptor complex. (3) This complex phosphorylates R-Smad transcription factors (Smad2 in green and Smad3 in blue), recruited to plasma membrane by the anchor protein SARA. (4) Heterodimers or homodimers of R-Smad bind the Co-Smad (Smad4) to form the transcriptionally active heterotrimers. (5) Smad oligomers are actively transported to the nucleus, where (6) they bind to specific promoter regions and regulate gene expression. Specifically, Smad2-Smad2-Smad4 homotrimer is recruited to ARE while Smad3-Smad3-Smad4 homotrimer and Smad3-Smad2-Smad4 heterotrimer bind to CAGA box in the promoter regions of their target genes. (B) In the basal state Smad2 directed transcription (ARE) is accomplished only by Smad2-Smad2-Smad4 homotrimer while Smad3 targets (CAGA box) are regulated by both Smad3-Smad2-Smad4 and Smad3-Smad3-Smad4 trimers. Increasing the levels of Smad2 (Smad2 GOF) shifts the balance to overexpress Smad2 targets (ARE). Similarly, Smad3 GOF leads to overexpression of Smad3 targets. Depletion of Smad2 protein (Smad2 LOF) contributes not only to downregulate Smad2 targets, but also to increase Smad3 driven transcription.
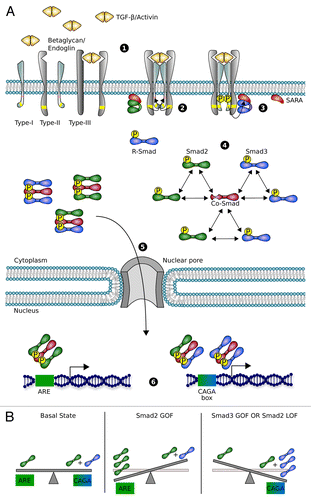
Despite this apparently simple mechanism of activation, the Nodal/Activin/TGF-β pathway plays major and diverse roles during embryo development. The concentration gradient of Nodal is essential for the establishment of the anterior–posterior (A/P) axis and the induction of the mesendoderm layer. Subsequently, together with BMPs, they organize the left–right (L/R) asymmetry, finishing the specification of the body plan.Citation1,Citation7 Counteracting BMP and Shh gradients regulate closure and dorsal–ventral (D/V) polarization of the neural tube.Citation8-Citation10 BMP/Activin ligands are expressed dorsally in the newly originated neural tube,Citation11 whereas TGF-β 2, 3 isoforms are preferentially expressed in the notochord and the floor plate,Citation12,Citation13 concomitant with Shh expression. The opposing gradients of these ligands are involved in the specification pattern for each neuronal subtype in the neural tube. Nonetheless, the particular functions of each member of the Activin/TGF-β subfamily during neurogenesis are beginning to be understood.
In the dorsal pole of the neural tube, Activin signaling promotes the formation of the dorsal interneuron 3 (dI3) precursor cells.Citation14 Besides, TGF-β factors contribute to regulate the balance between proliferation and differentiation of neural stem cells in conjunction with other pathways. TGF-β counterbalances the proliferative signals of IGF1/AKT in the forebrain and Wnt/FGF8 in the midbrain.Citation15,Citation16 In addition, TGF-β1 signaling participates in neuronal differentiation by modulating the expression of regulators of the cell cycle,Citation17,Citation18 whereas the isoforms TGF-β2,3 are essential for the specification of dopaminergic neurons induced by Shh.Citation19
The role of Activin/TGF-β signaling in the regulation of neurogenesis is ultimately mediated by the downstream transcription factors Smad2 and Smad3. Particularly, Smad3 triggers the differentiation program of ventral interneuron progenitors through transcription of bHLH proteins such as NeuroM and Mash1, inhibition of Id1,2,Citation20,Citation21 and promoting cell-cycle exit by transcribing cell-cycle inhibitors p15, p21, or p27.Citation17,Citation20,Citation21 In contrast, Smad2 activity is correlated with Nodal signaling throughout embryogenesis, including A/P patterning and endoderm formation, although its specific function in vertebrate neurogenesis remains unclear.Citation7 However, recent studies suggest a role of Smad2 in the regulation of axonal morphogenesis in telencephalon and metencephalon,Citation22,Citation23 which correlates with the pro-survival effects that TGF-β has in neurons in other contexts.Citation24,Citation25 The role of Smad2 in neurogenesis appears to be related to SnoN, a modulator of axonal development and growth.Citation26 The SnoN pathway is receiving increased importance during embryogenesis, since recent findings suggest a high convergence with other important pathways, including Nodal/TGF-βCitation27 and cell cycle regulators such as p53 and APC/Cdh1.Citation28 Furthermore, in some cell lines, SnoN expression seems to be positively and negatively regulated by both R-Smads, revealing a potential implication of both R-Smads in this pathway.Citation29
Related to the expression profile, Smad2 is widely distributed in the neuroepithelium, and latterly in the telencephalon and metencephalon.Citation30 Nonetheless, in the context of the developing neural tube, Smad2 was not detected in previous studies.Citation20 We showed that Smad2 is expressed in the ventricular zone of the developing spinal cord in the chick neural tube, and that its expression partially overlaps with the expression pattern of Smad3, which is a requirement for a potential interplay between both R-Smads at the cellular level.Citation31
As reviewed above, Smad2 and Smad3 are functionally nonequivalent,Citation5,Citation32 despite their common upstream activation mechanism and their 92% identity in their amino acid sequences.Citation32-Citation34 Knockout (KO) studies in mice, show early embryonic lethality in Smad2 KO. This was attributed to disruption of the A/P axis within the epiblast and failure in the formation of the ectoderm, mesoderm, and endoderm.Citation34 In contrast, the KO of Smad3 is not embryonic lethal, although mice present deficiencies in the immune response and high predisposition to tumorgenesis.Citation35 In addition, Smad2 and Smad3 have been shown to recruit different co-factors and target different regulatory sequences.Citation36 In particular, Smad3 binds directly to a CAGA-box in the promoter of PAI-1 or c-Jun, whereas Smad2 binds indirectly through Smad4 and co-factors, such as FoxH1 and Mix families, to Activin response elements (ARE) or other regulatory sequences.Citation33,Citation37 Moreover, recent studies suggest that cell-type specific master transcription factors such as Oct4, Myod1, or PU.1 determine Smad2 and Smad3 targets, and hence direct TGF-β effects in a context-dependent manner.Citation38
Despite the important and multiple functions of the TGF-β signaling in many cellular processes and diseases,Citation39,Citation40 there are still relevant open questions regarding to the global mechanisms that govern TGF-β dynamics and function, with the 2 R-Smads playing redundant roles in some scenariosCitation9,Citation10 while antagonizing in others.Citation41,Citation42
To answer the question of how these 2 highly similar molecules targeting different DNA regulatory sequences can synergize or antagonize depending on the cellular context, we compared the function of both R-Smads in the context of vertebrate neurogenesis.Citation31 Our studies revealed a simultaneous cooperation and antagonism between Smad2 and Smad3 occurring at the same cellular context. Experiments performed in the chick neural tube by in ovo electroporation showed an increase of Smad3-sepecific transcription targets after Smad2-Smad3 gain of function (GOF) compared with wild-type (WT) or Smad3 GOF alone. Surprisingly, Smad2 loss of function (LOF) also induced a strong increase of Smad3 activity (). Additionally, Smad2-Smad3 GOF resulted in an increase of the amount of neural progenitors undergoing differentiation, specifically of ventral interneurons, compared with WT or GOF of Smad3.Citation20 Correspondingly, LOF of Smad2 also induced a strong increase in differentiation, compared with the WT situation.
In order to address the potential underlying mechanisms that could induce this dual interplay between the R-Smads, we followed a computational approach. Previous models of the TGF-β signaling pathway focused on the dynamics of the nucleocytoplasmic shuttling of R-Smad complexes,Citation43 the dynamics of signal processing, the generation of transient responses,Citation44 and endocytosis.Citation45 Most of these mathematical models assume that Smad2 and Smad3 are indistinguishable. These approaches take advantage of a detailed characterization of several potential interactions in the pathway to understand the effect of different regulatory mechanisms and crosstalks.Citation46
Contrary, our modeling approach focused on the R-Smads oligomerization after phosphorylation, in order to understand the dual antagonism/cooperation observed experimentally. This minimal model successfully reproduced all experimental results when we allowed the heterotrimer Smad2-Smad3-Smad4 to bind and regulate transcription in Smad3-specific regulatory sequences. We observed by co-inmunoprecipitation that Smad2 and Smad3 physically interact in a complex with Smad4, and that the amount of Smad3 bound to Smad4 depended on the amount of Smad2 available and vice versa. The affinity of phosphorylated Smad2 to form trimers with Smad2 vs. Smad3 is unknown. Nonetheless, the model predicted stronger affinity of the R-Smads to form the heterotrimer Smad2-Smad3-Smad4, than Smad2-Smad2-Smad4 or Smad3-Smad3-Smad4.
Overall, our results evidence a scenario of competition between R-Smad to form the 3 potential heterotrimers that depends on the concentration and the binding affinities of the R-Smads. Thus, the selection between cooperation and antagonism of the R-Smads is not cell type-dependent, occurring in the same cellular context as a consequence of the interaction between Smad2, Smad3, and Smad4.
Many aspects of the TGF-β pathway were not included in the analysis for the sake of simplicity. In our model, we assumed Michaelis–Menten kinetics for the phosphorylation dynamics of the R-Smads, and therefore the amount of phosphorylated R-Smads depended directly on the amount of available R-Smads after receptor activation. Moreover, we considered that protein production and degradation are balanced. Consequently, the concentration of each R-Smad was assumed constant. A more detailed analysis of our model, including a full mathematical description of the known interactions of the TGF-β pathway, remains to be studied. Potential candidates to include in the model are compartmentalization, nucleo-cytoplasmatic translocation of the R-Smads,Citation47 and inhibition by I-Smads (Smad6 and Smad7) and E3 ligases.Citation48 The I-Smads induce a well-characterized negative feedback loop that can induce nonlinear effects, such as desensitization of the pathway and robustness against perturbations.Citation49 Interestingly, some E3 ligases such as Arkadia have been demonstrated to positively contribute to TGF-β signaling. Arkadia has an essential role during embryogenesis because, under TGF-β activation, it degrades negative feedback inhibitors of the pathway, such as Smad7 and SnoN, a negative co-factor for Smad3/4 directed transcription.Citation44,Citation50 All these potential interactions will result in a more realistic mathematical approximation to the true dynamics of the pathway, but the increasing complexity and the amount of unknown reaction parameters will complicate the analysis and the understanding of the consequences of the interplay between the R-Smads.
The arising characteristics of the TGF-β system constitute a paradigm of how the network of interactions can determine the strength, dynamics and even the function of proteins in a given pathway. These emerging characteristics induced by a complex network have been studied widely in biological systems.Citation51 In the context of neurogenesis, Shh graded responses have been proposed to be interpreted via a nonlinear network of interacting genes.Citation8 In addition, domain specification of the neural tube has been also shown to be regulated by a network of interacting genes with multiple interconnected positive and negative feedback loops.Citation52 For instance, a feedback in Delta-Notch signaling that mediates lateral inhibition is key in the regulation of pro-neural genes and neural differentiation.Citation53 In fact, the characterization of the interactions within the network has been shown to be decisive for a full understanding of different developmental processes like somite formation,Citation54 palate,Citation55 and digit formation in miceCitation56 and pigmentation pattern in animal skinCitation57 and also in treatment and prognosis of pathologies such as cancer.Citation58-Citation60 These studies evidence that a complete understanding of the TGF-β and other signaling networks involved in neurogenesis should take into account not only the study of the biochemical aspects of the proteins, but also the analysis of the interaction network.
Disclosure of Potential Conflicts of Interest
No potential conflicts of interest were disclosed.
References
- WuMY, HillCS. Tgf-β superfamily signaling in embryonic development and homeostasis. Dev Cell2009; 16:329 - 43; http://dx.doi.org/10.1016/j.devcel.2009.02.012; PMID: 19289080
- OshimoriN, FuchsE. The harmonies played by TGF-β in stem cell biology. Cell Stem Cell2012; 11:751 - 64; http://dx.doi.org/10.1016/j.stem.2012.11.001; PMID: 23217421
- FengXH, DerynckR. Specificity and versatility in tgf-β signaling through Smads. Annu Rev Cell Dev Biol2005; 21:659 - 93; http://dx.doi.org/10.1146/annurev.cellbio.21.022404.142018; PMID: 16212511
- HeldinCHH, MiyazonoK, ten DijkeP. TGF-β signalling from cell membrane to nucleus through SMAD proteins. Nature1997; 390:465 - 71; http://dx.doi.org/10.1038/37284; PMID: 9393997
- MassaguéJ, SeoaneJ, WottonD. Smad transcription factors. Genes Dev2005; 19:2783 - 810; http://dx.doi.org/10.1101/gad.1350705; PMID: 16322555
- ten DijkeP, HillCS. New insights into TGF-β-Smad signalling. Trends Biochem Sci2004; 29:265 - 73; http://dx.doi.org/10.1016/j.tibs.2004.03.008; PMID: 15130563
- BrennanJ, LuCC, NorrisDP, RodriguezTA, BeddingtonRS, RobertsonEJ. Nodal signalling in the epiblast patterns the early mouse embryo. Nature2001; 411:965 - 9; http://dx.doi.org/10.1038/35082103; PMID: 11418863
- DessaudE, McMahonAP, BriscoeJ. Pattern formation in the vertebrate neural tube: a sonic hedgehog morphogen-regulated transcriptional network. Development2008; 135:2489 - 503; http://dx.doi.org/10.1242/dev.009324; PMID: 18621990
- JiaS, WuD, XingC, MengA. Smad2/3 activities are required for induction and patterning of the neuroectoderm in zebrafish. Dev Biol2009; 333:273 - 84; http://dx.doi.org/10.1016/j.ydbio.2009.06.037; PMID: 19580801
- JiaS, RenZ, LiX, ZhengY, MengA. smad2 and smad3 are required for mesendoderm induction by transforming growth factor-beta/nodal signals in zebrafish. J Biol Chem2008; 283:2418 - 26; http://dx.doi.org/10.1074/jbc.M707578200; PMID: 18025082
- LiuA, NiswanderLA. Bone morphogenetic protein signalling and vertebrate nervous system development. Nat Rev Neurosci2005; 6:945 - 54; http://dx.doi.org/10.1038/nrn1805; PMID: 16340955
- LiemKFJJr., TremmlG, JessellTM. A role for the roof plate and its resident TGFbeta-related proteins in neuronal patterning in the dorsal spinal cord. Cell1997; 91:127 - 38; http://dx.doi.org/10.1016/S0092-8674(01)80015-5; PMID: 9335341
- CooleyJR, YatskievychTA, AntinPB. Embryonic expression of the transforming growth factor β ligand and receptor genes in chicken. Dev Dyn2014; 243:497 - 508; http://dx.doi.org/10.1002/dvdy.24085; PMID: 24166734
- TimmerJ, ChesnuttC, NiswanderL. The activin signaling pathway promotes differentiation of dI3 interneurons in the spinal neural tube. Dev Biol2005; 285:1 - 10; http://dx.doi.org/10.1016/j.ydbio.2005.05.039; PMID: 16039645
- SeoaneJ, LeHV, ShenL, AndersonSA, MassaguéJ. Integration of Smad and forkhead pathways in the control of neuroepithelial and glioblastoma cell proliferation. Cell2004; 117:211 - 23; http://dx.doi.org/10.1016/S0092-8674(04)00298-3; PMID: 15084259
- FalkS, WurdakH, IttnerLM, IlleF, SumaraG, SchmidMT, DraganovaK, LangKS, ParatoreC, LeveenP, et al. Brain area-specific effect of TGF-β signaling on Wnt-dependent neural stem cell expansion. Cell Stem Cell2008; 2:472 - 83; http://dx.doi.org/10.1016/j.stem.2008.03.006; PMID: 18462697
- MisumiS, KimTSS, JungCG, MasudaT, UrakawaS, IsobeY, FuruyamaF, NishinoH, HidaH. Enhanced neurogenesis from neural progenitor cells with G1/S-phase cell cycle arrest is mediated by transforming growth factor β1. Eur J Neurosci2008; 28:1049 - 59; http://dx.doi.org/10.1111/j.1460-9568.2008.06420.x; PMID: 18783370
- GomesFC, SousaVdeO, RomãoL. Emerging roles for TGF-β1 in nervous system development. Int J Dev Neurosci2005; 23:413 - 24; http://dx.doi.org/10.1016/j.ijdevneu.2005.04.001; PMID: 15936920
- FarkasLM, DünkerN, RoussaE, UnsickerK, KrieglsteinK. Transforming growth factor-β(s) are essential for the development of midbrain dopaminergic neurons in vitro and in vivo. J Neurosci2003; 23:5178 - 86; PMID: 12832542
- García-CampmanyL, MartíE. The TGFbeta intracellular effector Smad3 regulates neuronal differentiation and cell fate specification in the developing spinal cord. Development2007; 134:65 - 75; http://dx.doi.org/10.1242/dev.02702; PMID: 17138664
- EstarásC, AkizuN, GarcíaA, BeltránS, de la CruzX, Martínez-BalbásMA. Genome-wide analysis reveals that Smad3 and JMJD3 HDM co-activate the neural developmental program. Development2012; 139:2681 - 91; http://dx.doi.org/10.1242/dev.078345; PMID: 22782721
- UeberhamU, LangeP, UeberhamE, BrücknerMK, Hartlage-RübsamenM, PannickeT, RohnS, CrossM, ArendtT. Smad2 isoforms are differentially expressed during mouse brain development and aging. Int J Dev Neurosci2009; 27:501 - 10; http://dx.doi.org/10.1016/j.ijdevneu.2009.04.001; PMID: 19375497
- WangL, NomuraM, GotoY, TanakaK, SakamotoR, AbeI, SakamotoS, ShibataA, EncisoPL, AdachiM, et al. Smad2 protein disruption in the central nervous system leads to aberrant cerebellar development and early postnatal ataxia in mice. J Biol Chem2011; 286:18766 - 74; http://dx.doi.org/10.1074/jbc.M111.223271; PMID: 21464123
- PinedaJR, DaynacM, ChicheporticheA, Cebrian-SillaA, Sii FeliceK, Garcia-VerdugoJM, BoussinFD, MouthonMA. Vascular-derived TGF-β increases in the stem cell niche and perturbs neurogenesis during aging and following irradiation in the adult mouse brain. EMBO Mol Med2013; 5:548 - 62; http://dx.doi.org/10.1002/emmm.201202197; PMID: 23526803
- BrionneTC, TesseurI, MasliahE, Wyss-CorayT. Loss of TGF-β 1 leads to increased neuronal cell death and microgliosis in mouse brain. Neuron2003; 40:1133 - 45; http://dx.doi.org/10.1016/S0896-6273(03)00766-9; PMID: 14687548
- StegmüllerJ, HuynhMA, YuanZ, KonishiY, BonniA. TGFbeta-Smad2 signaling regulates the Cdh1-APC/SnoN pathway of axonal morphogenesis. J Neurosci2008; 28:1961 - 9; http://dx.doi.org/10.1523/JNEUROSCI.3061-07.2008; PMID: 18287512
- ZhuQ, KimYH, WangD, OhSP, LuoK. SnoN facilitates ALK1-Smad1/5 signaling during embryonic angiogenesis. J Cell Biol2013; 202:937 - 50; http://dx.doi.org/10.1083/jcb.201208113; PMID: 24019535
- ZhuQ, LuoK. SnoN in regulation of embryonic development and tissue morphogenesis. FEBS Lett2012; 586:1971 - 6; http://dx.doi.org/10.1016/j.febslet.2012.03.005; PMID: 22710172
- ZhuQ, Pearson-WhiteS, LuoK. Requirement for the SnoN oncoprotein in transforming growth factor beta-induced oncogenic transformation of fibroblast cells. Mol Cell Biol2005; 25:10731 - 44; http://dx.doi.org/10.1128/MCB.25.24.10731-10744.2005; PMID: 16314499
- de Sousa LopesSM, CarvalhoRL, van den DriescheS, GoumansMJ, ten DijkeP, MummeryCL. Distribution of phosphorylated Smad2 identifies target tissues of TGF β ligands in mouse development. Gene Expr Patterns2003; 3:355 - 60; http://dx.doi.org/10.1016/S1567-133X(03)00029-2; PMID: 12799085
- MíguezDG, Gil-GuiñónE, PonsS, MartíE. Smad2 and Smad3 cooperate and antagonize simultaneously in vertebrate neurogenesis. J Cell Sci2013; 126:5335 - 43; http://dx.doi.org/10.1242/jcs.130435; PMID: 24105267
- BrownKA, PietenpolJA, MosesHL. A tale of two proteins: differential roles and regulation of Smad2 and Smad3 in TGF-beta signaling. J Cell Biochem2007; 101:9 - 33; http://dx.doi.org/10.1002/jcb.21255; PMID: 17340614
- DennlerS, HuetS, GauthierJM. A short amino-acid sequence in MH1 domain is responsible for functional differences between Smad2 and Smad3. Oncogene1999; 18:1643 - 8; http://dx.doi.org/10.1038/sj.onc.1202729; PMID: 10102636
- DunnNR, KoonceCH, AndersonDC, IslamA, BikoffEK, RobertsonEJ. Mice exclusively expressing the short isoform of Smad2 develop normally and are viable and fertile. Genes Dev2005; 19:152 - 63; http://dx.doi.org/10.1101/gad.1243205; PMID: 15630024
- AshcroftGS, YangX, GlickAB, WeinsteinM, LetterioJL, MizelDE, AnzanoM, Greenwell-WildT, WahlSM, DengC, et al. Mice lacking Smad3 show accelerated wound healing and an impaired local inflammatory response. Nat Cell Biol1999; 1:260 - 6; http://dx.doi.org/10.1038/12971; PMID: 10559937
- MorikawaM, KoinumaD, MiyazonoK, HeldinCH. Genome-wide mechanisms of Smad binding. Oncogene2013; 32:1609 - 15; http://dx.doi.org/10.1038/onc.2012.191; PMID: 22614010
- DerynckR, ZhangY, FengXH. Smads: transcriptional activators of TGF-β responses. Cell1998; 95:737 - 40; http://dx.doi.org/10.1016/S0092-8674(00)81696-7; PMID: 9865691
- MullenAC, OrlandoDA, NewmanJJ, LovénJ, KumarRM, BilodeauS, ReddyJ, GuentherMG, DeKoterRP, YoungRA. Master transcription factors determine cell-type-specific responses to TGF-β signaling. Cell2011; 147:565 - 76; http://dx.doi.org/10.1016/j.cell.2011.08.050; PMID: 22036565
- AkhurstRJ, HataA. Targeting the TGFβ signalling pathway in disease. Nat Rev Drug Discov2012; 11:790 - 811; http://dx.doi.org/10.1038/nrd3810; PMID: 23000686
- MassaguéJ. TGFbeta in Cancer. Cell2008; 134:215 - 30; http://dx.doi.org/10.1016/j.cell.2008.07.001; PMID: 18662538
- LabbéE, SilvestriC, HoodlessPA, WranaJL, AttisanoL. Smad2 and Smad3 positively and negatively regulate TGF β-dependent transcription through the forkhead DNA-binding protein FAST2. Mol Cell1998; 2:109 - 20; http://dx.doi.org/10.1016/S1097-2765(00)80119-7; PMID: 9702197
- YangYCC, PiekE, ZavadilJ, LiangD, XieD, HeyerJ, PavlidisP, KucherlapatiR, RobertsAB, BöttingerEP. Hierarchical model of gene regulation by transforming growth factor β. Proc Natl Acad Sci U S A2003; 100:10269 - 74; http://dx.doi.org/10.1073/pnas.1834070100; PMID: 12930890
- SchmiererB, TournierAL, BatesPA, HillCS. Mathematical modeling identifies Smad nucleocytoplasmic shuttling as a dynamic signal-interpreting system. Proc Natl Acad Sci U S A2008; 105:6608 - 13; http://dx.doi.org/10.1073/pnas.0710134105; PMID: 18443295
- NicklasD, SaizL. Characterization of negative feedback network motifs in the TGF-β signaling pathway. PLoS One2013; 8:e83531; http://dx.doi.org/10.1371/journal.pone.0083531; PMID: 24386222
- CellièreG, FengosG, HervéM, IberD. Plasticity of TGF-β signaling. BMC Syst Biol2011; 5:184; http://dx.doi.org/10.1186/1752-0509-5-184; PMID: 22051045
- ZiZ, ChapnickDA, LiuX. Dynamics of TGF-β/Smad signaling. FEBS Lett2012; 586:1921 - 8; http://dx.doi.org/10.1016/j.febslet.2012.03.063; PMID: 22710166
- InmanGJ, NicolásFJ, HillCS. Nucleocytoplasmic shuttling of Smads 2, 3, and 4 permits sensing of TGF-beta receptor activity. Mol Cell2002; 10:283 - 94; http://dx.doi.org/10.1016/S1097-2765(02)00585-3; PMID: 12191474
- Saritas-YildirimB, SilvaEM. The role of targeted protein degradation in early neural development. Genesis2014; 52:287 - 99; http://dx.doi.org/10.1002/dvg.22771; PMID: 24623518
- MíguezDG. Network nonlinearities in drug treatment. Interdiscip Sci2013; 5:85 - 94; http://dx.doi.org/10.1007/s12539-013-0165-x; PMID: 23740389
- LevyL, HowellM, DasD, HarkinS, EpiskopouV, HillCS. Arkadia activates Smad3/Smad4-dependent transcription by triggering signal-induced SnoN degradation. Mol Cell Biol2007; 27:6068 - 83; http://dx.doi.org/10.1128/MCB.00664-07; PMID: 17591695
- OltvaiZN, BarabásiAL. Systems biology. Life’s complexity pyramid. Science2002; 298:763 - 4; http://dx.doi.org/10.1126/science.1078563; PMID: 12399572
- KichevaA, CohenM, BriscoeJ. Developmental pattern formation: insights from physics and biology. Science2012; 338:210 - 2; http://dx.doi.org/10.1126/science.1225182; PMID: 23066071
- Formosa-JordanP, IbañesM, AresS, FradeJM. Regulation of neuronal differentiation at the neurogenic wavefront. Development2012; 139:2321 - 9; http://dx.doi.org/10.1242/dev.076406; PMID: 22669822
- KaernM, MíguezDG, MuñuzuriAP, MenzingerM. Control of chemical pattern formation by a clock-and-wavefront type mechanism. Biophys Chem2004; 110:231 - 8; http://dx.doi.org/10.1016/j.bpc.2004.02.006; PMID: 15228959
- EconomouAD, OhazamaA, PorntaveetusT, SharpePT, KondoS, BassonMA, Gritli-LindeA, CobourneMT, GreenJB. Periodic stripe formation by a Turing mechanism operating at growth zones in the mammalian palate. Nat Genet2012; 44:348 - 51; http://dx.doi.org/10.1038/ng.1090; PMID: 22344222
- ShethR, MarconL, BastidaMF, JuncoM, QuintanaL, DahnR, KmitaM, SharpeJ, RosMA. Hox genes regulate digit patterning by controlling the wavelength of a Turing-type mechanism. Science2012; 338:1476 - 80; http://dx.doi.org/10.1126/science.1226804; PMID: 23239739
- MíguezDG, MuñuzuriAP. On the orientation of stripes in fish skin patterning. Biophys Chem2006; 124:161 - 7; http://dx.doi.org/10.1016/j.bpc.2006.06.014; PMID: 16844282
- MíguezDG. The role of asymmetric binding in ligand-receptor systems with 1:2 interaction ratio. Biophys Chem2010; 148:74 - 81; http://dx.doi.org/10.1016/j.bpc.2010.02.012; PMID: 20332059
- Doldán-MartelliV, GuantesR, MíguezDG. A mathematical model for the rational design of chimeric ligands in selective drug therapies. CPT Pharmacometrics Syst Pharmacol2013; 2:e26; http://dx.doi.org/10.1038/psp.2013.2; PMID: 23887616
- Ruiz-HerreroT, EstradaJ, GuantesR, MiguezDG. A tunable coarse-grained model for ligand-receptor interaction. PLoS Comput Biol2013; 9:e1003274; http://dx.doi.org/10.1371/journal.pcbi.1003274; PMID: 24244115