Abstract
The present study describes the generation and the characterization of a stable cell line of neural stem cells derived from embryonic mouse hypothalamus. These cells (AC1) grow as an adherent culture in defined serum-free medium and express typical markers of neurogenic radial glia and of hypothalamic precursors. After prolonged expansion, AC1 cells may be efficiently induced to differentiate into neurons and astroglial cells in vitro and start to express some hormonal neuropeptides, like TRH, CRH, and POMC. Based on the capabilities of AC1 cells to be stably expanded and to develop neuroendocrine lineages in vitro, these cells might represent a novel tool to elucidate the mechanisms involved in the development of the hypothalamus and in the specific differentiation of neuroendocrine neurons.
In mouse, the majority of the neuroendocrine neurons become post-mitotic between embryonic day (E) 12 and 14. After E14, these neurons start to produce specific neurohormones and migrate from the germinal ventricular neuroepithelium to their final destinations, where they form discrete nuclei.Citation1 Previous mouse genetic studies have identified several transcription factors, with specific temporal and regional patterns of expression, that form a developmental “transcription factor code”Citation2 and that control the development of the hypothalamic neuroendocrine nuclei.Citation3
Although some of the genetic programs that control the formation of the hypothalamic nuclei are starting to be uncovered, the selection and lineage of neuroendocrine progenitors as well as the specification of their neuronal identities remain largely unknown. A significant improvement for the study of these aspects might stem from the establishment of in vitro hypothalamic precursor cell systems.
Recently it has been shown that the adult mouse hypothalamus contains resident neural stem cells (NSCs), located in neurogenic niches and identified as α-tanycytes, which derive from fetal radial glia.Citation4To date, most of the studies aimed at isolating and expanding hypothalamic NSCs in vitro have focused on postnatal or adult rat sources. Although some of these works reported the generation of neuroendocrine cells, which can secrete hypothalamic neurohormones,Citation5 none of them has been successful in obtaining pure and stable neurogenic cell lines. Further, other investigators have described the generation of hypothalamic-like NSCs and neurons from pluripotent embryonic stem cells (ESCs),Citation6,Citation7 but with very low efficiency. More recently, the isolation of neurospheres from E18 rat hypothalamus able to differentiate into neurons has been reported.Citation8
In the present study, we sought to address this by generating a stable cell line of fetal mouse hypothalamic NSCs (named AC1) that grows as monolayer, retains hallmarks of hypothalamic radial glia, and may efficiently differentiates into hypothalamic neurons with neuroendocrine features.
The AC1 cell line has been derived from E14.5 fetal hypothalamus using a procedure described by Conti et al.Citation9 and reported in the Supplementary Data. LC1 cells, generated from ES 46C CAG cells, were propagated as previously describedCitation9 and used as a control neural stem cell line for the comparison of the expression of stem cell and neuronal differentiating factors.
Once established, AC1 and LC1 cell lines were routinely grown in uncoated 25 cm2 flasks in Self-Renewal Medium (SRM) (see Supplementary Materials and Methods). The AC1 cells used for this study have been propagated for at least 25 passages (more than 100 duplications) and underwent to two cycles of cryostorage without significant modification of their morphology, doubling time or capacity of growth as a monolayer.
AC1 and LC1 cells were differentiated according to an optimized protocol previously described.Citation10 Briefly, the day after plating in SRM, the cells were shifted to differentiation conditions in D1 medium composed of EUROMED-N medium supplemented with 1% B27, 0.5% N2, and 10 ng/mL bFGF. After 3 d, the cultures were gently detached and the cells plated on laminin coated (3 µg/mL) plastic 4-well plates at a density of 6–7 × 104 cells/cm2 in D2 medium (1:3 mix of DMEM/F-12 and Neurobasal medium, 0.5% N2, 1% B27, 10 ng/mL bFGF, and 20 ng/mL of brain-derived growth factor [BDNF]). After 3 d of culture in D2 medium, the cells were exposed to D3 medium (1:3 mix of DMEM/F-12 and Neurobasal medium, 0.5% N2, 1% B27, 6.7 ng/mL bFGF, and 20 ng/mL BDNF). The cells were maintained under these conditions for an additional 7–15 d, and the D3 medium was partially changed every 2–3 d. Descriptions of the other procedures adopted (growth assay, immunofluorescence and RT-PCR) are reported in Supplementary Materials and Methods.
In order to isolate and establish a stable culture of hypothalamic embryonic radial glia cells, we dissected and dissociated the hypothalamic region of E14.5 mouse brain () to obtain a single cell suspension. The cells were then plated and maintained in defined SRM (see Supplementary Materials and Methods and ref. Citation9). Hypothalamic neural precursors initially aggregated and grew as floating structures (). Five days after plating, the aggregates were harvested, dissociated, and re-plated into fresh medium. At this stage, the cells attached within 2–3 d and grew as a population of adherent cells (). At this time, the cells were harvested and replated into fresh medium generating a population of adherent bipolar cells (). These cells, named AC1, have been then continuously and rapidly propagated with a doubling time of approximately 24 h (Fig. S1A). In order to define the identity of the AC1 cells, we analyzed the expression of immature and mature neural lineage cell markers by RT-PCR and immunofluorescence assays. RT-PCR analyses indicated that AC1 cells express Nestin, Sox2, and Pax6 (Fig. S1B), which are indicative of NSC identity and are highly expressed in mouse ES and fetal-derived adherent radial glia-like NSCs.Citation9 To corroborate these results and confirm the radial glia identity of stabilized AC1 cells we performed double immunofluorescence experiments. For comparison, we used LC1 cells as they represent an established model of adherent mouse radial glia-like NSCs (Fig. S2A).Citation9 Double immunofluorescence experiments for nestin/SOX2 () and PAX6/vimentin () showed an intense immunoreactivity for these markers in more than 98% of AC1 cells. Like the LC1 cell line, AC1 cells were also immunopositive for Olig2 (a maker of ventral neural tube) and RC2 (a phosphorylated form of nestin specifically expressed in radial glia cells)Citation11 (), while they were immunonegative for markers of neuronal (βIII-tubulin) and astroglial (glial fibrillary acidic protein, GFAP) lineages (). This indicates that self-renewal culture conditions preserved the multipotency of AC1 cells and suppressed their differentiation toward mature lineages.
Figure 1. (A) Images of the hypothalamic area dissected from wild-type E14.5 mouse brain to generate parental AC1 cells (panels 1–2). The cells were plated in medium supplemented with of bFGF and EGF (schematic in 3). (B–D) Phase-contrast images of AC1 cells taken 5 d after the initial plating in vitro (P0) (B), at passage 1 (P1) (C), and passage 2 (P2) (D). Scale bars: 10 mm (A), 100 μm (B-D).
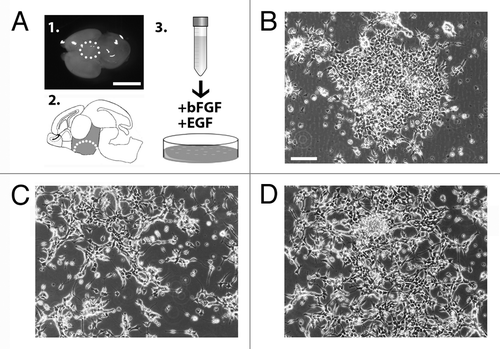
Figure 2. (A–D) Self renewing AC1 cells at (passage 22) immunostained for neural stem cell markers SOX2, nestin, PAX6, vimentin, Olig2, for the radial glia marker RC2 and for markers for neurons (βIII-tubulin) and astrocytes (GFAP). Examples of immunopositive and immunonegative cells are indicated with solid arrowheads and empty arrows, respectively. Nuclei were counterstained with DAPI (A’–D’). (E) RT-PCR analysis on self-renewing AC1 cells revealed the expression of markers for developing hypothalamic progenitors such as Brn2, Arnt2, and Mash1. RNA from E14.5 hypothalamus was chosen as positive control (Ctrl+). The picture is representative of three independent experiments with consistent results. (F) Self-renewing AC1 cells at (passage 22) immunostained for ARNT2 and MASH1. Examples of double immunopositive cells are indicated with a solid arrowhead. Nuclei were counterstained with DAPI (F’). Immunofluorescence images are representative of at least two independent experiments. Scale bar: 50 μm (A–D’, F, and F’).
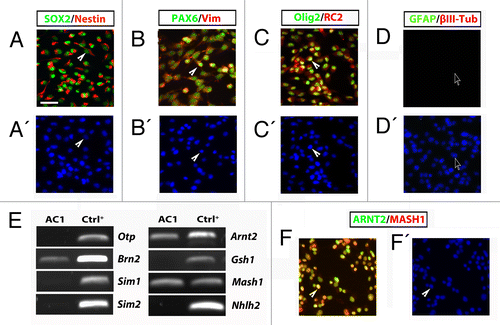
NSCs in the developing brain are characterized by a region-specific identity. In order to define the hypothalamic identity of AC1 cells, we performed RT-PCR screening using embryonic hypothalamic markers. We focused our attention on a specific set of transcription factors known to be involved in the development of different neuroendocrine cell lineages, such as Otp, Brn2, Sim1, Sim2, Arnt2, Gsh1, Mash1, and Nhlh2. Specifically, we found that cultures of AC1 cells expressed Arnt2 and Mash1; of note, the transcript for Brn2 was faintly detected only at early passages (up to passage 7) () but it was lost in most advanced cultures (passage 22) possibly due to an initial heterogeneity of AC1 cultures that became more homogeneous at later passages. Instead, Otp, Sim1, Sim2, Gsh1, and Nhlh2 transcripts were absent (). Immunofluorescence analysis confirmed the presence of a clear and homogeneous nuclear signal for both ARNT2 and MASH1 almost in all AC1 cells under self-renewing growth, with a high proportion of cells showing colocalization of the two transcription factors (). These data indicate that AC1 cells exhibit hallmarks of neural (Mash1) and hypothalamic progenitors (Arnt2 and Brn2).
To test the differentiative potential of AC1 cells, these cells were grown in culture conditions previously shown to promote the acquisition of neuronal and astroglial identities of NSCs.Citation10 Thus, AC1 and LC1 cells were cultured in differentiation media without EGF but with a gradual decrease of FGF-2 and a simultaneous increase of BDNF concentrations (see Materials and Methods).
After 3–5 d of culture in these conditions, morphological changes and the acquisition of a neuronal phenotype were observed. Phase contrast microscopy inspection revealed that the differentiating protocol induced the loss of typical self-renewing associated morphology () and the acquisition of a more neuronal-like phenotype. After 3 d of culture and onwards, AC1 cells exhibited thin and elongated processes, typical of mature neurons () that gradually increased in size and number with differentiation time (). Immunofluorescence analysis confirmed that in these conditions AC1 cells exhibit a strong decrease of nestin expression and instead started to express typical neuronal markers such as βIII-tubulin and microtubule-associated protein-2ab (MAP2ab) and, with less extent, the glial marker GFAP ().
Figure 3. (A–C) Phase-contrast images of stably AC1 cell cultures taken before, in self renewal conditions, (A) and after 3 (B), and 15 (C) days of in vitro differentiation (DIV). Solid arrowheads point at examples of neurites emerging from AC1 cell bodies. (D–F) Immunofluorescence images, representative of at least two independent experiments, of AC1 cells stained for neural precursors (βIII-tubulin, MAP2ab and nestin) and astroglial (GFAP) markers (D–F). Nuclei were counterstained with DAPI. Solid arrowheads point at examples of immunopositive neurites for the indicated markers. (G) RT-PCR on AC1 cells at different time points of differentiation (0, 3, and 15 DIV) revealed changes in the expression of markers for developing hypothalamic neurons with the appearance of transcripts for Otp, Brn2, Sim1, Sim2, and Gsh1. Scale bar: 50 μm (A–F).
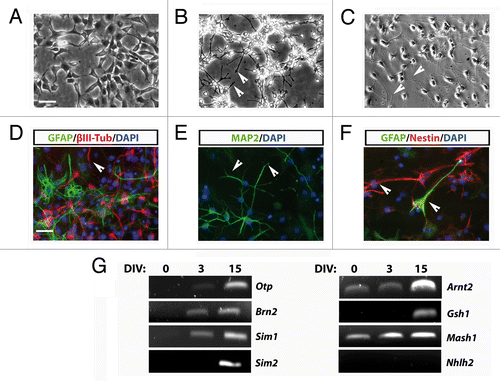
Measurement of neural cell frequency after the differentiation protocol showed that nestin immunoreactivity was restricted near to 18.4% of the cells and that 48.3% of the cells were immunopositive for βIII-tubulin, 52.5% for MAP2ab, and 17.4% for GFAP. These results are strictly comparable to those obtained in LC1 cells differentiated under the same experimental conditions (nestin+ve cells: 10.4%; βIII-Tubulin+ve cells: 53.5%; MAP2ab+ve cells: 44.7%; GFAP+ve cells: 4.3%; Fig. S2B)Citation9 and to most radial glia-like NSC lines, which show a neurogenic potential of approximately 50–85%.Citation10,Citation12 Immunofluorescence analysis also confirmed the expression of ARNT2 and MASH1 in almost all differentiated AC1 cells, with an high level of colocalization (Fig. S3A). Moreover, MASH1 signal appeared present mainly in MAP2ab-positive neurons; similarly, ARNT2 immunoreactivity was highly colocalized with βIII-tubulin positive cells. (Fig. S3B and C).
In order to verify the hypothalamic identity of AC1 cells after differentiation, we analyzed the expression of typical neuroendocrine hypothalamic markers. Candidate gene expression analysis by RT-PCR was performed on stabilized AC1 cells in self-renewal conditions and after 3 and 15 d of in vitro differentiation (DIV) (). We observed four different groups of expression patterns. First, we found an apparent gradual increase of Arnt2 and Mash1, two genes already present in self-renewal conditions, at 3DIV and 15DIV. Moreover, the differentiation of AC1 cells was accompanied by the appearance of transcripts for Otp, Brn2, and Sim1, whose expression appeared early (3DIV) after the shift to differentiating conditions and was maintained at the later time of observation. Of note, the Brn2 transcript, weakly present in early cultures, appeared to be lost in the most advanced cultures; however, the exposure of the cells to differentiating conditions restored Brn2 expression early at 3DIV. Sim2 and Gsh1 transcripts became detectable only after prolonged exposure to differentiating settings (15DIV), while we never detect the transcript for Nhlh2 either in self-renewing or differentiated AC1 cells.
Based on the results indicating that AC1 cells can generate neuroendocrine neurons, we then asked whether differentiated AC1 cells expressed hypothalamic peptides. To address this question, we studied the expression of different peptides and of tyrosine hydroxylase, a marker of dopaminergic neurons, by RT-PCR under self-renewing and differentiating conditions. As shown in , these factors were all expressed in adult hypothalamic RNA extracts, used as control (Ctrl+); no transcripts were evident in samples not incubated with reverse transcriptase (Ctrl−). In RNA extracted by AC1 cells, we did not find any transcript for the factors considered in cells maintained in self-renewing culture conditions (DIV0). After 3DIV, AC1 cells started to express proopiomelanocortin (Pomc); a faint signal of transcript for Trh was also present in AC1 cells at this time of observation. At a later time point of neural differentiation (15DIV) transcripts for Pomc, Trh, and Crh were clearly detected. These results confirmed the capacity of differentiating AC1 cells to assume specific hypothalamic neuropeptidergic phenotypes.
Figure 4. (A) RT-PCR analysis for the expression of hypothalamic peptides and tyrosine hydroxylase (Th), as markers of mature hypothalamic neurons, in AC1 cells at different time points of differentiation (0, 3, and 15 DIV) revealed the presence of Pomc transcript at 3 and 15 DIV and the expression of Crh and Trh at 15 DIV. The picture is representative of three independent experiments with consistent results. Ctrl+, positive control (adult mouse hypothalamus); Ctrl−, PCR negative control (RNA samples not retrotranscribed). Avp, arginine-vasopressin; Npy, neuropeptide Y, Ot, oxytocin; Pomc, proopiomelanocortin; Ss, somatostatin; Th, tyrosine hydroxylase; Gapdh, Glyceraldehyde 3-phosphate dehydrogenase. (B) Schematic representation of the transcription factors suggested to be required for the development of hypothalamic nuclei (hypothalamic transcription factor code) and the differentiation of neuroendocrine neurons in mouse. In gray those not expressed, and as well as the pathways not activated, in AC1 cells under the neuronal differentiation protocol. PVN, paraventricular nucleus; aPV anterior periventricular nucleus,; SON, supraoptic nucleus; ARC, arcuate nucleus; VMN, ventromedial nucleus; DA, dopaminergic neurons of the A11 group.
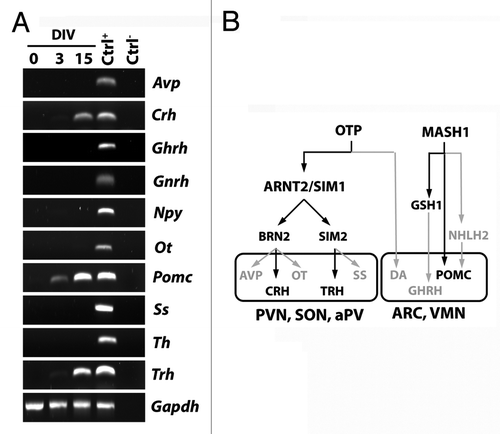
In contrast to our rapidly increasing knowledge of hypothalamic neuronal varieties and their functions, the developmental processes that govern the specification of the different neuroendocrine subtypes remain poorly understood. With this view, the availability of in vitro stable self-renewing hypothalamic NSCs might offer substantial advantages.
Here we report the establishment of a novel in vitro experimental model of hypothalamic NSCs, named AC1 cells, obtained from the developing mouse hypothalamus at E14.5.Citation1 These cells can be propagated in vitro, maintain high neurogenic potential and can generate neuronal cells expressing some hypothalamic peptides. AC1 cells have a radial glia identity and maintain the positional code of their hypothalamic origin. Because of the direct derivation of adult hypothalamic NSCs (identified as α-tanycytes) from local fetal radial glia, AC1 cells could represent a valuable tool to better understand the molecular mechanisms behind the developmental transition from radial glia to α-tanycytes.Citation4
Moreover, AC1 cells differ from the other in vitro isolated hypothalamic precursors models described so far and can be considered complementary to these systems, in respect to purity, stability and neuron differentiation efficiency.Citation10,Citation12,Citation13 For instance, AC1 cells can be propagated in vitro for more than 100 doubling times without loss of their composition and radial glia identity, as confirmed by the expression of specific molecular markers (i.e., nestin, SOX2, RC2, vimentin).Citation9,Citation14 In addition, AC1 cells grow as an adherent monolayer, a condition that allows easy cell manipulation and that is pivotal to perform phenotypic assays.Citation15,Citation16
In fact, although the isolation of hypothalamic neural precursors with neuroendocrine characteristics has been previously reported, none of these studies has successfully generated a stable radial glia-like hypothalamic NSCs at high efficiency.Citation5,Citation7,Citation8,Citation17 Markakis and co-authors first described the isolation of progenitor cells from 7-wk-old rat hypothalamus; these cells grew as monolayer and generated, following retinoic acid and forskolin treatment, only a small percentage (between 0.5 and 5%) of immature neurons.Citation5 Since they were not expanded in vitro for more than 5–7 passages and were not subcloned, to our mind these cultures are reminiscent of primary cultures rather than stable NSC lines. Furthermore, these cells expressed neuroendocrine peptides both in differentiated and undifferentiated cultures, possibly reflecting an identity of hypothalamic committed precursor identity rather than that of hypothalamic NSCs.
More recently, Salvi and co-authors isolated NSCs from rat embryonic (E18) and adult hypothalamusCitation8 forming contrast to AC1 cells, these cells grew in suspension as neurospheres, a mixed culture system that is poorly enriched in NSCs and that makes the interpretation of the expression and molecular profiles difficult.Citation18 In addition, these cells showed limited expandability (maximum of 6 passages) and scarce neuronal differentiation efficiency (about 5% of βIII-tubulin immunoreactive cells); furthermore, once differentiated they were restricted to the GnRH neuronal subtype possibly indicating that these cells represent a GnRH-committed population. Noteworthy, precursors of GnRH neurons originate outside the hypothalamus and migrate into this region from E11.
Other studies have described the development of neuroendocrine cells in vitro from mouse ESCs (mESCs). For instance, it has been shown that mESCs can differentiate into hypothalamic dopaminergic neurons following treatment with the signaling molecules SHH and BMP7.Citation6 More recently, Sasai’s lab reported that neuroectodermal cells derived from ESCs might differentiate into rostral hypothalamic-like progenitors and into hypothalamic neuroendocrine neurons expressing OTP, BRN2, and vasopressin.Citation7 However, while mESCs represent a good system to study developmental processes, these cells may give rise to very heterogeneous lineages; in contrast AC1 cells, derived from a restricted (hypothalamic) population of NSCs, would have the advantage of being a system more suitable to molecular screenings.
Finally, the hypothalamic origin of AC1 cells is supported by our findings. Consistent with the most recent hypothesis of gene specification in the generation of hypothalamic neuronal diversity,Citation19,Citation20 AC1 cells mainly express Arnt2 and Mash1 and maintain this expression throughout the propagation in culture. In particular, Arnt2 is expressed early in hypothalamic neuroepitheliumCitation21 and plays a role in the differentiation of mature neurosecretory neurons of the hypothalamic paraventricular, supraoptic, and anterior periventricular nuclei,Citation22-Citation24 whereas the proneural gene Mash1 is required for the generation of ventral neuroendocrine progenitors of ventromedial and arcuate nuclei.Citation25 These results are therefore suggestive of the presence in AC1 cells of hypothalamic stem cells committed to develop the two main class of neuroendocrine nuclei. The lack of Otp, the main gene indicative of hypothalamic development, in AC1 cellsCitation19 is instead justified by the observation that proliferating progenitors of the hypothalamic ventricular zone do not express Otp.Citation26
Further, the finding of a broader pattern of expression of the “hypothalamic transcription factor code” in AC1 cells, including Otp and the major downstream genes (Sim1, Arnt2, Brn2, Gsh1, and Sim2), after neural differentiation, demonstrated that the AC1 cell line is indeed composed of hypothalamic neural progenitors.
Consistent with the activation of specific hypothalamic genetic pathways,Citation19,Citation20 differentiated AC1 cells also express genes coding for some hypothalamic peptides, such as Trh, Crh, and Pomc. The expression of Crh is consistent with the activation of the Otp-Arnt2/Sim1-Brn2 differentiative pathway. The expression of Trh may result from the activation Otp-Arnt2/Sim1-Sim2 pathway (). Accordingly, it has been reported that BDNF, a growth factor present in the differentiation protocol adopted in this study, may induce the differentiation of TRH-producing neurons as well as the expression of Trh gene.Citation27,Citation28 The presence of Pomc transcript, usually expressed by ARC neurons, in differentiated AC1 cells fits with the expression of MASH1,Citation20,Citation25 which is required for normal development of POMC-expressing neurons, although it does not directly affect POMC expression.Citation25
The absence of transcripts for other neuroendocrine peptides (like Avp, Ot, Ss, and Ghrh) and TH, despite the activation of the main hypothalamic transcription factors, seems to suggest either the lack in differentiated AC1 cells of AVP and OT-producing magnocellular neurons or of the activation of still unidentified factors or pathways involved in specific differentiation that will be the subject of future investigations.
In conclusion, in the present study we provide evidence that AC1 cells represent a stable and accessible source of mouse hypothalamic NSCs with a specific radial glia identity. AC1 cells would represent a useful tool to investigate in vitro the mechanisms possibly involved in development, differentiation, and fate of hypothalamic neurons with neuroendocrine features. Deciphering the genetic patterns governing the development of the neuroendocrine system will also help to better understand the molecular pathogenesis of hypothalamic dysfunctions and to establish novel diagnostic/therapeutic tools.
Additional material
Download Zip (274.6 KB)Disclosure of Potential Conflicts of Interest
No potential conflicts of interest were disclosed.
Acknowledgments
This work was supported by Italian “Ministero della Salute” (GR-2008-1137632 to A.C. and GR-2008-1146615 to L.C.) and MIUR (PRIN 2005: 2005051740_001 to L.C. and PRIN2003 2003060512_001 and FIRST2007 to R.M.).
The authors thank Daria Messelodi, Dr Roberta Noseda, and Dr Elio Messi for their technical support, and Dr Francesca Mackenzie for reading the manuscript.
The authors declare that they have no significant competing financial interests that might have influenced the work described in this manuscript.
References
- MarkakisEA, SwansonLW. Spatiotemporal patterns of secretomotor neuron generation in the parvicellular neuroendocrine system. Brain Res Brain Res Rev1997; 24:255 - 91; http://dx.doi.org/10.1016/S0165-0173(97)00006-4; PMID: 9385456
- GuillemotF. Spatial and temporal specification of neural fates by transcription factor codes. Development2007; 134:3771 - 80; http://dx.doi.org/10.1242/dev.006379; PMID: 17898002
- SzarekE, CheahPS, SchwartzJ, ThomasP. Molecular genetics of the developing neuroendocrine hypothalamus. Mol Cell Endocrinol2010; 323:115 - 23; http://dx.doi.org/10.1016/j.mce.2010.04.002; PMID: 20385202
- LeeDA, BedontJL, PakT, WangH, SongJ, Miranda-AnguloA, TakiarV, CharubhumiV, BalordiF, TakebayashiH, et al. Tanycytes of the hypothalamic median eminence form a diet-responsive neurogenic niche. Nat Neurosci2012; 15:700 - 2; http://dx.doi.org/10.1038/nn.3079; PMID: 22446882
- MarkakisEA, PalmerTD, Randolph-MooreL, RakicP, GageFH. Novel neuronal phenotypes from neural progenitor cells. J Neurosci2004; 24:2886 - 97; http://dx.doi.org/10.1523/JNEUROSCI.4161-03.2004; PMID: 15044527
- OhyamaK, EllisP, KimuraS, PlaczekM. Directed differentiation of neural cells to hypothalamic dopaminergic neurons. Development2005; 132:5185 - 97; http://dx.doi.org/10.1242/dev.02094; PMID: 16284116
- WatayaT, AndoS, MugurumaK, IkedaH, WatanabeK, EirakuM, KawadaM, TakahashiJ, HashimotoN, SasaiY. Minimization of exogenous signals in ES cell culture induces rostral hypothalamic differentiation. Proc Natl Acad Sci U S A2008; 105:11796 - 801; http://dx.doi.org/10.1073/pnas.0803078105; PMID: 18697938
- SalviR, ArsenijevicY, GiacominiM, ReyJP, VoirolMJ, GaillardRC, RisoldPY, PralongF. The fetal hypothalamus has the potential to generate cells with a gonadotropin releasing hormone (GnRH) phenotype. PLoS One2009; 4:e4392; http://dx.doi.org/10.1371/journal.pone.0004392; PMID: 19197372
- ContiL, PollardSM, GorbaT, ReitanoE, ToselliM, BiellaG, SunY, SanzoneS, YingQL, CattaneoE, et al. Niche-independent symmetrical self-renewal of a mammalian tissue stem cell. PLoS Biol2005; 3:e283; http://dx.doi.org/10.1371/journal.pbio.0030283; PMID: 16086633
- SpiliotopoulosD, GoffredoD, ContiL, Di FeboF, BiellaG, ToselliM, CattaneoE. An optimized experimental strategy for efficient conversion of embryonic stem (ES)-derived mouse neural stem (NS) cells into a nearly homogeneous mature neuronal population. Neurobiol Dis2009; 34:320 - 31; http://dx.doi.org/10.1016/j.nbd.2009.02.007; PMID: 19236914
- MissonJP, EdwardsMA, YamamotoM, CavinessVSJr.. Identification of radial glial cells within the developing murine central nervous system: studies based upon a new immunohistochemical marker. Brain Res Dev Brain Res1988; 44:95 - 108; http://dx.doi.org/10.1016/0165-3806(88)90121-6; PMID: 3069243
- OnoratiM, BinettiM, ContiL, CamnasioS, CalabreseG, AlbieriI, Di FeboF, ToselliM, BiellaG, MartynogaB, et al. Preservation of positional identity in fetus-derived neural stem (NS) cells from different mouse central nervous system compartments. Cell Mol Life Sci2011; 68:1769 - 83; http://dx.doi.org/10.1007/s00018-010-0548-7; PMID: 20981563
- GoffredoD, ContiL, Di FeboF, BiellaG, TosoniA, VagoG, BiunnoI, MoianaA, BologniniD, ToselliM, et al. Setting the conditions for efficient, robust and reproducible generation of functionally active neurons from adult subventricular zone-derived neural stem cells. Cell Death Differ2008; 15:1847 - 56; http://dx.doi.org/10.1038/cdd.2008.118; PMID: 19011641
- PollardSM, ContiL. Investigating radial glia in vitro. Prog Neurobiol2007; 83:53 - 67; http://dx.doi.org/10.1016/j.pneurobio.2007.02.008; PMID: 17449166
- PollardS, ContiL, SmithA. Exploitation of adherent neural stem cells in basic and applied neurobiology. Regen Med2006; 1:111 - 8; http://dx.doi.org/10.2217/17460751.1.1.111; PMID: 17465825
- GorbaT, ContiL. Neural stem cells as tools for drug discovery: novel platforms and approaches. Expert Opin Drug Discov2013; 8:1083 - 94; http://dx.doi.org/10.1517/17460441.2013.805199; PMID: 23725548
- El MajdoubiM. Stem cell-derived in vitro models for investigating the effects of endocrine disruptors on developing neurons and neuroendocrine cells. J Toxicol Environ Health B Crit Rev2011; 14:292 - 9; http://dx.doi.org/10.1080/10937404.2011.578553; PMID: 21790313
- ContiL, CattaneoE. Neural stem cell systems: physiological players or in vitro entities?. Nat Rev Neurosci2010; 11:176 - 87; http://dx.doi.org/10.1038/nrn2938; PMID: 20107441
- Del GiaccoL, PistocchiA, CotelliF, FortunatoAE, SordinoP. A peek inside the neurosecretory brain through Orthopedia lenses. Dev Dyn2008; 237:2295 - 303; http://dx.doi.org/10.1002/dvdy.21668; PMID: 18729222
- JoYH, ChuaSJr.. Transcription factors in the development of medial hypothalamic structures. Am J Physiol Endocrinol Metab2009; 297:E563 - 7; http://dx.doi.org/10.1152/ajpendo.00064.2009; PMID: 19383874
- AitolaMH, Pelto-HuikkoMT. Expression of Arnt and Arnt2 mRNA in developing murine tissues. J Histochem Cytochem2003; 51:41 - 54; http://dx.doi.org/10.1177/002215540305100106; PMID: 12502753
- SchonemannMD, RyanAK, McEvillyRJ, O’ConnellSM, AriasCA, KallaKA, LiP, SawchenkoPE, RosenfeldMG. Development and survival of the endocrine hypothalamus and posterior pituitary gland requires the neuronal POU domain factor Brn-2. Genes Dev1995; 9:3122 - 35; http://dx.doi.org/10.1101/gad.9.24.3122; PMID: 8543156
- MichaudJL, RosenquistT, MayNR, FanCM. Development of neuroendocrine lineages requires the bHLH-PAS transcription factor SIM1. Genes Dev1998; 12:3264 - 75; http://dx.doi.org/10.1101/gad.12.20.3264; PMID: 9784500
- HosoyaT, OdaY, TakahashiS, MoritaM, KawauchiS, EmaM, YamamotoM, Fujii-KuriyamaY. Defective development of secretory neurones in the hypothalamus of Arnt2-knockout mice. Genes Cells2001; 6:361 - 74; http://dx.doi.org/10.1046/j.1365-2443.2001.00421.x; PMID: 11318878
- McNayDE, PellingM, ClaxtonS, GuillemotF, AngSL. Mash1 is required for generic and subtype differentiation of hypothalamic neuroendocrine cells. Mol Endocrinol2006; 20:1623 - 32; http://dx.doi.org/10.1210/me.2005-0518; PMID: 16469766
- AcamporaD, PostiglioneMP, AvantaggiatoV, Di BonitoM, VaccarinoFM, MichaudJ, SimeoneA. Progressive impairment of developing neuroendocrine cell lineages in the hypothalamus of mice lacking the Orthopedia gene. Genes Dev1999; 13:2787 - 800; http://dx.doi.org/10.1101/gad.13.21.2787; PMID: 10557207
- HuangEJ, ReichardtLF. Neurotrophins: roles in neuronal development and function. Annu Rev Neurosci2001; 24:677 - 736; http://dx.doi.org/10.1146/annurev.neuro.24.1.677; PMID: 11520916
- UbietaR, UribeRM, GonzálezJA, García-VázquezA, Pérez-MonterC, Pérez-MartínezL, Joseph-BravoP, CharliJL. BDNF up-regulates pre-pro-TRH mRNA expression in the fetal/neonatal paraventricular nucleus of the hypothalamus. Properties of the transduction pathway. Brain Res2007; 1174:28 - 38; http://dx.doi.org/10.1016/j.brainres.2007.08.026; PMID: 17854778