Abstract
The human helicase and ATPase up-frameshift suppressor 1 (UPF1), traditionally known as a major player in several RNA quality control mechanisms, is emerging as a crucial caretaker of the stability of the genome. Work from my laboratory has provided insight into the function of UPF1 during DNA metabolism and has revealed that this versatile enzyme sustains the proper replication of telomeres, the protective structures located at the ends of linear eukaryotic chromosomes. We have supplied direct evidence that telomere replication is not completed in cells with compromised UPF1 function, leading to the accumulation of DNA damage and telomere abnormalities. We also have isolated a number of factors that physically interact with UPF1 and might represent molecular links between UPF1 and telomeres. In this paper, I re-evaluate the functions of UPF1 in maintaining the stability of telomeres and of the genome at large and suggest a model that explains how UPF1 might be recruited and function during telomere replication.
The functions of UPF1 (also known as suppressor with morphogenetic defects in genitalia 2, SMG2, and regulator of nonsense transcripts 1, RENT1) have been analyzed largely in the context of mRNA (mRNA) quality control mechanisms, mostly taking place in the cytoplasm.Citation1-Citation3 Historically, UPF1 has been singled out as a key player in nonsense-mediated mRNA decay (NMD).Citation4-Citation6 NMD is a sophisticated, evolutionarily conserved cellular pathway able to recognize aberrant mRNA molecules carrying premature stop codons (PTCs) and to trigger their degradation, thereby preventing the translation of potentially harmful C-terminally truncated proteins.Citation1-Citation3 In human cells, NMD also regulates the expression levels of physiological mRNAs and mutes the transcriptional noise associated with the genome by promoting the decay of transcripts from ancient transposons and endogenous retroviruses.Citation7 Alternative RNA surveillance pathways requiring UPF1 activity include Staufen 1 (Stau1)-mediated mRNA decay and replication-dependent histone mRNA degradation; in these pathways, mRNA decay is mediated by the physical interaction of UPF1 with the RNA binding proteins Stau1 and stem-loop binding protein (SLBP), respectively.Citation8-Citation11
As already mentioned, NMD execution occurs mostly in the cytoplasm.Citation12 Consistently, indirect immunofluorescence (IF) experiments performed with antibodies raised against human UPF1 have revealed that the majority of UPF1 molecules are dispersed diffusely through the cytoplasm of human cancer cells.Citation13,Citation14 Nevertheless, a faint but reproducible staining of the nucleus is also observed (for an example, see ). The use of leptomycin B to block chromosomal region maintenance 1 (CRM1)-mediated nuclear export leads to the rapid nuclear accumulation of endogenous as well as green fluorescence protein (GFP)-tagged UPF1.Citation14 Moreover, IF staining of cells treated with mild detergents to extract soluble proteins clearly reveals that a substantial fraction of insoluble UPF1 produces punctuate staining in the nucleus (). Collectively, these experiments indicate that UPF1 shuttles between the nucleus and the cytoplasm and suggest that a fraction of nuclear UPF1 stably associates with chromatin. Interestingly, yeast Upf1p is thought to be localized exclusively in the cytoplasm,Citation15 indicating that putative nuclear functions associated with UPF1 emerged late during evolution and might represent a unique feature of mammalian UPF1 proteins.
Figure 1. Confocal images of endogenous UPF1 distribution in human HeLa cancer cells. Indirect immunofluorescence staining was performed using goat polyclonal antibodies raised against a C-terminal peptide of UPF1 on fixed cells either untreated, to detect total UPF1 (upper panels), or pre-treated with mild detergents, to detect insoluble UPF1 (lower panels). UPF1 antibodies were revealed using secondary antibodies conjugated with Alexa 488 fluorochrome (shown in green), whereas DNA was stained using DAPI (in blue). Note the focal staining of insoluble UPF1 in the nucleus. Scale bars correspond to 10 μm.
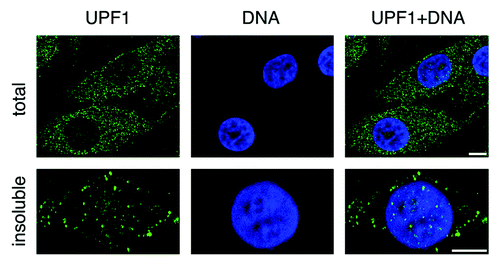
Using protein gel blot analysis of chromatin fractions prepared from unperturbed, cell-cycle synchronized and gamma-irradiated human cultured cells, we were the first to determine that UPF1 binds to chromatin.Citation16,Citation17 We showed that UPF1 is indeed a chromatin component and that, strikingly, UPF1 association with chromatin is enhanced during the progression of the S-phase, when chromosomal DNA is replicated, and upon DNA damage induced by gamma rays.Citation16 Subsequently, using chromatin immunoprecipitation (ChIP) experiments performed with antibodies against UPF1, we further demonstrated that chromatin-bound UPF1 is enriched at telomeres, the heterochromatic nucleoprotein structures located at the ends of linear eukaryotic chromosomes.Citation18-Citation20 More recently, work from the Mühlemann laboratory has revealed that UPF1 also associates with actively transcribing, PTC+ and PTC- reporter genes that have been stably integrated into the genome of human cancer cells.Citation21 The association of UPF1 with transcribed PTC-containing genes is thought to prevent the splicing and export of PTC+ pre-mRNAs.Citation21 On the other side, the in vivo association of UPF1 with telomeres constituted the primary concrete and direct evidence that UPF1 is involved in regulating telomeric DNA metabolism.
The first indication that UPF1 helps to maintain genome stability came from experiments employing short hairpin RNA (shRNA) plasmids to deplete UPF1 in human cancer cells. UPFI depletion leads to the accumulation of nuclear DNA repair foci, which contain the DNA damage marker γH2AX and the single-stranded DNA binding protein replication protein A (RPA).Citation16,Citation17,Citation22 Importantly, the depletion of UPF2, another NMD effector, does not trigger a DNA damage response although it impairs NMD execution to levels similar to that of UPF1.Citation16 This suggests that inefficient canonical NMD does not compromise genome integrity and reveals alternative roles for UPF1 in preserving genome integrity that do not depend on NMD. The DNA damage response evoked by UPF1 depletion was followed by cell-cycle arrest at the onset of the S-phase, accompanied by the accumulation of proliferating-cell nuclear antigen (PCNA) molecules in the cell nuclei, in a distribution pattern that is typical of the early S-phase.Citation16 In addition, the γH2AX accumulation induced by UPF1 depletion was prevented when cells were concomitantly depleted of the ataxia-telangiectasia and Rad3-related (ATR) protein, a phosphoinositide-3-kinase-related protein kinase (PIKK) that is a major player in signaling and stabilizing arrested replication forks.Citation16,Citation23,Citation24 In successive studies, we have shown that UPF1-depleted cells accumulated telomeric aberrations, including telomere-free chromosome ends and fragile, shredded telomeres, preferentially at chromatids replicated by the leading-strand semi-conservative replication machinery.Citation18,Citation25 Based on this experimental evidence, we were the first to propose that UPF1 is required for the complete replication of telomeric DNA (see model in ) and that in cells depleted for UPF1, replication fork progression through telomeric DNA was halted, generating DNA damage and single-stranded DNA; this eventually degenerates into DNA double strand breaks (DSBs), leading to the loss of entire telomeric tracts. Further corroborating this hypothesis, a part of the nuclear foci containing γH2AX and RPA that arises upon depletion of UPF1 co-localizes with telomeric heterochromatin.Citation25
Figure 2. Speculative model depicting UPF1 association with a replicating telomere. Repetitive telomeric DNA, in blue, is composed of double stranded 5′-TTAGGG-3′/5′-CCCTAA-3′ repeats and it unwinds into single-stranded telomeric DNA within the replication fork, shown as a blue shaded ellipse. UPF1 physically interacts with the lagging-strand polymerase polδ and, possibly, with the leading-strand polymerase polε. Both polymerases are in gray and the arrows indicate the direction of replication on the two strands. The ATPase activity of UPF1 is indicated by the reaction ATP → ADP + inorganic phosphate (Pi). ATR (in yellow) phosphorylates UPF1, presumably within its C-terminal tail. Human EST1A (in green) directly interacts with UPF1. Black arrows indicate interactions between UPF1, hEST1A, TPP1 (in yellow within the shelterin complex), and telomerase (in white). The black inhibitory bar indicates the putative function associated with UPF1 in displacing TERRA from the replicating telomere.
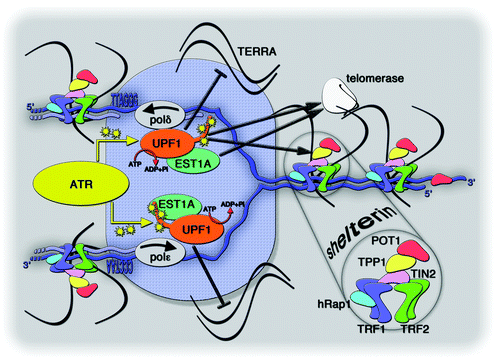
Our studies have set the stage for the detailed characterization of the role of UPF1 in maintaining the stability of telomeres. Still, a number of crucial questions remain to be addressed experimentally to fully understand the details of the telomeric functions linked to UPF1. It is unclear, for example, exactly how UPF1 promotes telomere leading-strand replication. Using shRNA complementation assays, we showed that an ATPase-dead mutant allele of UPF1 is unable to restore normal telomere replication.Citation25 Because the ATPase activity of UPF1 sustains its helicase activity,Citation26,Citation27 it seems safe to conclude that the function of UPF1 during telomere replication involves the unwinding of telomeric nucleic acid molecules. This notion is indeed consistent with the reported accumulation of UPF1 on S-phase chromatin and with the presence of UPF1 at telomeres during the S-phase.Citation16,Citation25 It is now necessary to understand what telomeric substrates are being unwound by UPF1. The most straightforward possibility is that UPF1 may be unwinding telomeric DNA in front of the replication fork, and is thus performing as a canonical replicative helicase. This hypothesis is supported by the fact that UPF1 physically interacts with independent subunits of the replicative DNA polymerase delta (polδ; ).Citation16,Citation28 In apparent contrast with this hypothesis is the fact that the telomeric dysfunctions observed in UPF1-depleted cells mostly derive from defects in leading-strand semi-conservative replication,Citation25 whereas polδ is known to act within the lagging-strand replication machinery. Nevertheless, it remains to be determined if UPF1 also associates to the leading-strand replication machinery, perhaps through interaction with DNA polymerase epsilon (polε; ). Indeed, UPF1 might be required for both leading- and lagging-strand replication of telomeric DNA, as suggested by the slight but consistent accumulation of fragility also at lagging-strand telomeres.Citation25 The more prevalent accumulation of leading-strand fragile telomeres could indicate that UPF1 action is more important for leading-strand replication or that defects in telomere lagging-strand replication occur with equal frequency but are more efficiently buffered and repaired, perhaps by telomerase, a specialized reverse transcriptase that is able to synthesize telomeric repeats de novo and to add them to the 3′ end of telomeres.Citation29,Citation30 It will be interesting to re-analyze the strand specificity of the telomeric defects that arise upon UPF1 depletion in cells in which telomerase activity has been inhibited.
Given the well-established connection between UPF1 and RNA metabolism, UPF1 might sustain telomere replication by acting on telomeric repeat-containing RNA (TERRA), a recently discovered type of non-coding RNA.Citation18,Citation31-Citation33 TERRA molecules are transcribed in a centromere-to-telomere direction by DNA-dependent RNA polymerase II (RNAPII), which uses the C-rich telomeric strand as a template. TERRA molecules, therefore, contain G-rich telomeric RNA repeats.Citation18,Citation31-Citation33 A large fraction of human TERRA is transcribed from CpG dinucleotide-rich promoters located in subtelomeric regions 250–1,000 bp away from the first telomeric repeat, and remains post-transcriptionally associated with telomeres through mechanisms that could involve telomeric repeat binding factors 1 and 2 (TRF1 and TRF2, respectively), which are components of the multiprotein telomeric complex shelterin (), and telomerase.Citation18-Citation20,Citation33-Citation37 Indeed, TERRA was shown to interact with these three factors in cell extracts and in vitro.Citation34,Citation37 The constant presence of an RNA moiety consisting of telomeric RNA repeats could halt the progression of the replication fork through the telomere by associating with the single-stranded telomeric DNA that is transiently generated during replication, either by directly base pairing with the C-rich telomeric strand or by forming G-quadruplex intermediates with the G-rich telomeric strand.Citation38,Citation39 The helicase activity of UPF1 might therefore displace TERRA from telomeric ribonucleoprotein complexes -possibly containing TRF1, TRF2 and telomerase- or directly resolve RNA–DNA hybrids that form transiently at the telomeric replication fork.Citation40 Supporting a model in which UPF1 physically displaces TERRA from telomeres (), we have shown that cells mildly depleted for UPF1 by stable infection with shRNA-expressing lentiviruses accumulate TERRA molecules at telomeric heterochromatin in the absence of detectable changes in the half-life of TERRA.Citation18,Citation41 However, the accumulation of TERRA may be a consequence rather than a cause of the telomere dysfunctions induced by UPF1. Telomere uncapping induced by TRF2 knockdown or exposure to telomere G-strand DNA oligonucleotides has been shown to increase TERRA steady-state levels through pathways requiring the tumor suppressor protein p53.Citation42 Therefore, TERRA accumulation might be part of the complex DNA damage response evoked by uncapped telomeres that may occur in UPF1-deficient cells.
Another intriguing question raised by observations in my laboratory concerns the functional significance of the physical interaction between UPF1 and telomerase (). Both endogenous and ectopically expressed epitope-tagged UPF1 molecules physically interact with telomerase in human cancer cell nuclear extracts, independently of nucleic acid molecules.Citation25 It is unclear if this interaction is directly established between UPF1 and human telomerase reverse transcriptase (hTERT), the catalytic subunit of the telomerase holoenzyme.Citation30 Like UPF1, the human ever-shorter telomere protein A (hEST1A, also known as SMG6)—an NMD effector that interacts directly with UPF1—directly associates with telomerase through protein-protein and protein-RNA interactions with hTERT and human telomerase RNA (hTR), the RNA moiety of the telomerase holoenzyme, respectively ().Citation30,Citation43-Citation45 hEST1A, therefore, may mediate UPF1/hTERT interaction; in vitro experiments using recombinant proteins and immunoprecipitation experiments performed in cells depleted for either factor will help clarify this issue. In addition, we also have shown that depletion of ATR strongly reduces the amount of active telomerase co-immunoprecipitating with UPF1 without affecting the total cellular activity of telomerase.Citation25 Therefore, ATR may mediate UPF1/telomerase interaction either by creating a physical bridge between the two molecules or, more likely, by modifying the UPF1 phosphorylation state (see below). Regardless of how the interaction between UPF1 and telomerase is established, a hint suggesting its functional relevance comes from the observation that cells overexpressing an hTERT allele C-terminally fused to a human influenza hemagglutinin tag (hTERT-HA) fail to properly recruit UPF1 to telomeres.Citation25 Although it is catalytically active in vitro, hTERT-HA is unable to elongate telomeres in vivo, possibly because tag interference prevents the proper recruitment of the protein to telomeric loci.Citation46 Therefore, overexpressed nucleoplasmic hTERT may tether UPF1 away from telomeric loci, suggesting that telomerase may be involved in recruiting UPF1 at telomeres.
The interaction between UPF1 and telomerase may also constitute a regulatory step in telomerase activity. The Saccharomyces cescerevisiae helicase petite integration frequency 1 (Pif1p) interacts with telomerase and negatively regulates telomerase-mediated telomere elongation and telomere healing of intrachromosomal DSBs.Citation47-Citation49 Pif1p is thought to negatively regulate telomerase-mediated elongation of telomeres by unwinding the template region of telomerase component 1 (TLC1) RNA from single stranded telomeric 3′ ends.Citation47 Remarkably, although the murine hortologue of Pif1p interacts with telomerase at low levels, it does not play a major role in either regulating telomerase-mediated telomere elongation or telomere healing.Citation50,Citation51 Another helicase, perhaps UPF1, might have acquired the yeast Pif1p telomeric functions in mammalian cells. The generation of UPF1 alleles with separate semi-conservative telomere replication and telomerase regulation functions will help tremendously in verifying this intriguing hypothesis.
The process by which UPF1 is recruited to the telomere in vivo also remains unclear. As previously noted, the physical interaction between UPF1 and telomerase might represent a crucial step for UPF1 localization at telomeric heterochromatin.Citation25 The physical interaction between UPF1 and polδCitation16,Citation28 also suggests that UPF1 might travel together with the replication fork and be deposited by this machinery at sites of replicating DNA, including telomeres (). In addition to telomerase and replication-fork core components, other factors may be involved in UPF1 localization to telomeres. Indeed, in ChIP experiments ATR depletion led to decreased UPF1 binding to telomeres.Citation25 Similarly, UPF1 fails to properly accumulate on chromatin fractions from cells depleted for ATR, either treated with gamma rays or left untreated.Citation16 Human UPF1 is a phosphoprotein containing 28 consensus sites for PIKK-mediated phosphorylation (Serine/Threonine-Glutamine), 14 of which are clustered within its 140 C-terminal amino acids. ATR is able to directly phosphorylate UPF1 in vitro, although the specific substrate residues on UPF1 still need to be identified, and ATR depletion leads to a substantial decrease in cellular phosphorylated UPF1.Citation16 Therefore, it is likely that ATR-mediated phosphorylation of UPF1 is the molecular event contributing to UPF1 loading or stabilization at telomeres (). Indeed, ATR was shown to localize to telomeric heterochromatin in human cells transiting through the S-phase,Citation52 suggesting that, at telomeres, ATR could act locally on UPF1 to maintain or activate it. It will be interesting to identify the phosphorylation residues of UPF1 that are modified by ATR and test whether abrogation of such phosphorylation indeed prevents the localization of UPF1 at telomeres and onto the chromatin of replicating cells.
We have now reported a novel physical interaction between UPF1 and the shelterin component TPP1 (derived from the three acronyms TINT1, PTOP and PIP1).Citation19,Citation20,Citation25 TPP1 is an integral component of the telomeric heterochromatin and is recruited at telomeres through direct interaction with TRF1-interacting protein 2 (TIN2), another shelterin factor; TIN2, in turn, binds to telomeres through interaction with TRF1 and TRF2 ().Citation19,Citation20 UPF1 interaction with TPP1, which was demonstrated both using endogenous and ectopically expressed proteins, depends on the presence of an N-terminal oligonucleotide/oligosaccharide binding (OB)-fold domain on TPP1.Citation25 ShRNA-mediated depletion of TPP1 did not substantially alter the telomeric localization of UPF1, suggesting that TPP1 is not a recruitment factor for UPF1 (our unpublished observations); however, the remaining cellular levels of TPP1 after shRNA treatment might be sufficiently high to promote UPF1 binding to telomeric chromatin. The use of TPP1 gene knockout systems will help clarify this particular issue. Still, TPP1 may regulate UPF1 activity at telomeres during replication rather than recruiting or stabilizing it. Interestingly, TPP1 and the protection of telomeres 1 (POT1) protein form a complex that directly associates with active telomerase and regulates telomerase activity at telomeres.Citation53 An appealing hypothesis is that UPF1 might be part of the same complex and contributes to the TPP1/POT1-mediated regulation of telomerase.
A final, more general question is whether UPF1 is involved in the replication of genomic loci other than telomeres. The foci detected in the nucleus of pre-extracted cells in UPF1 IF experiments are unlikely to correspond to telomeres alone (). In addition, and as noted previously, UPF1 depletion leads to the accumulation of nuclear foci containing γH2AX and RPA, only a fraction of which co-localize with telomeric markers.Citation25 These observations indicate that UPF1 does not accumulate only at telomeres but instead broadly associates with different chromatin domains and promotes the stability of different genomic loci. The large-scale identification of the genomic regions that are preferentially bound by UPF1 and of those that become unstable upon UPF1 depletion is necessary to unravel the general function of UPF1 on chromatin. It is conceivable that UPF1 could promote the replication of a number of genomic regions sharing structural features with telomeres. For example, UPF1 might be generally required for the replication of repetitive DNA sequences or of heterochromatic loci constitutively associated with RNA moieties. Careful characterization of the common features of the genomic loci requiring UPF1 for their stability will reveal the nuclear functions of this versatile helicase.
It is now accepted that UPF1 has multiple cellular roles that allow it to promote both RNA and DNA quality control. The intimate connection of UPF1 with genomic stability executors such as ATR and the severe DNA damage occurring at different genomic locations, including telomeres, in UPF1-depleted cells place the protein among the cellular caretakers of genomic integrity. The double duties of UPF1 in RNA and DNA metabolism suggest a scenario in which UPF1 coordinates the stability of nucleic acids both in the nucleus and in the cytoplasm. A careful dissection of the functional relevance of the interactions between UPF1 and its different interaction partners, including ATR, TPP1, and telomerase, as well as the development of functionally separate UPF1 alleles will help appreciate the multifaceted functions of this versatile enzyme.
Acknowledgments
I am sincerely grateful to Joachim Lingner for enthusiastically supporting the establishment and development of the UPF1 projects when I was in his laboratory. I am grateful to the members of my laboratory and to Sarantis Gagos for helpful discussions. I apologize to my colleagues whose work was not cited in this article owing to space limitations. My laboratory is funded by ETH Zürich (ETH-03 08–3), the Swiss National Science Foundation (PP00P3–123356), the European Research Council (BFTERRA) and Fondazione Cariplo (2008–2507).
References
- Hwang J, Maquat LE. Nonsense-mediated mRNA decay (NMD) in animal embryogenesis: to die or not to die, that is the question. Curr Opin Genet Dev 2011; 21:422 - 30; http://dx.doi.org/10.1016/j.gde.2011.03.008; PMID: 21550797
- Isken O, Maquat LE. The multiple lives of NMD factors: balancing roles in gene and genome regulation. Nat Rev Genet 2008; 9:699 - 712; http://dx.doi.org/10.1038/nrg2402; PMID: 18679436
- Nicholson P, Yepiskoposyan H, Metze S, Zamudio Orozco R, Kleinschmidt N, Mühlemann O. Nonsense-mediated mRNA decay in human cells: mechanistic insights, functions beyond quality control and the double-life of NMD factors. Cell Mol Life Sci 2010; 67:677 - 700; http://dx.doi.org/10.1007/s00018-009-0177-1; PMID: 19859661
- Leeds P, Peltz SW, Jacobson A, Culbertson MR. The product of the yeast UPF1 gene is required for rapid turnover of mRNAs containing a premature translational termination codon. Genes Dev 1991; 5:2303 - 14; http://dx.doi.org/10.1101/gad.5.12a.2303; PMID: 1748286
- Page MF, Carr B, Anders KR, Grimson A, Anderson P. SMG-2 is a phosphorylated protein required for mRNA surveillance in Caenorhabditis elegans and related to Upf1p of yeast. Mol Cell Biol 1999; 19:5943 - 51; PMID: 10454541
- Sun X, Perlick HA, Dietz HC, Maquat LE. A mutated human homologue to yeast Upf1 protein has a dominant-negative effect on the decay of nonsense-containing mRNAs in mammalian cells. Proc Natl Acad Sci USA 1998; 95:10009 - 14; http://dx.doi.org/10.1073/pnas.95.17.10009; PMID: 9707591
- Mendell JT, Sharifi NA, Meyers JL, Martinez-Murillo F, Dietz HC. Nonsense surveillance regulates expression of diverse classes of mammalian transcripts and mutes genomic noise. Nat Genet 2004; 36:1073 - 8; http://dx.doi.org/10.1038/ng1429; PMID: 15448691
- Kim YK, Furic L, Desgroseillers L, Maquat LE. Mammalian Staufen1 recruits Upf1 to specific mRNA 3′UTRs so as to elicit mRNA decay. Cell 2005; 120:195 - 208; http://dx.doi.org/10.1016/j.cell.2004.11.050; PMID: 15680326
- Kim YK, Furic L, Parisien M, Major F, DesGroseillers L, Maquat LE. Staufen1 regulates diverse classes of mammalian transcripts. EMBO J 2007; 26:2670 - 81; http://dx.doi.org/10.1038/sj.emboj.7601712; PMID: 17510634
- Kaygun H, Marzluff WF. Regulated degradation of replication-dependent histone mRNAs requires both ATR and Upf1. Nat Struct Mol Biol 2005; 12:794 - 800; http://dx.doi.org/10.1038/nsmb972; PMID: 16086026
- Muller B, Blackburn J, Feijoo C, Zhao X, Smythe C. DNA-activated protein kinase functions in a newly observed S phase checkpoint that links histone mRNA abundance with DNA replication. J Cell Biol 2007; 179:1385 - 98; http://dx.doi.org/10.1083/jcb.200708106; PMID: 18158334
- Singh G, Jakob S, Kleedehn MG, Lykke-Andersen J. Communication with the exon-junction complex and activation of nonsense-mediated decay by human Upf proteins occur in the cytoplasm. Mol Cell 2007; 27:780 - 92; http://dx.doi.org/10.1016/j.molcel.2007.06.030; PMID: 17803942
- Applequist SE, Selg M, Raman C, Jack HM. Cloning and characterization of HUPF1, a human homolog of the Saccharomyces cerevisiae nonsense mRNA-reducing UPF1 protein. Nucleic Acids Res 1997; 25:814 - 21; http://dx.doi.org/10.1093/nar/25.4.814; PMID: 9064659
- Mendell JT, ap Rhys CM, Dietz HC. Separable roles for rent1/hUpf1 in altered splicing and decay of nonsense transcripts. Science 2002; 298:419 - 22; http://dx.doi.org/10.1126/science.1074428; PMID: 12228722
- Atkin AL, Altamura N, Leeds P, Culbertson MR. The majority of yeast UPF1 co-localizes with polyribosomes in the cytoplasm. Mol Biol Cell 1995; 6:611 - 25; PMID: 7545033
- Azzalin CM, Lingner J. The human RNA surveillance factor UPF1 is required for S phase progression and genome stability. Curr Biol 2006; 16:433 - 9; http://dx.doi.org/10.1016/j.cub.2006.01.018; PMID: 16488880
- Azzalin CM, Lingner J. The double life of UPF1 in RNA and DNA stability pathways. Cell Cycle 2006; 5:1496 - 8; http://dx.doi.org/10.4161/cc.5.14.3093; PMID: 16861888
- Azzalin CM, Reichenbach P, Khoriauli L, Giulotto E, Lingner J. Telomeric repeat containing RNA and RNA surveillance factors at mammalian chromosome ends. Science 2007; 318:798 - 801; http://dx.doi.org/10.1126/science.1147182; PMID: 17916692
- O'Sullivan RJ, Karlseder J. Telomeres: protecting chromosomes against genome instability. Nat Rev Mol Cell Biol 2010; 11:171 - 81; PMID: 20125188
- Palm W, de Lange T. How Shelterin Protects Mammalian Telomeres. Annu Rev Genet 2008; 42:301 - 34; http://dx.doi.org/10.1146/annurev.genet.41.110306.130350; PMID: 18680434
- de Turris V, Nicholson P, Orozco RZ, Singer RH, Muhlemann O. Cotranscriptional effect of a premature termination codon revealed by live-cell imaging. RNA 2011; 17; http://dx.doi.org/10.1261/rna.02918111; PMID: 22028363
- Chawla R, Azzalin CM. The telomeric transcriptome and SMG proteins at the crossroads. Cytogenet Genome Res 2008; 122:194 - 201; http://dx.doi.org/10.1159/000167804; PMID: 19188687
- Cimprich KA, Cortez D. ATR: an essential regulator of genome integrity. Nat Rev Mol Cell Biol 2008; 9:616 - 27; http://dx.doi.org/10.1038/nrm2450; PMID: 18594563
- Lopez-Contreras AJ, Fernandez-Capetillo O. The ATR barrier to replication-born DNA damage. DNA Repair (Amst) 2010; 9:1249 - 55; http://dx.doi.org/10.1016/j.dnarep.2010.09.012; PMID: 21036674
- Chawla R, Redon S, Raftopoulou C, Wischnewski H, Gagos S, Azzalin CM. Human UPF1 interacts with TPP1 and telomerase and sustains telomere leading-strand replication. EMBO J 2011; 30:4047 - 58; http://dx.doi.org/10.1038/emboj.2011.280; PMID: 21829167
- Kashima I, Yamashita A, Izumi N, Kataoka N, Morishita R, Hoshino S, et al. Binding of a novel SMG-1-Upf1-eRF1-eRF3 complex (SURF) to the exon junction complex triggers Upf1 phosphorylation and nonsense-mediated mRNA decay. Genes Dev 2006; 20:355 - 67; http://dx.doi.org/10.1101/gad.1389006; PMID: 16452507
- Weng Y, Czaplinski K, Peltz SW. Genetic and biochemical characterization of mutations in the ATPase and helicase regions of the Upf1 protein. Mol Cell Biol 1996; 16:5477 - 90; PMID: 8816461
- Carastro LM, Tan CK, Selg M, Jack HM, So AG, Downey KM. Identification of delta helicase as the bovine homolog of HUPF1: demonstration of an interaction with the third subunit of DNA polymerase delta. Nucleic Acids Res 2002; 30:2232 - 43; http://dx.doi.org/10.1093/nar/30.10.2232; PMID: 12000843
- Cifuentes-Rojas C, Shippen DE. Telomerase regulation. Mutat Res 2011; http://dx.doi.org/10.1016/j.mrfmmm.2011.10.003; PMID: 22032831
- Hug N, Lingner J. Telomere length homeostasis. Chromosoma 2006; 115:413 - 25; http://dx.doi.org/10.1007/s00412-006-0067-3; PMID: 16741708
- Arora R, Brun CM, Azzalin CM. TERRA: Long Noncoding RNA at Eukaryotic Telomeres. Prog Mol Subcell Biol 2011; 51:65 - 94; http://dx.doi.org/10.1007/978-3-642-16502-3_4; PMID: 21287134
- Luke B, Lingner J. TERRA: telomeric repeat-containing RNA. EMBO J 2009; 28:2503 - 10; http://dx.doi.org/10.1038/emboj.2009.166; PMID: 19629047
- Schoeftner S, Blasco MA. Developmentally regulated transcription of mammalian telomeres by DNA-dependent RNA polymerase II. Nat Cell Biol 2008; 10:228 - 36; http://dx.doi.org/10.1038/ncb1685; PMID: 18157120
- Deng Z, Norseen J, Wiedmer A, Riethman H, Lieberman PM. TERRA RNA binding to TRF2 facilitates heterochromatin formation and ORC recruitment at telomeres. Mol Cell 2009; 35:403 - 13; http://dx.doi.org/10.1016/j.molcel.2009.06.025; PMID: 19716786
- Farnung BO, Giulotto E, Azzalin CM. Promoting transcription of chromosome ends. Transcr 2010; 1:140 - 3; http://dx.doi.org/10.4161/trns.1.3.13191; PMID: 21326888
- Nergadze SG, Farnung BO, Wischnewski H, Khoriauli L, Vitelli V, Chawla R, et al. CpG-island promoters drive transcription of human telomeres. RNA 2009; 15:2186 - 94; http://dx.doi.org/10.1261/rna.1748309; PMID: 19850908
- Redon S, Reichenbach P, Lingner J. The non-coding RNA TERRA is a natural ligand and direct inhibitor of human telomerase. Nucleic Acids Res 2010; 38:5797 - 806; http://dx.doi.org/10.1093/nar/gkq296; PMID: 20460456
- Xu Y, Kimura T, Komiyama M. Human telomere RNA and DNA form an intermolecular G-quadruplex. Nucleic Acids Symp Ser (Oxf) 2008; 52:169 - 70; http://dx.doi.org/10.1093/nass/nrn086; PMID: 18776307
- Xu Y, Suzuki Y, Ito K, Komiyama M. Telomeric repeat-containing RNA structure in living cells. Proc Natl Acad Sci USA 2010; 107:14579 - 84; http://dx.doi.org/10.1073/pnas.1001177107; PMID: 20679250
- Franks TM, Singh G, Lykke-Andersen J. Upf1 ATPase-dependent mRNP disassembly is required for completion of nonsense- mediated mRNA decay. Cell 2010; 143:938 - 50; http://dx.doi.org/10.1016/j.cell.2010.11.043; PMID: 21145460
- Azzalin CM, Lingner J. Telomeres: the silence is broken. Cell Cycle 2008; 7:1161 - 5; http://dx.doi.org/10.4161/cc.7.9.5836; PMID: 18418035
- Caslini C, Connelly JA, Serna A, Broccoli D, Hess JL. MLL associates with telomeres and regulates telomeric repeat-containing RNA transcription. Mol Cell Biol 2009; 29:4519 - 26; http://dx.doi.org/10.1128/MCB.00195-09; PMID: 19528237
- Redon S, Reichenbach P, Lingner J. Protein RNA and protein protein interactions mediate association of human EST1A/SMG6 with telomerase. Nucleic Acids Res 2007; 35:7011 - 22; http://dx.doi.org/10.1093/nar/gkm724; PMID: 17940095
- Reichenbach P, Höss M, Azzalin CM, Nabholz M, Bucher P, Lingner J. A human homolog of yeast Est1 associates with telomerase and uncaps chromosome ends when overexpressed. Curr Biol 2003; 13:568 - 74; http://dx.doi.org/10.1016/S0960-9822(03)00173-8; PMID: 12676087
- Snow BE, Erdmann N, Cruickshank J, Goldman H, Gill RM, Robinson MO, et al. Functional conservation of the telomerase protein Est1p in humans. Curr Biol 2003; 13:698 - 704; http://dx.doi.org/10.1016/S0960-9822(03)00210-0; PMID: 12699629
- Counter CM, Hahn WC, Wei W, Caddle SD, Beijersbergen RL, Lansdorp PM, et al. Dissociation among in vitro telomerase activity, telomere maintenance, and cellular immortalization. Proc Natl Acad Sci USA 1998; 95:14723 - 8; http://dx.doi.org/10.1073/pnas.95.25.14723; PMID: 9843956
- Boule JB, Vega LR, Zakian VA. The yeast Pif1p helicase removes telomerase from telomeric DNA. Nature 2005; 438:57 - 61; http://dx.doi.org/10.1038/nature04091; PMID: 16121131
- Schulz VP, Zakian VA. The saccharomyces PIF1 DNA helicase inhibits telomere elongation and de novo telomere formation. Cell 1994; 76:145 - 55; http://dx.doi.org/10.1016/0092-8674(94)90179-1; PMID: 8287473
- Zhou J, Monson EK, Teng SC, Schulz VP, Zakian VA. Pif1p helicase, a catalytic inhibitor of telomerase in yeast. Science 2000; 289:771 - 4; http://dx.doi.org/10.1126/science.289.5480.771; PMID: 10926538
- Snow BE, Mateyak M, Paderova J, Wakeham A, Iorio C, Zakian V, et al. Murine Pif1 interacts with telomerase and is dispensable for telomere function in vivo. Mol Cell Biol 2007; 27:1017 - 26; http://dx.doi.org/10.1128/MCB.01866-06; PMID: 17130244
- Reynolds GE, Gao Q, Miller D, Snow BE, Harrington LA, Murnane JP. PIF1 disruption or NBS1 hypomorphism does not affect chromosome healing or fusion resulting from double-strand breaks near telomeres in murine embryonic stem cells. DNA Repair (Amst) 2011; 10:1164 - 73; http://dx.doi.org/10.1016/j.dnarep.2011.09.002; PMID: 21945094
- Verdun RE, Karlseder J. The DNA damage machinery and homologous recombination pathway act consecutively to protect human telomeres. Cell 2006; 127:709 - 20; http://dx.doi.org/10.1016/j.cell.2006.09.034; PMID: 17110331
- Wang F, Podell ER, Zaug AJ, Yang Y, Baciu P, Cech TR, et al. The POT1-TPP1 telomere complex is a telomerase processivity factor. Nature 2007; 445:506 - 10; http://dx.doi.org/10.1038/nature05454; PMID: 17237768