Abstract
The spatial and temporal regulation of gene expression during development requires the concerted actions of sequence-specific transcriptional regulators and epigenetic chromatin modifiers, which are thought to function within precise nuclear compartments. However, coordination of these activities within the dynamic context of the nuclear environment is still largely unresolved. Here we discuss the implications of our recent work showing that transcriptional repression by the Msx1 homeoprotein is associated with global redistribution of the H3K27me3 repressive mark to the nuclear periphery during development.
Introduction
Vertebrate homeobox genes encompass an extensive family of developmental regulators with broad significance for virtually all aspects of embryogenesis. Among these, the Msx1 homeobox gene is expressed in various tissue contexts during embryonic development, which include the limbs, neural crest, neural tube, craniofacial derivatives and the mammary gland.Citation1-Citation11 Although expressed in diverse tissue environments, in these various contexts Msx1 expression is usually detected in undifferentiated progenitor cells that play key roles in regulating cellular differentiation.Citation1-Citation11 As a transcriptional repressor, the Msx1 homeoprotein regulates cellular differentiation by repression of target genes during development in varied biological contexts,Citation7,Citation9,Citation12-Citation14 however, the mechanisms by which Msx1 mediates repression of specific target genes during development are still unclear.
Epigenetic chromatin modifiers play critical roles in controlling gene expression programs during development. Among these, the Polycomb proteins, which form multi-protein complexes that comprise the PRC1 and PRC2 complexes, function to repress gene expression.Citation15-Citation17 In mammalian cells, the PRC2 complex, which includes the enzymatic component, Ezh2, as well as Suz12 and EED, imparts the repressive trimethyl mark at lysine 27 of histone H3 (H3K27me3).Citation18-Citation20 The PRC2 complex regulates many biological processes, including differentiation, maintaining cell identity, and stem-cell plasticity.Citation15,Citation21-Citation28 Notably, Ezh2 is expressed in undifferentiated myoblast cells and inhibits differentiation of these cells, as does Msx1.Citation29,Citation30 In addition to epigenetic modifications, another important component of transcriptional regulation is the spatial organization of chromatin within the nucleus. Indeed, it is now widely appreciated that the spatial organization of the nucleus is a nonrandom, highly dynamic and context-dependent process and that the sub-nuclear positioning of genes contributes to their transcriptional status of either being induced or repressed.Citation31-Citation39 However, the mechanisms modulating the recruitment of the mammalian PRC2 complex to chromatin within precise nuclear compartments remain unclear.
In a recent study, we reported that transcriptional repression by the Msx1 homeoprotein is associated with a global redistribution of the H3K27me3 repressive mark to the nuclear periphery during development.Citation40 Here we discuss these findings and their implications.
Msx1 and Its Repressed Target Genes are Located at The Nuclear Periphery
During development, Msx1 is localized at the nuclear periphery in the nascent limb of mouse embryos in vivo as well as in myoblast cells in culture, but not in other tissues where Msx1 is expressed in embryos, such as the neural tube. This observation that Msx1 is localized to the nuclear periphery in specific biological contexts raises two important questions: (1) why does Msx1 localize at the nuclear periphery, and (2) how does Msx1 localize at the nuclear periphery in specific biological contexts? Regarding the issue of why Msx1 is localized to the periphery, it appears that this localization is necessary for Msx1 to repress its target genes, which are also at the periphery. Indeed, when we identified Msx1 target genes and then assessed their sub-nuclear localization in myoblast cells we found that many of the repressed (but not activated) genes were located at the nuclear periphery. Specifically, we expressed exogenous Msx1 in C2C12 cells, which is a proliferating myoblast cell line that expresses low levels of endogenous Msx1 and then used a combination of gene expression profiling and chromatin-immunoprecipitation followed by high-throughput sequencing (ChIP-Seq) to identify Msx1 target genes and then finally verified these as target for endogenous Msx1 in the developing limb. This to the identification of 79 upregulated (activated) and 87 downregulated (repressed) target genes.Citation2,Citation12,Citation13 Among these, genes that were both bound and downregulated by Msx1 were associated with the nuclear periphery in both C2C12 cells and primary myoblast cells, while genes that were bound and upregulated by Msx1 were not.
Msx1 Interacts with PRC2 Complex
Consistent with the function of Msx1 as a transcriptional repressor and negative regulator of muscle cell differentiation,Citation2,Citation3,Citation9,Citation12,Citation13 we found that target genes repressed by Msx1 include those involved in muscle cell differentiation. Interestingly, Msx1 binds to multiple sites on such target genes in myoblast cells as well as in the developing limb. It is possible that the multiple Msx1 genomic binding sites are involved in controlling gene expression through complex chromatin interactions.Citation40,Citation41 Notably, these Msx1 genomic binding sites include known regulatory regions such as the CER of MyoD, as well as the -58 kb distal regulatory element of Myf5.Citation12,Citation14,Citation42,Citation43
The culmination of data from us and others has shown that Msx1 regulates target gene expression in the context of a large protein complexCitation7,Citation12,Citation13,Citation44-Citation48 (Wang and Abate-Shen, unpublished data). The simplest model is that Msx1 regulates target gene expression during development by forming a protein complex with universal protein partners that are present in all or most cell types, as well as cell type specific protein partners that occur in distinct biological contexts (). We presume that the universal protein partners play critical roles for the activities of Msx1 in all or most cell contexts, while the cell type specific protein partners contribute to the selective activities of Msx1 in distinct biological contexts.
Figure 1. Msx1 forms a multi-protein complex to regulate target gene expression. The proposed “universal” protein partners are those that Msx1 interacts with in all (or most) cell types; the cell type specific (tissue specific) protein partners are those restricted to distinct biological contexts.
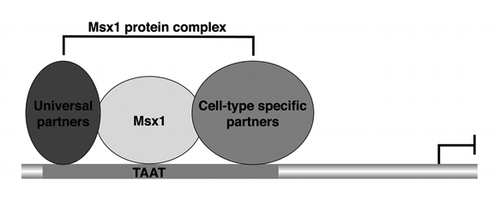
What are the consequences of these protein interactions? We believe that one important one is their effect on chromatin modifications. Indeed, Msx1 genomic binding is specifically coincident with enrichment of the H3K27me3 repressive mark on repressed target genes.Citation15,Citation16,Citation18,Citation21,Citation25,Citation28,Citation49 This observation suggests that Msx1 represses gene expression through recruitment of the Polycomb repressive mark H3K27me3 to target genes at the nuclear periphery.
Consistent with the observation that Msx1 genomic binding promotes enrichment of H3K27me3 on target genes, Msx1 directly associates with components of the PRC2 complexCitation15,Citation49 in myoblast cell as well as in the developing limb at the nuclear periphery. Notably, the homeodomain of Msx1 that mediates DNA binding also serves as a protein interaction domain with PRC2 complex.Citation5,Citation7,Citation47,Citation48 Furthermore, Msx1 and PRC2 are co-localized at regulatory regions of Msx1 target genes, and Msx1 association with PRC2 complexes is necessary for myoblast differentiation and repression of myogenic targets.
Collectively, our data suggest that Msx1 interacts with the PRC2 complex through its homeodomain and recruits it to target genes to repress their expression. Additionally, the observation that recruitment of the PRC2 complex by Msx1 is mediated via the homeodomain, suggests that other homeoproteins may also recruit PRC2 complex to target genes to regulate their expression. However, this remains to be experimentally established.
Msx1 Redistributes H3K27me3 Mark in Gene-Specific Way
As discussed above, Msx1 recruits the PRC2 complex to its target genes and is associated with enrichment of the H3K27me3 mark on Msx1 targets. This raises the interesting question of whether Msx1 affects the activity of the PRC2 complex to increase total levels of the H3K27me3 mark. Our data suggests that Msx1 does not change the overall levels of H3K27me3, but rather redistributes the PRC2 complex and H3K27me3 mark in gene-specific manner away from Msx1 non-target genes and toward Msx1 target genes ().
Figure 2. Msx1 redistributes the PRC2 complex and H3K27me3 marks to target genes. (A) Gene specific effects. (B) Global redistribution.
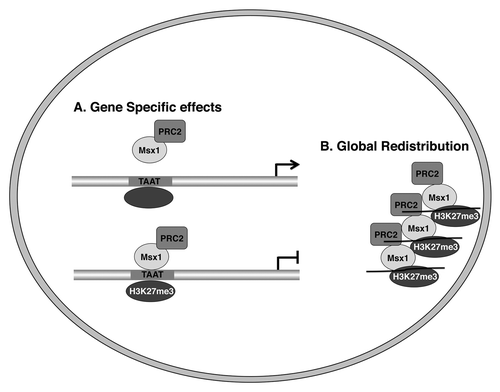
This mode of gene regulation via redistribution of the universal chromatin modifiers, such as the PRC2 complex, is exemplified by sequestering of the PRC2 complex by Msx1 away from its non-target genes such as the muscle specific genes, Mck and MHCIIb that have the transcriptional factor YY1 bound at their promoters.Citation29,Citation30,Citation50 How does Msx1 redistribute the PRC2 complex and H3K27me3 mark in a gene-specific manner? There are three possibilities: (1) that the PRC2 complex has a stronger affinity for Msx1 than it does for YY1;Citation50 (2) that posttranslational modification of the PRC2 complex modulates its ability to interact with different partnersCitation29,Citation30,Citation50 or (3) that Msx1 recruits the YY1/PRC2 complex away from YY1 targets to Msx1 targets as observed in Msx1-expressing myoblast cells (Wang and Abate-Shen, unpublished data). We prefer the third possibility, since our additional studies suggest that other homeoproteins, as well as other transcription factors, may also function via redistribution of specific histone methyltransferases and specific histone marks to target genes to regulate their expression.
Msx1 Redistributes H3K27me3 Mark to The Nuclear Periphery in Global Way
The observations that Msx1 redistributes the PRC2 complex and the H3K27me3 histone mark away from genes that are not targets of Msx1 to its target genes, and that Msx1 is localized to the nuclear periphery in myoblast cells and in the developing limb, led us to investigate whether Msx1 promotes a global redistribution of PRC2 complex and H3K27me3 mark to the nuclear periphery. Indeed, we found this to be the case in myoblast cells in vitro and in the developing limb of mouse embryos in vivo (). Notably, the H3K27me3 mark was previously find to be enriched in lamina-associated domains (LADs).Citation51 Comparison of LAD sites with the Msx1 genomic binding sites revealed that approximately 20% of Msx1-bound regions were located within or were overlapping LADs (unpublished observations). Conversely, the majority (68–71%) of LADs contained at least one Msx1-bound region and 434 of 580 (75%) LADs associated with the nuclear periphery contained at least one Msx1-bound region (unpublished observations). Thus, the distinctive localization of Msx1 to the nuclear periphery is accompanied by enrichment of its repressed target genes, as well as a tendency for Msx1 genomic binding to occur in proximity to LADs associated with the nuclear periphery.
Notably, studies have shown that mouse embryonic stem cells show enrichment for the H3K27me3 mark at the nuclear periphery, whereas differentiated cells display a more diffuse pattern of H3K27me3 throughout the nucleus.Citation25,Citation50 Our studies have shown that the H3K27me3 repressive mark was globally enriched at the nuclear periphery in myogenic progenitor cells during development.Citation40 These findings suggest that enrichment of the H3K27me3 mark at the nuclear periphery may be a common feature of stem cells.
Finally, since we identified an almost equal number of upregulated target genes as there were repressed genes,Citation40 we wondered how Msx1 regulates these activated target genes. Counterintuitively, Msx1 genomic binding on these activated target genes is also coincident with enrichment of the H3K27me3 repressor mark. However, the activated but not repressed targets are also enriched for the activator mark H3K4me3, and these activated genes are not located at the nuclear periphery,Citation40 suggesting that perhaps, the transcriptional outcome is dependent on sub-nuclear location of target genes as well as the status of histone marks on these target genes. Indeed, recent studies have shown that repositioning of MyoD to the nuclear interior is associated with its activation and binding by TAF3 during myoblast differentiation.Citation52 Therefore, whether a target gene is “activated” or “repressed” may depend on its sub-nuclear localization, the distribution of activator and repressor chromatin marks and components of the core transcriptional complex.Citation16,Citation20,Citation34,Citation52 In general, our studies provide insight into the general features of how chromatin modifications contribute to the dynamic spatial and temporal control of lineage-specific target genes in diverse cell types during development.
Models for Msx1 Recruits The PRC2 Complex and H3K27me3 Mark to The Nuclear Periphery
Still unresolved is the interesting question of how Msx1 redistributes the PRC2 complex and H3K27me3 mark to the nuclear periphery. We observed that this redistribution is not only dependent on Msx1, but also its protein partners such as PIAS1. Indeed, the transcriptionally active Msx1 protein complex includes PIAS1 as well as the PRC2 complex.Citation12,Citation40 Notably, the N-terminal of Msx1, Msx1 (139–303), is required for interaction with another protein partner, namely histone H1b, but still retains the ability to bind the PRC2 complex.Citation13,Citation40 The loss of binding to one key number of the Msx1 transcriptional machinery results in the failure of redistribution of the PRC2 complex and the H3K27me3 mark to the nuclear periphery.Citation13,Citation40 These observations suggest that the co-operative binding of the Msx1, the PRC2 complex, and other Msx1 protein partners at target genes contributes to the global redistribution of the H3K27me3 mark to the nuclear periphery in myoblast cells. Additionally, as note above, Msx1 is robustly expressed in the limb bud as well as in the neural tube during development, but is localized at the nuclear periphery only in the limb but not in the neural tube; thus, cell context also contributes to these features.
There are four possible mechanisms by which Msx1 may recruit the PRC2 complex and the H3K27me3 mark to the nuclear periphery (). The first case depicts Msx1 binding to a component of the nuclear lamina, thereby tethering its target genes to the nuclear periphery. In parallel, Msx1 may recruit the PRC2 complex to its target genes and lead to enrichment of H3K27me3 at the nuclear periphery. In the second scenario, Msx1 may recruit PRC2 to targets, leading to local H3K27 methylation; chromatin with the H3K27me3 mark may have intrinsic affinity for the nuclear lamina, leading to relocation of the Msx1 target genes to the nuclear periphery. In the third possibility, Msx1 may (for some reason) only be able to bind to genes at the periphery; thus, the PRC2 complex is only recruited to the peripheral genes, and consequentially H3K27me3 accumulates at the nuclear periphery (in this model there is no movement of genes). In the fourth case, cooperative binding between Msx1 and PRC2 complex involves other important tissue specific or cell-type specific factors. The as yet unknown tissue specific or cell-type specific factors interact with Msx1/PRC2 complex to form a multi-protein complex. The resulting Msx1 protein complex may bind to a component of the nuclear lamina, and consequentially H3K27me3 accumulates on Msx1 target genes localized at the nuclear periphery (in this model there also is no movement of genes).
We prefer the fourth possibility for the following reasons: (1) our FISH data show that expression of Msx1 in myoblast cells does not affect localization of the Msx1 repressed target gene to the nuclear periphery;Citation40 (2) Msx1 is localized at the nuclear periphery in the limb bud but not in the neural tube;Citation40 (3) Msx1 interacts with Lamin A and nuclear lamina associated protein Emerin in myoblast cells (Wang and Abate-Shen, unpublished data). Further studies will be required to distinguish between these possibilities to determine the precise mechanism by which Msx1 brings PRC2/H3K27me3 to the nuclear periphery. In particular, the identification of cell-type specific or tissue specific protein partners will help us understand how Msx1 redistributes the PRC2 complex and the H3K27me3 mark in specific developmental contexts. High throughput identification of Msx1 complex by proteomic approaches from different tissues during development will provide unique insight into how Msx1 redistributes the PRC2 complex and the H3K27me3 mark to the nuclear periphery in specific developmental contexts during development.
Conclusions
We propose that transcriptional repression and regulation of differentiation by the Msx1 homeoprotein are regulated at different layers. First, Msx1 needs to form a protein complex to control target gene expression (), an important component of which is to bring the PRC2 complex to repressed target genes. Indeed, the interaction of Msx1 with PRC2 is required for transcriptional repression and myoblast differentiation. In this respect, our studies provide new insights regarding how sequence-specific transcription factors control gene expression programs via interaction with protein partners. Second, we have shown that Msx1 recruits the PRC2 complex from genes not regulated by Msx1 to its own target genes, and, in turn, redistributes the H3K27me3 repressive mark from non-targets to Msx1 target genes in a gene-specific way (). Notably, recruitment of the PRC2 complex and redistribution of the H3K27me3 repressive mark by Msx1 is mediated via the homeodomain, suggesting that other homeoproteins may also recruit methyltransferase enzymes to target genes in a similar manner and regulate their expression. Finally, our studies have shown that in specific developmental contexts, Msx1 recruits the PRC2 complex and promotes the dynamic redistribution of the H3K27me3 repressive mark in a global way to the nuclear periphery (). In the broadest sense, our findings suggest that transcriptional repression can be viewed not only as a consequence of the dynamic control of chromatin modifications but also the result of “locating” repressive marks to appropriate spatial domains within the nucleus. Further studies are required to identify the mechanism of how Msx1 brings PRC2/H3K27me3 to the nuclear periphery.
Acknowledgments
We thank Drs Shazia Irshad and Aditya Dutta for many discussions and helpful comments on the manuscript. This work was supported by the T.J. Martell Foundation for Leukemia, Cancer and AIDS Research (C.A.-S.) and by grant HD029446 (C.A.-S.).
References
- Lallemand Y, Nicola MA, Ramos C, Bach A, Cloment CS, Robert B. Analysis of Msx1; Msx2 double mutants reveals multiple roles for Msx genes in limb development. Development 2005; 132:3003 - 14; http://dx.doi.org/10.1242/dev.01877; PMID: 15930102
- Hu G, Lee H, Price SM, Shen MM, Abate-Shen C. Msx homeobox genes inhibit differentiation through upregulation of cyclin D1. Development 2001; 128:2373 - 84; PMID: 11493556
- Odelberg SJ, Kollhoff A, Keating MT. Dedifferentiation of mammalian myotubes induced by msx1. Cell 2000; 103:1099 - 109; http://dx.doi.org/10.1016/S0092-8674(00)00212-9; PMID: 11163185
- Cornelison DD, Olwin BB, Rudnicki MA, Wold BJ. MyoD(-/-) satellite cells in single-fiber culture are differentiation defective and MRF4 deficient. Dev Biol 2000; 224:122 - 37; http://dx.doi.org/10.1006/dbio.2000.9682; PMID: 10926754
- Bendall AJ, Abate-Shen C. Roles for Msx and Dlx homeoproteins in vertebrate development. Gene 2000; 247:17 - 31; http://dx.doi.org/10.1016/S0378-1119(00)00081-0; PMID: 10773441
- Houzelstein D, Auda-Boucher G, Chéraud Y, Rouaud T, Blanc I, Tajbakhsh S, et al. The homeobox gene Msx1 is expressed in a subset of somites, and in muscle progenitor cells migrating into the forelimb. Development 1999; 126:2689 - 701; PMID: 10331980
- Bendall AJ, Ding J, Hu G, Shen MM, Abate-Shen C. Msx1 antagonizes the myogenic activity of Pax3 in migrating limb muscle precursors. Development 1999; 126:4965 - 76; PMID: 10529415
- Catron KM, Wang H, Hu G, Shen MM, Abate-Shen C. Comparison of MSX-1 and MSX-2 suggests a molecular basis for functional redundancy. Mech Dev 1996; 55:185 - 99; http://dx.doi.org/10.1016/0925-4773(96)00503-5; PMID: 8861098
- Woloshin P, Song K, Degnin C, Killary AM, Goldhamer DJ, Sassoon D, et al. MSX1 inhibits myoD expression in fibroblast x 10T1/2 cell hybrids. Cell 1995; 82:611 - 20; http://dx.doi.org/10.1016/0092-8674(95)90033-0; PMID: 7664340
- Davidson D. The function and evolution of Msx genes: pointers and paradoxes. Trends Genet 1995; 11:405 - 11; http://dx.doi.org/10.1016/S0168-9525(00)89124-6; PMID: 7482767
- Davidson DR, Crawley A, Hill RE, Tickle C. Position-dependent expression of two related homeobox genes in developing vertebrate limbs. Nature 1991; 352:429 - 31; http://dx.doi.org/10.1038/352429a0; PMID: 1677742
- Lee H, Quinn JC, Prasanth KV, Swiss VA, Economides KD, Camacho MM, et al. PIAS1 confers DNA-binding specificity on the Msx1 homeoprotein. Genes Dev 2006; 20:784 - 94; http://dx.doi.org/10.1101/gad.1392006; PMID: 16600910
- Lee H, Habas R, Abate-Shen C. MSX1 cooperates with histone H1b for inhibition of transcription and myogenesis. Science 2004; 304:1675 - 8; http://dx.doi.org/10.1126/science.1098096; PMID: 15192231
- Goldhamer DJ, Brunk BP, Faerman A, King A, Shani M, Emerson CP Jr.. Embryonic activation of the myoD gene is regulated by a highly conserved distal control element. Development 1995; 121:637 - 49; PMID: 7720572
- Margueron R, Reinberg D. The Polycomb complex PRC2 and its mark in life. Nature 2011; 469:343 - 9; http://dx.doi.org/10.1038/nature09784; PMID: 21248841
- Sawarkar R, Paro R. Interpretation of developmental signaling at chromatin: the Polycomb perspective. Dev Cell 2010; 19:651 - 61; http://dx.doi.org/10.1016/j.devcel.2010.10.012; PMID: 21074716
- Schuettengruber B, Cavalli G. Recruitment of polycomb group complexes and their role in the dynamic regulation of cell fate choice. Development 2009; 136:3531 - 42; http://dx.doi.org/10.1242/dev.033902; PMID: 19820181
- Cao R, Wang L, Wang H, Xia L, Erdjument-Bromage H, Tempst P, et al. Role of histone H3 lysine 27 methylation in Polycomb-group silencing. Science 2002; 298:1039 - 43; http://dx.doi.org/10.1126/science.1076997; PMID: 12351676
- Kuzmichev A, Nishioka K, Erdjument-Bromage H, Tempst P, Reinberg D. Histone methyltransferase activity associated with a human multiprotein complex containing the Enhancer of Zeste protein. Genes Dev 2002; 16:2893 - 905; http://dx.doi.org/10.1101/gad.1035902; PMID: 12435631
- Schwartz YB, Pirrotta V. Polycomb silencing mechanisms and the management of genomic programmes. Nat Rev Genet 2007; 8:9 - 22; http://dx.doi.org/10.1038/nrg1981; PMID: 17173055
- Ezhkova E, Lien WH, Stokes N, Pasolli HA, Silva JM, Fuchs E. EZH1 and EZH2 cogovern histone H3K27 trimethylation and are essential for hair follicle homeostasis and wound repair. Genes Dev 2011; 25:485 - 98; http://dx.doi.org/10.1101/gad.2019811; PMID: 21317239
- Woo CJ, Kharchenko PV, Daheron L, Park PJ, Kingston RE. A region of the human HOXD cluster that confers polycomb-group responsiveness. Cell 2010; 140:99 - 110; http://dx.doi.org/10.1016/j.cell.2009.12.022; PMID: 20085705
- Mendenhall EM, Koche RP, Truong T, Zhou VW, Issac B, Chi AS, et al. GC-rich sequence elements recruit PRC2 in mammalian ES cells. PLoS Genet 2010; 6:e1001244; http://dx.doi.org/10.1371/journal.pgen.1001244; PMID: 21170310
- Sing A, Pannell D, Karaiskakis A, Sturgeon K, Djabali M, Ellis J, et al. A vertebrate Polycomb response element governs segmentation of the posterior hindbrain. Cell 2009; 138:885 - 97; http://dx.doi.org/10.1016/j.cell.2009.08.020; PMID: 19737517
- Luo L, Gassman KL, Petell LM, Wilson CL, Bewersdorf J, Shopland LS. The nuclear periphery of embryonic stem cells is a transcriptionally permissive and repressive compartment. J Cell Sci 2009; 122:3729 - 37; http://dx.doi.org/10.1242/jcs.052555; PMID: 19773359
- Ezhkova E, Pasolli HA, Parker JS, Stokes N, Su IH, Hannon G, et al. Ezh2 orchestrates gene expression for the stepwise differentiation of tissue-specific stem cells. Cell 2009; 136:1122 - 35; http://dx.doi.org/10.1016/j.cell.2008.12.043; PMID: 19303854
- Bracken AP, Dietrich N, Pasini D, Hansen KH, Helin K. Genome-wide mapping of Polycomb target genes unravels their roles in cell fate transitions. Genes Dev 2006; 20:1123 - 36; http://dx.doi.org/10.1101/gad.381706; PMID: 16618801
- Boyer LA, Plath K, Zeitlinger J, Brambrink T, Medeiros LA, Lee TI, et al. Polycomb complexes repress developmental regulators in murine embryonic stem cells. Nature 2006; 441:349 - 53; http://dx.doi.org/10.1038/nature04733; PMID: 16625203
- Palacios D, Mozzetta C, Consalvi S, Caretti G, Saccone V, Proserpio V, et al. TNF/p38α/polycomb signaling to Pax7 locus in satellite cells links inflammation to the epigenetic control of muscle regeneration. Cell Stem Cell 2010; 7:455 - 69; http://dx.doi.org/10.1016/j.stem.2010.08.013; PMID: 20887952
- Caretti G, Di Padova M, Micales B, Lyons GE, Sartorelli V. The Polycomb Ezh2 methyltransferase regulates muscle gene expression and skeletal muscle differentiation. Genes Dev 2004; 18:2627 - 38; http://dx.doi.org/10.1101/gad.1241904; PMID: 15520282
- Rajapakse I, Groudine M. On emerging nuclear order. J Cell Biol 2011; 192:711 - 21; http://dx.doi.org/10.1083/jcb.201010129; PMID: 21383074
- Meister P, Mango SE, Gasser SM. Locking the genome: nuclear organization and cell fate. Curr Opin Genet Dev 2011; 21:167 - 74; http://dx.doi.org/10.1016/j.gde.2011.01.023; PMID: 21345665
- Peric-Hupkes D, Meuleman W, Pagie L, Bruggeman SW, Solovei I, Brugman W, et al. Molecular maps of the reorganization of genome-nuclear lamina interactions during differentiation. Mol Cell 2010; 38:603 - 13; http://dx.doi.org/10.1016/j.molcel.2010.03.016; PMID: 20513434
- Eskiw CH, Cope NF, Clay I, Schoenfelder S, Nagano T, Fraser P. Transcription factories and nuclear organization of the genome. Cold Spring Harb Symp Quant Biol 2010; 75:501 - 6; http://dx.doi.org/10.1101/sqb.2010.75.046; PMID: 21467135
- Zhao R, Bodnar MS, Spector DL. Nuclear neighborhoods and gene expression. Curr Opin Genet Dev 2009; 19:172 - 9; http://dx.doi.org/10.1016/j.gde.2009.02.007; PMID: 19339170
- Towbin BD, Meister P, Gasser SM. The nuclear envelope--a scaffold for silencing?. Curr Opin Genet Dev 2009; 19:180 - 6; http://dx.doi.org/10.1016/j.gde.2009.01.006; PMID: 19303765
- Misteli T. Beyond the sequence: cellular organization of genome function. Cell 2007; 128:787 - 800; http://dx.doi.org/10.1016/j.cell.2007.01.028; PMID: 17320514
- Lanctôt C, Cheutin T, Cremer M, Cavalli G, Cremer T. Dynamic genome architecture in the nuclear space: regulation of gene expression in three dimensions. Nat Rev Genet 2007; 8:104 - 15; http://dx.doi.org/10.1038/nrg2041; PMID: 17230197
- Fraser P, Bickmore W. Nuclear organization of the genome and the potential for gene regulation. Nature 2007; 447:413 - 7; http://dx.doi.org/10.1038/nature05916; PMID: 17522674
- Wang J, Kumar RM, Biggs VJ, Lee H, Chen Y, Kagey MH, et al. The Msx1 Homeoprotein Recruits Polycomb to the Nuclear Periphery during Development. Dev Cell 2011; 21:575 - 88; http://dx.doi.org/10.1016/j.devcel.2011.07.003; PMID: 21852201
- Carroll JS, Meyer CA, Song J, Li W, Geistlinger TR, Eeckhoute J, et al. Genome-wide analysis of estrogen receptor binding sites. Nat Genet 2006; 38:1289 - 97; http://dx.doi.org/10.1038/ng1901; PMID: 17013392
- Buchberger A, Freitag D, Arnold HH. A homeo-paired domain-binding motif directs Myf5 expression in progenitor cells of limb muscle. Development 2007; 134:1171 - 80; http://dx.doi.org/10.1242/dev.02798; PMID: 17301086
- Hadchouel J, Carvajal JJ, Daubas P, Bajard L, Chang T, Rocancourt D, et al. Analysis of a key regulatory region upstream of the Myf5 gene reveals multiple phases of myogenesis, orchestrated at each site by a combination of elements dispersed throughout the locus. Development 2003; 130:3415 - 26; http://dx.doi.org/10.1242/dev.00552; PMID: 12810589
- Bendall AJ, Rincón-Limas DE, Botas J, Abate-Shen C. Protein complex formation between Msx1 and Lhx2 homeoproteins is incompatible with DNA binding activity. Differentiation 1998; 63:151 - 7; http://dx.doi.org/10.1046/j.1432-0436.1998.6330151.x; PMID: 9697309
- Song YJ, Lee H. YB1/p32, a nuclear Y-box binding protein 1, is a novel regulator of myoblast differentiation that interacts with Msx1 homeoprotein. Exp Cell Res 2010; 316:517 - 29; http://dx.doi.org/10.1016/j.yexcr.2009.12.003; PMID: 20004191
- Song YJ, Lee H. PIAS1 negatively regulates ubiquitination of Msx1 homeoprotein independent of its SUMO ligase activity. Mol Cells 2011; 32:221 - 6; http://dx.doi.org/10.1007/s10059-011-1020-8; PMID: 21717107
- Zhang H, Catron KM, Abate-Shen C. A role for the Msx-1 homeodomain in transcriptional regulation: residues in the N-terminal arm mediate TATA binding protein interaction and transcriptional repression. Proc Natl Acad Sci U S A 1996; 93:1764 - 9; http://dx.doi.org/10.1073/pnas.93.5.1764; PMID: 8700832
- Zhang H, Hu G, Wang H, Sciavolino P, Iler N, Shen MM, et al. Heterodimerization of Msx and Dlx homeoproteins results in functional antagonism. Mol Cell Biol 1997; 17:2920 - 32; PMID: 9111364
- Margueron R, Reinberg D. Chromatin structure and the inheritance of epigenetic information. Nat Rev Genet 2010; 11:285 - 96; http://dx.doi.org/10.1038/nrg2752; PMID: 20300089
- Singh K, Dilworth FJ. Redirecting traffic in the nucleus. Dev Cell 2011; 21:390 - 2; http://dx.doi.org/10.1016/j.devcel.2011.08.025; PMID: 21920308
- Guelen L, Pagie L, Brasset E, Meuleman W, Faza MB, Talhout W, et al. Domain organization of human chromosomes revealed by mapping of nuclear lamina interactions. Nature 2008; 453:948 - 51; http://dx.doi.org/10.1038/nature06947; PMID: 18463634
- Yao J, Fetter RD, Hu P, Betzig E, Tjian R. Subnuclear segregation of genes and core promoter factors in myogenesis. Genes Dev 2011; 25:569 - 80; http://dx.doi.org/10.1101/gad.2021411; PMID: 21357673