Abstract
When living egg chambers of Drosophila are isolated in a saline solution and gently squashed between a microscope slide and coverslip, prominent nuclear bodies (1 - 20 mm diameter) can be seen inside the oocyte nucleus or germinal vesicle (GV). These bodies do not pre-exist within the GV and are not seen in material that is fixed in paraformaldehyde before squashing. Instead, they form spontaneously within minutes after an egg chamber is damaged and the cytoplasm is exposed to the isolation medium. Electron microscopy shows that the bodies lack an investing membrane and consist of closely packed, irregular particles 30-50 nm in diameter. We used GFP-tagged proteins from the Carnegie Protein Trap Library to identify 22 proteins that are either enriched in the bodies or excluded from them. We were unable to discern common features of proteins that are concentrated in the bodies, such as isoelectric point, molecular weight, or biological process. Induced bodies are formed in GVs of flies that are null for coilin or WDR79, proteins that are required for formation of Cajal bodies (CBs). We performed fluorescence recovery after photobleaching (FRAP) experiments on five GFP-tagged proteins that are enriched in the bodies. Four of the proteins regained the full pre-bleach fluorescence intensity, indicating that the contents of the bodies are in dynamic equilibrium with the surrounding nucleoplasm. Induced nuclear bodies presumably form as a result of unusual physico-chemical changes in the Drosophila GV. We suggest that their behavior serves as a useful model for self-assembly of nuclear bodies in general, and we discuss the possibility that similar bodies may occur normally in cells of other organisms.
Introduction
In addition to the chromosomes nuclei contain a variety of organelles referred to loosely as nuclear bodies, among which are nucleoli, Cajal bodies, histone locus bodies, speckles, paraspeckles, PML bodies and nuclear stress bodies.Citation1 These bodies differ markedly with respect to size, composition, relationship to the chromosomes and association with cell type or physiological state of the cell. Nevertheless, they share certain general characteristics, especially the lack of a bounding membrane and rapid exchange of their component macromolecules between the body itself and the surrounding nucleoplasm.
The biogenesis of nuclear bodies has received considerable attention in recent years. Two broad categories are generally recognized—hierarchical assembly and self-organization—the first requiring a nucleating molecule or structure about which the body is formed, the second requiring only that the component molecules reach a critical concentration before they aggregate spontaneously. The most intensively studied body in the first category is the nucleolus, which forms by ordered recruitment of the RNA polymerase I machinery to the ribosomal DNA locus.Citation2–Citation4 In contrast, Cajal bodies can form when only one of their component molecules occurs at a locally high concentration.Citation5 In this case there seems to be no order in which the components must assemble and there is no specific gene locus for nucleation. Still a third category of assembly has been suggested for the histone locus body, which may require an RNA transcript for initial nucleation, but processing molecules later aggregate to the RNA more or less independently.Citation6–Citation8
Here we describe the rapid induction of nuclear bodies in the Drosophila GV by slight damage to the cell, exposing its contents to a simple salt solution. These induced nuclear bodies share several properties with normally occurring nuclear bodies, including lack of an investing membrane, simple ultrastructure, specific macromolecular composition and rapid exchange of components between the body and the surrounding nucleoplasm. Thus these bodies display the defining characteristics of a self-assembling body and provide a convenient experimental model for further analysis.
Results
Induced nuclear body formation.
In a typical experiment the ovaries from a well-fed adult female Drosophila melanogaster were isolated in an isotonic saline solution (OR2). One or more stage-10 egg chambers were dissected and placed on a standard microscope slide in a small drop of the same solution, and a coverslip was added. The preparation was then observed by phase contrast or differential interference microscopy (DIC). In an intact egg chamber the GV appears as a more or less homogeneous sphere within which one can see a small mass of condensed chromatin, the karyosome (). If the pressure of the coverslip is sufficient to break the egg chamber and expose the oocyte and nurse cells to the medium, dramatic changes occur within the GV. Within 3–5 minutes one sees the formation of previously undetectable bodies, often at one side of the GV ( and Video S1). When first detectable the bodies are roughly 1–3 µm in diameter, but they soon fuse into irregularly-shaped structures that eventually equilibrate into one or more large spheres, up to 20 µm in diameter. The process is quite variable from one GV to the next, both in the number of initial bodies, the patterns of fusion and the final sizes of the bodies. Typically, the bodies reach a state of equilibrium 10 to 12 min after the initial squash. Body formation depends on exposure of the egg chamber contents to the isolation medium, not to squashing as such. Thus, when one pokes a small hole in an isolated egg chamber with a fine needle without squashing under a coverslip, the bodies still form.
We observed formation of induced bodies in egg chambers of different stages, from as early as stage 4–6 until GV breakdown during stage 12 (). At the earlier stages we see only a single body, which may be closely associated with the karyosome (, stage 7–8). Multiple bodies are induced in later stages and there is no special association with the karyosome. Bodies never form in nurse cell nuclei, regardless of the stage of the egg chamber. We often see rodshaped “needles” in both the GV and the nurse cell nuclei (, stage 11). These needles are a normal feature of the nuclei and are present whether or not the cell contents are exposed to the external medium.
In younger egg chambers, up to about stage 8, the GV normally contains a prominent nuclear body attached to the karyosome (, stage 7–8). Because of its composition, particularly the presence of coilin and small nuclear ribonucleoproteins (snRNPs), we have called this structure a Cajal body (CB).Citation9 By stage 10, when most of our observations on induced bodies were made, the CB has fragmented or disappeared entirely. To determine whether the induced nuclear bodies are related to CBs, we looked for the presence of coilin. We broke open stage 10 egg chambers in OR2 medium, waited 10 min for nuclear bodies to form, fixed and immunolabeled the tissue with an antibody against Drosophila coilin. Fluorescence was not seen in the bodies, although there was weak diffuse fluorescence in the nucleoplasm. Because these experiments were carried out on stage 10 egg chambers, no endogenous CBs were present. We also looked at transgenic flies that expressed EYFP-coilin (tag on the N-terminus). Coilin tends to be overexpressed in the transgenic flies, although CBs are easily recognizable.Citation9 One can see fluorescent CBs in egg chambers up to about stage 8, but generally there are none at stage 10. Nuclear bodies were induced as expected in the transgenic flies, but EYFP-tagged coilin was excluded from them (). Fluorescent coilin was visible in the nucleoplasm and presumably would have concentrated in the induced bodies if they were CBs. Experiments were also conducted on two mutant strains that lack CBs entirely, coil199 and WDR79MB10832. Oocytes from both strains formed induced nuclear bodies identical to those seen in wild type flies. Based on the absence of coilin from the bodies when formed in wild type flies and on the formation of bodies in strains that are genetically deficient for CBs, we conclude that the induced bodies are not related to CBs.
We examined induced nuclear bodies by electron microscopy (). Because it was not feasible to squash egg chambers under a coverslip and then prepare them for electron microscopy, we used a fine tungsten needle to make a small hole in the egg chamber and expose the oocyte to the external medium. Under these conditions, nuclear bodies formed as usual within a few minutes. However, the GV was not round and the nuclear envelope was somewhat distorted in such preparations. Nevertheless, the induced bodies were easily recognizable because of their overall large size, their shape and the presence of internal vacuoles (). In addition one can recognize the karyosome and the needle-like structures visualized in the DIC images. At higher magnification the nuclear bodies are seen to consist of indistinct granules, approximately 30–50 nm in diameter, of higher contrast than the background nucleoplasm (). Of particular note is the absence of a surrounding membrane, a feature shared with normally occurring nuclear bodies.
Factors that prevent induced bodies from forming.
Our initial experiments were carried out in OR2 medium, a traditional Ringer's solution developed for use with amphibian oocytes.Citation10 We obtained similar results with a solution consisting only of 0.15 M NaCl. However, nuclear bodies were not induced when we squashed stage-10 egg chambers in Grace's Medium,Citation11 a complex nutritive medium originally designed for insect tissue culture (). Because Grace's medium contains relatively high concentrations of both Ca++ (9 mM) and Mg++ (22 mM), we added these ions separately to 0.15 mM NaCl and tested for the formation of nuclear bodies. Bodies failed to form when either ion was present at 10 mM or higher, suggesting that ionic conditions are critical (). To test whether the induction of bodies requires ongoing oxidative metabolism, we added NaN3, which inhibits energy-dependent cell functioning by blocking cell respiration. The induced bodies still formed in egg chambers that were squashed in 0.02% NaN3 in OR2 medium. Because formation of the bodies is sensitive to the composition of the medium, but occurs in the absence of cell respiration, we believe that their formation is a purely physical-chemical phenomenon unrelated to major physiological processes.
Protein composition of the induced bodies.
We used the Carnegie Protein Trap LibraryCitation12 to screen for proteins that are found in the induced bodies. In this collection, enhanced green fluorescent protein (EGFP) exons have been inserted into endogenous genes. Therefore, these fly lines permit one to see the expression patterns of various proteins under control of their normal promoters. There is considerable variation in the absolute strength of expression in the GV. We were primarily interested in the relative concentration in the bodies compared to the nucleoplasmic background ( and Table S1). A number of fusion proteins preferentially accumulate in the induced bodies, including: nucleoplasmin (Nlp, nuclear chaperone), Spt6 (transcription elongation factor), posterior sex combs (Psc, polycomb group protein), Dek, topoisomerase 1 (Top1), lamin C (LamC), mapmodulin (binds microtubule associated proteins), strawberry notch (sno, notch signaling pathway), Pellino (Pli, Toll signaling pathway), and telomere fusion (tefu, telomere protection). Some proteins enriched in the induced bodies are also found in the nucleoplasm, such as nucleoplasmin. Others, such as topoisomerase 1, are predominantly found in the induced bodies. The telomere fusion protein is found in foci within the induced bodies. We also sometimes see small granules that contain topoisomerase I, but these are typically on the external periphery of the bodies. We also identified proteins that are excluded from the bodies. These include: poly (A) binding protein (Pabp2, polyadenylation of mRNAs), darkener of apricot (Doa, LAMMER kinase), apontic (apt, transcription factor), Zn72D (splicing), Mi-2 (ATPase), modifier of mdg4 (mod(mdg4), chromatin insulator), decondensation factor 31 (Df31, chromatin structure), SNF4Aγ (protein kinase), pointed (pnt) and kismet (kis, trithorax group protein).
Although pABP2 does not accumulate in the induced bodies, it is enriched in the needle-like structures mentioned earlier (). Poly (A) binding protein nuclear 1 (PABPN1) was previously shown to be enriched in rod-shaped inclusions in neurons from the rat supraoptic nucleus.Citation13
A list of proteins localized in the induced bodies and the nucleoplasm is given in Supplemental Table 1. We also list the isoelectric points and molecular weights of these proteins. We found no obvious correlation between the localization of the protein and either of these properties. We also failed to discern any pattern based on the known properties of these proteins or the metabolic pathways with which they are associated.
Dynamics of induced bodies.
To study the dynamics of proteins within the induced bodies, we performed fluorescence recovery after photobleaching (FRAP) experiments, using five protein trap lines that were expressed at a high level in the bodies (Top1-GFP, Dek-GFP, Mapmodulin-GFP, Psc-GFP and tefu-GFP). Stage-10 egg chambers were gently squashed in OR2 + DAPI and held for 10–15 minutes to permit the bodies to form. Single bodies within the GV were bleached rapidly (about 20–25 s) using a laser scanning confocal microscope, and the recovery of fluorescence was followed as a function of time. Correction was made for bleaching due to imaging. Thirty-two separate FRAP experiments were carried out (Table S2); the results of a single experiment with Top1-GFP are shown in and B. In this experiment the bleached body regained its original fluorescence intensity with a half-time of 45 sec. In a total of 7 experiments the mean recovery time for Top1-GFP was 28 sec with a range of 13–56 sec. Top1-GFP recovered more slowly than Dek-GFP, Mapmodulin-GFP and Psc-GFP, but faster than tefu-GFP. Recovery was incomplete in the case of tefu-GFP. The immobile fraction was 48% and consisted of foci within the induced bodies that did not recover after bleaching. Overall, the FRAP data reveal that proteins in the induced bodies exchange dynamically with proteins in the nucleoplasm, much like proteins in biologically significant nuclear compartments.
Discussion
Interphase nuclei typically exhibit a variety of nuclear bodies, loosely defined as any morphologically distinct region of the nucleus that is not clearly a chromosome or part of a chromosome (such as heterochromatic masses). Even this broad definition runs into a problem with the nucleolus, which is often considered a nuclear body, but is clearly a hypertrophied gene locus and therefore part of a chromosome. The list of described nuclear bodies is long, including Cajal bodies, speckles, histone locus bodies, paraspecles, PML bodies, the perinucleolar compartment, nuclear stress bodies and various “orphan” bodies.Citation1 Although each type of nuclear body can usually be recognized on the basis of its unique composition, they all share a few general characteristics, among which are lack of a bounding membrane, multiple specific molecular components (both RNA and proteins), and rapid exchange of these components with the nucleoplasm. Thus, despite their morphological stability over periods of minutes or hours, nuclear bodies are dynamic structures.
The induced bodies we describe here lack a bounding membrane, contain multiple specific proteins, and are dynamic in the sense that at least some of their components are in rapid equilibrium with the nucleoplasm. Their formation in the presence of azide demonstrates their independence from ongoing cellular metabolism, and suggests that formation of naturally occurring nuclear bodies might at times be primarily dependent on physico-chemical rather than metabolic factors.
In several respects the induced bodies most closely resemble the nucleolus-like bodies (NLBs) found in the pronuclei and early cleavage nuclei of mammals (). NLBs appear in both the male and female pronuclei shortly after fertilization, often as small bodies that coalesce into one or a few large spherical structures.Citation14 They disappear during the first cleavage and reappear in the nuclei of the two-celled embryo. E.M. observations show that NLBs lack an investing membrane and have a more or less homogeneous fine structure similar to that described here for the induced bodies.Citation15 Strikingly, NLBs lack typical nucleolar and CB components, underscoring the fact that they are not nucleoli in the usual sense nor are they clearly related to CBs. Thus, there are similarities between the induced bodies in Drosophila oocytes and NLBs in cleavage stage mammalian embryos: rapid formation, coalescence into larger structures, large size relative to the size of the nucleus, lack of a surrounding membrane, homogenous fine structure at the E.M. level and lack of obvious homology to well-characterized nuclear bodies. They differ in one respect: nucleoplasmin is excluded from the NLBs,Citation16 whereas it is enriched in the Drosophila bodies. It may well be that both cases illustrate an inherent tendency of complex protein solutions to segregate into different phases when subjected to change in ionic conditions. In this case, neither the induced bodies nor NLBs would necessarily represent a nuclear organelle in the sense of a biologically determined structure with specific functions relevant to cell physiology. Alternatively, one could argue that the induced bodies represent a stress response on the part of the oocyte to the unusual ionic conditions to which it has been exposed. This hypothesis would not be easy to test in the Drosophila system, because the oocytes and egg chambers cannot be maintained in a lifelike condition for more than about 1 h after formation of the nuclear bodies.
Materials and Methods
Fly stocks.
Drosophila melanogaster strains were maintained at 21–23°C on a standard cornmeal-based medium. Adult female flies were fed on wet yeast for 2–4 days before use. Stocks used include y w, EYFP-coilin,Citation9coil199,Citation9 and WDR79MB10832 (Bloomington Stock Center).
Induction of nuclear bodies.
Egg chambers were dissected from well-fed female flies in OR2 (82.5 mM NaCl, 2.5 mM KCl, 1 mM CaCl2, 1 mM MgCl2, 1 mM Na2HPO4, 5 mM HEPES, pH 8.0),Citation10 + 1 µg/mL DAPI (4′,6 diamidino-2-phenylindole). The egg chambers were gently squashed between a coverslip and a slide, and the edge of the coverslip was sealed with nail polish. GVs were immediately visualized by DIC or fluorescence microscopy. In most cases, observations were made on stage-9 to stage-11 egg chambers.
Electron microscopy.
Oocytes from stage-10 egg chambers were gently punctured with Dumont #5 forceps or with a tungsten needle. The egg chambers remained in OR2 for about 10 minutes. They were then fixed in 3% glutaraldehyde, 1% formaldehyde, 2 mM Ca++ in 0.1 M cacodylate buffer, pH 7.4, embedded in agarose and contrasted with 1% OsO4 + 1% K3Fe(CN)6 for 1 hr., followed by 0.5% UO2(C2H3O2)2 for 1 hr. After dehydration in a graded ethanol series the agarose block was embedded in Epon 812 resin. Sections of approximately 85 nm thickness were cut with a Leica Ultracut S microtome, stained with Reynold's lead citrate for 3 min and imaged with a Tecnai 12 electron microscope (FEI) and Gatan 794 camera.
Immunolabeling.
Oocytes were punctured with a needle or forceps in OR2 and remained in the medium for another 10 min. They were then fixed in 4% paraformaldehyde in OR2, washed in phosphate buffered saline (PBS) and treated with antibody. Washing and antibody solutions contained 0.3% Triton X-100. Egg chambers were blocked in 10% horse serum in PBS + 0.02% sodium azide for at least ½ hr. They were then labeled with primary antibody overnight, washed with PBS and stained with secondary antibody 4 hr or more. DAPI was also included at a concentration of 1.0 µg/mL to stain DNA. Tissues were rinsed with PBS + Triton X-100 and then mounted in 50% glycerol + 1 mg/ml phenylenediamine under a 22-mm2 coverslip. The coverslip was sealed to the slide with nail polish. Slides were stored for up to a month at −20°C.
Microscopy.
All DIC and fluorescent images were taken with an Olympus BX61 microscope with a 40x, 1.30 N.A. or a 100x 1.40 N.A. objective and a digital CCD camera (Hammamatsu C4742-95). Image contrast and brightness were modified in Photoshop. Confocal images were taken with a Leica SP5 laser scanning confocal microscope.
Antibodies.
Two antibodies were used: guinea pig polyclonal GP3T against Drosophila coilinCitation9 and rabbit polyclonal against GFP (Torrey Pines).
Fluorescence recovery after photobeaching (FRAP).
FRAP analysis was made on egg chambers from several protein trap lines in which a specific protein was labeled with GFP.Citation12 Stage-10 egg chambers were dissected in OR2 + DAPI (1 µg/mL), gently squashed between a coverslip and slide, and the coverslip was sealed with nail polish. Approximately 10–15 min after squashing, a single induced nuclear body was bleached with the 488 nm argon laser (80% intensity for 20–25 sec.) of the Leica SP5 confocal microscope. The entire GV was imaged before and after bleaching with a 40x, 1.25 NA oil objective. Intensity data were collected by the SP5 microscope and subsequently analyzed with the Kaleida Graph program (Synergy Software). Mean intensities were determined for the bleached body, an unbleached body in the same GV, and an area in the background outside of the GV. Mean intensities were corrected by subtracting the intensity of a background area of equal size.
Disclosure of Potential Conflict of Interest
No potential conflicts of interest were disclosed.
Abbreviations
CB | = | Cajal body |
GV | = | germinal vesicle |
NLB | = | nucleolus-like body |
Figures and Tables
Figure 1 Individual frames from a video of a stage-10 GV of Drosophila, showing gradual formation of induced nuclear bodies. The first frame (t = 0) was taken approximately five minutes after the egg chamber had been gently squashed between a glass slide and coverslip. In this GV the first bodies to form were numerous and irregular in outline, but eventually most coalesced into three large bodies. Arrow points to the karyosome, which remained unchanged. Imaged by DIC. Bar = 10 µm. A video of another GV can be watched in the Supplemental Material.
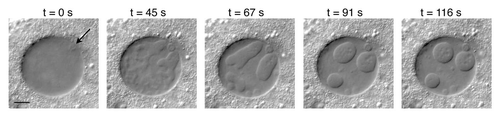
Figure 2 Induced nuclear bodies in GVs from egg chambers of increasing size. In general, smaller GVs form only one or two bodies, whereas the largest GVs often display 15–20 bodies. Stage 7–8: a Cajal body (CB) is normally found in GVs up to about stage 8, at which time it disappears. The induced nuclear body (NB) in this stage 7–8 GV is independent of the CB, but is closely associated with the karyosome (K). Stage 9: nuclear bodies may contain vacuoles of trapped nucleoplasm, especially evident in this stage 9 GV. Stage 10: Multiple nuclear bodies are common in larger GVs. The karyosome (K) is in focus in this image. Stage 11: The needle-like inclusion (N) seen here occurs regularly in GVs and nurse cell nuclei of untreated oocytes. Bar = 10 µm.
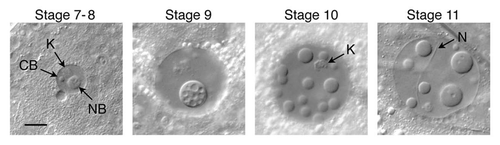
Figure 3 Induced nuclear bodies in a GV from a transgenic line expressing EYFP-coilin. (A) DIC image. (B) Fluorescence image. The exclusion of EYFP-coilin from the bodies suggests that they are unrelated to CBs. Bar = 10 µm.
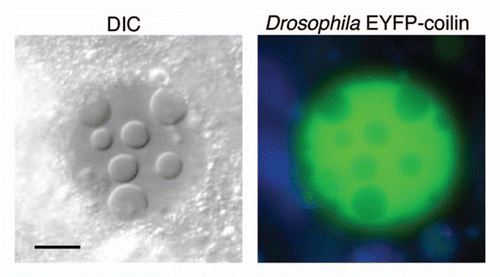
Figure 4 (A) Electron micrograph of an induced nuclear body in a GV from a stage-10 oocyte. The internal vacuoles in the nuclear body appear to be pools of trapped nucleoplasm. Bar = 5 µm. (B) Higher magnification of part of another nucleus shows that the body contains irregular electron-dense particles with diameters in the range of 30–50 nm, suspended in material indistinguishable from the nucleoplasm. Note the clearly defined membranes of the nuclear envelope (NE) but the absence of a membrane surrounding the nuclear body. Bar = 0.5 µm.
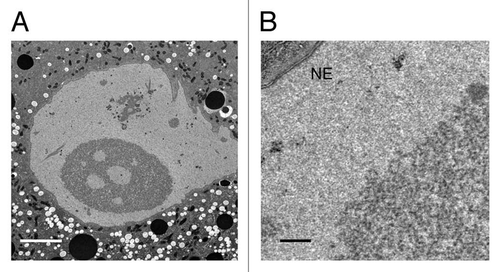
Figure 5 (A) GVs exposed to a solution containing only NaCl develop nuclear bodies. (B) Nuclear bodies do not form when GVs are exposed to Grace's insect culture medium. (C) Bodies fail to form when the isolation medium contains MgCl2 in the 10–30 mM range. The same is true for CaCl2. Bar = 10 µm.
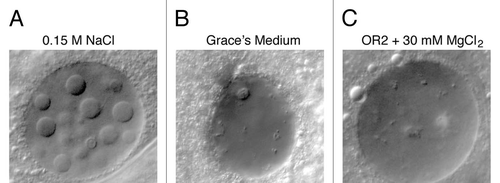
Figure 6 Nuclear bodies were induced in lines from the Carnegie Protein Trap Library.Citation12 In each case, a specific protein is labeled with GFP, making it possible to determine whether that protein is included or excluded from the body. For protein abbreviations see the text and Flybase (http://flybase.org/). Twenty-two protein trap lines were examined, as shown in Table S1. Bar = 10 µm.
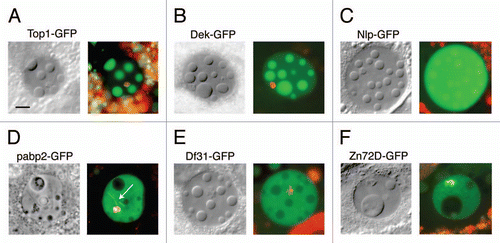
Figure 7 FRAP analysis of GFP-labeled topoisomerase 1, a protein that is strongly localized in the induced nuclear bodies. (A) The first panel shows a GV that contains several large nuclear bodies. The largest of these (circle) was bleached at t = 0 s. Recovery from bleaching was rapid, as shown in images taken at 6.8 s, 101 s and 276 s. (B) Graph of the average intensity of the body throughout the experiment, normalized to a pre-bleach intensity of 1.0. The half time for recovery was 45 s and full recovery was reached at about 200 s. Bar = 10 µm.
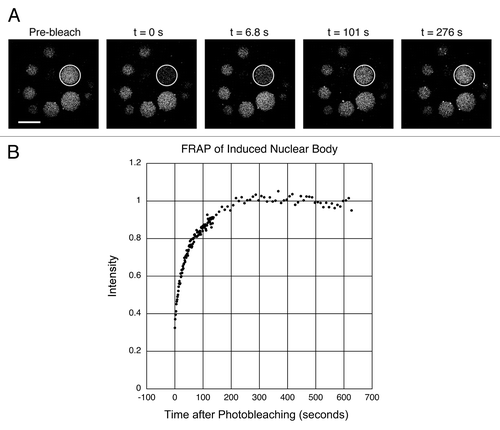
Figure 8 DIC image of a living mouse egg shortly before fusion of the two pronuclei. In this focal plane the female pronucleus displays a single large nuclear body, whereas the slightly larger male pronucleus shows one small and two large bodies. The polar body nucleus also appears to contain a small nuclear body. Note the superficial similarity to the induced nuclear bodies of Drosophila.
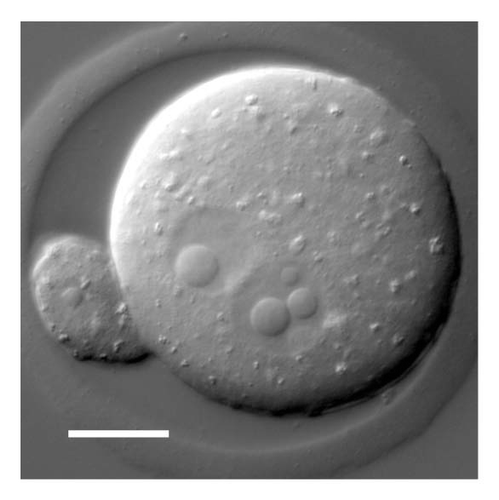
Acknowledgements
We thank Michael Sepanski for help with the electron microscopy and Katie McDole for providing mouse embryos. Supported in part by National Institutes of Health grant GM 33,397 to J.G.G. J.G.G. is American Cancer Society Professor of Developmental Genetics.
References
- Misteli T, Spector DL. The Nucleus 2011; Cold Spring Harbor, NY Cold Spring Harbor Laboratory Press
- Dundr M, Misteli T, Olson MO. The dynamics of postmitotic reassembly of the nucleolus. J Cell Biol 2000; 150:433 - 446
- Hernandez-Verdun D. The nucleolus: a model for the organization of nuclear functions. Histochem Cell Biol 2006; 126:135 - 148
- Savino TM, Gebrane-Younes J, De Mey J, Sibarita JB, Hernandez-Verdun D. Nucleolar assembly of the rRNA processing machinery in living cells. J Cell Biol 2001; 153:1097 - 1110
- Kaiser TE, Intine RV, Dundr M. De novo formation of a subnuclear body. Science 2008; 322:1713 - 1717
- Shevtsov SP, Dundr M. Nucleation of nuclear bodies by RNA. Nat Cell Biol 2011; 13:167 - 173
- White AE, Burch BD, Yang XC, Gasdaska PY, Dominski Z, Marzluff WF, et al. Drosophila histone locus bodies form by hierarchical recruitment of components. J Cell Biol 2011; 193:677 - 694
- Dundr M. Seed and grow: a two-step model for nuclear body biogenesis. J Cell Biol 2011; 193:605 - 606
- Liu JL, Wu Z, Nizami Z, Deryusheva S, Rajendra TK, Gao H, et al. Coilin is essential for Cajal body organization in Drosophila melanogaster. Mol Biol Cell 2009; 20:1661 - 1670
- Wallace RA, Jared DW, Dumont JN, Sega MW. Protein incorporation by isolated amphibian oocytes: III. Optimum incubation conditions. J Exp Zool 1973; 184:321 - 333
- Grace TD. Establishment of four strains of cells from insect tissues grown in vitro. Nature 1962; 195:788 - 789
- Buszczak M, Paterno S, Lighthouse D, Bachman J, Planck J, Owen S, et al. The Carnegie Protein Trap Library: A Versatile Tool for Drosophila Developmental Studies. Genetics 2007; 175:1505 - 1531
- Villagra NT, Bengoechea R, Vaque JP, Llorca J, Berciano MT, Lafarga M. Nuclear compartmentalization and dynamics of the poly(A)-binding protein nuclear 1 (PABPN1) inclusions in supraoptic neurons under physiological and osmotic stress conditions. Mol Cell Neurosci 2008; 37:622 - 633
- Austin CR. The formation, growth and conjugation of the pronuclei in the rat egg. J Roy Micr Soc 1951; 71:295 - 306
- Ferreira J, Carmo-Fonseca M. The biogenesis of the coiled body during early mouse development. Development 1995; 121:601 - 612
- Burns KH, Viveiros MM, Ren Y, Wang P, DeMayo FJ, Frail DE, et al. Roles of NPM2 in Chromatin and Nucleolar Organization in Oocytes and Embryos. Science 2003; 300:633 - 636