Abstract
The nucleolus is organized around a scaffolding of rDNA tandem repeats. These repeats, known as ribosomal cassettes, are each composed of ribosomal RNA (rRNA) genes preceding a long intergenic spacer (IGS) that has been classically perceived to be transcriptionally silent. Recent study of the IGS has contradicted the dogma that these spacers are merely inert regions of the genome, instead suggesting they are biologically significant, complex and plurifunctional transcriptional units that appear central to proper cellular functioning. Through the timely induction of various ribosomal IGS noncoding RNA (IGS RNA) transcripts, the cell is capable of both regulating rRNA synthesis and sequestering large numbers of proteins, thereby modulating essential molecular networks. Here we discuss our current understanding of the organization and function of the IGS.
The human genome contains approximately 400 copies of ribosomal DNA (rDNA), found in tandem on the short arms of all five acrocentric chromosomes (13, 14, 15, 21, and 22). Actively transcribed rRNA cassettes are consolidated within the nucleolus creating “ribosome factories” that account for the production of the majority of total cellular RNA. In mammals, nucleoli are highly dynamic structures that assemble and disassemble during each mitotic cycle. Reformation occurs during interphase, where all molecules necessary for its basic structure and functional organization are recruited.Citation1-Citation4 Under proliferative conditions the most commonly ascribed function of the nucleolus is the transcription, processing and modification of the rRNAs as they are assembled into ribosomal subunits.
rDNA is transcribed by RNA polymerase I (PolI) as a single 13.3kb polycistronic transcript comprising sequentially the 5′-external transcribed spacer (ETS), 18S rRNA, internal transcribed spacer (ITS) 1, 5.8S rRNA, ITS2, 28S rRNA and 3′-ETS (). The resulting pre-rRNA, known as 47S, is matured via a series of processing and chemical modification steps that include cleavage, pseudouridylation and methylation at selected residues.Citation5 Ultimately, three distinct rRNA species (18S, 5.8S, 28S) are produced and assembled with proteins into pre-ribosomal subunits that take part in protein synthesis in the cytoplasm. As is the case with most metabolically important genes, transcription of rDNA can be modulated in response to a variety of cellular and pathological stimuli including amino acid starvation, aging, viral infection or toxic lesions.Citation6,Citation7 This modulation is accomplished by a complex series of enhancers, repressors and transcription factors that interact with PolI or its DNA regulatory elements. Though it can be initiated and interrupted, PolI-dependent transcription of rRNA genes is usually considered a constitutive process in metabolically active cells, in contrast to many RNA Polymerase II-regulated transcripts that are rapidly induced as needed.
Figure 1. Synthesis of rRNA from the ribosomal cassette. The human ribosomal cassette is composed of a ~13kb transcribed region followed by a ~30 kb intergenic spacer. RNA polymerase I is recruited to a promoter region, upstream of the ribosomal gene and transcribes a single polycistronic transcript that is then processed by either of two pathways into the 18S, 5.8S and 28S rRNAs.
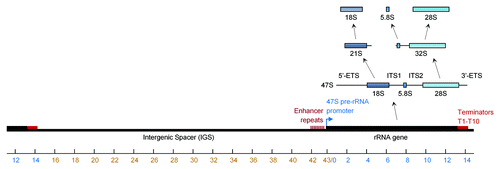
The plurifunctional nature of the nucleolus is evident in its response to physiological stimuli and stress conditions, such as anaerobic metabolism, heat shock or transcriptional stress. These conditions trigger significant structural and functional changes that are important for cellular adaptation and survival. Hallmarks of nucleolar remodeling include a decrease or interruption in rRNA synthesisCitation8,Citation9 as well as the capture of numerous seemingly unrelated factors involved in a wide array of cellular functions.Citation10-Citation13 Though these phenomena have been observed for many years, they have only recently been mechanistically linked to the expression of novel species of noncoding RNA (ncRNA) originating from the ribosomal intergenic spacer (IGS).Citation13,Citation14 In this Commentary, recent and unexpected advances in our understanding of the transcriptional activity and biological role of the IGS are discussed.
The Ribosomal Intergenic Spacer
Human rRNA coding sequences are separated by a ~30 kb region formerly referred to as the non-transcribed spacer,Citation15-Citation17 which until recently had not been attributed any significant function. Early work posited that this region either played a structural role within the nucleolus, allowing for the formation of tightly packed nucleolar organizing regionsCitation18 or provided some form of genomic stabilization through the attachment of specific sequences to the nuclear matrix.Citation19 Evolutionarily, the IGS appears to have increased in size over time, measuring only 2.5 kb in yeasts, 5.1 kb in Drosophilia melanogaster, 5.7 kb in Xenopus laevis, while the chickens, mouse and primate sequences are all approximately 30kb in length.Citation20 At the sequence level the IGS differs considerably from the rRNA coding sequences, notably through its high level of variability both in length and nucleotide composition.Citation16,Citation17,Citation21-Citation23 Disparities in length have been attributed to unequal homologous exchanges,Citation24 while sequence divergences are caused by the incorporation of a large number of retrotransposons, or alu elementsCitation20 and microsatellite variations thought to be caused by slipped-strand mispairing during DNA replication.Citation25
Despite the seemingly disorganized composition and a historical lack of evidence suggesting transcriptional activity, the ribosomal IGS appears to be more functionally relevant than previously believed. Promoter mapping studies of the Xenopus laevi IGS identified spacer promoters that share 90% homology with those of rRNA genes.Citation26,Citation27 These promoters were capable of activating PolI-mediated transcription, though the resulting nascent transcripts were rapidly terminated well upstream of the rRNA sequences by T3 termination sites.Citation28 While similar promoters were also found in mice,Citation29 no known function was conclusively attributed to these transcripts, though a potential role in rRNA transcription activation was speculated.Citation30,Citation31 Recently, several groups have confirmed the existence of a number of ncRNA transcripts originating from these regions of the genome. Functional studies of these molecules have revealed new mechanisms for regulating rRNA expression, as well as a novel post-translation regulatory pathway termed the nucleolar detention pathway.Citation32
IGS Transcript-mediated Regulation of rRNA Synthesis
Analysis of the IGS region 2 kb upstream of the rRNA start site identified a 150–250 nucleotide PolI-mediated transcript, known as the promoter-associated RNA (pRNA). This molecule was shown to be involved in targeting TIP5, the large subunit of the nucleolar remodeling complex (NoRC), to the ribosomal cassettes. Recruitment of the NoRC results in the transcriptionally-repressive histone modifications H3K9me1 and H4K20me3 and in HP1 binding, ultimately inhibiting rRNA synthesis.Citation14,Citation33 Interestingly, the secondary structure of the pRNA is apparently more conserved than its sequence. Though mouse and human pRNA transcripts share less than 50% sequence identity, both possess a highly conserved stem-loop structure. In fact, expression of the human variant can rescue the phenotype of pRNA-depleted mouse cells, demonstrating the significance of the structure.Citation34 The importance of secondary structure within the IGS is particularly striking considering the overall divergence in sequence similarity between mice and humans, as the IGS of these related species share only ~40% sequence similarity.
Transcription of pRNA is driven by a spacer promoter that is 90% homologous to the rRNA promoter yet produces pRNA at a rate 1,000-fold lower.Citation35 The resulting transcript is present at very low levels throughout most of the cell cycle, as it is rapidly degraded by the exosome. However, during mid-S phase pRNA levels transiently increase 2-fold, allowing it to repress rRNA synthesis in late replication.Citation35 Although pRNA originates from a regions closely associated with rRNA, expression of these transcripts appears to be regulated independently,Citation35 establishing pRNA as the first example of a functional ribosomal IGS-derived transcript ().
Figure 2. Novel transcripts of the IGS. (A) The ribosomal intergenic spacer encodes multiple stimuli-specific transcripts. These RNAs are transcribed as precursor molecules that are then processed into a functional transcript capable of interacting with proteins and other cellular elements. (B) IGS22RNA is induced in response to heat shock while IGS28RNA is specific to acidosis.
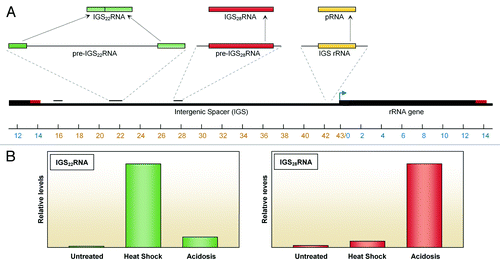
IGS Transcript-mediated Protein Immobilization
In addition to its function as a site of ribosomal biogenesis, the nucleolus is well known for the fundamental role that it plays in regulating molecular networks. In response to a variety of physiological and stress conditions, large numbers of proteins are captured and immobilized by the nucleolar architecture,Citation12,Citation13,Citation36,Citation37 effectively sequestering them away from their binding partners and causing downstream pathways to collapse. Well-documented examples include the von-Hippel Lindau (VHL) tumor suppressor protein in response to anaerobic metabolism,Citation13,Citation36-Citation38 the murine double minute 2 (MDM2) / promyelocytic (PML) protein complex in response to transcriptional stressCitation13,Citation37,Citation39 as well as the heat shock protein 70kDa (Hsp70) in response to heat shock.Citation13,Citation40 These proteins share a consensus amino acid sequence that targets them to the IGS for static detention in the nucleolus, the Nucleolar Detention Signal (NoDS).Citation12,Citation13 This discrete code is composed of an arginine motif (R-R-I/L-X3-r) along with several hydrophobic repeats (L-Φ/N-L/V; where Φ represents any hydrophobic residue).Citation12 Bioinformatic studies suggest that up to 9% of all proteins encode an NoDS and consequently could be regulated by the nucleolus.
The mechanism by which NoDS-containing proteins are captured and immobilized by the nucleolus has recently been elucidated with the identification of novel species of stress-induced ribosomal IGS transcripts. In response to anaerobic metabolism, physiological acidification of the extracellular milieu to cell-specific pH leads to the accumulation of a ~400bp transcript 28kb downstream of the start of the rRNA genes (IGS28RNA) (). Strikingly, this region has recently also been shown to possess prominent epigenetic markers typically linked to transcriptional activation.Citation41 IGS28RNA rapidly recruits and immobilizes NoDS-containing proteins at its site of transcription, thus inactivating them. Silencing of IGS28RNA allows proteins to evade this capture and to retain their dynamic profile under acidotic conditions. The IGS is therefore at the center of a systemic post-translational regulatory pathway that mediates cellular adaption to hypoxic stress.
In what appears to be a homologous pathway, heat shock induces the accumulation of two independent transcripts located at 16kb and 22kb, and referred to as IGS16RNA and IGS22RNA respectively. These RNAs appear to undergo posttranscriptional processing events, notably involving the removal of flanking external and internal spacers and the ligation of two RNA fragments (). This raises the possibility that novel editing pathways are involved and poses the question of the functional significance of post-transcriptional processing of IGS transcripts. In fact, transient overexpression of IGS RNAs has shown that the processed form of IGS22RNA is capable of immobilizing NoDS-containing proteins, whereas its unprocessed counterpart failed to produce the same effect. This suggests that IGS RNA processing is an activatory step that is essential to their function. Additional stresses like DNA damage have been shown to cause the nucleolar sequestration of NoDS-containing proteins.Citation39,Citation42 Given this diversity of stimuli, it is highly probable that the IGS contains additional sites of capture. Though they remain to be identified, novel IGS RNAs comparable to 16, 22 and 28 could be involved.
Concluding Remarks
The findings discussed here demonstrate that the IGS is not an inert region of the genome but in fact a complex and plurifunctional transcriptional unit organized into discrete loci. Some of these loci, located in close proximity to rDNA, serve to control rRNA transcription by recruiting chromatin remodeling complexes. Other loci, located deeper within the IGS, regulate molecular networks by capturing proteins in a stress-specific manner. The possibility that these two systems are related is not implausible. In fact, TIP5, the subunit of the NoRC that interacts with pRNA, contains an NoDS. Likewise, sequestration of proteins at the IGS28 locus has been shown to trigger nucleolar condensation and a decrease in precursor rRNA synthesis.Citation9 The vast number of different proteins that appear to be regulated in trans by the IGS suggests that it is central to a variety of canonical cellular processes. In additional to the bioinformatics data mentioned here, proteins empirically shown to be targets of the IGS include the catalytic subunit of DNA polymerase (POLD1), the DNA methyl transferase 1 (DNMT1), the E3 ubiquitin ligases VHL, MDM2 and RNF8, the anaphase promoting complex 2 (APC2), the 26S proteasome component Sug1 and the chaperone Hsp70, among many others.Citation13 Certainly, the IGS and its transcripts play an unforeseen yet central role in cell biology. Further characterization of the IGS may establish it as one of the cell’s primary regulatory systems.
References
- Mélèse T, Xue Z. The nucleolus: an organelle formed by the act of building a ribosome. Curr Opin Cell Biol 1995; 7:319 - 24; http://dx.doi.org/10.1016/0955-0674(95)80085-9; PMID: 7662360
- Scheer U, Hock R. Structure and function of the nucleolus. Curr Opin Cell Biol 1999; 11:385 - 90; http://dx.doi.org/10.1016/S0955-0674(99)80054-4; PMID: 10395554
- Hernandez-Verdun D. Assembly and disassembly of the nucleolus during the cell cycle. Nucleus 2011; 2:189 - 94; http://dx.doi.org/10.4161/nucl.2.3.16246; PMID: 21818412
- Muro E, Gébrane-Younís J, Jobart-Malfait A, Louvet E, Roussel P, Hernandez-Verdun D. The traffic of proteins between nucleolar organizer regions and prenucleolar bodies governs the assembly of the nucleolus at exit of mitosis. Nucleus 2010; 1:202 - 11; http://dx.doi.org/10.4161/nucl.1.2.11334; PMID: 21326952
- Mullineux ST, Lafontaine DL. Mapping the cleavage sites on mammalian pre-rRNAs: Where do we stand?. Biochimie 2012; In press http://dx.doi.org/10.1016/j.biochi.2012.02.001; PMID: 22342225
- Grummt I. Regulation of mammalian ribosomal gene transcription by RNA polymerase I. Prog Nucleic Acid Res Mol Biol 1999; 62:109 - 54; http://dx.doi.org/10.1016/S0079-6603(08)60506-1; PMID: 9932453
- Shaw P, Doonan J. The nucleolus. Playing by different rules?. Cell Cycle 2005; 4:102 - 5; http://dx.doi.org/10.4161/cc.4.1.1467; PMID: 15655371
- Ghoshal K, Jacob ST. Heat shock selectively inhibits ribosomal RNA gene transcription and down-regulates E1BF/Ku in mouse lymphosarcoma cells. Biochem J 1996; 317:689 - 95; PMID: 8760351
- Mekhail K, Rivero-Lopez L, Khacho M, Lee S. Restriction of rRNA synthesis by VHL maintains energy equilibrium under hypoxia. Cell Cycle 2006; 5:2401 - 13; http://dx.doi.org/10.4161/cc.5.20.3387; PMID: 17102617
- Andersen JS, Lam YW, Leung AK, Ong SE, Lyon CE, Lamond AI, et al. Nucleolar proteome dynamics. Nature 2005; 433:77 - 83; http://dx.doi.org/10.1038/nature03207; PMID: 15635413
- Moore HM, Bai B, Boisvert FM, Latonen L, Rantanen V, Simpson JC, et al. Quantitative proteomics and dynamic imaging of the nucleolus reveal distinct responses to UV and ionizing radiation. Mol Cell Proteomics 2011; 10:M111, 009241; http://dx.doi.org/10.1074/mcp.M111.009241; PMID: 21778410
- Mekhail K, Rivero-Lopez L, Al-Masri A, Brandon C, Khacho M, Lee S. Identification of a common subnuclear localization signal. Mol Biol Cell 2007; 18:3966 - 77; http://dx.doi.org/10.1091/mbc.E07-03-0295; PMID: 17652456
- Audas TE, Jacob MD, Lee S. Immobilization of proteins in the nucleolus by ribosomal intergenic spacer noncoding RNA. Mol Cell 2012; 45:147 - 57; http://dx.doi.org/10.1016/j.molcel.2011.12.012; PMID: 22284675
- Mayer C, Schmitz KM, Li J, Grummt I, Santoro R. Intergenic transcripts regulate the epigenetic state of rRNA genes. Mol Cell 2006; 22:351 - 61; http://dx.doi.org/10.1016/j.molcel.2006.03.028; PMID: 16678107
- Shaw PJ, Jordan EG. The nucleolus. Annu Rev Cell Dev Biol 1995; 11:93 - 121; http://dx.doi.org/10.1146/annurev.cb.11.110195.000521; PMID: 8689574
- Gonzalez IL, Sylvester JE. Complete sequence of the 43-kb human ribosomal DNA repeat: analysis of the intergenic spacer. Genomics 1995; 27:320 - 8; http://dx.doi.org/10.1006/geno.1995.1049; PMID: 7557999
- Hillis DM, Dixon MT. Ribosomal DNA: molecular evolution and phylogenetic inference. Q Rev Biol 1991; 66:411 - 53; http://dx.doi.org/10.1086/417338; PMID: 1784710
- Kaplan FS, Murray J, Sylvester JE, Gonzalez IL, O’Connor JP, Doering JL, et al. The topographic organization of repetitive DNA in the human nucleolus. Genomics 1993; 15:123 - 32; http://dx.doi.org/10.1006/geno.1993.1020; PMID: 8432523
- Wachtler F, Schöfer C, Schedle A, Schwarzacher HG, Hartung M, Stahl A, et al. Transcribed and nontranscribed parts of the human ribosomal gene repeat show a similar pattern of distribution in nucleoli. Cytogenet Cell Genet 1991; 57:175 - 8; http://dx.doi.org/10.1159/000133140; PMID: 1743070
- Sylvester JE, Whiteman DA, Podolsky R, Pozsgay JM, Respess J, Schmickel RD. The human ribosomal RNA genes: structure and organization of the complete repeating unit. Hum Genet 1986; 73:193 - 8; http://dx.doi.org/10.1007/BF00401226; PMID: 3015766
- Gonzalez IL, Gorski JL, Campen TJ, Dorney DJ, Erickson JM, Sylvester JE, et al. Variation among human 28S ribosomal RNA genes. Proc Natl Acad Sci U S A 1985; 82:7666 - 70; http://dx.doi.org/10.1073/pnas.82.22.7666; PMID: 3865188
- Sasaki T, Okazaki T, Muramatsu M, Kominami R. Variation among mouse ribosomal RNA genes within and between chromosomes. Mol Biol Evol 1987; 4:594 - 601; PMID: 3444415
- Sylvester JE, Petersen R, Schmickel RD. Human ribosomal DNA: novel sequence organization in a 4.5-kb region upstream from the promoter. Gene 1989; 84:193 - 6; http://dx.doi.org/10.1016/0378-1119(89)90155-8; PMID: 2606358
- Erickson JM, Schmickel RD. A molecular basis for discrete size variation in human ribosomal DNA. Am J Hum Genet 1985; 37:311 - 25; PMID: 2984926
- Levinson G, Gutman GA. Slipped-strand mispairing: a major mechanism for DNA sequence evolution. Mol Biol Evol 1987; 4:203 - 21; PMID: 3328815
- Moss T, Birnstiel ML. The putative promoter of a Xenopus laevis ribosomal gene is reduplicated. Nucleic Acids Res 1979; 6:3733 - 43; http://dx.doi.org/10.1093/nar/6.12.3733; PMID: 493120
- Moss T, Boseley PG, Birnstiel ML. More ribosomal spacer sequences from Xenopus laevis. Nucleic Acids Res 1980; 8:467 - 85; http://dx.doi.org/10.1093/nar/8.3.467; PMID: 7003549
- Morgan GT, Reeder RH, Bakken AH. Transcription in cloned spacers of Xenopus laevis ribosomal DNA. Proc Natl Acad Sci U S A 1983; 80:6490 - 4; http://dx.doi.org/10.1073/pnas.80.21.6490; PMID: 6579535
- Kuhn A, Grummt I. A novel promoter in the mouse rDNA spacer is active in vivo and in vitro. EMBO J 1987; 6:3487 - 92; PMID: 3428263
- Caudy AA, Pikaard CS. Xenopus ribosomal RNA gene intergenic spacer elements conferring transcriptional enhancement and nucleolar dominance-like competition in oocytes. J Biol Chem 2002; 277:31577 - 84; http://dx.doi.org/10.1074/jbc.M202737200; PMID: 12080048
- Paalman MH, Henderson SL, Sollner-Webb B. Stimulation of the mouse rRNA gene promoter by a distal spacer promoter. Mol Cell Biol 1995; 15:4648 - 56; PMID: 7623857
- Audas TE, Jacob MD, Lee S. The nucleolar detention pathway: A cellular strategy for regulating molecular networks. Cell Cycle 2012; In press
- Schmitz KM, Mayer C, Postepska A, Grummt I. Interaction of noncoding RNA with the rDNA promoter mediates recruitment of DNMT3b and silencing of rRNA genes. Genes Dev 2010; 24:2264 - 9; http://dx.doi.org/10.1101/gad.590910; PMID: 20952535
- Mayer C, Neubert M, Grummt I. The structure of NoRC-associated RNA is crucial for targeting the chromatin remodelling complex NoRC to the nucleolus. EMBO Rep 2008; 9:774 - 80; http://dx.doi.org/10.1038/embor.2008.109; PMID: 18600236
- Santoro R, Schmitz KM, Sandoval J, Grummt I. Intergenic transcripts originating from a subclass of ribosomal DNA repeats silence ribosomal RNA genes in trans. EMBO Rep 2010; 11:52 - 8; http://dx.doi.org/10.1038/embor.2009.254; PMID: 20010804
- Mekhail K, Gunaratnam L, Bonicalzi ME, Lee S. HIF activation by pH-dependent nucleolar sequestration of VHL. Nat Cell Biol 2004; 6:642 - 7; http://dx.doi.org/10.1038/ncb1144; PMID: 15181450
- Mekhail K, Khacho M, Carrigan A, Hache RR, Gunaratnam L, Lee S. Regulation of ubiquitin ligase dynamics by the nucleolus. J Cell Biol 2005; 170:733 - 44; http://dx.doi.org/10.1083/jcb.200506030; PMID: 16129783
- Mekhail K, Khacho M, Gunaratnam L, Lee S. Oxygen sensing by H+: implications for HIF and hypoxic cell memory. Cell Cycle 2004; 3:1027 - 9; http://dx.doi.org/10.4161/cc.3.8.1075; PMID: 15280664
- Bernardi R, Scaglioni PP, Bergmann S, Horn HF, Vousden KH, Pandolfi PP. PML regulates p53 stability by sequestering Mdm2 to the nucleolus. Nat Cell Biol 2004; 6:665 - 72; http://dx.doi.org/10.1038/ncb1147; PMID: 15195100
- Welch WJ, Feramisco JR. Nuclear and nucleolar localization of the 72,000-dalton heat shock protein in heat-shocked mammalian cells. J Biol Chem 1984; 259:4501 - 13; PMID: 6368558
- Zentner GE, Saiakhova A, Manaenkov P, Adams MD, Scacheri PC. Integrative genomic analysis of human ribosomal DNA. Nucleic Acids Res 2011; 39:4949 - 60; http://dx.doi.org/10.1093/nar/gkq1326; PMID: 21355038
- Andrysik Z, Bernstein WZ, Deng L, Myer DL, Li YQ, Tischfield JA, et al. The novel mouse Polo-like kinase 5 responds to DNA damage and localizes in the nucleolus. Nucleic Acids Res 2010; 38:2931 - 43; http://dx.doi.org/10.1093/nar/gkq011; PMID: 20100802