Abstract
Sirtuin 6 (Sirt6), a mammalian Sir2 (silent information regulator-2) ortholog, is an NAD+-dependent histone deacetylase that modulates chromatin structure and genomic stability. Sirt6 knockout cells demonstrate genomic instability, and a deficiency of Sirt6 in mice leads to an aging phenotype early in life. Some nuclear sirtuins, such as Sirt7, localize to the nucleolus, and others, such as Sirt1, are mainly found in the nucleoplasm, with a minor population in the nucleolus. However, Sirt6 has been reported to be a nucleoplasmic protein that is excluded from the nucleolus. Because of the importance of a protein’s localization to its interactions and functions, we evaluated Sirt6 sub-cellular localization, expression and functions throughout the cell cycle in HeLa cells. Our results showed that during interphase, Sirt6 was mostly localized to the nucleus, although it was not absent from the nucleolus. Sirt6 was enriched in the nucleolus in the G1 phase of the cell cycle, while S phase nucleoli were almost entirely free of Sirt6. During mitosis, the Sirt6 expression level was increased, and while Sirt6 was not associated with condensed chromosomes, it partially co-localized with mitotic spindles. Cells overexpressing Sirt6 had a lower proliferation rate with a lower percentage of cells in mitosis. These findings suggest roles for Sirt6 in the nucleolus and in the mitotic phase of the cell cycle.
Keywords: :
Introduction
The silent information regulator (Sir) genes are required for transcriptional silencing in the budding yeast S. cerevisiae, and among them, Sir2 is highly conserved from prokaryotes to eukaryotes.Citation1 As an NAD+-dependent histone deacetylase, Sir2 has been found to be necessary for transcriptional silencing at the silent mating loci and at telomeres and in rDNA (rDNA)Citation2; Sir2 also regulates recombination, genomic stability and cellular longevity.Citation3-Citation5 Among the seven mammalian Sir2 homologs (sirtuin 1-7, Sirt1-7),Citation2 Sirt1 is the closest ortholog to Sir2 and is also the most studied sirtuin. It deacetylates histones, as well as a broad range of transcription factors, and has roles in many cellular processes, such as metabolism, cell differentiation and stress response; it has also been implicated in the development of cancer.Citation6 Interestingly, Sirt1 has functions in the cytoplasm, nucleoplasm and even in the nucleolus, where it is involved in the epigenetic regulation of rDNA loci.Citation7,Citation8 Sirt7, the nearest homolog to Sirt6, is a nucleolar protein that functions in the resumption of rDNA transcription upon the cell’s exit from mitosis.Citation9,Citation10 Sirt6 is reportedly a chromatin-associated nuclear protein that is involved in transcription, genome and telomere stabilization, DNA repair, and metabolic homeostasis.Citation11-Citation17 Sirt6 knockout cells show genomic instability and hypersensitivity to DNA damage,Citation14,Citation18 and a deficiency of Sirt6 in mice leads to an early aging phenotype, a shortened life span and severe metabolic problems.Citation14 As a histone deacetylase, Sirt6 deacetylates at least two lysines: lysines 9 and 56 of histone H3 (H3K9 and H3K56).Citation13,Citation19,Citation20 It was shown that by acetylating H3K9, Sirt6 modulates telomeric chromatinCitation13 and inhibits the expression of a subset of nuclear factor kappa B (NFκB) target genes.Citation12 In addition to histones, Sirt6 can deacetylate the C-terminal binding protein (CtBP) interacting protein (CtIP) and thus promote DNA repair.Citation11 Sirt6 also promotes mono-ADP ribosylation, an alternative NAD+-dependent reaction that has been reported for some other members of the sirtuin family.Citation21,Citation22
Of the 7 sirtuins, Sirt1 and 6 are localized to the nucleoplasm and Sirt7 is localized to the nucleolus.Citation21,Citation23 We noticed that the nucleoli of cultured cells were not free of Sirt6, which was contrary to a previous report of Sirt6 being excluded from the nucleolus.Citation23 In light of the importance of protein localization to its function, we tried to evaluate Sirt6 localization in cultured cells, the underlying mechanisms of its localization and its possible functions, especially in the nucleolus.
Results
Sirt6 nucleolar localization
A rabbit antibody was produced against Sirt6 amino acids 178–250 of the human Sirt6 protein – a region that was not conserved in other members of the family and is 93% identical between human and rat Sirt6. In HeLa cells transfected with FLAG-tagged wild-type Sirt6 (FLAG-Sirt6), staining for this antibody co-localized with FLAG tag staining in the nucleus, and our antibody did not show cross-reactivity with other nuclear sirtuins (). By western blot, the Sirt6 antibody specifically recognized FLAG-Sirt6, a 49-kDa polypeptide ().
Figure 1. Specificity of the Sirt6 antibody. (A) An anti-FLAG antibody and the anti-Sirt6 antibody that was generated for this study were perfectly co-localized in cells overexpressing Sirt6 (first row). HeLa cells transfected with FLAG-tagged Sirt1, Sirt7 or HA-tagged Sirt2 did not show any increase in the Sirt6 signal, indicating a lack of cross-reactivity of the antibody with other nuclear sirtuins (rows 2–4). Plot profile analysis of selected cells are shown on the right. (B) In a western blot of the lysate of Sirt6-overexpressing cells, the Sirt6 antibody [Sirt6 (M)] detected the same 49 kDa band as the FLAG antibody. Compared with the bands detected by commercial anti-Sirt6 antibodies against its N- and C-termini [Sirt6 (N) and Sirt6(C), respectively], Sirt6 (M) detected one extra lower-molecular weight band, as did the Sirt6(C) antibody. The identity of the lower-molecular weight Sirt6 remains unknown. Scale bar, 10 µm.
![Figure 1. Specificity of the Sirt6 antibody. (A) An anti-FLAG antibody and the anti-Sirt6 antibody that was generated for this study were perfectly co-localized in cells overexpressing Sirt6 (first row). HeLa cells transfected with FLAG-tagged Sirt1, Sirt7 or HA-tagged Sirt2 did not show any increase in the Sirt6 signal, indicating a lack of cross-reactivity of the antibody with other nuclear sirtuins (rows 2–4). Plot profile analysis of selected cells are shown on the right. (B) In a western blot of the lysate of Sirt6-overexpressing cells, the Sirt6 antibody [Sirt6 (M)] detected the same 49 kDa band as the FLAG antibody. Compared with the bands detected by commercial anti-Sirt6 antibodies against its N- and C-termini [Sirt6 (N) and Sirt6(C), respectively], Sirt6 (M) detected one extra lower-molecular weight band, as did the Sirt6(C) antibody. The identity of the lower-molecular weight Sirt6 remains unknown. Scale bar, 10 µm.](/cms/asset/03ecd484-37a6-4f12-a80d-9e88e72df992/kncl_a_10921134_f0001.gif)
When immunostained with the above Sirt6 antibody, most asynchronous HeLa cells showed both nucleoplasmic and nucleolar immunoreactivity of endogenous Sirt6, and among them, ~60% showed enrichment of the protein in the nucleolus (). Sirt6 was also enriched in the nucleoli of cells subjected to serum deprivation, whereas treatment of HeLa cells with low doses of actinomycin D caused Sirt6 to be released from the nucleolus and spread into the nucleoplasm (). Previously, Sirt6 was said to be a nuclear protein that is excluded from the nucleolus,Citation23 while Sir2, Sirt1 and Sirt7 have known functions in the nucleolus.Citation7,Citation8,Citation24 Our observations indicated a great variation of nucleolar localization of Sirt6 among different cells and under different culture conditions, ranging from no nucleolar Sirt6 in a few cells to a relatively high level of nucleolar Sirt6 in a majority of cells. This suggested that Sirt6 might shuttle rapidly, and its localization might change with cell cycle progression. We thus investigated Sirt6 sub-cellular localization in relation to the different steps of the cell cycle.
Figure 2. Sub-nuclear localization of endogenous Sirt6. (A) Asynchronous HeLa cells stained with anti-Sirt6 (M) show both nucleoplasmic and nucleolar staining. (B) Compared with cells cultured under normal conditions, serum deprivation causes the nucleolar Sirt6 signal to increase, while actinomycin D treatment causes nucleolar Sirt6 to be released into the nucleoplasm. (C) Sirt6 does not co-localize with intense DAPI staining, especially in the perinucleolar region. AMD, actinomycin D; NL, normal; SD, serum deprivation. Scale bars, 10 µm.
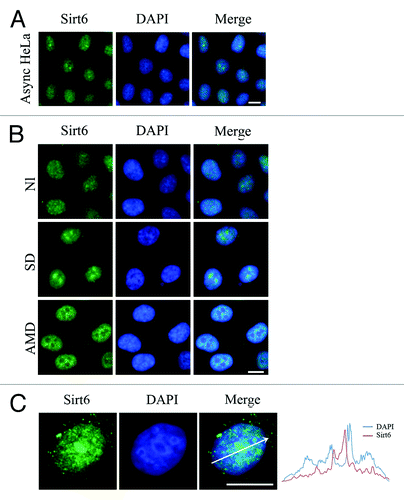
On a separate note, Sirt6 was reported to be linked to heterochromatic regions,Citation23 but interestingly, our study in HeLa cells showed that Sirt6 was enriched in areas with weak DAPI staining, which represent euchromatin. Meanwhile, areas with condensed chromatin, including the nucleolar periphery, showed less Sirt6 staining ().
Nucleolar localization sequence (NoLS) of Sirt6
Nuc-PLoc software, a package of computational tools,Citation25 predicted that Sirt6 would localize to the nucleolus. The Nucleolar Localization Sequence Detector, NoD,Citation26,Citation27 predicted one NoLS in the C-terminal 28 aa sequence (between aa 328 and 355, PASPKRERPTSPAPHRPPKRVKAKAVPS) of the Sirt6 protein (). The 7 amino acid sequence from residue 345 to 351 (PKRVKAK) was previously reported as a nuclear localization signal (NLS) in Sirt6 and is partially responsible for the translocation of this protein to the nucleus.Citation15 Our results also showed that a Sirt6 mutant lacking the last 35 aa (Sirt6ΔC) was partially delocalized from the nucleus to the cytoplasm. In HeLa cells, overexpressed FLAG-Sirt6ΔC was found in the cytoplasm and nucleus but not in the nucleolus. Thus, although the C-terminus is not sufficient for the nuclear localization of Sirt6, the lack of the C-terminus seemed sufficient to completely exclude Sirt6 from the nucleolus (). Hence, the Sirt6 C-terminus most likely serves both as a partial NLS and a NoLS.
Figure 3. Nucleolar localization of Sirt6. (A) Based on the Sirt6 primary sequence, the Nucleolar Localization Sequence Detector predicted a NoLS in its C-terminus. (B) Overexpressed Sirt6ΔC, which lacks the last 35 residues, shows a partial localization to nucleus but is excluded from the nucleolus. (C) Sirt6 mutants, which mimic phosphorylated or dephosphorylated Sirt6, as well as the catalytically inactive form of Sirt6, can all translocate to nucleoli and are enriched in the nucleoli in the G1 phase. Scale bars, 10 µm.
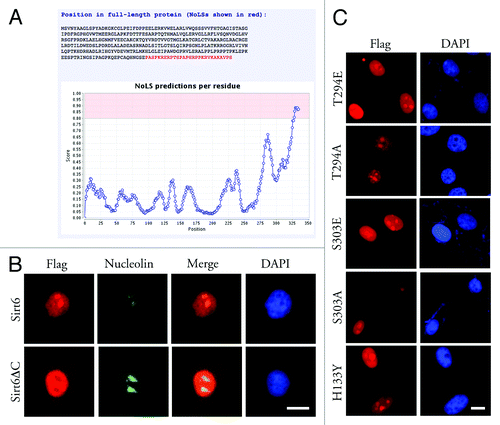
Sirt6 nucleolar localization is independent of its phosphorylation (at sites 294 and 303) and enzymatic activity
In HeLa cells that have been arrested in the G1 and M phases of the cell cycle, an evaluation of protein phosphorylation has shown that Sirt6 is phosphorylated at Thr294 and Ser303 in the G1 phase, but not during mitosis.Citation28 Phosphorylation/dephosphorylation has known roles in the cell cycle-dependent expression of genes and in the localization of proteins.Citation29 To evaluate if this phosphorylation/dephosphorylation of Sirt6 has any role in its nucleolar localization, the following mutants of Sirt6 were constructed: T294A, S303A (mimicking dephosphorylated Sirt6), T294E and S303E (mimicking phosphorylated Sirt6). G1-synchronized HeLa cells transfected with these constructs showed a nucleolar localization of all four mutants. Thus, the phosphorylation/dephosphorylation of Sirt6 at these sites seemed to not be important to its nucleolar translocation ().
The H133Y mutation abrogates the catalytic activity of Sirt6 and alters the dynamics of Sirt6 association with chromatin in cells.Citation15 To determine if the enzymatic activity of Sirt6 is needed for its nucleolar localization, HeLa cells were transfected with FLAG-Sirt6(H133Y) and synchronized at the G1 phase. The cells showed nucleolar localization of mutant Sirt6 (). Thus, to be localized to the nucleolus, Sirt6 does not need to be enzymatically active and associated with chromatin.
Antibodies against the C-terminus of Sirt6 do not detect nucleolar Sirt6
Previous studies, most of which used antibodies against the Sirt6 C-terminus, reported Sirt6 localization to the nucleus, but not to the nucleolus. Our immunolabeling of endogenous Sirt6 in HeLa cells used an antibody against the Sirt6 C-terminus and also failed to detect nucleolar Sirt6; instead, a diffuse nucleoplasmic staining with perinucleolar enrichment was observed (). To demonstrate that this is not a non-specific staining artifact, the localization of FLAG-Sirt6-HA (FLAG at the N-terminus and HA at the C-terminus of Sirt6) was compared with that of Sirt6-FLAG (FLAG at the C-terminus) (). In transfected HeLa cells, the C-terminal antibody and the anti-FLAG and anti-HA antibodies did not show a nucleolar signal when Sirt6 was tagged at its C-terminus, whereas the signal was very obvious in the nucleolus for N-terminally tagged Sirt6.
Figure 4. Antibodies against the Sirt6 C-terminus or C-terminal tags cannot detect nucleolar Sirt6. (A) In G1-arrested cells, antibodies against the Sirt6 N-terminus (Sigma) (upper row) and against a middle region of Sirt6 (middle row) show a nucleoplasmic signal and a strong nucleolar signal. Meanwhile, the antibody against the C-terminus (Sigma) does not show a nucleolar signal, but instead shows a diffuse nucleoplasmic signal and a strong signal in the perinucleolar region (lower row). (B) When Sirt6 was tagged with FLAG at its N-terminus and HA at its C-terminus, the anti-FLAG antibody shows nucleolar Sirt6, while anti-HA is not detectable in the nucleolus (upper row). Anti-FLAG also cannot detect any Sirt6 when the tag is at the C-terminus (lower row). Scale bars, 10 µm.
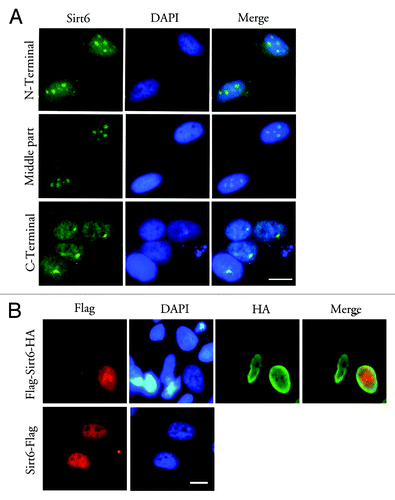
The nucleolar localization of Sirt6 is cell cycle dependent
To investigate the sub-nuclear localization of Sirt6 during the cell cycle, HeLa cells were synchronized in the G1/S phase by a double thymidine block, released and fixed at different time points. During interphase, both overexpressed FLAG-Sirt6 and endogenous Sirt6 were mostly localized to the nucleus, but the ratio of nucleoplasmic/nucleolar Sirt6 changed as the cell cycle progressed. Two hours after releasing the cells from the double thymidine block, when most of the cells were in S phase, nucleoli were almost free of Sirt6, while in cells that were synchronized in G1/G0 (using either sodium butyrate (NaBt) or serum deprivation), Sirt6 showed an obvious enrichment in the nucleolus. The distribution of FLAG-Sirt6 in the nucleolus is shown in . Examples of intensity plots of FLAG, DAPI and nucleolin signals are shown on the right to better illustrate the sub-nuclear localization of Sirt6. The distribution of endogenous Sirt6 is shown in . Nucleolar Sirt6 was also detected using electron microscopy (EM) in HeLa cells synchronized in the G1 phase using NaBt (). The EM results showed that Sirt6 is enriched in G1 phase cells, mostly in the granular components (GCs) of nucleoli, and is almost absent in the fibrillar centers (FCs).
Figure 5. Sirt6 is enriched in the nucleolus in the G1 phase of the cell cycle. (A) Distribution of Sirt6 in the nucleus. Comparison with nucleolin and DAPI shows that Sirt6 is enriched in the nucleolus during the G1 phase of the cell cycle. In S phase, the nucleolus is almost free of Sirt6. The framed cells in the panels of the third column were analyzed, as shown in the photomicrographs in the last column, and the quantitative results are shown on the right. Scale bars, 10 µm. (B) Endogenous Sirt6 is also enriched in the nucleolus in G1, while in S phase, the Sirt6 signal is stronger in the nucleoplasm than in the nucleolus. (C) Immunoperoxidase electron microscopy of G1-arrested HeLa cells using anti-Sirt6 confirms nucleolar Sirt6 and shows that it is mostly in GCs (a). Panel b shows the negative control staining with no primary antibody. N, nucleus; Nucl, nucleolus; F, fibrillar center; D, dense fibrillar component; G, granular component. Scale bars, 1 µm.
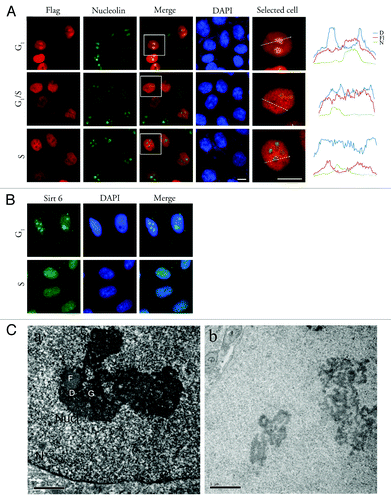
In mitosis, Sirt6 expression increases
The nucleolus is disassembled in prophase and re-assembled at the end of mitosis.Citation30 To have a more complete view of Sirt6 subcellular localization, we evaluated its localization during mitosis as well. Twelve hours after release from the double thymidine block, cells were fixed to evaluate Sirt6 localization in mitotic cells. In these cells, Sirt6 was spread out in the cytoplasm and was not associated with chromosomes (). Additionally, Sirt6 was partially co-localized with α-tubulin at the mitotic spindles. Both endogenous and overexpressed FLAG-Sirt6 showed a similar distribution in the mitotic cells. An antibody against the Sirt6 C-terminus also confirmed that during mitosis, Sirt6 is not associated with chromatin, but this antibody did not detect Sirt6 at mitotic spindles (data not shown). Sirt6 co-localized with DNA again in late telophase.
Figure 6. Sirt6 localization and expression level in mitosis. (A) In mitosis, endogenous Sirt6 localizes to the cytoplasm, partially co-localizes with mitotic spindles, and does not associate with condensed chromatin. In telophase, Sirt6 co-localizes with the nuclei of new cells. (B) Immunostaining of mitotic HeLa cells shows a stronger signal for endogenous Sirt6 compared with the signal in neighboring cells (left panel) in interphase (p < 0.0001, Student’s t-test). This difference was also significant when cells in each mitotic step of prophase/prometaphase, metaphase, anaphase and telophase were compared with interphase cells separately (p < 0.0001). The Sirt6 signal intensity in metaphase was significantly higher than in prophase/prometaphase and telophase (p < 0.005). (C) Immunoblotting also showed a higher level of endogenous Sirt6 in mitotic cells than in asynchronous Hela cells and cells arrested in G1, S or G2. Scale bars, 10 µm.
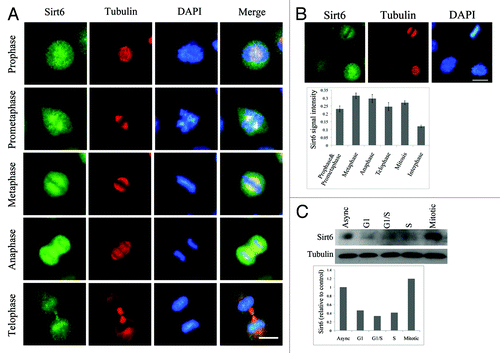
On the immunostained cell culture preparation, mitotic cells showed a significantly higher level of Sirt6 compared with neighboring interphase cells (p < 0.0001, Student’s t-test) (). This difference was also significant when mitotic cells were categorized into prophase/prometaphase, metaphase, anaphase and telophase, according to DAPI and α-tubulin staining, and each step was compared with interphase cells (p < 0.0001). Metaphase cells showed the highest level of Sirt6 (p < 0.005). Levels of endogenous Sirt6 in mitotic cells were also compared with those in asynchronous cells and cells arrested in G1, S and G2 using a western blot. The results showed that Sirt6 levels increased in mitotic cells, confirming the immunostaining results ().
Cells with high expression of FLAG-Sirt6 showed a delay in entering mitosis
At 12 h after synchronization in G1/S, immunolabeling of cells transfected with FLAG-Sirt6 showed a lower percentage of mitotic cells (p = 0.02, Student’s t-test) compared with cells transfected by FLAG-H133Y (). This difference was much stronger when the percentage of mitotic cells was compared in cells with high expression of exogenous proteins (Sirt6 vs. H133Y) (p < 0.005, Student’s t-test). This result suggested a delay in entering mitosis among cells with a high expression of Sirt6. We also assessed the proliferation rate of cultured cells transfected by wild type and mutant Sirt6 by using a 3-(4,5-dimethylthiazol-2-yl)-2,5-diphenyl tetrazolium bromide (MTT) assay. The result showed that cells transfected with FLAG-Sirt6 had a lower proliferation rate (). This ruled out the possibility of a fast asynchronous entrance of Sirt6-transfected cells into mitosis. To evaluate the possibility of apoptosis induced by a high level of exogenous Sirt6, we compared apoptosis rate in Sirt6 overexpressing cells and their untransfected neighboring cells. We also compared apoptosis rate in Sirt6 overexpressing cells with the cells expressing a mutant form of Sirt6, W42R, which is toxic to the cells and increased apoptosis rate in host cells (unpublished data). To measure apoptosis rate, nuclear staining using DAPI together with antibody against cleaved caspase-3 (CC3) was used and the level of CC3 staining was measured as OD using ImageJ software. Our results showed that Sirt6 overexpression does not have an apoptosis-inducing effect in the cells cultured under normal conditions (Fig. S1). Furthermore, Flow cytometric analysis of cell cycle distribution of HeLa cells overexpressing Sirt6 and Sirt6 nonfunctional mutant (H133Y) with PI staining showed that the percentage of S phase cells is higher in Sirt6 group compared with H133Y group. These results confirm that Sirt6 overexpression causes S phase arrest, delay in entering mitosis and a lower proliferation rate.
Figure 7. Sirt6 delays host cell mitosis. (A) The left panel shows that 12 h after transfected cells were synchronized in early S phase, the percentage of transfected cells that were in mitosis was much less for Sirt6 than for the nonfunctional Sirt6 mutant (H133Y). The difference is significant (Trans) (p = 0.02), especially when we compared the mitotic cell percentage in cells expressing Sirt6 or the mutant at a high level (Trans high) (p < 0.005). The percentage of mitotic cells in neighboring untransfected cells was not different between the two groups (Untrans). (B) An MTT assay shows a lower proliferation rate in Sirt6-overexpressing cells compared with cells overexpressing the Sirt6 mutant. Scale bar, 10 µm.
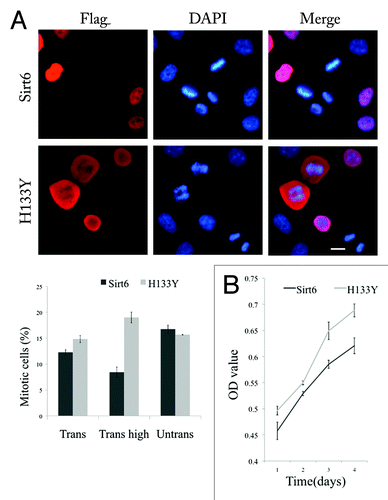
Discussion
Sirt6 is believed to be a nuclear protein that is excluded from nucleoli and associated with heterochromatin.Citation23 Our results showed, however, that although Sirt6 is mainly in the nucleus, its sub-nuclear localization changes during the cell cycle. While Sirt6 is largely excluded from the nucleolus during S phase, it can be detected there during the rest of interphase and is enriched in the nucleolus during the G1 phase. We found that the Sirt6 C-terminus, which is partially responsible for its nuclear localization, also serves as a NoLS. Additionally, Sirt6 mutants could still localize to the nucleolus, regardless of the state of their phosphorylation and catalytic activity. Therefore, we did not have any construct that showed complete nuclear but no nucleolar localization with which to evaluate the results of preventing nucleolar localization. However, it is possible that other modifications or protein interactions are involved in this shuttling. The difference in the detection of nucleolar Sirt6 by antibodies against its C-terminus or against C-terminal tags could be accounted for by the cleavage of the Sirt6 C-terminus after its arrival at the nucleolus, or perhaps Sirt6 might interact with some nucleolar proteins that could mask the C-terminal epitope of Sirt6. Theoretically, some posttranslational modifications in the nucleolus may alter the C-terminal reactivity of Sirt6 to the antibodies against different domains. We also thought about different isomers of Sirt6 in the cell (for example some nucleolar isomers lacking C-terminal), but using Flag-Sirt6- HA we showed that even for a single Sirt6 molecule the antibody against the N-terminal tag detects Sirt6 in the nucleolus while antibody against the tag in the C-terminal cannot detect the same molecule.
We showed that Sirt6 does not co-localize with high density DAPI staining, which represents heterochromatin, especially in the perinucleolar region. A large fraction of Sirt1 is associated with euchromatin,Citation31 and Sirt1 interacts with histone H1 and promotes heterochromatin formation.Citation32,Citation33 Thus, further studies are needed to evaluate the exact role of Sirt6 on chromatin regulation.
The nucleolus plays important roles in ribosome biogenesis, as well as in other recently found functions in cell-cycle control and stress response.Citation34 In yeast, only half of rDNA repeats are transcribed, and the rest are repressed by a mechanism that involves Sir2. The yeast Sir2 protein binds to Net1, which associates with rDNA and recruits Sir2 to the nucleolus.Citation24,Citation35,Citation36 Net1, Sir2 and Cdc14 are components of a multifunctional complex, RENT (regulator of nucleolar silencing and telophase) which is involved in nucleolar silencing and exit from mitosis.Citation37 The nucleolar proteome is largely conserved, and proteomic studies have shown that more than 90 percent of human nucleolar proteins have yeast homologs.Citation38 The mammalian rDNA locus is also under epigenetic regulation by protein complexes, such as NoRC (nucleolar remodeling complex) and eNoSC (energy-dependent nucleolar silencing complex), which involve histone deacetylases and methyltransferases.Citation8 A notable exception is Sirt1, the key component of the eNoSC complex,Citation8 which is essentially nucleoplasmic but shows a faint nucleolar location.Citation39 Epigenetic mechanisms, such as DNA methylation and histone modification, are major mechanisms in silencing mammalian rRNA genes.Citation8 Among mammalian sirtuins, Sirt7 is exclusively nucleolar and is a component of the RNA polymerase I (Pol I) transcriptional machinery, where it functions by stimulating Pol I transcription.Citation9 Recently, Sirt7 was found to be involved in the resumption of rDNA transcription upon exit from mitosis.Citation10 Here, we report for the first time that another member of the sirtuin family, Sirt6, also shows nucleolar localization, and its nucleolar level changes during the cell cycle. Based on the role of Sir2 in rDNA repression and the functions of other sirtuin members (Sirt1 and 7) in the nucleolus, it is very possible that Sirt6 plays a role in rRNA transcription. Low doses of actinomycin D selectively inhibit transcription by RNA Pol I and causes the release of proteins such as Sirt7 which are involved in rDNA transcription from the nucleolus to the nucleoplasm.Citation9 The treatment of HeLa cells with actinomycin D caused the release of endogenous Sirt6 from the nucleolus. This result first confirms nucleolar localization of Sirt6 and highlights the signal intensity that we see in normal cell, serum deprived cell and G1 arrested cell. It also gives preliminary information suggesting a role for Sirt6 in rDNA transcription system and suggests possible interacting partners for Sirt6 in the nucleolus, which is discussed below. However, none of available Sirt6 antibodies which are against N-terminal or middle part of Sirt6 are suitable for immunoprecipitation and our ChIP assay failed to detect a direct association of Sirt6 with rDNA, which might be due either to technical problems or to the possibility of an indirect association. Furthermore, EM studies showed that in G1-arrested cells, Sirt6 localized mostly to the GCs of the nucleolus, which raises the possibility that Sirt6 plays a role in the modification of later steps of rRNA processing and the maturation of ribosomal structures. Transcription of rDNA is modulated during the cell cycle, from low levels in the early G1 phase to its highest levels in the S and G2 phases.Citation40 G1 enrichment of nucleolar Sirt6 suggests that Sirt6 plays a repressor role with regards to ribosome biogenesis. Furthermore, Sirt6 impacts DNA repair, and interestingly, both ribosome biogenesis and rDNA stability are linked to aging.Citation41,Citation42 rDNA is the most unstable part of the genome because of its repetitive nature. Thus, in a manner similar to telomere shortening, rDNA instability may trigger DNA damage response (DDR), causing cell cycle arrest and the activation of DNA damage repair pathways.Citation41 Therefore, it is possible that the Sirt6 role in the nucleolus has a direct impact on the process of aging due to its possible effects on ribosome biogenesis or rDNA damage repair. Beside all of these possibilities, for some proteins, the nucleolus is a place of sequestration, which prevents proteins from reaching their targets, such as those in the nucleoplasm.Citation43
It was previously reported that GFP-tagged Sirt6 and Sirt7 remain associated with condensed chromosomes in mitosis.Citation23 However, it was later shown that in contrast to GFP-Sirt7, endogenous Sirt7 remains associated with nucleolar organizer regions (NORs) and co-localizes with the upstream binding factor (UBF) during mitosis.Citation10 In this study, we showed that mitotic HeLa cells show a high level of Sirt6, with the highest level in metaphase, and this suggests that Sirt6 may play a role in mitosis, which would require Sirt6 to be regulated during the cell cycle as well as during the mitosis steps themselves. We also showed that endogenous Sirt6 did not associate with condensed chromatin during mitosis, but showed a diffuse staining in the cytoplasm, was excluded from chromatin, and was partially localized to mitotic spindles. Interestingly, the other nucleolar protein, nucleolar phosphoprotein B23 (or nucleophosmin), which is also present in GCs and involved in rRNA processing, shows a similar localization in mitosis.Citation34 B23 is also released from nucleoli to the nucleoplasm after actinomycin D treatment, which is comparable to Sirt6 relocation. B23, however, is a known substrate of Sirt1,Citation44 and we suggest that its possible interaction with Sirt6 should also be evaluated. The fact that antibodies against the Sirt6 C-terminus could not detect nucleolar Sirt6, along with the fact that Sirt6 localizes to the mitotic spindles, suggests that the spindle-localized Sirt6 had previously been released from the nucleolus, and for some reason, its C-terminus is not detectable.
According to our results, in cells with a high expression of exogenous Sirt6, mitosis was delayed or inhibited relative to cells with a high expression of mutant Sirt6. Previous studies did not show any change in cell cycle checkpoints in Sirt6-deficient cells.Citation14 However, during mitosis, the Sirt2 expression level also increases, and Sirt2 is associated with centrosomes, mitotic spindles, and the midbody.Citation45,Citation46 By regulating the anaphase-promoting complex/cyclosome (APC/C) activity, Sirt2 contributes to normal mitotic progression and has roles in exit from mitosis and most likely in maintaining chromatin stability.Citation47 Interestingly, the Sirt1 expression level also increases in prometaphase, and Sirt1 localizes to mitotic chromatin and promotes chromosomal condensation.Citation48
Because of the nucleoplasmic/nucleolar shuttling of Sirt6 during the cell cycle, and because of our finding during mitosis, we propose that Sirt6 might play a role in cell cycle progression, especially in chromatin condensation and in the regulation of ribosome biogenesis, as well as in other functions involving the nucleolus. It is hypothesized that Sirt6 functions in forming complexes with other proteins, perhaps with other members of the sirtuin family. These results raise new and important questions regarding the functions of this protein, which await future investigations. The issue of the cell-type specificity of Sirt6 functional roles also remains to be tested. Certain Sirt6 characteristics, for example, may be observed only in cancer cells.
Materials and Methods
Cloning of Sirt6 constructs
The open reading frame (ORF) of human Sirt6 (Accession: BC005026, protein ID: AAH05026) and nucleotides 1-960 (used to construct Sirt6ΔC) were amplified by PCR using a cDNA template purchased from the MGC (cDNA clone MGC: 12574; IMAGE: 2820472) (Invitrogen-FL1002). The fragment was inserted between the BamHI and HindIII sites of the FLAG tag pXJ40 vectorCitation49 to produce FLAG-tagged Sirt6 mammalian expression plasmids. Sirt6 was also tagged at both the N-terminus and C-terminus with FLAG and HA, respectively, by designing a reverse primer that contains the HA tag sequence. Point mutants of Sirt6 were generated by the GeneTailor™ Site-Directed Mutagenesis Kit (Invitrogen).
Cell culture and transfection
Cells were grown in the presence of 5% CO2 at 37°C, cultured in Dulbecco’s modified Eagle’s medium (DMEM, Sigma) supplemented with 10% fetal bovine serum (FBS, Sigma) and 1% Pen-Strep. The cells were transfected with Sirt6 plasmids using electroporation (MP-100 Microporator, Digital Bio Technology) and cultured on coverslips for appropriate times (48 h in asynchronous cultures, and different time points after releasing synchronous cultures) before being fixed. For the inhibition of RNA polymerase I transcription, cells were treated with 50 ng/ml actinomycin D (Sigma) for 2 h before being fixed.
Immunocytochemistry assays
The cells were fixed using paraformaldehyde (3%) for 20 min at room temperature, washed with PBS and blocked with PBS-T-NGS (0.3% Triton X-100 and 6% normal goat serum in 0.1 M PBS). Then, the cells were stained with primary antibodies and corresponding secondary antibodies. DAPI was used to stain the nuclei. Stained cells were observed and analyzed using a fluorescence microscope or a laser scanning confocal microscope (Olympus Fluoview FV 1000, Olympus). Anti-α-tubulin B-5–1-2 (T5168), anti-FLAG M2 (F1804), Anti-HA (H 6908), anti-Sirt6 antibodies (N-terminal (S4322) and C-terminal (S4197)), anti-nucleolin (N6288) and 4',6-diamidino-2-phenylindole (DAPI) were purchased from Sigma. The Sirt6 antibody that was generated for this study was raised in rabbit against amino acids 178–250 of the human Sirt6 protein.
To compare the signal intensity (fluorescent signal) of Sirt6 in the nucleoplasm and nucleolus and to compare that signal with DAPI intensity, confocal images were merged and the signals were analyzed by plot profile using ImageJ software.
For apoptosis assay, cells were subjected to immunostaining [using anti-cleaved caspase 3 (CC3) antibody] and signal intensity of CC3 was measured as optical density (OD) using imageJ software. After subtracting background signal, intensity of signal was compared between transfected and untransfected cells.
Immunoperoxidase staining
For immuno EM, immunoperoxidase (Avidin-biotin complex method) was performed using an ABC kit (Vectorlabs), and sections were sliced (~100 nm thickness) and observed under TEM. For details, refer to protocols described previously.Citation50 A Philips EM 208S electron microscope was used for the EM observation.
Cell synchronization
For the double thymidine block (early S-phase block), HeLa cells were cultured in 24-well or 6-well plates. At 30–40% confluency, the cells were washed two times with PBS, and DMEM+10% FBS+1% Pen-Strep and 2 mM thymidine was added to them for 18 h (first block). The thymidine was removed by washing the cells with PBS, and fresh media was added for the next 9 h to release the cells. For the second block, DMEM + 10% FBS + 1% Pen-Strep and 2 mM thymidine were added to the cells for 17 h. After that, the thymidine was removed, the cells were washed with PBS and fresh media was added. Cells progressed synchronously through the G2 and mitotic phases and therefore could be fixed at different time points to have cells in different phases of cell cycle.
For the thymidine-nocodazole block (mitotic block), HeLa cells at 40% confluency were washed with PBS, and DMEM + 10% FBS + 1% Pen-Strep and 2 mM thymidine were added for 24 h (S phase block). The thymidine was removed by washing the cells with PBS, and fresh media (DMEM + 10% FBS + 1% Pen-Strep) was added for the next 3 h to release the cells. 100 ng/ml nocodazole was then added to the media for 12 h (mitotic block). Nocodazole was removed by washing with PBS, and fresh medium was added to release the cells. To prepare a lysate of mitotic cells after nocodazole treatment, cells were collected using a shake-off method and seeded on another plate with fresh media. They were incubated for 1 h and then lysed.
To block the cells in G1, HeLa cells were cultured in normal media, and for 24 h, 6 mM NaBt was added to the media. To synchronize the cells in G0, HeLa cells were cultured in serum-deprived media (DMEM + 0.2% FBS) for 72 h.
Immunoblotting
Protein was extracted from the cells using an M-PER extraction kit (Pierce, Rockford, IL), resolved by SDS-PAGE gel, and transferred to PVDF membrane. After washing and blocking, membranes were incubated with primary antibody overnight at 4°C. Then, the membranes were incubated for one more hour with the secondary antibody (alkaline phosphatase-conjugated antibodies), and the signal was detected using CDP-Star reagent (Roche) on Kodak light films (Kodak). Anti-β-actin (A5316) was purchased from Sigma.
MTT assay
Transfected cells were seeded in 96-well plates (2000 cell/well). For measurement, a MTT (Sigma) stock solution, which was prepared in PBS (5 mg/ml), was added to cells in fresh medium for 1 h under cell culture conditions. The precipitates were dissolved by adding dimethylsulfoxide to the wells, and the absorbance was read by an Elisa reader (SpectraMax M5, Molecular Devices) at 595 nm.
Additional material
Download Zip (2.2 MB)Disclosure of Potential Conflicts of Interest
The authors do not have any conflict of interest.
Acknowledgments
This work was supported by the Singapore Biomedical Research Council (BMRC/06/1/21/19/460).
References
- Dutnall RN, Pillus L. Deciphering NAD-dependent deacetylases. Cell 2001; 105:161 - 4; http://dx.doi.org/10.1016/S0092-8674(01)00305-1; PMID: 11336664
- Blander G, Guarente L. The Sir2 family of protein deacetylases. Annu Rev Biochem 2004; 73:417 - 35; http://dx.doi.org/10.1146/annurev.biochem.73.011303.073651; PMID: 15189148
- Gasser SM, Cockell MM. The molecular biology of the SIR proteins. Gene 2001; 279:1 - 16; http://dx.doi.org/10.1016/S0378-1119(01)00741-7; PMID: 11722841
- Guarente L. Sir2 links chromatin silencing, metabolism, and aging. Genes Dev 2000; 14:1021 - 6; PMID: 10809662
- Imai S, Armstrong CM, Kaeberlein M, Guarente L. Transcriptional silencing and longevity protein Sir2 is an NAD-dependent histone deacetylase. Nature 2000; 403:795 - 800; http://dx.doi.org/10.1038/35001622; PMID: 10693811
- Zhang T, Kraus WL. SIRT1-dependent regulation of chromatin and transcription: linking NAD(+) metabolism and signaling to the control of cellular functions. Biochim Biophys Acta 2010; 1804:1666 - 75; PMID: 19879981
- Murayama A, Ohmori K, Fujimura A, Minami H, Yasuzawa-Tanaka K, Kuroda T, et al. Epigenetic control of rDNA loci in response to intracellular energy status. Cell 2008; 133:627 - 39; http://dx.doi.org/10.1016/j.cell.2008.03.030; PMID: 18485871
- Salminen A, Kaarniranta K. SIRT1 regulates the ribosomal DNA locus: epigenetic candles twinkle longevity in the Christmas tree. Biochem Biophys Res Commun 2009; 378:6 - 9; http://dx.doi.org/10.1016/j.bbrc.2008.11.023; PMID: 19010308
- Ford E, Voit R, Liszt G, Magin C, Grummt I, Guarente L. Mammalian Sir2 homolog SIRT7 is an activator of RNA polymerase I transcription. Genes Dev 2006; 20:1075 - 80; http://dx.doi.org/10.1101/gad.1399706; PMID: 16618798
- Grob A, Roussel P, Wright JE, McStay B, Hernandez-Verdun D, Sirri V. Involvement of SIRT7 in resumption of rDNA transcription at the exit from mitosis. J Cell Sci 2009; 122:489 - 98; http://dx.doi.org/10.1242/jcs.042382; PMID: 19174463
- Kaidi A, Weinert BT, Choudhary C, Jackson SP. Human SIRT6 promotes DNA end resection through CtIP deacetylation. Science 2010; 329:1348 - 53; http://dx.doi.org/10.1126/science.1192049; PMID: 20829486
- Kawahara TL, Michishita E, Adler AS, Damian M, Berber E, Lin M, et al. SIRT6 links histone H3 lysine 9 deacetylation to NF-kappaB-dependent gene expression and organismal life span. Cell 2009; 136:62 - 74; http://dx.doi.org/10.1016/j.cell.2008.10.052; PMID: 19135889
- Michishita E, McCord RA, Berber E, Kioi M, Padilla-Nash H, Damian M, et al. SIRT6 is a histone H3 lysine 9 deacetylase that modulates telomeric chromatin. Nature 2008; 452:492 - 6; http://dx.doi.org/10.1038/nature06736; PMID: 18337721
- Mostoslavsky R, Chua KF, Lombard DB, Pang WW, Fischer MR, Gellon L, et al. Genomic instability and aging-like phenotype in the absence of mammalian SIRT6. Cell 2006; 124:315 - 29; http://dx.doi.org/10.1016/j.cell.2005.11.044; PMID: 16439206
- Tennen RI, Berber E, Chua KF. Functional dissection of SIRT6: identification of domains that regulate histone deacetylase activity and chromatin localization. Mech Ageing Dev 2010; 131:185 - 92; http://dx.doi.org/10.1016/j.mad.2010.01.006; PMID: 20117128
- Xiao C, Kim HS, Lahusen T, Wang RH, Xu X, Gavrilova O, et al. SIRT6 deficiency results in severe hypoglycemia by enhancing both basal and insulin-stimulated glucose uptake in mice. J Biol Chem 2010; 285:36776 - 84; http://dx.doi.org/10.1074/jbc.M110.168039; PMID: 20847051
- Zhong L, D’Urso A, Toiber D, Sebastian C, Henry RE, Vadysirisack DD, et al. The histone deacetylase Sirt6 regulates glucose homeostasis via Hif1alpha. Cell 2010; 140:280 - 93; http://dx.doi.org/10.1016/j.cell.2009.12.041; PMID: 20141841
- Gan L, Mucke L. Paths of convergence: sirtuins in aging and neurodegeneration. Neuron 2008; 58:10 - 4; http://dx.doi.org/10.1016/j.neuron.2008.03.015; PMID: 18400158
- Michishita E, McCord RA, Boxer LD, Barber MF, Hong T, Gozani O, et al. Cell cycle-dependent deacetylation of telomeric histone H3 lysine K56 by human SIRT6. Cell Cycle 2009; 8:2664 - 6; http://dx.doi.org/10.4161/cc.8.16.9367; PMID: 19625767
- Yang B, Zwaans BM, Eckersdorff M, Lombard DB. The sirtuin SIRT6 deacetylates H3 K56Ac in vivo to promote genomic stability. Cell Cycle 2009; 8:2662 - 3; http://dx.doi.org/10.4161/cc.8.16.9329; PMID: 19597350
- Liszt G, Ford E, Kurtev M, Guarente L. Mouse Sir2 homolog SIRT6 is a nuclear ADP-ribosyltransferase. J Biol Chem 2005; 280:21313 - 20; http://dx.doi.org/10.1074/jbc.M413296200; PMID: 15795229
- Frye RA. Characterization of five human cDNAs with homology to the yeast SIR2 gene: Sir2-like proteins (sirtuins) metabolize NAD and may have protein ADP-ribosyltransferase activity. Biochem Biophys Res Commun 1999; 260:273 - 9; http://dx.doi.org/10.1006/bbrc.1999.0897; PMID: 10381378
- Michishita E, Park JY, Burneskis JM, Barrett JC, Horikawa I. Evolutionarily conserved and nonconserved cellular localizations and functions of human SIRT proteins. Mol Biol Cell 2005; 16:4623 - 35; http://dx.doi.org/10.1091/mbc.E05-01-0033; PMID: 16079181
- Straight AF, Shou W, Dowd GJ, Turck CW, Deshaies RJ, Johnson AD, et al. Net1, a Sir2-associated nucleolar protein required for rDNA silencing and nucleolar integrity. Cell 1999; 97:245 - 56; http://dx.doi.org/10.1016/S0092-8674(00)80734-5; PMID: 10219245
- Shen HB, Chou KC. Nuc-PLoc: a new web-server for predicting protein subnuclear localization by fusing PseAA composition and PsePSSM. Protein Eng Des Sel 2007; 20:561 - 7; http://dx.doi.org/10.1093/protein/gzm057; PMID: 17993650
- Li LH, Wu LJ, Tashiro SI, Onodera S, Uchiumi F, Ikejima T. Activation of the SIRT1 pathway and modulation of the cell cycle were involved in silymarin’s protection against UV-induced A375-S2 cell apoptosis. J Asian Nat Prod Res 2007; 9:245 - 52; http://dx.doi.org/10.1080/10286020600604260; PMID: 17566917
- Scott MS, Boisvert FM, McDowall MD, Lamond AI, Barton GJ. Characterization and prediction of protein nucleolar localization sequences. Nucleic Acids Res 2010; 38:7388 - 99; http://dx.doi.org/10.1093/nar/gkq653; PMID: 20663773
- Dephoure N, Zhou C, Villén J, Beausoleil SA, Bakalarski CE, Elledge SJ, et al. A quantitative atlas of mitotic phosphorylation. Proc Natl Acad Sci U S A 2008; 105:10762 - 7; http://dx.doi.org/10.1073/pnas.0805139105; PMID: 18669648
- Okuwaki M, Tsujimoto M, Nagata K. The RNA binding activity of a ribosome biogenesis factor, nucleophosmin/B23, is modulated by phosphorylation with a cell cycle-dependent kinase and by association with its subtype. Mol Biol Cell 2002; 13:2016 - 30; http://dx.doi.org/10.1091/mbc.02-03-0036; PMID: 12058066
- Hernandez-Verdun D. Assembly and disassembly of the nucleolus during the cell cycle. Nucleus 2011; 2:189 - 94; http://dx.doi.org/10.4161/nucl.2.3.16246; PMID: 21818412
- Michan S, Sinclair D. Sirtuins in mammals: insights into their biological function. Biochem J 2007; 404:1 - 13; http://dx.doi.org/10.1042/BJ20070140; PMID: 17447894
- Vaquero A, Scher M, Erdjument-Bromage H, Tempst P, Serrano L, Reinberg D. SIRT1 regulates the histone methyl-transferase SUV39H1 during heterochromatin formation. Nature 2007; 450:440 - 4; http://dx.doi.org/10.1038/nature06268; PMID: 18004385
- Vaquero A, Scher M, Lee D, Erdjument-Bromage H, Tempst P, Reinberg D. Human SirT1 interacts with histone H1 and promotes formation of facultative heterochromatin. Mol Cell 2004; 16:93 - 105; http://dx.doi.org/10.1016/j.molcel.2004.08.031; PMID: 15469825
- Boisvert FM, van Koningsbruggen S, Navascués J, Lamond AI. The multifunctional nucleolus. Nat Rev Mol Cell Biol 2007; 8:574 - 85; http://dx.doi.org/10.1038/nrm2184; PMID: 17519961
- Carmo-Fonseca M, Mendes-Soares L, Campos I. To be or not to be in the nucleolus. Nat Cell Biol 2000; 2:E107 - 12; http://dx.doi.org/10.1038/35014078; PMID: 10854340
- Cioci F, Vogelauer M, Camilloni G. Acetylation and accessibility of rDNA chromatin in Saccharomyces cerevisiae in (Delta)top1 and (Delta)sir2 mutants. J Mol Biol 2002; 322:41 - 52; http://dx.doi.org/10.1016/S0022-2836(02)00749-0; PMID: 12215413
- Shou W, Seol JH, Shevchenko A, Baskerville C, Moazed D, Chen ZW, et al. Exit from mitosis is triggered by Tem1-dependent release of the protein phosphatase Cdc14 from nucleolar RENT complex. Cell 1999; 97:233 - 44; http://dx.doi.org/10.1016/S0092-8674(00)80733-3; PMID: 10219244
- Andersen JS, Lam YW, Leung AK, Ong SE, Lyon CE, Lamond AI, et al. Nucleolar proteome dynamics. Nature 2005; 433:77 - 83; http://dx.doi.org/10.1038/nature03207; PMID: 15635413
- Espada J, Ballestar E, Santoro R, Fraga MF, Villar-Garea A, Németh A, et al. Epigenetic disruption of ribosomal RNA genes and nucleolar architecture in DNA methyltransferase 1 (Dnmt1) deficient cells. Nucleic Acids Res 2007; 35:2191 - 8; http://dx.doi.org/10.1093/nar/gkm118; PMID: 17355984
- Gonugunta VK, Nair BC, Rajhans R, Sareddy GR, Nair SS, Vadlamudi RK. Regulation of rDNA transcription by proto-oncogene PELP1. PLoS One 2011; 6:e21095; http://dx.doi.org/10.1371/journal.pone.0021095; PMID: 21695158
- Kobayashi T. How does genome instability affect lifespan?: roles of rDNA and telomeres. Genes Cells 2011; 16:617 - 24; http://dx.doi.org/10.1111/j.1365-2443.2011.01519.x; PMID: 21605287
- Lempiäinen H, Shore D. Growth control and ribosome biogenesis. Curr Opin Cell Biol 2009; 21:855 - 63; http://dx.doi.org/10.1016/j.ceb.2009.09.002; PMID: 19796927
- Visintin R, Amon A. The nucleolus: the magician’s hat for cell cycle tricks. Curr Opin Cell Biol 2000; 12:752; http://dx.doi.org/10.1016/S0955-0674(00)00165-4; PMID: 11063944
- Shandilya J, Swaminathan V, Gadad SS, Choudhari R, Kodaganur GS, Kundu TK. Acetylated NPM1 localizes in the nucleoplasm and regulates transcriptional activation of genes implicated in oral cancer manifestation. Mol Cell Biol 2009; 29:5115 - 27; http://dx.doi.org/10.1128/MCB.01969-08; PMID: 19581289
- North BJ, Verdin E. Interphase nucleo-cytoplasmic shuttling and localization of SIRT2 during mitosis. PLoS One 2007; 2:e784; http://dx.doi.org/10.1371/journal.pone.0000784; PMID: 17726514
- Dryden SC, Nahhas FA, Nowak JE, Goustin AS, Tainsky MA. Role for human SIRT2 NAD-dependent deacetylase activity in control of mitotic exit in the cell cycle. Mol Cell Biol 2003; 23:3173 - 85; http://dx.doi.org/10.1128/MCB.23.9.3173-3185.2003; PMID: 12697818
- Kim HS, Vassilopoulos A, Wang RH, Lahusen T, Xiao Z, Xu X, et al. SIRT2 maintains genome integrity and suppresses tumorigenesis through regulating APC/C activity. Cancer Cell 2011; 20:487 - 99; http://dx.doi.org/10.1016/j.ccr.2011.09.004; PMID: 22014574
- Fatoba ST, Okorokov AL. Human SIRT1 associates with mitotic chromatin and contributes to chromosomal condensation. Cell Cycle 2011; 10:2317 - 22; http://dx.doi.org/10.4161/cc.10.14.15913; PMID: 21636977
- Manser E, Huang HY, Loo TH, Chen XQ, Dong JM, Leung T, et al. Expression of constitutively active alpha-PAK reveals effects of the kinase on actin and focal complexes. Mol Cell Biol 1997; 17:1129 - 43; PMID: 9032240
- Liang F, Hatanaka Y, Saito H, Yamamori T, Hashikawa T. Differential expression of gamma-aminobutyric acid type B receptor-1a and -1b mRNA variants in GABA and non-GABAergic neurons of the rat brain. J Comp Neurol 2000; 416:475 - 95; http://dx.doi.org/10.1002/(SICI)1096-9861(20000124)416:4<475::AID-CNE5>3.0.CO;2-V; PMID: 10660879