Abstract
Cellular stresses significantly affect nuclear transport systems. Nuclear transport pathways mediated by importin β-family members, which are active under normal conditions, are downregulated. During thermal stress, a nuclear import pathway mediated by a novel carrier, which we named Hikeshi, becomes active. Hikeshi is not a member of the importin β family and mediates the nuclear import of Hsp70s. Unlike importin β family-mediated nuclear transport, the Hikeshi-mediated nuclear import of Hsp70s is not coupled to the GTPase cycle of the small GTPase Ran but rather is coupled with the ATPase cycle of Hsp70s. Hikeshi-mediated nuclear import is essential for the attenuation and reversal of the thermal stress response in human cells. The mechanism and functions of this newly identified nuclear import pathway will be discussed.
Introduction
In eukaryotic cells, hundreds of molecules are exchanged between the nucleus and the cytoplasm every minute. This process, called nucleocytoplasmic transport, is crucial not only for basic cellular activities but also for regulating various cellular events. Based on the literatures and database information, we can estimate that as much as ~30% of the proteins expressed in cells are nuclear proteins,Citation1 indicating that the nucleocytoplasmic transport is the major intracellular trafficking pathway in terms of the quantity and diversity of molecules that are transported.
To enter and exit the nucleus, molecules must translocate through nuclear pore complexes (NPCs), which are large protein assemblies that are embedded in the nuclear envelope.Citation2-Citation4 NPCs allow the passive diffusion of small molecules, such as ions and proteins smaller than ~30 KDa. However, larger molecules must bind to a nucleocytoplasmic transport carrier; these are typically hydrophobic because the transport channel of the NPCs is hydrophobic.Citation5 The best-characterized transport carriers are the members of the importin β family. Theses proteins are conserved from yeast to mammals and are considered to facilitate the nuclear transport of most proteins and many different RNAs.
Since 1995, when the first nuclear import carrier (importin β) was identified,Citation6-Citation8 our understanding of the basic mechanism of nucleocytoplasmic transport has advanced significantly.Citation9-Citation12 One key feature of this transport is that cargoes can continue to accumulate in one compartment against a chemical concentration gradient, i.e., from the cytoplasm to the nucleus or from the nucleus to the cytoplasm. To achieve this, carriers bind to cargo in one compartment, translocate through NPCs and dissociate from the cargo in the target compartment. The GTPase cycle of the small GTPase Ran is coupled with importin β-mediated transport pathways and plays a crucial role in the cargo binding and release that occurs in the nucleus or in the cytoplasm. Each nuclear import or export cycle consumes one GTP hydrolyzed by Ran, which serves as a driving force of the transport. To date, studies of nuclear protein import or export have focused almost exclusively on the Importin-Ran system, and the different transport pathways have not been identified/investigated.
Recently, we identified a transport pathway that is mediated by a novel carrier protein, Hikeshi, that becomes active during the thermal stress.Citation13 Hikeshi does not belong to the importin β, and it is evolutionarily conserved from yeast to mammals. Hikeshi-medited trasnport does not use the Ran system, but likely uses the ATPase cycle of the molecular chaperone Hsp70 as a driving force. The mechanism and physiological significance of Hikeshi-mediated nuclear import will be discussed.
Environmental Stresses Affect the Nuclear Transport
Upon exposure to environmental stresses, cells respond by altering many aspects of cellular physiology to protect cells from stress damage. After release from stresses, cells must attenuate the stress response and restore normal activities to survive. A shift in the temperature from the physiological state (heat shock) causes protein misfolding, protein dysfunction or protein aggregation, and thus perturbs protein homeostasis.Citation14 In response to heat shock, one prominent phenomenon observed in cells is the increase in the cellular level of molecular chaperones known as heat-shock proteins (HSPs), which play essential roles in maintaining protein homeostasis.Citation15 In addition to heat shock, a large variety of environmental stresses are known to induce the expression of HSPs.Citation16,Citation17 Therefore, the heat-shock response is considered synonymous with the cellular stress response. In addition, among many other stresses, heat-shock stress is most susceptible to reversion from stress damage within a short time frame. Heat-shock stress is therefore an excellent model system in which to study a cellular stress response, as well as the recovery of cells from stress.
During stresses, normal transcription and translation are downregulated, whereas stress-specific mechanisms are upregulated.Citation18,Citation19 Little was known about the nuclear transport during stress; however, several groups reported that stresses, such as heat-shock and oxidative stresses, induce nuclear retention and inhibition of the nuclear export of importin α, an adaptor molecule that connects classical nuclear localization signals (NLSs) to importin β, perturbing the importin α/importin β pathway.Citation20,Citation21 Furthermore, in yeast and in mammalian cells, different stresses induce the cytoplasmic localization of Ran, implying perturbation of the Ran GTPase cycle, which could negatively affect all pathways mediated by importin β family members.Citation22-Citation24 On the other hand, it was known for nearly 30 y that the major molecular chaperone Hsp70/Hsc70 (Hsp70s) strongly accumulates in the nucleus in response to heat shock.Citation25-Citation28 However, neither the mechanism of its nuclear accumulation nor its nuclear function was known. Studies using microinjection and reconstituted transport showed that the nuclear import activity of Hsp70s is upregulated during thermal stressCitation13,Citation20(). This evidence indicates that cellular stress significantly affects the nuclear transport system: the conventional nuclear transport pathway is downregulated, whereas stress-specific nuclear transport becomes active ().
Figure 1. Heat-shock stress downregulates the conventional nuclear transport pathway but upregulates the nuclear import of Hsp70s. (A) Nuclear import of importin β family cargoes or Hsc70 was examined in living cells by microinjection (left panels) or in reconstituted transport assay using digitonin-permeabilized semi-intact cells (right panels). In living cells, SV40 T-NLS (Importin β cargo) efficiently accumulates in the nucleus whereas Hsc70s do not under normal condition. In contrast, Hsc70s accumulate efficiently in the nucleus whereas SV40 T-NLS does not under the heat shock condition. Results of 5min after cytoplasmic injection are shown. In reconstituted transport, SV40 T-NLS and M9 (transportin cargo) accumulate efficiently in the nucleus of permeabilized cells whereas Hsc70 do not in the presence of cytosol prepared from normal cells. In contrast, Hsc70s accumulate efficiently in the nucleus of permeabilized cells whereas SV40 T-NLS and M9 do not in the presence of cytosol prepared from heat shock treated cells. (B) Illustration of nuclear transport under normal condition and heat shock stress condition.
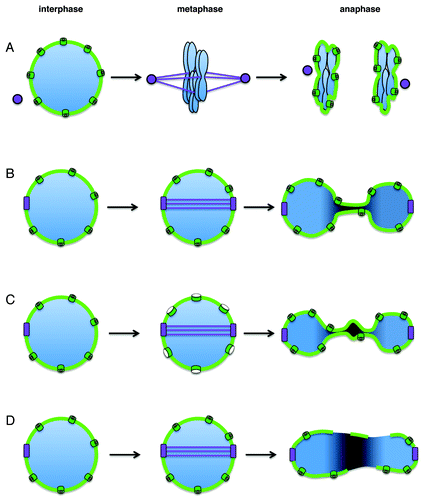
The Search for a Factor that Mediates the Stress-Induced Nuclear Import of Hsp70s
What mediates the nuclear import of Hsp70s under stress conditions when importin β family member-mediated nuclear transport is downregulated? To answer this question, we initially reconstituted heat shock-induced nuclear import using a cell-free transport assay using digitonin-permeabilized semi-intact cells.Citation29 Because Hsp70 is a “sticky” protein, we could not identify its interaction partner molecule(s) required for its nuclear import via a simple binding assay (e.g., pull-down assays or immunoprecipitation experiments). In the reconstituted transport experiment, a combination of cytosol or permeabilized cells prepared from either normal or heat shock-stressed HeLa cells revealed that the nuclear import activity of Hsp70s was present in the cytosol prepared from stressed cells.Citation13 The biochemical fractionation of the cytosol prepared from stressed cells, followed by an examination of the nuclear import activity of Hsc70 in the cell-free transport assay, identified a protein encoded by human chromosome 11 open reading frame 73 (C11orf73) as a factor essential for the nuclear import of Hsp70s.Citation13 C11orf73 encodes a protein that is evolutionarily conserved from yeast to mammals, but its function was unknown. Bacterially expressed recombinant C11orf73 protein supported the nuclear import of Hsc70 in the cell-free transport assay, and the knockdown of C11orf73 using siRNA inhibited the heat shock-induced nuclear import of Hsp70s in living cells, demonstrating the essential role of C11orf73 in the nuclear import of Hsp70s. Based on the cellular function described below, we renamed C11orf73 as Hikeshi.
Identification of Hikeshi as a Nuclear Import Carrier
Although it was evident that Hikeshi is essential for the heat shock-induced nuclear import of Hsp70s, our primary question was whether Hikeshi functions as a nuclear import carrier. If Hikeshi is a nuclear import carrier, it must bind to NPC components (nucleoporins) and be able to translocate through nuclear pore complexes, as all known nuclear transport carriers do.Citation30-Citation33 It was also important that the binding between Hikeshi and Hsp70s be regulated to allow the nuclear accumulation of Hsp70s against a chemical concentration gradient. In the case of importin β, the GTPase cycle of Ran plays a crucial role in this active transport: cargoes bind to importin β in the cytoplasm where the RanGTP concentration is low but dissociate in the nucleus where RanGTP concentration is high because RanGTP binding to importin β triggers the cargo release.Citation9-Citation12 We therefore examined whether Hikeshi fulfills the above two criteria of a carrier: first, its ability to translocate through NPCs through interactions with FG-repeat containing nucleoporins (FG-Nups), and second, the regulation of its binding to Hsp70s.
Hikeshi translocates through NPCs and interacts with FG-Nups
GFP-Hikeshi, when expressed in living cells, localized diffusively throughout the cytoplasm and the nucleus. When incubated with digitonin-permeabilized semi-intact cells, GFP-Hikeshi enters the nucleus in the absence of soluble factors or energy sources, showing that it is capable of translocating through NPCs on its own. This translocation was inhibited by the addition of importin β or wheat germ agglutinin (WGA),Citation30 indicating that its nuclear migration occurs through a specific interaction with nucleoporins. Although the details of NPC translocation are currently not understood, it is now widely accepted that all known transport carriers translocate through the central channel of NPCs, which are filled with hydrophobic FXFG- or GLFG-repeats (FG-repeats), and that translocation proceeds through interactions between the carriers and FG repeats of nucleoporins.Citation5,Citation10,Citation11 When examined in a Bead Hallo assay,Citation34 Hikeshi bound to FG-repeats containing nucleoporins, and this binding was inhibited by importin β, indicating that Hikeshi translocate through NPCs by interacting with the FG repeats of nucleoporins in a manner similar to that of importin β.
Co-chaperones regulates the binding of Hikeshi to Hsp70s
Demonstrating the physical interaction of Hikeshi and Hsp70s was initially challenging. Hikeshi bound to Hsp70s in a pull-down assay from cell extract, and it mediated the nuclear import of Hsc70s in the presence of cell extract.Citation13 Interestingly, the interaction of Hikeshi with Hsp70s in the crude cell extract and the Hikeshi-dependent transport of Hsp70 always depended on the presence of ATP. However, Hikeshi neither supported the nuclear import of Hsc70 nor bound to Hsp70s in the absence of cell extract, even in the presence of ATP. These observations showed that Hikeshi and Hsp70s do associate with each other but suggest that their binding requires some soluble factor(s) in conjunction with ATP. To identify the soluble factor(s) necessary for the Hikeshi-Hsp70 interaction, we again performed biochemical fractionation and followed the nuclear import of Hsp70 in the presence of Hikeshi in cell-free transport assay. We identified Hsp110 family members as the cofactors required for Hsp70 import by Hikeshi. Hsp110 is a co-chaperone of Hsp70, which functions as a nucleotide exchange factor of Hsp70s.
In cells, Hsp70s do not function alone, but always function with co-chaperones that promote the ATPase cycle of Hsp70s.Citation35,Citation36 For example, Hsp70s nucleotide exchange factors, such as Hsp110, convert the ADP-bound form of Hsp70 to the ATP-bound form, whereas J-domain proteins such as Hsp40 convert the ATP-bound form to the ADP-bound form. To better characterize the involvement of the ATPase cycle of Hsp70s in Hikeshi-binding/transport, we examined the effects of co-chaperones on the Hikeshi-mediated nuclear import of Hsc70. Hsc70 was first pre-incubated with Hsp110 in the presence of ATP and then subjected to the transport assay after the removal of Hsp110. We found that Hikeshi was able to mediate the nuclear import of the pre-incubated Hsc70 in the absence of soluble factors. Furthermore, in the absence of other soluble factors, Hikeshi mediated the transport of an ATPase-deficient point mutated Hsp70Citation37 (ATP-fixed form) even without pre-incubation with Hsp110. Conversely, the addition of Hsp40 disrupted the binding of Hikeshi and Hsp70s and inhibited the nuclear import of Hsc70 mediated by Hikeshi. These results show that Hikeshi binds to the ATP-bound form of Hsp70s but dissociate from the ADP-bound formCitation13 (). If the binding occurs in the cytoplasm and dissociation occurs in the nucleoplasm, then this property could explain the directionality of the transport.
Figure 2. Regulation of the binding of Hikeshi to Hsp70s and the transport model. (A) Binding and release of Hikeshi to Hsp70s is regulated by co-chaperones that modulate the nucleotide form of Hsp70s. Hikeshi binds to the ATP-bound form of Hsp70s, but dissociates from the ADP-bound form. (B) Current working model of the Hikeshi-mediated nuclear import of Hsp70s. In the cytoplasm, Hikeshi binds to the ATP-bound form Hsp70s, translocate through the NPCs. In the nucleus, Hikeshi dissociates from the ADP-bound form Hsp70s by action of J-domain-containing co-chaperones, such as the Hsp40 family, allowing Hsp70s to function as a molecular chaperone in the nucleus.
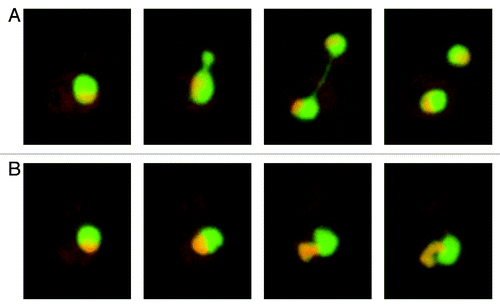
Taken together, these results show that Hikeshi fulfills the criteria of a nuclear transport carrier. Our current working model of Hikeshi-mediated nuclear import pathway is depicted in . Importantly, our transport model highlights the involvement of co-chaperones in the Hikeshi-mediated nuclear import of Hsp70s. It must be noted that there exist many different co-chaperones in cells that are involved in either nucleotide exchange or ATP hydrolysis of Hsp70s. It is possible that other groups of co-chaperones, besides co-chaperones used in this study, participate in driving import of Hsp70s mediated by Hikeshi. It is important to determine which co-chaperones (or groups of co-chaperone) that are involved in the transport during stress to verify our model through careful analysis of their behaviors and regulation of activities during normal condition and stress condition. In any case, the proposed transport pathway is different from any of the known transport pathway reported previously, not only because it is mediated by a novel carrier but also because its driving force appears to be the ATPase cycle of Hsp70s, which is unique among reported nuclear transport pathways.
Physiological Significance of Hikeshi-Mediated Nuclear Import
To examine the physiological significance of Hikeshi-mediated nuclear import, we first examined the cellular effects of Hikeshi depletion using siRNAs.Citation13 Hikeshi is expressed in normal cells, and its expression level increases by two- to 3-fold during thermal stress in HeLa cells. Hikeshi depletion apparently does not affect cell growth under normal condition: however, more than 70% of cells failed to grow after heat treatment when Hikeshi is depleted (). Such growth defects were rescued, at least in part, by expressing conventional basic NLS-tagged Hsc70, which is imported into the nucleus by the importin α and importin β pathways, just before the heat shock treatment. The results confirm for the first time in the 30 y since the initial report of the stress-induced nuclear accumulation of Hsp70s, that the presence of Hsp70s in the nucleus during thermal stress is indeed important for cells to survive after stress.
Figure 3. Hikeshi-mediated nuclear import is required to protect cells from heat shock damage. (A) siRNA-mediated Hikeshi knockdown inhibits the heat shock-induced nuclear accumulation of Hsp70s in living cells. (B) siRNA-mediated Hikeshi knockdown significantly reduces the cell viability after release from heat shock stress. Time course experiments show that the Hikeshi-depleted cells start dying several hours after the release from stress. T shows the time (hr) after the temperature shift-down to the physiological temperature. This effect of Hikeshi-depletion was rescued for about 50%, by expressing conventional basic NLS tagged Hsc70 just before the heat-shock treatment.
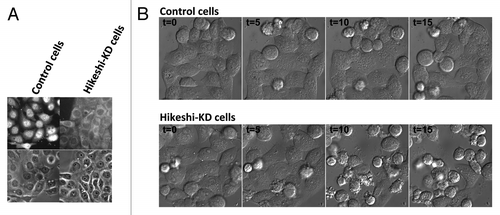
What causes cell death in the Hikeshi-depleted cells? In time course experiments, we noted that cells do not die immediately after exposure to stress but rather begin dying several hours after release from stress, indicating that the cells die during recovery from stress.Citation13 Consistent with this observation, in Hikeshi-depleted cells, the cellular levels of Hsp70 rapidly increases as in normal cells in response to heat shock, indicating that the Hikeshi-depleted cells respond to heat shock normally. However, unlike normal cells, the expression of Hsp70 does not decrease after the cells are returned to physiological temperature. In a parallel observation, nuclear structure called nuclear stress granules (nSGs), which are considered to represent the activity of the heat shock factors (HSFs) that activate Hsp70s expression,Citation38,Citation39 rapidly appear in both Hikeshi-depleted cells and normal cells in response to heat shock, again showing that the Hikeshi-depleted cells can respond to heat shock normally. However, nSGs, which rapidly disappear in normal cells soon after a temperature shift-down persist for much longer time in the Hikeshi-depleted cells, which is consistent with the persistently high expression levels of Hsp70 after temperature shift down. Our results show that the heat-shock response cannot be attenuated in Hikeshi-depleted cells even after a release from stress. Surprisingly, the effect of the Hikeshi depletion was not restricted to the attenuation of HSF activity but also seemed to have broader effects on the reversion of the heat shock-induced nuclear phenotype. For example, function and structure of nucleolus is affected with various cellular stresses.Citation40,Citation41 It is known that some nucleolar proteins are released from the nucleolus and dispersed into the nucleoplasm in response to heat shock, but they re-accumulate in the nucleolus after release from stress.Citation42-Citation44 This re-accumulation was not observed in the Hikeshi-depleted cells.Citation13
All of the above observations show that Hikeshi is required to protect cells from heat shock damage and is required for the attenuation and reversion of multiple heat shock-induced nuclear phenotypes. These functions are reminiscent of “Hikeshi,” which is the traditional Edo-era Japanese compound word meaning firefighter, smokejumper or troubleshooter. Our results provide evidence for the physiological significance of Hikeshi-mediated nuclear import pathway activated during thermal stress.
Perspectives
Revealing a nuclear transport pathway that becomes active during thermal stress will raise fundamental new questions. For example, in spite of many reports demonstrating that stresses affect numerous nuclear events involving DNA metabolism and RNA biogenesis, which must be reverted upon release from stresses in order for cells to survive, the mechanisms underlying the reversion of stress-induced nuclear phenotypes have not been studied in depth to date. Our findings clearly show that there are active mechanism(s) for the reversion of the thermal stress-induced nuclear phenotype and that the activity of Hsp70s inside the nucleus is crucial, at least in part for this reversion. Because the depletion of Hikeshi seems to affect the reversion of various nuclear phenotypes, it is possible that the Hikeshi mediates nuclear import of proteins other than Hsp70s that are also responsible for reverting stress-induced nuclear phenotypes. Finding of Hikeshi-mediated nuclear transport pathway will provide a new avenue for research to address questions regarding how cells recover from thermal stress damage.
Another intriguing question to address is the molecular mechanism underlying the activation of Hikeshi-mediated nuclear import. Preliminary analysis shows that, at least in a reconstituted system, posttranslational protein modifications such as the phosphorylation of Hikeshi or Hsp70s are not involved in the activation of Hikeshi-mediated nuclear import. Because the ATPase cycle of Hsp70s, which is regulated by co-chaperones, is likely involved in the Hikeshi-mediated nuclear import, the import activation mechanism might involve the regulation of the chaperone system. Further study of the nuclear transport switching mechanism should provide new insights into the regulation of both the nuclear transport system and the molecular chaperone system. In addition, because Hikeshi is evolutionarily conserved protein from yeast to mammals, it is also important to analyze the functions of Hikeshi in other organisms to know the conservation and divergence of nuclear transport system and molecular chaperone system from aspects of evolution.
Acknowledgments
We thank members of the Cellular Dynamics Lab for helpful comments and discussions. This work was supported by RIKEN Special Funding for Cellular System, ASI FY2012 Fund for Seeds of Collaborative Research to S.K., MEXT grant-in-aids to S.K., and funding awarded to N.I. by the Japan Society for the Promotion of Science (JSPS) through the “Funding Program for Next Generation World-Leading Researchers (NEXT Program),” initiated by the Council for Science and Technology Policy (CSTP).
References
- Simpson JC, Wellenreuther R, Poustka A, Pepperkok R, Wiemann S. Systematic subcellular localization of novel proteins identified by large-scale cDNA sequencing. EMBO Rep 2000; 1:287 - 92; http://dx.doi.org/10.1093/embo-reports/kvd058; PMID: 11256614
- Hoelz A, Debler EW, Blobel G. The structure of the nuclear pore complex. Annu Rev Biochem 2011; 80:613 - 43; http://dx.doi.org/10.1146/annurev-biochem-060109-151030; PMID: 21495847
- Wente SR, Rout MP. The nuclear pore complex and nuclear transport. Cold Spring Harb Perspect Biol 2010; 2:a000562; http://dx.doi.org/10.1101/cshperspect.a000562; PMID: 20630994
- Imamoto N, Funakoshi T. Nuclear pore dynamics during the cell cycle. Curr Opin Cell Biol 2012; 24:453 - 9; http://dx.doi.org/10.1016/j.ceb.2012.06.004; PMID: 22770730
- Ribbeck K, Görlich D. The permeability barrier of nuclear pore complexes appears to operate via hydrophobic exclusion. EMBO J 2002; 21:2664 - 71; http://dx.doi.org/10.1093/emboj/21.11.2664; PMID: 12032079
- Chi NC, Adam EJ, Adam SA. Sequence and characterization of cytoplasmic nuclear protein import factor p97. J Cell Biol 1995; 130:265 - 74; http://dx.doi.org/10.1083/jcb.130.2.265; PMID: 7615630
- Imamoto N, Shimamoto T, Kose S, Takao T, Tachibana T, Matsubae M, et al. The nuclear pore-targeting complex binds to nuclear pores after association with a karyophile. FEBS Lett 1995; 368:415 - 9; http://dx.doi.org/10.1016/0014-5793(95)00699-A; PMID: 7635189
- Görlich D, Kostka S, Kraft R, Dingwall C, Laskey RA, Hartmann E, et al. Two different subunits of importin cooperate to recognize nuclear localization signals and bind them to the nuclear envelope. Curr Biol 1995; 5:383 - 92; http://dx.doi.org/10.1016/S0960-9822(95)00079-0; PMID: 7627554
- Görlich D, Kutay U. Transport between the cell nucleus and the cytoplasm. Annu Rev Cell Dev Biol 1999; 15:607 - 60; PMID: 10611974
- Weis K. Regulating access to the genome: nucleocytoplasmic transport throughout the cell cycle. Cell 2003; 112:441 - 51; http://dx.doi.org/10.1016/S0092-8674(03)00082-5; PMID: 12600309
- Stewart M. Molecular mechanism of the nuclear protein import cycle. Nat Rev Mol Cell Biol 2007; 8:195 - 208; http://dx.doi.org/10.1038/nrm2114; PMID: 17287812
- Chook YM, Süel KE. Nuclear import by karyopherin-βs: recognition and inhibition. Biochim Biophys Acta 2011; 1813:1593 - 606; http://dx.doi.org/10.1016/j.bbamcr.2010.10.014; PMID: 21029754
- Kose S, Furuta M, Imamoto N. Hikeshi, a nuclear import carrier for Hsp70s, protects cells from heat shock-induced nuclear damage. Cell 2012; 149:578 - 89; http://dx.doi.org/10.1016/j.cell.2012.02.058; PMID: 22541429
- Richter K, Haslbeck M, Buchner J. The heat shock response: life on the verge of death. Mol Cell 2010; 40:253 - 66; http://dx.doi.org/10.1016/j.molcel.2010.10.006; PMID: 20965420
- Akerfelt M, Morimoto RI, Sistonen L. Heat shock factors: integrators of cell stress, development and lifespan. Nat Rev Mol Cell Biol 2010; 11:545 - 55; http://dx.doi.org/10.1038/nrm2938; PMID: 20628411
- Morimoto RI. Regulation of the heat shock transcriptional response: cross talk between a family of heat shock factors, molecular chaperones, and negative regulators. Genes Dev 1998; 12:3788 - 96; http://dx.doi.org/10.1101/gad.12.24.3788; PMID: 9869631
- Morimoto RI. Proteotoxic stress and inducible chaperone networks in neurodegenerative disease and aging. Genes Dev 2008; 22:1427 - 38; http://dx.doi.org/10.1101/gad.1657108; PMID: 18519635
- Lackner DH, Bähler J. Translational control of gene expression from transcripts to transcriptomes. Int Rev Cell Mol Biol 2008; 271:199 - 251; http://dx.doi.org/10.1016/S1937-6448(08)01205-7; PMID: 19081544
- Mimnaugh EG, Chen HY, Davie JR, Celis JE, Neckers L. Rapid deubiquitination of nucleosomal histones in human tumor cells caused by proteasome inhibitors and stress response inducers: effects on replication, transcription, translation, and the cellular stress response. Biochemistry 1997; 36:14418 - 29; http://dx.doi.org/10.1021/bi970998j; PMID: 9398160
- Furuta M, Kose S, Koike M, Shimi T, Hiraoka Y, Yoneda Y, et al. Heat-shock induced nuclear retention and recycling inhibition of importin α. Genes Cells 2004; 9:429 - 41; http://dx.doi.org/10.1111/j.1356-9597.2004.00734.x; PMID: 15147272
- Miyamoto Y, Saiwaki T, Yamashita J, Yasuda Y, Kotera I, Shibata S, et al. Cellular stresses induce the nuclear accumulation of importin α and cause a conventional nuclear import block. J Cell Biol 2004; 165:617 - 23; http://dx.doi.org/10.1083/jcb.200312008; PMID: 15184398
- Czubryt MP, Austria JA, Pierce GN. Hydrogen peroxide inhibition of nuclear protein import is mediated by the mitogen-activated protein kinase, ERK2. J Cell Biol 2000; 148:7 - 16; http://dx.doi.org/10.1083/jcb.148.1.7; PMID: 10629214
- Kelley JB, Paschal BM. Hyperosmotic stress signaling to the nucleus disrupts the Ran gradient and the production of RanGTP. Mol Biol Cell 2007; 18:4365 - 76; http://dx.doi.org/10.1091/mbc.E07-01-0089; PMID: 17761537
- Kodiha M, Chu A, Matusiewicz N, Stochaj U. Multiple mechanisms promote the inhibition of classical nuclear import upon exposure to severe oxidative stress. Cell Death Differ 2004; 11:862 - 74; http://dx.doi.org/10.1038/sj.cdd.4401432; PMID: 15088071
- Lamian V, Small GM, Feldherr CM. Evidence for the existence of a novel mechanism for the nuclear import of Hsc70. Exp Cell Res 1996; 228:84 - 91; http://dx.doi.org/10.1006/excr.1996.0302; PMID: 8892974
- Pelham HR. Hsp70 accelerates the recovery of nucleolar morphology after heat shock. EMBO J 1984; 3:3095 - 100; PMID: 6441707
- Velazquez JM, Lindquist S. hsp70: nuclear concentration during environmental stress and cytoplasmic storage during recovery. Cell 1984; 36:655 - 62; http://dx.doi.org/10.1016/0092-8674(84)90345-3; PMID: 6421488
- Welch WJ, Feramisco JR. Nuclear and nucleolar localization of the 72,000-dalton heat shock protein in heat-shocked mammalian cells. J Biol Chem 1984; 259:4501 - 13; PMID: 6368558
- Adam SA, Marr RS, Gerace L. Nuclear protein import in permeabilized mammalian cells requires soluble cytoplasmic factors. J Cell Biol 1990; 111:807 - 16; http://dx.doi.org/10.1083/jcb.111.3.807; PMID: 2391365
- Kose S, Imamoto N, Tachibana T, Shimamoto T, Yoneda Y. Ran-unassisted nuclear migration of a 97-kD component of nuclear pore-targeting complex. J Cell Biol 1997; 139:841 - 9; http://dx.doi.org/10.1083/jcb.139.4.841; PMID: 9362503
- Nakielny S, Dreyfuss G. Import and export of the nuclear protein import receptor transportin by a mechanism independent of GTP hydrolysis. Curr Biol 1998; 8:89 - 95; http://dx.doi.org/10.1016/S0960-9822(98)70039-9; PMID: 9427645
- Nachury MV, Weis K. The direction of transport through the nuclear pore can be inverted. Proc Natl Acad Sci U S A 1999; 96:9622 - 7; http://dx.doi.org/10.1073/pnas.96.17.9622; PMID: 10449743
- Kose S, Imamoto N, Tachibana T, Yoshida M, Yoneda Y. β-subunit of nuclear pore-targeting complex (importin-β) can be exported from the nucleus in a Ran-independent manner. J Biol Chem 1999; 274:3946 - 52; http://dx.doi.org/10.1074/jbc.274.7.3946; PMID: 9933584
- Patel SS, Rexach MF. Discovering novel interactions at the nuclear pore complex using bead halo: a rapid method for detecting molecular interactions of high and low affinity at equilibrium. Mol Cell Proteomics 2008; 7:121 - 31; http://dx.doi.org/10.1074/mcp.M700407-MCP200; PMID: 17897934
- Kampinga HH, Craig EA. The HSP70 chaperone machinery: J proteins as drivers of functional specificity. Nat Rev Mol Cell Biol 2010; 11:579 - 92; http://dx.doi.org/10.1038/nrm2941; PMID: 20651708
- Mayer MP. Gymnastics of molecular chaperones. Mol Cell 2010; 39:321 - 31; http://dx.doi.org/10.1016/j.molcel.2010.07.012; PMID: 20705236
- Wilbanks SM, DeLuca-Flaherty C, McKay DB. Structural basis of the 70-kilodalton heat shock cognate protein ATP hydrolytic activity. I. Kinetic analyses of active site mutants. J Biol Chem 1994; 269:12893 - 8; PMID: 8175706
- Cotto J, Fox S, Morimoto R. HSF1 granules: a novel stress-induced nuclear compartment of human cells. J Cell Sci 1997; 110:2925 - 34; PMID: 9359875
- Shi Y, Mosser DD, Morimoto RI. Molecular chaperones as HSF1-specific transcriptional repressors. Genes Dev 1998; 12:654 - 66; http://dx.doi.org/10.1101/gad.12.5.654; PMID: 9499401
- Mayer C, Bierhoff H, Grummt I. The nucleolus as a stress sensor: JNK2 inactivates the transcription factor TIF-IA and down-regulates rRNA synthesis. Genes Dev 2005; 19:933 - 41; http://dx.doi.org/10.1101/gad.333205; PMID: 15805466
- Boulon S, Westman BJ, Hutten S, Boisvert FM, Lamond AI. The nucleolus under stress. Mol Cell 2010; 40:216 - 27; http://dx.doi.org/10.1016/j.molcel.2010.09.024; PMID: 20965417
- Daniely Y, Borowiec JA. Formation of a complex between nucleolin and replication protein A after cell stress prevents initiation of DNA replication. J Cell Biol 2000; 149:799 - 810; http://dx.doi.org/10.1083/jcb.149.4.799; PMID: 10811822
- Emmott E, Hiscox JA. Nucleolar targeting: the hub of the matter. EMBO Rep 2009; 10:231 - 8; http://dx.doi.org/10.1038/embor.2009.14; PMID: 19229283
- Nalabothula N, Indig FE, Carrier F. The nucleolus takes control of protein trafficking under stress. Mol Cell Pharmacol 2010; 2:203 - 12; PMID: 21499571