Abstract
We recently identified a set of chromosome domains that are early replicating uniquely in pluripotent cells. Their switch from early to late replication occurs just prior to germ layer commitment, associated with a stable form of gene silencing that is difficult to reverse. Here, we discuss results demonstrating that these domains are among the least sensitive regions in the genome to global digestion by either MNase or restriction enzymes. This inaccessible chromatin state persists whether these regions are in their physically distended early replicating or compact late replicating configuration, despite dramatic changes in 3D chromatin folding and long-range chromatin interactions, and despite large changes in transcriptional activity. This contrasts with the strong correlation between early replication, accessibility, transcriptional activity and open chromatin configuration that is observed genome-wide. We put these results in context with findings from other studies indicating that many structural (DNA sequence) and functional (density and activity of replication origins) properties of developmentally regulated replication timing (“switching”) domains resemble properties of constitutively late replicating domains. This suggests that switching domains are a type of late replicating domain within which both replication timing and transcription are subject to unique or additional layers of control not experienced by the bulk of the genome. We predict that understanding the unusual structure of these domains will reveal a novel principle of chromosome folding.
Introduction
DNA replication in mammals is regulated in a spatio-temporal manner that suggests a relationship to higher order chromatin structure in the nucleus. During early S phase, sites of DNA replication are detected throughout the interiorly located euchromatic regions of the nucleus while regions at the nuclear periphery are mainly replicated late during S phase.Citation1 Each genomic segment is replicated at a distinct time during S phase, which can be differentially regulated in the context of development and disease.Citation2,Citation3 Evidence for megabase-sized chromosomal domains of coordinate replication timing (“replication domains”; RDs) comes initially from cytological analyses,Citation4 though it has been difficult to gain further insight due to the lack of robust means to analyze this level of chromosome structure. For instance, elucidating what defines boundaries separating spatially segregated early and late RDs is central to our understanding of nuclear structure and function, but it has been almost impossible to approach this question without knowing where RD boundaries are localized with respect to DNA sequences. However, the situation changed dramatically with the recent development of genome-wide replication timing assays, which have comprehensively mapped RDs in various cell types.Citation2,Citation5,Citation6 Our group has profiled replication timing of more than 40 cell types from mouse and human. These data sets are available through Replicationdomain.orgCitation7 and a select group are available through the UCSC ENCODE genome browser. Using this method, we and others discovered that RD structures are reorganized genome-wide during embryonic stem cell (ESC) differentiation into various cell types, with approximately 50% of the genome undergoing changes in replication timing during development.Citation2,Citation8 Cell-type specific reorganization of RDs is coordinated with transcriptional changes and is conserved between mouse and human.Citation2,Citation9
In the course of these studies, we identified a set of domains that switch from early to late replicating just prior to germ layer commitment, approximately coincident with X-inactivation, and are late replicating in all other analyzed cell types.Citation8 We showed that these domains undergo an unusually stable form of gene silencing, in that both late replication and expression of genes within these domains is particularly resistant to reprogramming back to the pluripotent state.Citation8 Here, we highlight a recent study of the large-scale structure of these domains that has revealed the surprising finding that these domains undergo dramatic changes in their 3D organization and subnuclear chromatin interaction compartments while remaining exceptionally inaccessible to nucleases. We discuss these findings in the context of the general properties of developmentally regulated vs. constitutively replicated domains, and the extent to which this may be a more widespread mechanism.
A Novel Principle of Chromatin Folding
Domains that replicate early uniquely in the pluripotent cell state represent approximately 6% of the genome.Citation8,Citation10 What is the biological significance of these early to late (EtoL) domains? Both reversal of directed differentiation experiments and a comparison of genome-wide replication timing between fully reprogrammed and partially reprogrammed induced pluripotent stem cells (iPSCs) identified these EtoL switching domains as regions that resist replication-timing as well as transcriptional reprogramming, coincident with their switch to late replication.Citation8 Since these EtoL domains contain pluripotency associated genes such as Rex1 and Dppa2/4 that occlude the binding of pluripotent cell transcription factors during the early stages of the reprogramming process,Citation11 late replication may be linked to the stable silencing of these pluripotency-associated genes. What mechanism could constitute this link? One possibility is that EtoL switching domains form a specific chromatin structure that is particularly difficult to reprogram. To test this hypothesis, we examined chromatin accessibility in replication timing switching and non-switching domains genome-wide during differentiation of ESCs to neural precursor cells (NPCs) and found that EtoL switching domains do have unusual chromatin accessibility pattern.Citation12 First, MNase was used to digest chromatin from fixed nuclei in order to make direct comparisons to the structure of these domains determined by fluorescence in situ hybridization (FISH). We confirmed a strong genome-wide correlation of general accessibility to early replication previously shown by others,Citation13,Citation14 but found that EtoL switching domains, including Dppa2/4 and Rex1 domains, formed inaccessible chromatin before and after the replication-timing switch. In fact, we could detect almost no changes in accessibility despite large swings in replication timing. The same result was obtained when unfixed chromatin was used for MNase digestion. To confirm these results with higher molecular weight nucleases, we also performed digestions using a combination of restriction enzymes (NlaIII and MspI). To our knowledge, this is the first time restriction enzymes have been employed to probe global chromatin accessibility, but we obtained identical results to MNase digestion.
The fact that early replicating Dppa2/4 and Rex1 domains in ESCs form inaccessible chromatin but harbor transcriptionally active genes within them raises the possibility that another layer of chromatin structural change is occurring to form the transcriptionally permissive chromatin domain. Indeed, when we examined the distance between pairs of FISH probes spanning across these domains in the nucleus, we found an ESC-specific dramatic chromatin unfolding restricted to the region of replication timing switch, which returned to the normal degree of chromatin condensation after differentiation (). Observed chromatin reorganization at the cytological level suggests a spatial eviction of early Dppa2/4 and Rex1 domains from neighboring late replicating domains in ESCs and led us to examine intranuclear chromatin interaction patterns of these domains before and after cell differentiation. We previously demonstrated that replication timing strongly correlates with alternate compartments of chromatin interactions defined by Hi-C (high resolution chromatin conformation capture).Citation9,Citation15 Hence, we performed 4C (chromosome conformation capture on chip) using sites centrally located within the Dppa2/4 and Rex1 domains as baits. We found that, when early replicating, these domains interact with early replicating chromatin, while after switching to late replication, they interact with late replicating chromatin, providing the first demonstration of a dynamic switch in interaction compartments induced by differentiation. These findings were strengthened by a recent Hi-C study showing spatial partitioning of Dppa2/4 and Rex1 domains from neighboring chromosomal domains in mouse ESCs but not in neural cortex.Citation16 Taken together, the data show that a chromatin interaction compartment switch—without a change in accessibility—distinguishes these chromatin domains before and after differentiation, unveiling a novel principle of chromatin folding at developmentally regulated RDs.
Figure 1. A model for chromatin reorganization associated with a subnuclear compartment switch.Citation12 In pluripotent stem cells, domains subjected to EtoL regulation partially unfold and move into the early-replicating subnuclear chromatin interaction compartment, where they are suppressed from interacting with late-replicating chromatin, even neighboring late-replicating domains. During differentiation, these domains retract and acquire a similar spatial compaction to surrounding domains. In this configuration, they interact more frequently with late-replicating chromatin including neighboring domains, while, at the same time, retaining the identity of their self-interacting unit boundaries. FISH images show chromatin organization of the Dppa2/4 domain in ESCs and NPCs. Several FISH probes (red for the neighboring late domains and green for the entire early domain) were hybridized and detected simultaneously in ESCs and NPCs nuclei to visualize chromatin organization of the Dppa2/4 domain.
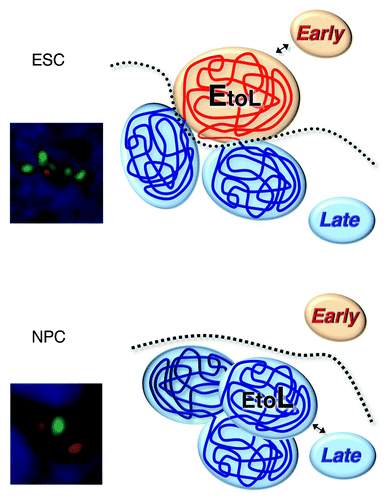
Unfolded Inaccessible Chromatin: No Longer an Oxymoron
Unfolding chromatin and moving it to a different sub-nuclear chromatin interaction compartment may also explain how genes in closed chromatin domains can be transcribed.Citation13 But why do two antagonizing mechanisms for chromatin regulation (unfolded yet inaccessible chromatin) co-exist in chromosomal domains containing pluripotency-associated genes? Given that these genes are expressed almost exclusively in pluripotent stem cells but remain silenced throughout the rest of development, it is possible that genes in closed chromatin are readily silenced in response to differentiation signals and are prevented from aberrant activation. Previous studies have revealed similar large-scale chromatin unfolding events, but some of these are closely linked to transcription,Citation17-Citation19 while others appear to be independent of transcription.Citation20,Citation21 This suggests that there are multiple mechanisms leading to large scale unfolding, only some of which require active transcription. Our RNA-DNA FISH and transcription inhibition experiments demonstrated that active transcription is not necessary to maintain the ESC-specific chromatin configuration at Dppa2/4 or Rex1 domains,Citation12 suggesting that the distended chromatin state described here reflects transcriptional competence of these domains rather than transcription per se.
Chromatin Interaction Compartments and Transcriptional Competence
The nucleus is highly compartmentalized into sub-nuclear domains in which specific sets of proteins are concentrated for various nuclear processes. It is increasingly recognized that interaction between these nuclear domains and chromatin, at least in part, contributes to establish and maintain cell type specific nuclear architecture and function.Citation22,Citation23 We observed a chromatin interaction compartment switch accompanied by a replication timing switch at developmentally regulated RDs. We still have no clear answer for what this compartment switch means, but several lines of evidence suggest a link to transcriptional competence. It has been shown that actively transcribed genes preferentially colocalize at “transcription factories” in which RNA polymerases are highly concentrated.Citation24 Transcription factories are found in the interior of the nucleus and close proximity of these factories to early replicating chromatin suggests a functional coupling of these two events in the nucleus.Citation25 This close relationship is supported by genome-wide studies showing that early replication is strongly correlated with transcriptional activity.Citation2,Citation26-Citation28 On the other hand, a lot of late replicating chromatin is localized near the nuclear periphery where transcriptional activity is generally low.Citation29,Citation30 In some gene loci, localization to the nuclear periphery is associated with transcriptional repression.Citation30-Citation34 Tethering of endogenous gene loci to the nuclear periphery can induce downregulation of some active genes.Citation30,Citation35,Citation36 Given the close relationship between late replication and silent nuclear domains, preferential interaction with neighboring late replicating chromatin compartment observed at Dppa2/4 and Rex1 domains may increase interaction of these chromosomal domains with transcriptionally repressive nuclear compartments. Indeed, Dppa2/4 and Rex1 form lamina-associated domains (LADs), transcriptionally repressive perinuclear domains, upon ESC differentiation to NPCs.Citation37 Interestingly, although LADs are smaller and do not change as much during differentiation as replication timing, LAD and RD boundaries align very closely at the Dppa2/4 and Rex1 domains(). What mechanism could link sub-nuclear compartments, replication timing and transcriptional competence? It was reported that extra-chromosomally replicating viral DNA can switch from acetylated to de-acetylated chromatin when stochastically replicating either early or late during consecutive S phases.Citation38 Hence one attractive hypothesis is that early and late replicating compartments are enriched with different sets of chromatin modifiers and therefore facilitate the formation of transcriptionally permissive and repressive chromatin structure, respectively. To date, there is little evidence for S-phase specific subnuclear compartmentalization of chromatin modifiers,Citation1 although that HDAC3 has shown to interact with Lap2b and localize specifically to nuclear periphery.Citation30,Citation39
Figure 2. Replication timing (A) and Lamin B1 binding (B) of the Dppa2/4 domain. The replication timing-switching domain is indicated in the red box. Figure was assembled using the ReplicationDomain.org database.Citation7
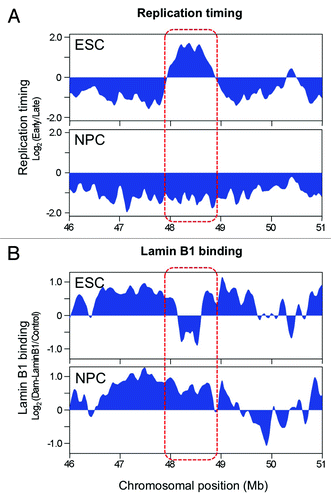
New Dimensions to DNA Replication Research
What is regulating RD structure? The conservation of replication timing and RD structures between mouse and human.Citation9,Citation40 and the consequences of chromosome rearrangements within RDs,Citation3,Citation41 suggest the existence of cis-regulatory elements that define these structural properties. On the other hand, dynamic reorganization of RD structure during cell differentiation suggests that epigenetic mechanisms also take part in the formation of RD structure. We previously showed that H3K9me2 is highly enriched at late replicating peripheral chromatin.Citation42 Surprisingly, RD structures are not affected in cells lacking G9a, the histone H3K9 dimethylase, despite the fact that mutant cells display loss of H3K9me2 mark and aberrant expression of genes within late replicating peripheral chromatin.Citation43 It has also been reported that mutations in other chromatin and DNA modifiers can show profound effects on gene expression patterns genome-wide but have little or no effect on replication timing.Citation44 By contrast, the only gene disruption shown to affect the global replication-timing program is an enigmatic nuclear matrix protein called Rif1, disruption of which has subtle effects on transcription.Citation45,Citation46 These results suggest that neither the structural changes in chromatin caused by these mutations nor the subsequent changes in transcriptional activity are sufficient to drive this level of chromosome reorganization.
RD boundaries closely align to topological chromosomal domain boundaries,Citation9,Citation12,Citation16 implying that the boundaries between units of chromatin that can self-interact also form the boundaries of units of replication regulation. Considering that chromatin looping by long-range interaction is a major mechanism behind topological domain formation, it is possible that factors mediating chromatin loop formation may play some roles in the regulation of RD structure. CTCF is one of the well-known proteins mediating chromatin loop formation. It has been shown that CTCF is enriched in topological domain boundaries revealed by Hi-C.Citation16 The observation that only 15% among all CTCF binding sites are associated with these boundaries suggests either that CTCF may require additional factors to confer its specific action at boundaries or that CTCF binding may reflect occupancy of some other regulatory factors at boundaries. Currently, little is known about roles of CTCF and its interacting proteins in RD formation. Interestingly, knockdown of Cohesin induces inefficient origin activation accompanied by increased chromatin loop size.Citation47 Although Cohesin and CTCF are known to cooperatively maintain chromatin loops, observed effects of Cohesin deficiency are independent of CTCF. Recently, it was reported that replication timing is affected by the binding of special AT-rich sequence binding protein 1 (SATB1) which is know to mediate chromatin loop formation.Citation48 Importantly however, while many proteins have shown to be involved in chromatin loop formation in mammalian cells, their roles in RD formation have not been examined and many loops that are formed and broken during transcriptional regulation have no effect on chromatin interaction compartments.Citation49
A study in yeast provides a detailed insight into the link between RD organization and long-range chromatin interaction. Yeast transcription factors Fkh1 and Fkh2 have been shown to regulate global replication timing potentially by bringing origins in close proximity in the nucleus.Citation50 These proteins bind near origins and also interact with origin recognition complex (ORC), which possibly allows them to bridge between origins to form a cluster. Replication timing is determined by the association of origins with limiting amounts of replication initiation factors such as Cdc45 during G1 phase.Citation51-Citation53 As loss of Fkh1 and Fkh2 results in either advanced or delayed origin firing, it is possible that origin clustering could affect spatial proximity of origins to replication initiation factors-enriched nuclear domains, thereby regulating their association during G1 phase. Recently Rif1 has been shown to be required for the global replication-timing program both in yeast and mammals.Citation45,Citation46,Citation54 In Rif1-deficient cells, some regions undergo EtoL replication timing switching, while others undergo LtoE switching. Perturbed spatial distribution of replication foci patterns and increased chromatin loop size are observed in Rif1-deficient mammalian cells. It will be interesting to examine effect of Rif1 mutation on chromatin interaction landscape in the nucleus, which may provide additional insight into the link between RD organization and long-range chromatin interaction. Thus, a topological view of DNA replication control opens new avenues for DNA replication research.
Switching Domains are a Type of Late Replicating Domain
Our finding that developmentally regulated RDs (switching domains) remain inaccessible even when early replicating supports our earlier contention that switching domains share properties of late replicating domains, as if late replication is the default replication timing for switching domains.Citation55 Indeed, many observations now suggest that there are general rules to set the default replication program, but that some additional property must be imposed upon switching domains for them to replicate early. First, the DNA sequence composition of switching regions, including GC content and the density of LINE elements, are similar between late and switching domains; for example, more than 6% of the sequence composition of both late and switching regions consist of LINE elements ().Citation55 Functional properties other than chromatin accessibility are also shared between late and switching regions. A recent genome-wide origin mapping study has shown that, as expected, early replication is positively correlated with the density and the activity (frequency of usage) of replication origins but, unexpectedly, switching regions have low origin density and activity even when they are early replicating.Citation56,Citation57 This observation is consistent with a model in which general regulation of replication timing within switching domains renders them late replicating, while early replication is mediated by some mechanism that can activate the relatively low number of potential origins within these domains earlier during S phase, without increasing the efficiency of their usage. Alternatively, a generalized increase in origin usage could remain undetected by the methods used in this report if there was an increase in low efficiency origin activity dispersed throughout a much larger portion of the domains. Regardless of the mechanism, these observations together suggest that developmentally regulated RDs are an independent breed of chromosomal domain with unusual regulatory properties. All the while, there are some properties of chromatin that do globally correlate with replication timing in the switching domains to an equal extent as the bulk of the genome, such as correlations to transcription and chromatin post-translational modifications associated with transcriptional activity.Citation9 Understanding what separates these properties that distinguish switching domains from constitutively late replicating domains should provide clues to the nature of this breed of chromosomal domain.
Figure 3. Replication timing changes predominantly in LINE-rich regions. Replication timing values (log2 early/late-S enrichment) are plotted for RefSeq genes in ESCs and NPCs, with values in the lower right and upper left representing LtoE and EtoL timing changes, respectively. Points are colored by the percentage of LINE-1 sequence assigned to each gene from the nearest 100 kb window, from blue (≥ 6% LINE) to yellow (< 6%LINE).
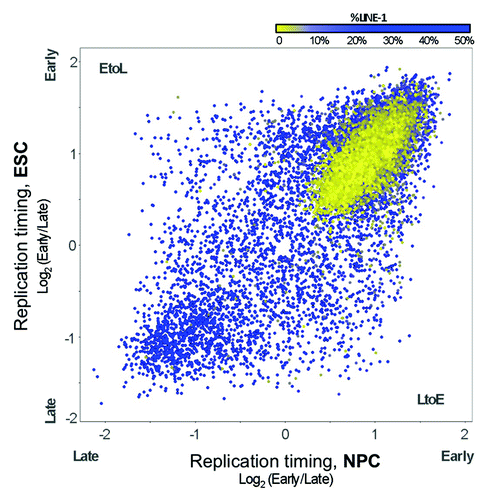
How Widespread Is This Novel Principle of Chromatin Folding?
The fact that 50% of the genome switches replication timing at some stage of development (possibly more will be revealed when other tissues, including extra-embryonic tissues, are explored) implies that if this novel mechanism applies to all switching regions, it is not unique but quite widespread. At present, the answer to this question is not clear. Based on replication timing profiles of 9 mouse cell types (), it is clear that accessibility and replication timing become progressively uncoupled the more dynamic the replication time of a domain is during development. However, FISH studies of 3D folding are laborious and single-locus based and it is not yet clear whether either Hi-C or replication timing is a reliable proxy for the scale of 3D folding that we have reported. Moreover, Hi-C maps are still very expensive to generate. While maps of DNaseI hypersensitive sites are now available for many mouse and human cell types,Citation58,Citation59 maps of global chromatin accessibility are limited. Also, our study indicated that while switching domains were generally less accessible than the bulk of the genome, regions switching LtoE during ESC differentiation were considerably more accessible than those switching EtoL (). Since accessibility data were only collected during one single cell fate change, it is difficult to predict how RDs switching “EtoL” or “LtoE” behave during other cell fate transitions. Moreover, not all replication timing switch domains resist reprogramming when differentiation is reversed.Citation8 Nonetheless, regions with large or frequent timing changes during mouse development predict low accessibility in ESCs and NPCs, despite these changes arising in different lineages (). Hence, while we certainly expect many more domains in the genome to share these properties that we have discovered through our studies of pluripotency replication fingerprints, it remains to be seen whether this novel mechanism applies to a small minority vs. a majority of the genome.
Figure 4. Timing and accessibility are uncoupled in replication timing switching regions. Correlation between replication timing values and accessibility levels (log2 nuclease accessible/inaccessible fractions) in ESCs and NPCs, ranked by the level of developmental timing changes across 9 mouse cell types. Correlations were measured in an overlapping moving window of 400/10,566 200 kb regions ranked by most static to most dynamic replication timing (smallest to largest standard deviation in timing values) during development.
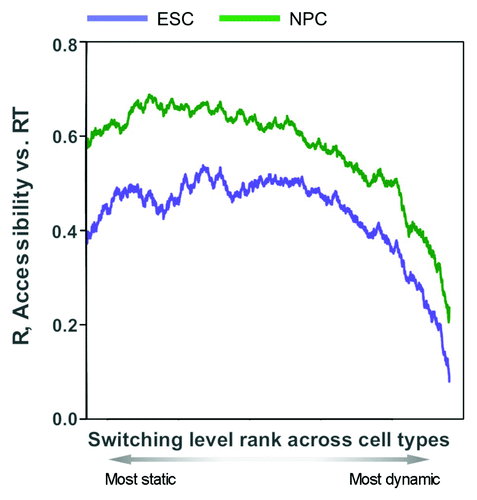
Figure 5. Low accessibility is a shared property of replication timing switching genes. (A) Accessibility level (log2 nuclease accessible/inaccessible fractions) as a function of developmental replication timing changes between ESCs and NPCs. In contrast to the general relationship between accessibility and early replication, timing switching regions in both directions (EtoL or LtoE) are relatively inaccessible even in ESCs (particularly EtoL), prior to observed changes in subnuclear organization and chromosomal contacts at EtoL loci. (B) Accessibility vs. median absolute developmental timing changes across cell types. Regions with large or frequent timing changes in mouse development predict low accessibility in ESCs and NPCs, despite these changes arising in different lineages. For both graphs, accessibility and timing values are measured at RefSeq gene promoters, with a shaded 95% confidence interval about the mean.
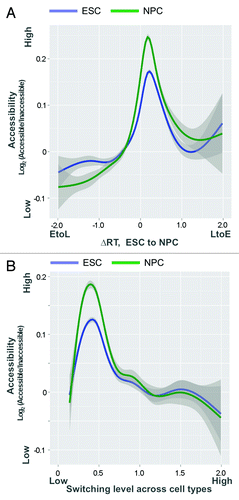
Conclusion
In summary, our results suggest that chromatin interaction compartment switch may be a general property of replication timing switching domains that form intrinsically inaccessible chromatin, and also suggest that this novel type of chromatin folding may serve as an epigenetic barrier for stable gene silencing. While there is a strong correlation between accessibility and early replication genome-wide, there is clearly a set of domains for which accessibility is uncoupled from replication timing, and this breed is even less accessible than the constitutive late regions of the genome, suggesting an unusually stable physical structure at this scale. Close alignment of RDs to topological domains supports the idea that RDs represent structural and functional units of mammalian chromosomes. Importantly, our results suggest that the expression of at least some genes within such regions may require a switch to an active early replicating sub-nuclear compartment. For genes within these regions, late and inaccessible is the default state, and some mechanism that transcends the normal early/late replication control must be invoked to move these regions into the early replicating compartment. For these reasons, we believe our results will lead to the elucidation of a novel and previously unanticipated mechanism of chromatin folding.
Abbreviations: | ||
RD | = | replication domain |
ESC | = | embryonic stem cell |
NPC | = | neural precursor cell |
iPSC | = | induced pluripotent stem cell |
FISH | = | fluorescence in situ hybridization |
Hi-C | = | high resolution chromatin conformation capture |
4C | = | chromosome conformation capture on chip |
LAD | = | lamina-associated domain |
Acknowledgments
We thank Ben Pope for discussion. Research in the Gilbert lab is supported by NIH grants GM083337 and GM085354 to D.M.G. S.T. is supported by a postodoctoral fellowship from The Uehara Memorial Foundation.
References
- Hiratani I, Takebayashi S, Lu J, Gilbert DM. Replication timing and transcriptional control: beyond cause and effect--part II. Curr Opin Genet Dev 2009; 19:142 - 9; http://dx.doi.org/10.1016/j.gde.2009.02.002; PMID: 19345088
- Hiratani I, Ryba T, Itoh M, Yokochi T, Schwaiger M, Chang CW, et al. Global reorganization of replication domains during embryonic stem cell differentiation. PLoS Biol 2008; 6:e245; http://dx.doi.org/10.1371/journal.pbio.0060245; PMID: 18842067
- Ryba T, Battaglia D, Chang BH, Shirley JW, Buckley Q, Pope BD, et al. Abnormal developmental control of replication-timing domains in pediatric acute lymphoblastic leukemia. Genome Res 2012; http://dx.doi.org/10.1101/gr.138511.112; PMID: 22628462
- Berezney R, Dubey DD, Huberman JA. Heterogeneity of eukaryotic replicons, replicon clusters, and replication foci. Chromosoma 2000; 108:471 - 84; http://dx.doi.org/10.1007/s004120050399; PMID: 10794569
- Hansen RS, Thomas S, Sandstrom R, Canfield TK, Thurman RE, Weaver M, et al. Sequencing newly replicated DNA reveals widespread plasticity in human replication timing. Proc Natl Acad Sci U S A 2010; 107:139 - 44; http://dx.doi.org/10.1073/pnas.0912402107; PMID: 19966280
- Ryba T, Battaglia D, Pope BD, Hiratani I, Gilbert DM. Genome-scale analysis of replication timing: from bench to bioinformatics. Nat Protoc 2011; 6:870 - 95; http://dx.doi.org/10.1038/nprot.2011.328; PMID: 21637205
- Weddington N, Stuy A, Hiratani I, Ryba T, Yokochi T, Gilbert DM. ReplicationDomain: a visualization tool and comparative database for genome-wide replication timing data. BMC Bioinformatics 2008; 9:530; http://dx.doi.org/10.1186/1471-2105-9-530; PMID: 19077204
- Hiratani I, Ryba T, Itoh M, Rathjen J, Kulik M, Papp B, et al. Genome-wide dynamics of replication timing revealed by in vitro models of mouse embryogenesis. Genome Res 2010; 20:155 - 69; http://dx.doi.org/10.1101/gr.099796.109; PMID: 19952138
- Ryba T, Hiratani I, Lu J, Itoh M, Kulik M, Zhang J, et al. Evolutionarily conserved replication timing profiles predict long-range chromatin interactions and distinguish closely related cell types. Genome Res 2010; 20:761 - 70; http://dx.doi.org/10.1101/gr.099655.109; PMID: 20430782
- Ryba T, Hiratani I, Sasaki T, Battaglia D, Kulik M, Zhang J, et al. Replication timing: a fingerprint for cell identity and pluripotency. PLoS Comput Biol 2011; 7:e1002225; http://dx.doi.org/10.1371/journal.pcbi.1002225; PMID: 22028635
- Sridharan R, Tchieu J, Mason MJ, Yachechko R, Kuoy E, Horvath S, et al. Role of the murine reprogramming factors in the induction of pluripotency. Cell 2009; 136:364 - 77; http://dx.doi.org/10.1016/j.cell.2009.01.001; PMID: 19167336
- Takebayashi S, Dileep V, Ryba T, Dennis JH, Gilbert DM. Chromatin-interaction compartment switch at developmentally regulated chromosomal domains reveals an unusual principle of chromatin folding. Proc Natl Acad Sci U S A 2012; 109:12574 - 9; http://dx.doi.org/10.1073/pnas.1207185109; PMID: 22807480
- Gilbert N, Boyle S, Fiegler H, Woodfine K, Carter NP, Bickmore WA. Chromatin architecture of the human genome: gene-rich domains are enriched in open chromatin fibers. Cell 2004; 118:555 - 66; http://dx.doi.org/10.1016/j.cell.2004.08.011; PMID: 15339661
- Bell O, Schwaiger M, Oakeley EJ, Lienert F, Beisel C, Stadler MB, et al. Accessibility of the Drosophila genome discriminates PcG repression, H4K16 acetylation and replication timing. Nat Struct Mol Biol 2010; 17:894 - 900; http://dx.doi.org/10.1038/nsmb.1825; PMID: 20562853
- Lieberman-Aiden E, van Berkum NL, Williams L, Imakaev M, Ragoczy T, Telling A, et al. Comprehensive mapping of long-range interactions reveals folding principles of the human genome. Science 2009; 326:289 - 93; http://dx.doi.org/10.1126/science.1181369; PMID: 19815776
- Dixon JR, Selvaraj S, Yue F, Kim A, Li Y, Shen Y, et al. Topological domains in mammalian genomes identified by analysis of chromatin interactions. Nature 2012; 485:376 - 80; http://dx.doi.org/10.1038/nature11082; PMID: 22495300
- Volpi EV, Chevret E, Jones T, Vatcheva R, Williamson J, Beck S, et al. Large-scale chromatin organization of the major histocompatibility complex and other regions of human chromosome 6 and its response to interferon in interphase nuclei. J Cell Sci 2000; 113:1565 - 76; PMID: 10751148
- Mahy NL, Perry PE, Bickmore WA. Gene density and transcription influence the localization of chromatin outside of chromosome territories detectable by FISH. J Cell Biol 2002; 159:753 - 63; http://dx.doi.org/10.1083/jcb.200207115; PMID: 12473685
- Naughton C, Sproul D, Hamilton C, Gilbert N. Analysis of active and inactive X chromosome architecture reveals the independent organization of 30 nm and large-scale chromatin structures. Mol Cell 2010; 40:397 - 409; http://dx.doi.org/10.1016/j.molcel.2010.10.013; PMID: 21070966
- Tumbar T, Sudlow G, Belmont AS. Large-scale chromatin unfolding and remodeling induced by VP16 acidic activation domain. J Cell Biol 1999; 145:1341 - 54; http://dx.doi.org/10.1083/jcb.145.7.1341; PMID: 10385516
- Sato N, Nakayama M, Arai K. Fluctuation of chromatin unfolding associated with variation in the level of gene expression. Genes Cells 2004; 9:619 - 30; http://dx.doi.org/10.1111/j.1356-9597.2004.00751.x; PMID: 15265005
- Francastel C, Schübeler D, Martin DI, Groudine M. Nuclear compartmentalization and gene activity. Nat Rev Mol Cell Biol 2000; 1:137 - 43; http://dx.doi.org/10.1038/35040083; PMID: 11253366
- Fraser P, Bickmore W. Nuclear organization of the genome and the potential for gene regulation. Nature 2007; 447:413 - 7; http://dx.doi.org/10.1038/nature05916; PMID: 17522674
- Schoenfelder S, Sexton T, Chakalova L, Cope NF, Horton A, Andrews S, et al. Preferential associations between co-regulated genes reveal a transcriptional interactome in erythroid cells. Nat Genet 2010; 42:53 - 61; http://dx.doi.org/10.1038/ng.496; PMID: 20010836
- Malyavantham KS, Bhattacharya S, Alonso WD, Acharya R, Berezney R. Spatio-temporal dynamics of replication and transcription sites in the mammalian cell nucleus. Chromosoma 2008; 117:553 - 67; http://dx.doi.org/10.1007/s00412-008-0172-6; PMID: 18600338
- Farkash-Amar S, Lipson D, Polten A, Goren A, Helmstetter C, Yakhini Z, et al. Global organization of replication time zones of the mouse genome. Genome Res 2008; 18:1562 - 70; http://dx.doi.org/10.1101/gr.079566.108; PMID: 18669478
- Desprat R, Thierry-Mieg D, Lailler N, Lajugie J, Schildkraut C, Thierry-Mieg J, et al. Predictable dynamic program of timing of DNA replication in human cells. Genome Res 2009; 19:2288 - 99; http://dx.doi.org/10.1101/gr.094060.109; PMID: 19767418
- Schwaiger M, Stadler MB, Bell O, Kohler H, Oakeley EJ, Schübeler D. Chromatin state marks cell-type- and gender-specific replication of the Drosophila genome. Genes Dev 2009; 23:589 - 601; http://dx.doi.org/10.1101/gad.511809; PMID: 19270159
- Deniaud E, Bickmore WA. Transcription and the nuclear periphery: edge of darkness?. Curr Opin Genet Dev 2009; 19:187 - 91; http://dx.doi.org/10.1016/j.gde.2009.01.005; PMID: 19231154
- Zullo JM, Demarco IA, Piqué-Regi R, Gaffney DJ, Epstein CB, Spooner CJ, et al. DNA sequence-dependent compartmentalization and silencing of chromatin at the nuclear lamina. Cell 2012; 149:1474 - 87; http://dx.doi.org/10.1016/j.cell.2012.04.035; PMID: 22726435
- Kosak ST, Skok JA, Medina KL, Riblet R, Le Beau MM, Fisher AG, et al. Subnuclear compartmentalization of immunoglobulin loci during lymphocyte development. Science 2002; 296:158 - 62; http://dx.doi.org/10.1126/science.1068768; PMID: 11935030
- Zink D, Amaral MD, Englmann A, Lang S, Clarke LA, Rudolph C, et al. Transcription-dependent spatial arrangements of CFTR and adjacent genes in human cell nuclei. J Cell Biol 2004; 166:815 - 25; http://dx.doi.org/10.1083/jcb.200404107; PMID: 15364959
- Ragoczy T, Bender MA, Telling A, Byron R, Groudine M. The locus control region is required for association of the murine beta-globin locus with engaged transcription factories during erythroid maturation. Genes Dev 2006; 20:1447 - 57; http://dx.doi.org/10.1101/gad.1419506; PMID: 16705039
- Williams RR, Azuara V, Perry P, Sauer S, Dvorkina M, Jørgensen H, et al. Neural induction promotes large-scale chromatin reorganisation of the Mash1 locus. J Cell Sci 2006; 119:132 - 40; http://dx.doi.org/10.1242/jcs.02727; PMID: 16371653
- Reddy KL, Zullo JM, Bertolino E, Singh H. Transcriptional repression mediated by repositioning of genes to the nuclear lamina. Nature 2008; 452:243 - 7; http://dx.doi.org/10.1038/nature06727; PMID: 18272965
- Finlan LE, Sproul D, Thomson I, Boyle S, Kerr E, Perry P, et al. Recruitment to the nuclear periphery can alter expression of genes in human cells. PLoS Genet 2008; 4:e1000039; http://dx.doi.org/10.1371/journal.pgen.1000039; PMID: 18369458
- Peric-Hupkes D, Meuleman W, Pagie L, Bruggeman SW, Solovei I, Brugman W, et al. Molecular maps of the reorganization of genome-nuclear lamina interactions during differentiation. Mol Cell 2010; 38:603 - 13; http://dx.doi.org/10.1016/j.molcel.2010.03.016; PMID: 20513434
- Lande-Diner L, Zhang J, Cedar H. Shifts in replication timing actively affect histone acetylation during nucleosome reassembly. Mol Cell 2009; 34:767 - 74; http://dx.doi.org/10.1016/j.molcel.2009.05.027; PMID: 19560427
- Somech R, Shaklai S, Geller O, Amariglio N, Simon AJ, Rechavi G, et al. The nuclear-envelope protein and transcriptional repressor LAP2beta interacts with HDAC3 at the nuclear periphery, and induces histone H4 deacetylation. J Cell Sci 2005; 118:4017 - 25; http://dx.doi.org/10.1242/jcs.02521; PMID: 16129885
- Yaffe E, Farkash-Amar S, Polten A, Yakhini Z, Tanay A, Simon I. Comparative analysis of DNA replication timing reveals conserved large-scale chromosomal architecture. PLoS Genet 2010; 6:e1001011; http://dx.doi.org/10.1371/journal.pgen.1001011; PMID: 20617169
- Pope BD, Chandra T, Buckley Q, Hoare M, Ryba T, Wiseman FK, et al. Replication-timing boundaries facilitate cell-type and species-specific regulation of a rearranged human chromosome in mouse. Hum Mol Genet 2012; 21:4162 - 70; http://dx.doi.org/10.1093/hmg/dds232; PMID: 22736031
- Wu R, Terry AV, Singh PB, Gilbert DM. Differential subnuclear localization and replication timing of histone H3 lysine 9 methylation states. Mol Biol Cell 2005; 16:2872 - 81; http://dx.doi.org/10.1091/mbc.E04-11-0997; PMID: 15788566
- Yokochi T, Poduch K, Ryba T, Lu J, Hiratani I, Tachibana M, et al. G9a selectively represses a class of late-replicating genes at the nuclear periphery. Proc Natl Acad Sci U S A 2009; 106:19363 - 8; http://dx.doi.org/10.1073/pnas.0906142106; PMID: 19889976
- Jørgensen HF, Azuara V, Amoils S, Spivakov M, Terry A, Nesterova T, et al. The impact of chromatin modifiers on the timing of locus replication in mouse embryonic stem cells. Genome Biol 2007; 8:R169; http://dx.doi.org/10.1186/gb-2007-8-8-r169; PMID: 17705870
- Cornacchia D, Dileep V, Quivy JP, Foti R, Tili F, Santarella-Mellwig R, et al. Mouse Rif1 is a key regulator of the replication-timing programme in mammalian cells. EMBO J 2012; 31:3678 - 90; http://dx.doi.org/10.1038/emboj.2012.214; PMID: 22850673
- Yamazaki S, Ishii A, Kanoh Y, Oda M, Nishito Y, Masai H. Rif1 regulates the replication timing domains on the human genome. EMBO J 2012; 31:3667 - 77; http://dx.doi.org/10.1038/emboj.2012.180; PMID: 22850674
- Guillou E, Ibarra A, Coulon V, Casado-Vela J, Rico D, Casal I, et al. Cohesin organizes chromatin loops at DNA replication factories. Genes Dev 2010; 24:2812 - 22; http://dx.doi.org/10.1101/gad.608210; PMID: 21159821
- Oda M, Kanoh Y, Watanabe Y, Masai H. Regulation of DNA Replication Timing on Human Chromosome by a Cell-Type Specific DNA Binding Protein SATB1. PLoS One 2012; 7:e42375; http://dx.doi.org/10.1371/journal.pone.0042375; PMID: 22879953
- Zhang Y, McCord RP, Ho YJ, Lajoie BR, Hildebrand DG, Simon AC, et al. Spatial organization of the mouse genome and its role in recurrent chromosomal translocations. Cell 2012; 148:908 - 21; http://dx.doi.org/10.1016/j.cell.2012.02.002; PMID: 22341456
- Knott SR, Peace JM, Ostrow AZ, Gan Y, Rex AE, Viggiani CJ, et al. Forkhead transcription factors establish origin timing and long-range clustering in S. cerevisiae. Cell 2012; 148:99 - 111; http://dx.doi.org/10.1016/j.cell.2011.12.012; PMID: 22265405
- Mantiero D, Mackenzie A, Donaldson A, Zegerman P. Limiting replication initiation factors execute the temporal programme of origin firing in budding yeast. EMBO J 2011; 30:4805 - 14; http://dx.doi.org/10.1038/emboj.2011.404; PMID: 22081107
- Tanaka S, Nakato R, Katou Y, Shirahige K, Araki H. Origin association of Sld3, Sld7, and Cdc45 proteins is a key step for determination of origin-firing timing. Curr Biol 2011; 21:2055 - 63; http://dx.doi.org/10.1016/j.cub.2011.11.038; PMID: 22169533
- Wong PG, Winter SL, Zaika E, Cao TV, Oguz U, Koomen JM, et al. Cdc45 limits replicon usage from a low density of preRCs in mammalian cells. PLoS One 2011; 6:e17533; http://dx.doi.org/10.1371/journal.pone.0017533; PMID: 21390258
- Hayano M, Kanoh Y, Matsumoto S, Renard-Guillet C, Shirahige K, Masai H. Rif1 is a global regulator of timing of replication origin firing in fission yeast. Genes Dev 2012; 26:137 - 50; http://dx.doi.org/10.1101/gad.178491.111; PMID: 22279046
- Hiratani I, Leskovar A, Gilbert DM. Differentiation-induced replication-timing changes are restricted to AT-rich/long interspersed nuclear element (LINE)-rich isochores. Proc Natl Acad Sci U S A 2004; 101:16861 - 6; http://dx.doi.org/10.1073/pnas.0406687101; PMID: 15557005
- Besnard E, Babled A, Lapasset L, Milhavet O, Parrinello H, Dantec C, et al. Unraveling cell type-specific and reprogrammable human replication origin signatures associated with G-quadruplex consensus motifs. Nat Struct Mol Biol 2012; 19:837 - 44; http://dx.doi.org/10.1038/nsmb.2339; PMID: 22751019
- Gilbert DM. Replication origins run (ultra) deep. Nat Struct Mol Biol 2012; 19:740 - 2; http://dx.doi.org/10.1038/nsmb.2352; PMID: 22864361
- Stamatoyannopoulos JA, Snyder M, Hardison R, Ren B, Gingeras T, Gilbert DM, et al, Mouse ENCODE Consortium. An encyclopedia of mouse DNA elements (Mouse ENCODE). Genome Biol 2012; 13:418; http://dx.doi.org/10.1186/gb-2012-13-8-418; PMID: 22889292
- Bernstein BE, Birney E, Dunham I, Green ED, Gunter C, Snyder M, ENCODE Project Consortium. An integrated encyclopedia of DNA elements in the human genome. Nature 2012; 489:57 - 74; http://dx.doi.org/10.1038/nature11247; PMID: 22955616