Abstract
Transcription of protein-coding genes in mammalian cells is coordinated with pre-mRNA processing as well as the assembly and nuclear export of mRNPs. Btf (BCLAF1) and TRAP150 (THRAP3) were previously reported to associate with in vitro spliced mRNPs and also as a part of the spliceosome, suggesting they are involved in pre-mRNA processing. Btf and TRAP150 are serine-arginine-rich (SR) proteins with significant sequence similarity, but the extent of their functional overlap is not yet clear. We show that both Btf and TRAP150 localize at a constitutively active β-tropomyosin (BTM) reporter minigene locus in mammalian cells. Both proteins also localize at a U2OS 2–6-3 reporter gene locus in a RNA polymerase II (RNAPII) transcription-dependent manner. While Btf and TRAP150 showed some overlap with reporter RNA and other pre-mRNA processing factors at transcription loci, they showed the most precise overlap with the exon junction complex (EJC) protein Magoh. Since EJC components have roles in nuclear export, we examined nuclear/cytoplasmic mRNA distribution after Btf or TRAP150 knockdown. Btf depletion caused an increase of β-tropomyosin minigene reporter transcripts in the cytoplasm as well as global increase of endogenous polyadenylated RNA in the cytoplasm, while TRAP150 depletion did not. We provide evidence that Btf has functions distinct from TRAP150 in regulating the subcellular distribution of mRNAs in human cells.
Introduction
In mammalian cells, transcription and processing of pre-mRNAs is coordinated to promote efficient gene expression. Many of the factors involved in the various steps of pre-mRNA processing reside in nuclear compartments called nuclear speckles.Citation1 The nuclear speckle proteome consists of at least 180 proteins that are predominantly mRNA processing factors.Citation2,Citation3 Nuclear speckles also contain many polyadenylated RNAs, including nuclear retained long noncoding RNAs such as MALAT1, that modulate splicing factor activity.Citation4 While most proteins identified by mass spectrometry analysis of purified nuclear speckles colocalize with splicing factors in nuclear speckles,Citation3 some of these factors exhibit limited nuclear speckle enrichment. Two such proteins include TRAP150 (“thyroid hormone associated protein of 150 kDa,” or THRAP3) and Btf (Bcl2-associated transcription factor, or BCLAF1). Interestingly, these two proteins share 39% identity and 66% similarity in their primary amino acid sequence, and they both localize predominantly in nucleoplasmic foci rather than in nuclear speckles. Btf and TRAP150 have an N-terminal RS domain, but they lack RNA recognition motifs that are typically found in splicing factors. Since our results show that Btf and TRAP150 localize in nucleoplasmic foci rather than in nuclear speckles where RS proteins typically reside, we hypothesized that Btf and TRAP150 may have primary functions in mRNA processing steps other than pre-mRNA splicing.
Btf and TRAP150 have previously been implicated at multiple steps of mRNA processing. Btf and TRAP150 were among the many factors found in synthetic affinity-purified in vitro spliced mRNPs, as well as spliceosome complexes.Citation5-Citation7 Btf co-immunoprecipitated with 9G8, hnRNP A1 and export factor TAP, while TRAP150 co-immunoprecipitated with splicing factors and EJC proteins and was demonstrated to play a role in nuclear mRNA decay.Citation8,Citation9 In addition, Btf and TRAP150 were identified in a protein complex with SNIP1, SkIP and Pinin that was important in the regulation of cyclin D1 mRNA stability.Citation10 In another study, TRAP150 interaction with phosphorylated polypyrimidine tract-binding protein (PSF) was demonstrated to block PSF binding to CD45 pre-mRNA, thereby regulating CD45 alternative splicing. This function for TRAP150 was not shared by Btf, despite being identified in the same complex with PSF.Citation11 All of these studies indicate roles for Btf and TRAP150 in pre-mRNA processing. However, there is still little known about what steps of mRNA synthesis and processing depend on Btf and TRAP150, or where within mammalian nuclei TRAP150 and Btf execute their functions. Btf and TRAP150 functions have often been reported independently of each another, or indirectly through the purification and analysis of large multimolecular complexes. Analysis of Btf and TRAP150 functions was the primary focus of our study, and we tested the hypothesis that individual depletion of Btf or TRAP150 affects pre-mRNA synthesis, splicing or some other aspect of mRNA expression. We directly compared Btf and TRAP150 by using in situ approaches to determine whether they have similar and/or different mRNA processing functions.
Here we show accumulation of both Btf and TRAP150 at a constitutively active β-tropomyosin (BTM) reporter locus as well as at an activated U2OS 2–6-3 gene locus. At transcription sites, both Btf and TRAP150 showed the most precise overlay with each other and with the EJC protein Magoh, than with other gene regulators. While we also observed significant overlap with reporter RNA and splicing factors, there was no overlap between TRAP150 or Btf and transcription activator. Finally, we used an RNAi approach to determine if independently silencing the expression of TRAP150 or Btf altered abundance or subcellular localization of mRNA. Btf depleted cells showed increased abundance of endogenous polyadenylated RNA and reporter mRNA in the cytoplasm that did not occur following TRAP150 depletion. Since immunoblots revealed that Btf depletion resulted in increased expression of TRAP150, we prevented this apparent compensatory TRAP150 expression upon Btf depletion by adding limiting amounts of TRAP150 siRNA duplex to the siRNA mixture. Our experiments demonstrate that it is Btf depletion, but not TRAP150 upregulation, that leads to a global change in subcellular distribution of mRNA. In summary, our data show that Btf has functions distinct from TRAP150 in mRNA metabolism. We reveal that modulating the expression of a single protein, Btf, has global impact upon the nuclear/cytoplasmic distribution of mRNAs in human cells.
Results
TRAP150 and Btf were identified in purified nuclear speckles,Citation2,Citation3 and YFP-tagged Btf showed overlap with splicing factors near the periphery of nuclear speckles.Citation3 We used our newly developed anti-Btf antibody (Fig. S1) and a commercially available anti-TRAP150 antibody to examine the distribution of endogenous Btf and TRAP150 in HeLa cells. Endogenous Btf and TRAP150 showed a predominantly nuclear distribution in small foci throughout the nucleoplasm, that were excluded from nucleoli () and did not overlap at nuclear speckles with the snRNP protein U1–70K () or with other splicing factors such as SRSF1 (Fig. S2A) that was similar in all cell lines examined. Likewise, CFP-Btf and CFP-TRAP150 also localized to nucleoplasmic foci, only some of which overlapped with SRSF1 in U2OS cells (Fig. S2B). If the punctate nuclear localization pattern of Btf and TRAP150 is transcription dependent, then the localization of these proteins should change significantly after RNAPII inhibition. Both TRAP150 and Btf localized at nuclear speckles upon inhibition of RNAPII with α-amanitin (). The fact that both of these proteins have an arginine-serine-rich (RS) domain and that they were both isolated in purified nuclear speckle fractions,Citation2,Citation3 is consistent with these observations.
Figure 1. Localization of TRAP150 and Btf is dependent on RNA Polymerase II activity. (A) In the absence of α-amanitin, TRAP150 (e, arrow) localizes in nucleoplasmic foci, shows some overlap with splicing factor U1–70K (f) in nuclear speckles and localizes to enlarged rounded speckles (a, arrow) after α-amanitin treatment similar to U1–70K (b, arrow). (B) Btf (m, arrow) localizes in nucleoplasmic foci, shows some overlap with splicing factor U1–70K (n) and localizes to enlarged rounded nuclear speckles (i, arrow) after α-amanitin treatment similar to U1–70K (j, arrow). DNA was stained with DAPI. Boxed regions in a, e, i and m are enlarged in insets. Scale bar, 5 µm.
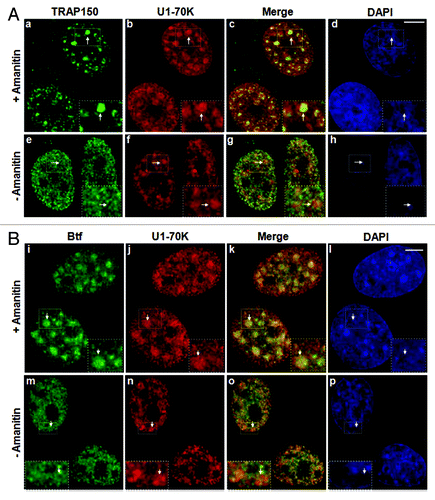
Two different cell lines with integrated reporter gene arrays were used to test whether Btf and TRAP150 accumulated at transcription sites along with other pre-mRNA synthesis and processing factors.Citation12,Citation14 The first reporter was a HeLa cell line with a stably integrated rat BTM reporter minigene (BTM HeLa, ) that is constitutively active and can be visualized by RNA fluorescence in situ hybridization (FISH) as a single transcription locus in each cell nucleus.Citation15,Citation16 BTM HeLa cells were processed for localization of BTM transcripts by RNA-FISH, followed by immunolabeling of splicing factor SRSF1 and either Btf or TRAP150. In contrast to the limited colocalization observed between Btf or TRAP150 with SRSF1 at nuclear speckles, both Btf and TRAP150 colocalized with SRSF1 at the BTM transcription site (). The rRNA processing factor fibrillarin did not overlap with the BTM transcription site (Fig. S3).
Figure 2. Btf and TRAP150 localize at transcription sites in situ. (A) Schematic representation of the rat β-tropomyosin (BTM) minigene that includes genomic sequence from exon 5 through exon 8. Introns are represented as thin lines connecting the exons. (B) HeLa cells stably expressing the rat BTM minigene were processed for RNA-FISH localization of BTM reporter RNA (b, g) and immunofluorescence localization of SRSF1 (c, h) and either TRAP150 (a) or Btf (f). (C) Schematic representation of U2OS 2–6-3 inducible reporter array.Citation17(D) U2OS 2–6-3 cells transfected with YFP-LacR and pTetON were left uninduced (o-r, w-z) or induced to activate the reporter (+Dox; k-n, s-v). At 2.5 h after dox addition, the cells were processed for immunofluorescence localization of TRAP150 (k-r) or Btf (s-z). DNA was stained with DAPI. Boxed regions in k and o are enlarged in insets. Scale bar, 5 µm.
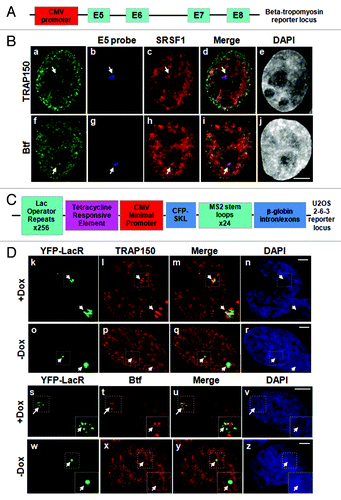
To examine if accumulation at gene loci is RNAPII transcription-dependent, we used U2OS 2–6-3 cells with an inducible reporter gene array ().Citation17 Transcription of this reporter array is activated by the transcription factor rtTA (encoded by pTetON) in the presence of doxycycline. In this array, the locus chromatin is visualized by expressing fluorescent-protein-tagged Lac repressor (LacR) to label operator sequences that lie upstream of the CMV minimal promoter. Reporter transcripts are visualized by expressing MS2 binding protein (MS2BP) to label MS2 stem loop structures in the transcripts.Citation17 Reporter transcripts can be detected at this locus by microscopy almost immediately after induction with doxycycline, and the locus chromatin completely decondenses within 2 h.Citation17 Endogenous TRAP150 and Btf accumulated at active loci (, k‒n and s‒v), but not at inactive loci (, o‒r and w‒z), consistent with RNAPII-dependent accumulation at these transcription sites.
We next asked if enrichment of Btf and TRAP150 at the active locus requires synthesis of nascent transcripts. Treatment of U2OS 2–6-3 cells with α-amanitin prevented transcript production in the presence of doxycycline as evidenced by absence of MS2-binding protein at the transcription site (). In amanitin-treated cells, neither TRAP150 () nor Btf () was detected at the activated transcription array (). Consistent with our data from HeLa cells (), Btf and TRAP150 accumulated in speckles following amanitin treatment, and in some cases, speckles were in close proximity with the locus. Control cells induced in parallel without amanitin showed TRAP150 and Btf at the active transcription site (). Taken together, the data in – show that Btf and TRAP150 are not likely to be part of an activator complex upstream of RNA polymerase II and demonstrate that nascent transcripts are needed for their recruitment to transcription sites. However, since Btf and TRAP150 had both been previously reported to have a role in transcription,Citation18-Citation20 we looked for the extent of their colocalization with transcriptional activator rtTA (). Endogenous Btf () and TRAP150 () localized at regions of the locus that were clearly distinct from rtTA, as well as from the lac repressor associated with the operator sequences. Average Pearson correlation coefficients (PCC) indicated minimal overlap among these regions (Fig. S4; Average PCC for 50 loci was 0.35 ± 0.01 for Btf or 0.02 ± 0.001 for TRAP150). It is possible that each of these “subcompartments” of the locus reflects a different step along the assembly line for mRNA biogenesis, and they can be distinguished here due to the robust synthesis and multicopy arrangement of this locus. Since Btf and TRAP150 were isolated in purified nuclear speckles, and well-characterized nuclear speckle proteins have demonstrated roles in pre-mRNA processing,Citation1 it is more likely that they have primary roles in pre-mRNA processing rather than transcription activation.Citation8 We therefore used the U2OS 2–6-3 cells to examine the extent of overlap between Btf or TRAP150 and well-characterized RNA processing proteins in order to determine the most likely role for TRAP150 and Btf in gene expression.
Figure 3. Localization of Btf and TRAP150 at transcription sites is dependent on RNA polymerase II activity. (A) In the absence of amanitin, TRAP150 (c) localized at the active locus. In the presence of amanitin, the locus was decondensed (e); however, MS2BP accumulation in speckles rather than at the active locus indicated that no transcripts were produced under these conditions (f) and TRAP150 (g) was absent. (B) In the absence of amanitin, Btf (j) localized at the active locus. In the presence of amanitin, the locus was decondensed (m); however, MS2BP accumulation in speckles rather than at the active locus indicated that no transcripts were produced under these conditions (n) and Btf (o) was absent. Boxed regions in a, e, i and m are enlarged in insets. (C) Btf and TRAP150 do not colocalize with transcriptional activator at the U2OS 2–6-3 reporter locus. U2OS 2–6-3 cells were transiently transfected with pTetON-YFP and CFP-LacR and seeded in the presence of doxycycline. After 2.5 h, cells were processed for immunolabeling of endogenous Btf or TRAP150. 100% of the cells counted showed that Btf (a) or TRAP150 (e) accumulate at the active locus (panel b and f) but does not colocalize with the transcriptional activator encoded by pTetON (rtTA; c and g). Boxed regions in b and f are enlarged in insets. Scale bar, 5 µm.
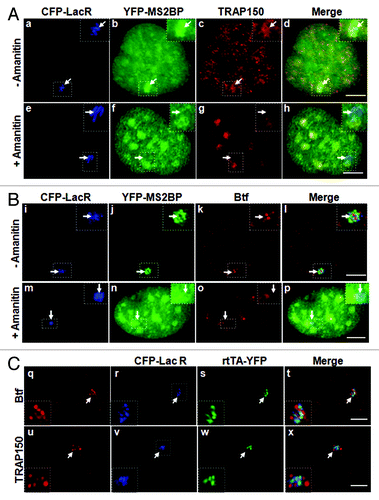
Btf and TRAP150 co-immunoprecipitated with EJC proteins,Citation9 and our WU10 anti-Btf antibody co-immunoprecipitated YFP-TRAP150 and YFP-Magoh from 293 cell extract (Fig. S5). However, it is not yet known if TRAP150 and Btf and the other proteins within these complexes all colocalize at transcription sites, or if such complexes are limited to nuclear speckles where all of these components have been identified.Citation2,Citation3 Multi-label immunofluorescence experiments were performed to examine if Btf and TRAP150 colocalize with reporter transcripts. We also examined if there is any colocalization of Btf or TRAP150 with each other, with splicing factor SRSF1 or with the EJC protein Magoh at reporter loci. As indicated for some of these multi-label experiments, we expressed CFP-tagged Btf/TRAP150 at levels that reflected localization patterns of their endogenous counterparts. Also, to label reporter transcripts at the active U2OS 2–6-3 locus, we expressed the MS2 stem loop binding protein fused with YFP (YFP-MS2BP). Expressing MS2BP is an accurate way to label active U2OS 2–6-3 reporter loci by microscopy, as the area of highest YFP-MS2BP accumulation was always in the nuclear region labeled by Lac repressor (Fig. S6A). We noted a striking overlap of Btf and TRAP150 that consistently showed an identical labeling pattern at the active locus and had a higher PCC than that observed for reporter RNA/Btf overlap or reporter RNA/TRAP150 overlap ( and Fig. S7A). SRSF1 showed significant colocalization with YFP-MS2BP-labeled reporter transcripts (Fig. S6B and C) as expected for this reporter system.Citation17 Similar to transcription factor rtTA (), SRSF1 labeling did not precisely coincide with the Lac repressor-labeled chromatin (). We observed significant overlap between SRSF1/Btf (, e‒h) or SRSF1/TRAP150 (, i‒l), although some areas of SRSF1 on the active transcription site did not have detectable overlap with Btf or TRAP150. While these results indicate that molecular complexes containing Btf and/or TRAP150 do not always completely overlap with splicing factor SRSF1, our experiments indicate an overall high coincidence of overlap (Fig. S7B and C; Average PCC for 50 loci was 0.80 ± 0.03 for both Btf and TRAP150).
Figure 4. Btf and TRAP150 colocalize with each other, with splicing factor SRSF1, and most precisely with Magoh at transcription sites in situ. (A) U2OS 2–6-3 cells expressing pTetON, YFP-MS2BP (panel a) and CFP-TRAP150 (panel b) were processed for immunolabeling of Btf (panel c). 50 out of the 50 loci scored showed that Btf and TRAP150 overlap extensively at the active locus. (B) U2OS 2–6-3 cells expressing YFP-LacR, pTetON and either CFP-Btf or CFP-TRAP150 were fixed 2.5 h after doxycycline addition and processed for immunolabeling of SRSF1 (a-l). 50 out of the 50 loci scored showed colocalization of Btf and TRAP150 with SRSF1 at the active locus. (C) U2OS 2–6-3 cells stably expressing mcherry-LacI (m, q) were transfected with YFP-Magoh (o, s) and either CFP-Btf (n) or CFP-TRAP150 (r). 50 out of the 50 loci scored showed remarkable colocalization of Btf and TRAP150 with Magoh at the active locus. Boxed regions in a, e, i, m and q are enlarged in insets. To avoid saturating image pixel intensity for CFP-Btf/CFP-TRAP150 at the region of the locus, low image exposure times were required; therefore, the nuclear CFP-Btf/CFP-TRAP150 that was also present in nucleoplasmic foci does not appear in these images. Scale bar, 5 µm.
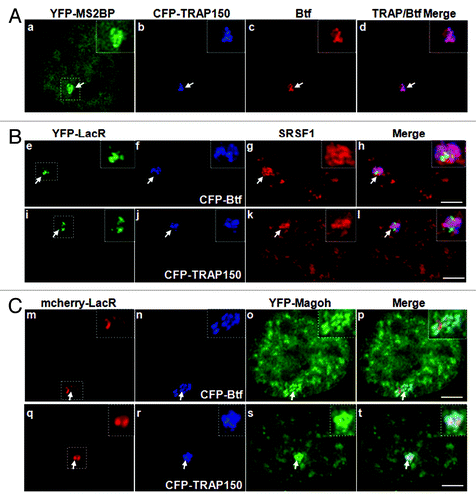
Furthermore, all of the sub regions within the activated reporter locus that were occupied by Btf or TRAP150 colocalized precisely with the EJC protein Magoh ( and Fig. S8; Average PCC for 50 loci was 0.85 ± 0.02 for Btf or 0.9 ± 0.03 for TRAP150). To determine the physiological relevance of colocalization of Btf and TRAP150 with each other, as well as with the EJC protein Magoh, we examined if TRAP150 and/or Magoh co-immunoprecipitate with Btf in extracts from 293 cells transfected with YFP-TRAP150 and YFP-Magoh. Btf complexes were immunoprecipitated using our anti-Btf antibody WU10 and separated by SDS-PAGE. YFP-TRAP150 and YFP-Magoh were present in the anti-Btf immunoprecipitates (Fig. S5). Taken together with our in situ data, the biochemical data from our lab and other labs suggests that Btf and TRAP150 associate with nascent transcripts along with other mRNA processing factors, most likely as components of the EJC.
Since EJC components have roles in nuclear export of mRNAs, we examined if silencing TRAP150 or Btf expression affects the subcellular distribution of mRNA. Toward this end, we examined specific effects on BTM transcripts, or global effects on polyadenylated RNA. We proceeded to examine if BTM reporter transcript abundance in the cytoplasm is altered following Btf depletion. HeLa cells treated with two different sets of Btf siRNAs or two different sets of TRAP150 siRNAs showed significant reduction in Btf or TRAP150, respectively, as compared with controls (). RT-PCR analysis of nuclear and cytoplasmic RNA fractions from these cells verified enrichment for specific nuclear (U2 snRNA) and cytoplasmic (S14) RNAs (). qRT-PCR using exon 5 primers targeting BTM transcripts revealed that Btf depletion caused more than 2-fold increase in cytoplasmic BTM, while there was no increase in cytoplasmic BTM in TRAP-depleted cells (). On the other hand, TRAP150-depleted samples showed modest increase of BTM transcripts in the nucleus (). Btf depletion vs. TRAP150 depletion therefore had opposite effects on subcellular mRNA distribution.
Figure 5. Btf depletion increases levels of BTM transcripts in the cytoplasm. (A) Immunoblots from cells treated in parallel confirm depletion of Btf or TRAP150. Beta-actin was used as a loading control. (B) Validation of nuclear/cytoplasmic RNA fractionation by RT-PCR using primers targeting human U2 snRNA (nuclear) and S14 (cytoplasmic) on total (lane T), nuclear (lane N) and cytoplasmic (lane C) RNA samples. (C) Representative qRT-PCR analysis shows the relative change in the BTM transcripts in nuclear vs. cytoplasmic compartments when normalized to GAPDH. Grey bars represent nuclear BTM RNA while black bars represent cytoplasmic BTM RNA. *p values ≤ 0.05; #p values ≤ 0.1.
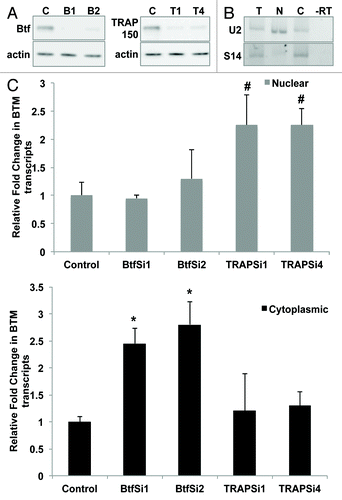
Reducing Btf levels could affect assembly or targeting of other mRNA processing factors to transcription sites, leading to altered splicing or indirect effects on mRNA distribution. In contrast to what was previously observed following depletion of other splicing factors,Citation16 we did not observe altered splicing of BTM transcripts after depletion of Btf or TRAP150 (data not shown). Since Btf depletion caused a dramatic difference in cytoplasmic levels of BTM transcripts, we looked for changes in recruitment of other mRNA processing factors to the BTM locus following Btf depletion. Btf-depleted cells showed recruitment of YFP-TRAP150 (Fig. 6Ah), SRSF1 (Fig. 6Ar) and YFP-Magoh (Fig. 6Abb) to the BTM transcription site at levels comparable to what was observed in cells treated with control siRNA duplexes (Fig. 6Ac, m and w). Similar results were observed using two different siRNA duplexes targeting Btf. Therefore, it is more likely that the changes in cellular mRNA distribution result from the absence of Btf than from altered recruitment of other splicing factors or partner proteins.
To see if Btf depletion affects the nuclear/cytoplasmic distribution of endogenous mRNAs globally, we performed RNA-FISH using oligo-dT probes to examine polyadenylated RNA in Btf- or TRAP150-depleted HeLa cells ( and ). Expression of Btf or TRAP150 was reduced by their respective siRNA duplexes (). Depletion of Btf with two different siRNA duplexes increased the abundance of cytoplasmic polyadenylated RNA (Fig. 7Af and j) compared with controls (Fig. 7Ab). The ratio of nuclear/cytoplasmic pixel intensity for polyadenylated RNA signal in Btf-depleted cells (0.69 in B1, 0.75 in B2) was less than in cells treated with non-targeting siRNA duplexes (1.9; ). The ratio of nuclear/cytoplasmic pixel intensity for polyadenylated RNA signal in TRAP150-depleted cells (, r and v; 2.0 in T1, 1.79 in T2) was similar to control (, n; 1.9).
Figure 7. Cytoplasmic polyadenylated RNA increases following depletion of Btf but not TRAP150. HeLa cells were transfected with control siRNA, (A) siRNA oligos specific for Btf (BtfSi1 and BtfSi2) or (B) siRNA oligos specific for TRAP150 (TRAPSi1 and TRAPSi4). Cells were processed 72 h post-transfection for RNA-FISH using Texas Red-conjugated oligo-dT50 to visualize polyadenylated RNA. (C) Immunoblots and corresponding densitometry plots showing relative expression levels of Btf or TRAP150. Beta-actin was used as a loading control. Data set shown is a representative of three independent experiments having similar results. Scale bar, 5 µm.
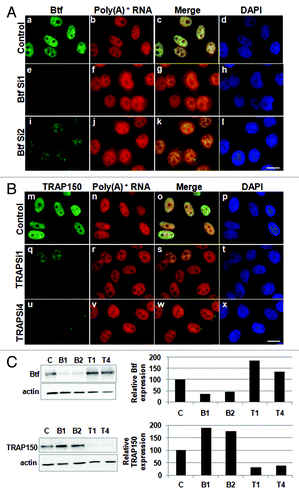
Figure 8. Cytoplasmic polyadenylated RNA increases in Btf-depleted cells when compensatory upregulation of TRAP150 is blocked. (A) HeLa cells were transfected with control siRNA or a mixture of BtfSi1 and TRAP150Si4. 72 h post transfection, the cells were processed for RNA-FISH using Texas Red-conjugated oligo-dT50 to visualize polyadenylated RNA distribution. (B, C) Immunoblots and corresponding densitometry plots showing relative expression levels of Btf or TRAP150. Beta-actin was used as a loading control. Densitometry shows the average of two replicate immunoblots. (D) Graph of nuclear vs. cytoplasmic ratio of polyadenylated RNA. Total fluorescence measurements were performed using ImageJ. Each bar represents the average ratio of pixel intensity for nuclear poly(A)+ RNA to pixel intensity for cytoplasmic poly(A)+ RNA in 25 cells from 3 independent experiments. Error bars represent s.d. of fluorescence intensities. *p values ≤ 0.05. Scale bar, 5 µm.
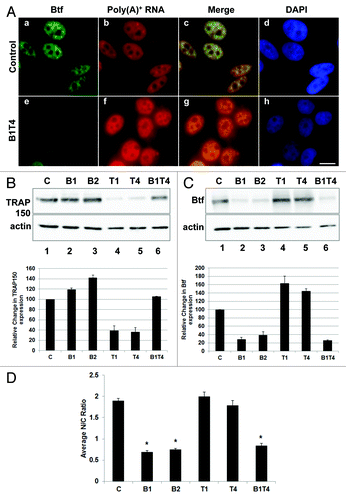
Immunoblots indicated that TRAP150 was upregulated in Btf-depleted cells, while Btf was upregulated in TRAP150-depleted cells ( and ), suggesting that the two proteins may functionally compensate for one another. It also left the question as to whether it is the absence of Btf or the increase in TRAP150 that is responsible for the cytoplasmic increase in polyadenylated RNA. We found that the apparent compensatory TRAP150 expression in Btf-depleted cells could be prevented by addition of limiting amounts of TRAP150 siRNA duplex along with the Btf siRNA duplex (). By adding limiting amounts of TRAP150 siRNA duplex to the siRNA mixture, the level of TRAP150 expression was maintained at levels approximately equal to that of controls (, compare lanes 1 and 6). When TRAP150 upregulation was prevented during Btf depletion, an increase in cytoplasmic polyadenylated RNA was observed as compared with controls (). Our experiments therefore demonstrate that it is Btf depletion, but not compensatory TRAP150 upregulation, that leads to a global change in subcellular distribution of polyadenylated RNA.
Discussion
We used in situ approaches to directly compare the mRNA processing activities of the non-classical SR proteins, Btf and TRAP150. We conclude that while these two proteins have several features in common, their functions are not entirely redundant. Notable differences in subcellular mRNA distribution were observed following knockdown of Btf vs. TRAP150, pointing toward a significant role for these proteins in mRNA export and/or stability. Since Btf and TRAP150 clearly colocalize with pre-mRNA processing factors at transcription sites in situ, it is possible that co-transcriptional recruitment to mRNAs is required for their impact on mRNA export and/or stability. Interestingly, we demonstrated that proteins having distinct functions, such as transcription activators and splicing factors, localize to adjacent yet spatially distinguishable regions within U2OS 2–6-3 transcription sites. We anticipate that the U2OS 2–6-3 gene reporter arrayCitation17 will be useful for the functional categorization of other newly identified proteins having suspected roles in pre-mRNA synthesis and processing.
Clear roles for Btf and TRAP150 in pre-mRNA splicing were not revealed in the reporter systems used here (S. Varia and P. Bubulya, unpublished data). Knockdown of Btf or TRAP150 did not affect constitutive or alternative splicing of our BTM reporter transcripts. However, others have observed that TRAP150 promoted splicing in vivo but not in vitro.Citation9 In addition, Btf co-immunoprecipitated with 9G8 and hnRNP A1.Citation8 Btf knockout in mice was not embryonic lethal,Citation21 and fibroblasts derived from the mice showed altered splicing of adenovirus E1A transcripts.Citation8 TRAP150 activated nuclear mRNA degradation, and both Btf and TRAP150 interacted with splicing factors and EJC proteins such as MLN51, RNPS1, REF, Upf3b, SRm160 and Y14 (Lee et al., 2010) as well as the export factor TAP.Citation8,Citation9,Citation22 Consistent evidence from multiple research groups therefore supports a connection between Btf/TRAP150, the EJC and export factors.
Our data does not rule out that Btf and TRAP150 may affect splicing for a subset of transcripts. In fact, it would be surprising if these proteins do not regulate splicing given that Btf and TRAP150 have been isolated in spliceosomal complexes. TRAP150 is a component of spliceosomal complex B that is loaded onto RNA during splicing, whereas Btf is a component of spliceosomal complex C that is loaded onto RNA along with the EJC proteins.Citation5-Citation7 Btf and TRAP150 also associate with in vitro spliced mRNPs. Btf association with mRNPs is mediated by cap binding complex (CBC), while TRAP150 association is not CBC-dependent.Citation5 Pre-catalytic and catalytically active splicing complexes, assembled in vitro on PM5 pre-mRNA substrates, were isolated and analyzed by mass spectrometry.Citation7 Btf and TRAP150 peptides were detected in both B and C complexes, although they were detected with higher frequency in B complexes. Magoh was detected in both complexes but showed much greater peptide abundance in C complexes, while other EJC components appeared in both B and C complexes. This mass spectrometry data would suggest less overlap of Magoh and Btf/TRAP150 at transcription sites than what we observed. Discrepancies among data sets could reflect differences in stability of interactions that occur on substrates generated and isolated in vitro vs. on nascent transcripts in situ. Given that they do appear in different molecular contexts, Btf and TRAP150 might collaborate with different sets of binding partners that confer distinct functions, or perhaps even promote association with distinct subsets of pre-mRNAs. It is still not clear what steps of splicing may be regulated by Btf or TRAP150. In the future, it will be interesting to examine more globally if knockdown of Btf or TRAP150 causes altered splicing for endogenous transcripts in human cells.
The RS domain in Btf and TRAP150 could interact with other SR splicing factors or export factors. SR proteins do help to mediate interaction between EJC components and nuclear export complexes.Citation23 EJC components including REF/Aly, Y14, SRm160, UAP56, RNPS1 and Magoh are recruited to transcription sites, and splicing of introns is required for the association of these proteins with nascent pre-mRNAs.Citation24 However, while EJC components are recruited to nascent transcripts, some export proteins such as NXF1/TAP are not.Citation24 An interaction between Btf/TRAP150 and TAP could link nascent transcripts with downstream export pathways. In Drosophila, a significant amount of bulk mRNA was cytoplasmic even in cells depleted of all EJC proteins, suggesting that other adaptor proteins mediate NXF1-mRNA interaction.Citation25 One possibility is that Btf or TRAP150 could control whether EJC association with transcripts is stable and could regulate EJC-dependent nonsense-mediated decay.Citation26 Or, they could serve as adaptor proteins to the export factor TAP and assist in the recruitment of mRNA from the sites of active transcription to nuclear pore complexes for export to the cytoplasm.
It was recently shown that EJC components assemble at perispeckle nuclear regions.Citation27,Citation28 Also, polyadenylated RNA and reporter transcripts that could be spliced were present in perispeckles, showing some overlap with active RNAPII.Citation28 Mutant EJC components defective in EJC assembly did not localize to perispeckle regions, and all EJC core proteins that only assemble following splicing were detected at perispeckles, so these regions are more likely to accumulate mRNAs that are spliced and EJC-bound than to simply store EJC factors.Citation28,Citation29 Another study showed pre-mRNAs in nuclear speckles, as well as mRNA accumulation in speckles following knockdown of TREX components UAP56 or Aly, suggesting that TREX components release mRNAs from speckles.Citation30 Targeting of mRNAs around or in nuclear speckles could be an underappreciated mRNP maturation step and would potentially require specific factors such as Btf that can interact both with the EJC as well as other nuclear speckle components via RS-RS interactions. Our experiments showing increased polyadenylated RNA in the cytoplasm specifically in Btf-depleted cells is consistent with the possibility that Btf retains mRNAs in the nucleus or destabilizes them. Since BTM transcripts were modestly elevated in the nucleus following TRAP150 depletion, TRAP150 may promote mRNA export. Alternatively, it may destabilize nuclear mRNA as previously demonstrated.Citation9
Perhaps Btf and TRAP150 are accessory proteins for the EJC, assembling into the mRNP complex in order to modulate downstream events such as mRNA export or stability. Our findings reveal distinct roles for Btf and TRAP150 in regulating mRNA distribution. If the expression of Btf and TRAP150 is directly compensatory, and if this ultimately impacts the assembly of Btf and TRAP150 into mRNP complexes, then modulation of Btf or TRAP150 expression would be a potential mechanism for altering mRNA distribution. Signaling pathways that impact abundance of Btf/ TRAP150, their differential stability upon post-translational modification, and/or their association with mRNPs could therefore have global effects on abundance and subcellular distribution of mRNAs.
Materials and Methods
Plasmid constructs
The full-length Btf cDNA open reading frameCitation31 was subcloned as a SpeI and BamHI into pEYFP-C1 with a modified multiple cloning site. Full length TRAP150 cDNA was a gift from Robert Roeder (Rockefeller University, New York). Full-length TRAP150 cDNA was subcloned as a KpnI + BamHI fragment into pEYFP-C1. The sequence of all constructs obtained using polymerase chain reaction (PCR) products was confirmed by DNA sequencing. YFP-Magoh cDNAs were a kind gift from Catherine Tomasetto (Institute of Genetics and Molecular and Cellular Biology, Université de Strasbourg, France). pSV2-CFP-Lac repressor, pSV2-YFP-Lac repressor, pEYFP-MS2BP and pTetON-YFP were a gift from David Spector (Cold Spring Harbor Laboratory, Cold Spring Harbor, NY).
Cell lines
U2OS 2–6-3 cellsCitation17 were a gift from David Spector (Cold Spring Harbor Laboratory, New York). U2OS 2–6-3 cells stably expressing mcherry-LacI were a gift from Prasanth Kannanganattu (University of Illinois at Urbana-Champaign, IL). BTM HeLa cells were previously described.Citation16
Antibodies
Affinity-purified rabbit polyclonal anti-Btf antibodies were generated at Covance (Denver, PA). Polyclonal antiserum (WU10) was directed against the peptide ERPSTTKDKHKEEDKNS located near the C-terminus of Btf. This peptide sequence is not found in TRAP150. WU10 antibody labels punctate foci in the nucleoplasm of HeLa cells (1:1000; see Fig. S1). Immunofluorescence labeling of Btf is significantly reduced following treatment of the cells with siRNA oligos targeting Btf. WU10 recognizes a band of approximately 150 kDa by immunoblot that migrates at the same position as Btf detected by a commercially available anti-Btf antibody (Bethyl, Cat. No. A300–608A). TRAP150 antibody was purchased from Bethyl (Cat. No. A300–956A). SRSF1 was detected with monoclonal anti-SRSF1/SF2/ASF antibody AK103 (1:2500; provided by Adrian Krainer, Cold Spring Harbor Laboratory, Cold Spring Harbor, NY). EYFP signal did not survive RNA-FISH procedure and for those experiments was detected with monoclonal EGFP antibody (1:100; Clontech, Cat. No. 632375).
Cell culture
U2OS 2–6-3, HeLa cells and BTM cells were grown in Dulbecco's modified eagle medium (DMEM) supplemented with 10% fetal bovine serum and 1% penicillin/streptomycin antibiotic. Cells were incubated at 37°C and 5% CO2. HeLa cells stably expressing the rat β-tropomyosin minigene were described previously.Citation15,Citation16 To inhibit RNA polymerase II transcription, cells were incubated with α-amanitin (50 µg/ml, Sigma-Aldrich, Cat. No. A2263) for 6 h.
Transfection
U2OS 2–6-3 cells were transfected as previously describedCitation17 by electroporation using a Gene Pulser II (BioRad, Hercules, CA) (170 V, 950 µF) to label different regions of the locus. Two µg CFP/YFP-lacR plasmid was used to label locus chromatin, 2 µg YFP-MS2BP plasmid was used to label MS2 stem loops in reporter transcripts, and 2 µg of respective plasmids were used to express various protein factors (rtTA, YFP-Btf, YFP-TRAP150, mCherry-SF2/ASF and YFP-Magoh). Three hours after transfection, the U2OS 2–6-3 gene locus was activated by addition of doxycycline (1 µg/ml) to the culture medium. Cells were processed for microscopy 2.5 h after doxycycline addition. HeLa cells or HeLa BTM cells were suspended in 200 µl of DMEM and transfected by electroporation with 2 µg of YFP-Btf, YFP-TRAP150 or YFP-Magoh DNA along with 40 µg of sheared Salmon Sperm carrier DNA (250 V, 950 µF) in a 4 mm gap cuvette. After electroporation, 1 ml DMEM was added to the cuvette and 200 µl of this suspension was seeded per coverslip for further analysis.
Immunofluorescence
Indirect immunofluorescence was performed as described.Citation13 Briefly, the cells were grown on glass coverslips in a 6-well dish and harvested at 50–60% confluency. Cells were washed with phosphate buffered saline (PBS) and immediately fixed with 2% formaldehyde in PBS for 15 min at room temperature. After three washes with PBS, the cells were then permeabilized with 0.2% Triton X-100 (prepared in PBS) for 5 min at room temperature. Subsequently, the cells were rinsed three times in PBS containing 0.5% normal goat serum (blocking reagent) and incubated in primary antibody for 1 h at room temperature. After three washes with phosphate buffered saline with normal goat serum (PBS/NGS), the cells were incubated in appropriate secondary antibodies conjugated with Texas Red or FITC. DAPI was added to the final PBS wash to label DNA.
RNA fluorescence in situ hybridization (RNA-FISH)
RNA-FISH was performed as described earlier.Citation13,Citation15,Citation16,Citation32 Cells were fixed with 2% formaldehyde for 15 min at room temperature followed by three washes with PBS, 5 min each. Extraction was performed in cytoskeleton (CSK) extraction buffer (100 mM NaCl, 300 mM sucrose, 3 mM MgCl2, 10 mM PIPES pH 6.8, 625 µl of 0.5% Triton-X-100, 2 mM VRC). Hybridization was performed for 3 h at 37°C in hybridization mixture consisting of 2X SSC with 1 mg/ml yeast tRNA, 10% dextran sulfate (w/v), 20% formamide and 20 ng/µl of RNA-FISH probes. Immunofluorescence following RNA-FISH was performed as above, with the exception that 0.5% bovine serum albumin (Amresco, Cat. No. 0903–10G) was used as a blocking agent to maintain RNase-free conditions. Exogenous YFP-Btf, YFP-TRAP150 or YFP-Magoh were transfected 48 h before RNA-FISH. RNA-FISH for poly(A)+ RNA was performed as describedCitation15,Citation32,Citation33 with 80 ng/µl of oligo-dT50 (Sigma-Aldrich, Cat. No. D6758–001) in 2X SSC containing 1 mg/ml yeast t-RNA, 10% dextran sulfate (w/v) and 25% formamide. Hybridization was performed at 42°C overnight. Cells were washed for 30 min in formamide solution at 42°C followed by one wash in 2X SSC for 30 min at room temperature. DAPI was added to the final PBS wash to label DNA.
Microscopy
Z-stacks (0.2 µm intervals) spanning entire nuclei were acquired on a DeltaVision RT using a 60X objective (1.4 numerical aperture; Olympus, Tokyo, Japan) and deconvolved using softWoRx 2.50 software (Deltavision by Applied Precision, Transfection Issaquah, WA). Raw images or deconvolved images were displayed as volume projections. To evaluate the extent of overlap between Btf, TRAP150 and other factors on U2OS 2–6-3 reporter loci, the average Pearson correlation coefficient for 50 loci per coverslip was calculated using SoftWorx software.
Immunoblotting
For immunoblotting of cell extracts, equal amounts of protein (30 µg of total protein) per sample was applied to 7% SDS-PAGE, transferred to nitrocellulose membrane and blocked in PBS containing 0.1% Tween 20 and 5% nonfat dry milk. Anti-Btf WU10 and anti-TRAP150 were used at 1:1000; anti-β-actin (Sigma-Aldrich, Cat. No. A5441) was used at 1:10,000. Secondary antibodies, either horseradish peroxidase (HRP)-conjugated donkey anti-mouse or HRP-conjugated donkey anti-rabbit IgG (Jackson ImmunoResearch Laboratories, Cat. Nos. 711–035–152 and 715–035–150), were used at 1:50,000. HRP was detected by using Pierce ECL western blotting substrate (Thermo Scientific, Cat. No. 32106).
RNA interference (RNAi) and RNA sample preparation
RNAi was performed as previously described.Citation13 Briefly, HeLa cells or HeLa BTM cells (1x105) were plated in antibiotic free medium and after 24 h siRNA mediated transfection was done using Oligofectamine (Invitrogen, Cat. No. 12252–011) alone (mock) or mixed with 60 pmol Dharmacon siRNA duplex targeting luciferase (Dharmacon RNA Technologies Cat. No. D-001210–02;), Btf [siGENOME 1 (D-020734–01) target sequence: GAACAUAGUACUCGGCAAA; siGENOME2 (D-020734–02) target sequence: GGAAUGAGACGACCUUAUG;) or TRAP150 [siGENOME 1 (D-019907–01) target sequence: GGUAUAAGCUCCGAGAUGAUU and siGENOME 4 (D-019907–04) target sequence: GUUGAUCUCCGCCUUGAUAUU]. For double knockdown of Btf and TRAP150, the cells were transfected with BtfSi1 oligos (60 pmoles) and TRAPSi4 (0.86 pmoles). 72 h post-transfection the cells were harvested for further analysis. Total RNA was isolated using the RNeasy kit (Qiagen, Cat. No. 74104). The Cytoplasmic and Nuclear RNA Purification kit (Norgen Biotek, Cat. No. 21000) was used for fractionating nuclear and cytoplasmic RNAs according to manufacturer’s protocols. Genomic DNA was removed from RNA samples using Turbo DNA-free kit (Ambion, Cat. No. AM1907).
PCR analysis
Quantitative RT-PCR was performed using qScript One-Step SYBR Green qRT-PCR kit, Low Rox (Quanta Biosciences, Cat. No. 95059).
An equal amount (100 ng) of nuclear or cytoplasmic RNA was used for each PCR reaction. Primers targeting BTM exon 5 (5′-CCAGGAAGCTGGTGATCCT-3′ and 5′- AGCCACCTCGGCTCTCTC-3′) were used to quantitate BTM transcript levels in . qPCR results were analyzed using the comparative Ct method.Citation34 Nuclear and cytoplasmic RNA were normalized to GAPDH (primers 5′-ATGTTCGTGATGGGTGTGAA-3′ and 5′-GGTGCTAAGCAGTTGGTGGT-3′); GAPDH transcript levels were unaltered in Btf- or TRAP150-depleted samples. Semi-quantitative RT-PCR was performed using primers targeting U2snRNA (5′- CATCGCTTCTCGGCCTTTTG-3′ and 5′-TGGAGGTACTGCAATACCAGG-3′) and S14 RNA (5′-GGCAGACCGAGATGAATCCTC-3′ and 5′- CAGGTCCAGGGGTCTTGGTCC-3′) to validate fractionation of nuclear and cytoplasmic RNA, respectively.
Figure 6. Depletion of Btf does not prevent recruitment of TRAP150 or Magoh to the BTM transcription site. (A) BTM HeLa cells were transiently transfected with YFP-TRAP150 or YFP-Magoh. Twenty-four hours post-transfection, the cells were again transfected with 60 pmoles of either control siRNA (panels a, k and u) or Btf siRNA 1 (panels f, p and z). 72 h after knockdown, the cells were processed for RNA-FISH localization of reporter RNA using oligos against exon 5 (panels b, g, l, q, v and aa) as describedCitation16 and immunofluorescence localization of Btf, splicing factor SF2/ASF or GFP. Arrows indicate the BTM transcription site. DNA was stained with DAPI. Scale Bar, 5 µm. (B) Immunoblot confirming depletion of Btf or TRAP150. Actin was used as a loading control.
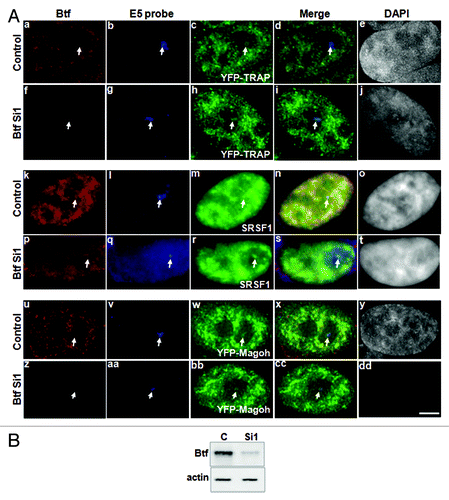
Additional material
Download Zip (3.8 MB)Acknowledgments
We thank S. Janicki (Wistar Institute, Philadelphia, PA) and D. Spector (Cold Spring Harbor Laboratory, Cold Spring Harbor, NY) for developing and providing the parental U2OS 2–6-3 cell line, K.V. Prasanth (Univ. of Illinois, Urbana-Champaign, IL) for providing mcherry-LacI U2OS 2–6-3 and C. Tomasetto (Université de Strasbourg, France) for providing YFP-Magoh plasmid. We thank T. Swigut (Stanford University, Palo Alto, CA), I. Kumaran (Cold Spring Harbor Laboratory, Cold Spring Harbor, NY), S. Baird (Wright State University, Dayton OH), M. Kadakia (Wright State University, Dayton, OH), J. Gomez-Cambronero (Wright State University, Dayton, OH), B. Andrews (Wright State University), Q. Shi (The Ohio State University, Columbus, OH) and V. Tripathi (Univ. of Illinois, Urbana-Champaign, IL) for technical assistance. We thank K.V. Prasanth, S.G. Prasanth (Univ. of Illinois, Urbana-Champaign, IL), J.P. McPherson (University of Toronto, Canada), J. Pence (Wright State University, Dayton OH) and all members of the Bubulya Lab for helpful insights. This work was supported by WSU startup funds, Ohio Board of Regents and NIH GM084407–02 awarded to PAB.
Disclosure of Potential Conflicts of Interest
No potential conflict of interest was disclosed.
References
- Spector DL, Lamond AI. Nuclear speckles. Cold Spring Harb Perspect Biol 2011; 3:3; http://dx.doi.org/10.1101/cshperspect.a000646; PMID: 20926517
- Mintz PJ, Patterson SD, Neuwald AF, Spahr CS, Spector DL. Purification and biochemical characterization of interchromatin granule clusters. EMBO J 1999; 18:4308 - 20; http://dx.doi.org/10.1093/emboj/18.15.4308; PMID: 10428969
- Saitoh N, Spahr CS, Patterson SD, Bubulya P, Neuwald AF, Spector DL. Proteomic analysis of interchromatin granule clusters. Mol Biol Cell 2004; 15:3876 - 90; http://dx.doi.org/10.1091/mbc.E04-03-0253; PMID: 15169873
- Tripathi V, Ellis JD, Shen Z, Song DY, Pan Q, Watt AT, et al. The nuclear-retained noncoding RNA MALAT1 regulates alternative splicing by modulating SR splicing factor phosphorylation. Mol Cell 2010; 39:925 - 38; http://dx.doi.org/10.1016/j.molcel.2010.08.011; PMID: 20797886
- Merz C, Urlaub H, Will CL, Lührmann R. Protein composition of human mRNPs spliced in vitro and differential requirements for mRNP protein recruitment. RNA 2007; 13:116 - 28; http://dx.doi.org/10.1261/rna.336807; PMID: 17095540
- Bessonov S, Anokhina M, Will CL, Urlaub H, Lührmann R. Isolation of an active step I spliceosome and composition of its RNP core. Nature 2008; 452:846 - 50; http://dx.doi.org/10.1038/nature06842; PMID: 18322460
- Wahl MC, Will CL, Lührmann R. The spliceosome: design principles of a dynamic RNP machine. Cell 2009; 136:701 - 18; http://dx.doi.org/10.1016/j.cell.2009.02.009; PMID: 19239890
- Sarras H, Alizadeh Azami S, McPherson JP. In search of a function for BCLAF1. ScientificWorldJournal 2010; 10:1450 - 61; http://dx.doi.org/10.1100/tsw.2010.132; PMID: 20661537
- Lee KM, Hsu IaW, Tarn WY. TRAP150 activates pre-mRNA splicing and promotes nuclear mRNA degradation. Nucleic Acids Res 2010; 38:3340 - 50; http://dx.doi.org/10.1093/nar/gkq017; PMID: 20123736
- Bracken CP, Wall SJ, Barré B, Panov KI, Ajuh PM, Perkins ND. Regulation of cyclin D1 RNA stability by SNIP1. Cancer Res 2008; 68:7621 - 8; http://dx.doi.org/10.1158/0008-5472.CAN-08-1217; PMID: 18794151
- Heyd F, Lynch KW. Phosphorylation-dependent regulation of PSF by GSK3 controls CD45 alternative splicing. Mol Cell 2010; 40:126 - 37; http://dx.doi.org/10.1016/j.molcel.2010.09.013; PMID: 20932480
- Huang S, Spector DL. Intron-dependent recruitment of pre-mRNA splicing factors to sites of transcription. J Cell Biol 1996; 133:719 - 32; http://dx.doi.org/10.1083/jcb.133.4.719; PMID: 8666659
- Sharma A, Takata H, Shibahara K, Bubulya A, Bubulya PA. Son is essential for nuclear speckle organization and cell cycle progression. Mol Biol Cell 2010; 21:650 - 63; http://dx.doi.org/10.1091/mbc.E09-02-0126; PMID: 20053686
- Dimitrova DS. DNA replication initiation patterns and spatial dynamics of the human ribosomal RNA gene loci. J Cell Sci 2011; 124:2743 - 52; http://dx.doi.org/10.1242/jcs.082230; PMID: 21807939
- Prasanth KV, Sacco-Bubulya PA, Prasanth SG, Spector DL. Sequential entry of components of the gene expression machinery into daughter nuclei. Mol Biol Cell 2003; 14:1043 - 57; http://dx.doi.org/10.1091/mbc.E02-10-0669; PMID: 12631722
- Sharma A, Markey M, Torres-Muñoz K, Varia S, Kadakia M, Bubulya A, et al. Son maintains accurate splicing for a subset of human pre-mRNAs. J Cell Sci 2011; 124:4286 - 98; http://dx.doi.org/10.1242/jcs.092239; PMID: 22193954
- Janicki SM, Tsukamoto T, Salghetti SE, Tansey WP, Sachidanandam R, Prasanth KV, et al. From silencing to gene expression: real-time analysis in single cells. Cell 2004; 116:683 - 98; http://dx.doi.org/10.1016/S0092-8674(04)00171-0; PMID: 15006351
- Kasof GM, Goyal L, White E. Btf, a novel death-promoting transcriptional repressor that interacts with Bcl-2-related proteins. Mol Cell Biol 1999; 19:4390 - 404; PMID: 10330179
- Ito M, Okano HJ, Darnell RB, Roeder RG. The TRAP100 component of the TRAP/Mediator complex is essential in broad transcriptional events and development. EMBO J 2002; 21:3464 - 75; http://dx.doi.org/10.1093/emboj/cdf348; PMID: 12093747
- Liu H, Lu Z-G, Miki Y, Yoshida K. Protein kinase C δ induces transcription of the TP53 tumor suppressor gene by controlling death-promoting factor Btf in the apoptotic response to DNA damage. Mol Cell Biol 2007; 27:8480 - 91; http://dx.doi.org/10.1128/MCB.01126-07; PMID: 17938203
- McPherson JP, Sarras H, Lemmers B, Tamblyn L, Migon E, Matysiak-Zablocki E, et al. Essential role for Bclaf1 in lung development and immune system function. Cell Death Differ 2009; 16:331 - 9; http://dx.doi.org/10.1038/cdd.2008.167; PMID: 19008920
- Lai M-C, Lee Y-HW, Tarn W-Y. The DEAD-box RNA helicase DDX3 associates with export messenger ribonucleoproteins as well as tip-associated protein and participates in translational control. Mol Biol Cell 2008; 19:3847 - 58; http://dx.doi.org/10.1091/mbc.E07-12-1264; PMID: 18596238
- Huang Y, Gattoni R, Stévenin J, Steitz JA. SR splicing factors serve as adapter proteins for TAP-dependent mRNA export. Mol Cell 2003; 11:837 - 43; http://dx.doi.org/10.1016/S1097-2765(03)00089-3; PMID: 12667464
- Custódio N, Carvalho C, Condado I, Antoniou M, Blencowe BJ, Carmo-Fonseca M. In vivo recruitment of exon junction complex proteins to transcription sites in mammalian cell nuclei. RNA 2004; 10:622 - 33; http://dx.doi.org/10.1261/rna.5258504; PMID: 15037772
- Gatfield D, Izaurralde E. REF1/Aly and the additional exon junction complex proteins are dispensable for nuclear mRNA export. J Cell Biol 2002; 159:579 - 88; http://dx.doi.org/10.1083/jcb.200207128; PMID: 12438415
- Saulière J, Haque N, Harms S, Barbosa I, Blanchette M, Le Hir H. The exon junction complex differentially marks spliced junctions. Nat Struct Mol Biol 2010; 17:1269 - 71; http://dx.doi.org/10.1038/nsmb.1890; PMID: 20818392
- Schmidt U, Richter K, Berger AB, Lichter P. In vivo BiFC analysis of Y14 and NXF1 mRNA export complexes: preferential localization within and around SC35 domains. J Cell Biol 2006; 172:373 - 81; http://dx.doi.org/10.1083/jcb.200503061; PMID: 16431928
- Daguenet E, Baguet A, Degot S, Schmidt U, Alpy F, Wendling C, et al. Perispeckles are major assembly sites for the exon junction core complex. Mol Biol Cell 2012; 23:1765 - 82; http://dx.doi.org/10.1091/mbc.E12-01-0040; PMID: 22419818
- Degot S, Le Hir H, Alpy F, Kedinger V, Stoll I, Wendling C, et al. Association of the breast cancer protein MLN51 with the exon junction complex via its speckle localizer and RNA binding module. J Biol Chem 2004; 279:33702 - 15; http://dx.doi.org/10.1074/jbc.M402754200; PMID: 15166247
- Dias AP, Dufu K, Lei H, Reed R. A role for TREX components in the release of spliced mRNA from nuclear speckle domains. Nat Commun 2010; 1:97; http://dx.doi.org/10.1038/ncomms1103; PMID: 20981025
- Nagase T, Seki N, Ishikawa K, Tanaka A, Nomura N. Prediction of the coding sequences of unidentified human genes. V. The coding sequences of 40 new genes (KIAA0161-KIAA0200) deduced by analysis of cDNA clones from human cell line KG-1. DNA Res 1996; 3:17 - 24; http://dx.doi.org/10.1093/dnares/3.1.17; PMID: 8724849
- Sacco-Bubulya P, Spector DL. Disassembly of interchromatin granule clusters alters the coordination of transcription and pre-mRNA splicing. J Cell Biol 2002; 156:425 - 36; http://dx.doi.org/10.1083/jcb.200107017; PMID: 11827980
- Huang S, Deerinck TJ, Ellisman MH, Spector DL. In vivo analysis of the stability and transport of nuclear poly(A)+ RNA. J Cell Biol 1994; 126:877 - 99; http://dx.doi.org/10.1083/jcb.126.4.877; PMID: 7519622
- Pfaffl MW. A new mathematical model for relative quantification in real-time RT-PCR. Nucleic Acids Res 2001; 29:e45; http://dx.doi.org/10.1093/nar/29.9.e45; PMID: 11328886