Abstract
Three recent papers, published just weeks apart, describe the use of fluorescent TALEs to tag specific DNA sequences in live cells and, in one case, also in fixed cells, the latter with potential clinical applications.
When Walther Flemming described mitosis at the end of the 19th century,Citation1 the live cell choreography of the chromosomes could be readily inferred, but the advent of phase-contrast microscopyCitation2 and, later, polarized light microscopyCitation3 gave cytologists of that era the thrills of their lifetimes, and this is so today as well. Indeed, I know not a single cell biologist, of any age, who does not rhapsodically marvel when seeing a cell divide, whether for the first or the 1000th time. Metaphase congression and anaphase movement are rarely equaled among the many dramatic phenomena of biology that can be conveyed in real-time. To be sure, everyone has other favorites, e.g., the beguiling differentiation pathway in Dictyostelium, cellularization of the insect embryo, and epiboly in vertebrate pre-gastrula embryos. Recent optogenetics-based video images of action potentials moving along live neuronsCitation4 are another spectacular show, and one can only imagine how much further this amazing technology will go in neurobiology. But while these and many other examples are all striking performances, they do not rise to the venerable status of iconic movies of mitosis or meiosis.
In contrast to observing their dynamic behavior during mitosis and meiosis, watching chromosomes move during interphase (and, to paraphrase Galileo, they do move) was a relatively neglected endeavor until the advent of GFP. The first study involved insertion of lac operators and expression of GFP-tagged lac repressor and revealed that the chromosomes of S. ceresvisiae move in the interphase nucleus by constrained diffusion within confinement volumes,Citation5 later confirmed in mammalian cells.Citation6 Related studies specifically tagged telomeres in live cells either by exposure to a fluorescent oligo that hybridized to telomeres,Citation7 inserting lac operators into the telomeric repeat,Citation8 or tagging telomeres by expressing GFP versions of telomere binding proteins.Citation9 Now, a new approach has been taken, based on transcription activator-like effectors (TALEs). In a flurry of just a few weeks, a boomlet of three papers appearedCitation10-Citation12 that each reported major findings and also, when taken together, signal the dawning of a new era in localizing DNA sequences in live cells.
Transcription activator-like effectors, TALEs, are distinctive types of transcription factors that were discovered in the plant pathogenic bacterial genus Xanthomonas.Citation13 Their specific DNA sequence recognition domains consist of a tandemly repeating array of 34/35 amino acid residues within each of which, variations occurring predominantly at positions 12 and 13 confer remarkable differences in DNA sequence specificity, a property that has been dramatically exploited to design TALEs with extraordinary specificity for targets in a variety of genomes, typically in conjunction with a conjoined nuclease to effect gene resection. However, it soon became apparent that TALEs lacking a nuclease but carrying a fluorescent tag would, in principle, be highly specific probes for DNA sequences, and it is this notion that drove the three recent studies.Citation10-Citation12 All three groups recognized, of course, that the initial proof of principle would need to involve repeated DNA sequences such as the telomeric repeat or centromere-associated satellite DNA sequences in order to maximize detection by the multiply bound TALE-FPs. The overall design principle of the TALE-FPs used in all three studies, with some minor variations, is shown for the case of telomeres in . Note that TALE-FPs can be designed to bind either DNA strand. It is also to be emphasized that the key reason TALEs can be used to detect DNA sequences in live cells, as well as in fixed cells,Citation11 is that they read the DNA sequence of one or the other DNA strand when interrogating it in double-stranded form.
Figure 1. Design of fluorescent TALEs specific for either strand of the human telomeric DNA sequence. The repeating amino acid elements of the two illustrated fluorescent TALEs were designedCitation20 to recognize either the “L” or “R” strand of the telomere sequence. The plasmids encoding these TALEs were constructed with in-frame Venus (yellow) or mCherry (red) fluorescent proteins at the C-termini. Reproduced from reference Citation11 by permission of the Proceedings of the National Academy of Sciences.
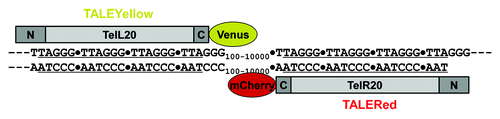
In the first study, Miyanari et al. developed a method they termed TALE-mediated genome visualization (TGV) and used it to label peri-centromeric satellite DNA in mouse embryonic stem cells.Citation10 Following expression of the TALE-GFP encoding plasmids after optimizing the TALE lengths for the DNA targets, they showed that the resulting signals in live cells impressively aligned with centromeres as defined by parallel fluorescent in situ hybridization in fixed cells and went on to track the intranuclear positions and spatial dynamics of these centromeric sequences throughout the cell cycle. These results were confirmed by chromatin immunoprecipitation of the peri-centromeric regions with GFP antibody. The authors then expanded the method to also tag telomeres and to also visualize centromeric DNA sequences in mouse embryonic stem cells and pre-implantation mouse embryos. Having characterized and optimized the method, Miyanari et al. then undertook an elegant genetic application, using their TGV method to differentially tag either of two alleles in mouse F1 embryonic stem cells derived from an inter-specific parental cross in which the interrogated satellite DNA sequences diverged at two positions and found that the TGV method robustly differentiated between the two alleles. This was a remarkable finding, as the authors themselves comment, and speaks to the extraordinary DNA sequence recognition discrimination of TALEs.
Eight weeks after the Miyanari et al. paper appeared, Ma et al. reported the use of fluorescent TALEs to tag both telomeres and centromeres in live human osteosarcoma cells.Citation11 They designed versions of these TALEs with a variety of fluorescent colors and also systematically optimized their lengths. After transfection of the appropriate plasmids, they observed bright signals corresponding to telomeres and centromeres, and by generating a stable cell line expressing a telomere-specific fluorescent TALE, they were able to image the intranuclear dynamics of telomeres in time-lapse videos. In additional experiments they designed fluorescent TALEs specific for centromeric satellite DNA sequences unique to either chromosome 15 or 18, two of the human chromosomes that bear a nucleolus-organizing region (NOR). Using a green TALE specific for the centromere of chromosome 15 and a red TALE for the telomere, they were able to triangulate these two regions in the short arm of this acrocentric chromosome with the NOR, as manifest as the nucleolus, all imaged in the living state. Finally, Ma et al. demonstrated that fluorescent TALEs can be used in fixed cells, analogous to oligonucleotides in standard fluorescent in situ hybridization (FISH). For this version of the method, the TALE’s were produced by coupled in vitro transcription-translation of plasmids encoding a desired telomere-specific TALE in-frame with a fluorescent protein or, in another twist, the use of a plasmid in which a dye-modified amino acid, lysine-green, was incorporated into the translated TALE. With this fixed cell method they were able to detect signals of varying intensity depending on the known average telomere lengths in different HeLa cell lines and were able, using a high-resolution fluorescence-activated cell sorter with an imaging capacity, to resolve and bin distinct populations of cells with different telomere lengths from a given sample and image them one by one, this a set of findings from which they pointed out potential clinical oncology and pathology applications.
The surging momentum for imaging repeated DNA sequences with fluorescent TALEs then continued, with a third publication appearing on Christmas,Citation12 just 16 d after the Ma et al. paper was published. Thanisch et al. designed a GFP-TALE specific for a 19 base-pair satellite repeat and expressed it in mouse embryonic stem cells. They showed that the resulting signals spatially aligned with the targeted sequences by FISH as well as based with protein markers for these chromocenters. One of the longstanding research interests of this group has been DNA replication, and they were able to use their fluorescent TALE labeling method to follow these satellite sequences through S-phase and demonstrate, in live cells, the replication timing of these major satellite sequences and the 3-D intranuclear positions of these late-firing replicons, constituting a reassuring in vivo validation of many biochemical or fixed cell studies. In another important extension of this labeling method, and one not addressed by either of the other two groups, Thanisch et al. conducted fluorescence recovery after photobleaching (FRAP) and fluorescence loss in photobleaching (FLIP) experiments to assess the intranuclear mobility of their GFP-TALE, being prompted to do so by their astute observation that the TALE was released from its chromosomal binding sites during mitosis. They observed a very large immobile fraction when areas around a chromocenter were bleached, compatible with relatively stable binding (during interphase), a conclusion that was reinforced by FLIP, in which large zones around a labeled site were bleached. Detailed analysis of these data and comparison with data for GFP alone led to the conclusion that a very large fraction of the GFP-TALE is quite stably bound to its target sites, but nonetheless with a small fraction that displays a higher off-rate, this a very valuable parameter to know for continuing applications and refinements of TALE-FP’s for DNA sequence visualization in live cells.
How do these new TALE-based methods compare with the previous ones? Although it would be churlish at this early stage to claim an inherent superiority of the TALE-based methods, several points are nonetheless evident. The use of an oligonucleotide to tag telomeresCitation7 has a cost of materials consideration greater than any plasmid-based method and also might be compromised in certain cells where the (passive or delivered) uptake and/or metabolic stability of the oligo is limiting. The method based on insertion of lac operatorsCitation8 requires more plasmid construction and cloning than in the TALE-based methods, as well as the generation of stable cell lines. In addition, the telomeres at only one arm of each of only three chromosomes were tagged in that study, and one would ideally want complete coverage across the entire karyotype.
With the advent of this body of work using TALEs to localize and track DNA sequences, one might have predicted that it would only be a matter of time before the small guide RNAs (sgRNAs) of the CRISPR/Cas system might be similarly harnessed. It didn’t take long. On December 19, 2013, half-way between the Ma et al. (December 9) and Thanisch et al. (December 25) papers, a repeated DNA sequence localization study appeared based on the CRISPR/Cas system,Citation14 and another CRISPR/Cas-based study localizing human chromosomal loci is imminent,Citation15 adding to the momentum of these new approaches to the 21st century toolkit of chromosomal sequence visualization.
With all this new, exciting progress, it is worthwhile to think back to the beginning, as epistemology. Everything in the half century of research on transcriptional regulation since Jacob and MonodCitation16,Citation17 would have predicted that every single transcription factor would have to possess very high DNA sequence recognition specificity, although neither they nor anyone else could have anticipated the magnitude of transcription factor diversity, the fact that these factors use cunning amino acid side-chain stereochemistry to read the DNA sequence,Citation18 and that there would ever be something like GFP. Nor could anyone have imagined that a bacterial genus that infects plants uses a refined type of transcription factors, TALEs, or that another amazing bacterial gene regulation pathway (CRISPR/Cas) was out there. Are there any better examples of how basic, unfettered research can lead to breakthrough progress? As we look forward to new applications of TALEs and CRISPR/Cas technology in genomics, let us endeavor to remind the public that, once again, the most fundamental biological research continues to bring these amazing discoveries and empower the future, an endless frontier.Citation19
Disclosure of Potential Conflicts of Interest
No potential conflict of interest was disclosed.
Acknowledgments
The cited work from the author’s laboratory was supported by grant MCB-1051398 from the U.S. National Science Foundation.
References
- Flemming W. Zell-substanz, Kern und Zelltheilung. Leipzig; Verlag von F.C.W. Vogel: 1882 .
- Zernike F. The concept of degree of coherence and its application to optical problems. Physica 1938; 5:785 - 95; http://dx.doi.org/10.1016/S0031-8914(38)80203-2
- Inoué S. [Polarization optical studies of the mitotic spindle. I. The demonstration of spindle fibers in living cells]. Chromosoma 1953; 5:487 - 500; PMID: 13082662
- Venkatachalam V, Brinks D, Maclaurin D, Hochbaum D, Kralj J, Cohen AE. Flash memory: photochemical imprinting of neuronal action potentials onto a microbial rhodopsin. J Am Chem Soc 2014; 27:1 - 3; PMID: 24428326
- Marshall WF, Straight A, Marko JF, Swedlow J, Dernburg A, Belmont A, Murray AW, Agard DA, Sedat JW. Interphase chromosomes undergo constrained diffusional motion in living cells. Curr Biol 1997; 7:930 - 9; http://dx.doi.org/10.1016/S0960-9822(06)00412-X; PMID: 9382846
- Bronstein I, Israel Y, Kepten E, Mai S, Shav-Tal Y, Barkai E, Garini Y. Transient anomalous diffusion of telomeres in the nucleus of mammalian cells. Phys Rev Lett 2009; 103:018102; http://dx.doi.org/10.1103/PhysRevLett.103.018102; PMID: 19659180
- Molenaar C, Wiesmeijer K, Verwoerd NP, Khazen S, Eils R, Tanke HJ, Dirks RW. Visualizing telomere dynamics in living mammalian cells using PNA probes. EMBO J 2003; 22:6631 - 41; http://dx.doi.org/10.1093/emboj/cdg633; PMID: 14657034
- Wang X, Kam Z, Carlton PM, Xu L, Sedat JW, Blackburn EH. Rapid telomere motions in live human cells analyzed by highly time-resolved microscopy. Epigenetics Chromatin 2008; 1:4; http://dx.doi.org/10.1186/1756-8935-1-4; PMID: 19014413
- Jegou T, Chung I, Heuvelman G, Wachsmuth M, Görisch SM, Greulich-Bode KM, Boukamp P, Lichter P, Rippe K. Dynamics of telomeres and promyelocytic leukemia nuclear bodies in a telomerase-negative human cell line. Mol Biol Cell 2009; 20:2070 - 82; http://dx.doi.org/10.1091/mbc.E08-02-0108; PMID: 19211845
- Miyanari Y, Ziegler-Birling C, Torres-Padilla M-E. Live visualization of chromatin dynamics with fluorescent TALEs. Nat Struct Mol Biol 2013; 20:1321 - 4; http://dx.doi.org/10.1038/nsmb.2680; PMID: 24096363
- Ma H, Reyes-Gutierrez P, Pederson T. Visualization of repetitive DNA sequences in human chromosomes with transcription activator-like effectors. Proc Natl Acad Sci U S A 2013; 110:21048 - 53; http://dx.doi.org/10.1073/pnas.1319097110; PMID: 24324157
- Thanisch K, Schneider K, Morbitzer R, Solovei I, Lahaye T, Bultmann S, Leonhardt H. Targeting and tracing of specific DNA sequences with dTALEs in living cells. [Epub ahead of print] Nucleic Acids Res 2013; http://dx.doi.org/10.1093/nar/gkt1348; PMID: 24371265
- Boch J, Bonas U. Xanthomonas AvrBs3 family-type III effectors: discovery and function. Annu Rev Phytopathol 2010; 48:419 - 36; http://dx.doi.org/10.1146/annurev-phyto-080508-081936; PMID: 19400638
- Chen B, Gilbert LA, Cimini BA, Schnitzbauer J, Zhang W, Li GW, Park J, Blackburn EH, Weissman JS, Qi LS, et al. Dynamic imaging of genomic loci in living human cells by an optimized CRISPR/Cas system. Cell 2013; 155:1479 - 91; http://dx.doi.org/10.1016/j.cell.2013.12.001; PMID: 24360272
- Anton T, Bultmann S, Leonhardt H, Markaki Y. Visualization of specific DNA sequences in living mouse embryo stem cells with a programmable fluorescent CRISPR/Cas system. Nucleus 2014; 5; Forthcoming http://dx.doi.org/10.4161/nucl.28488
- Jacob F, Monod J. Genetic regulatory mechanisms in the synthesis of proteins. J Mol Biol 1961; 3:318 - 56; http://dx.doi.org/10.1016/S0022-2836(61)80072-7; PMID: 13718526
- Ptashne M. The chemistry and regulation of genes and other things. J Biol Chem 2014; In press http://dx.doi.org/10.1074/jbc.X114.547323; PMID: 24385432
- Sauer RT, Yocum RR, Doolittle RF, Lewis M, Pabo CO. Homology among DNA-binding proteins suggests use of a conserved super-secondary structure. Nature 1982; 298:447 - 51; http://dx.doi.org/10.1038/298447a0; PMID: 6896364
- Science the endless frontier. A Report to the President by Vannevar Bush, Director of the Office of Scientific Research and Development. United States Government Printing Office, Washington, DC; 1945.
- Sanjana NE, Cong L, Zhou Y, Cunniff MM, Feng G, Zhang F. A transcription activator-like effector toolbox for genome engineering. Nat Protoc 2012; 7:171 - 92; http://dx.doi.org/10.1038/nprot.2011.431; PMID: 22222791