Abstract
Nuclear pore complexes are large macromolecular assemblies that facilitate the nucleocytoplasmic exchange of macromolecules. Because of their intricate composition, membrane association, and sheer size, the integration of various, complementary structure determination approaches is a prerequisite for elucidating their structure. We have recently employed such an integrated strategy to analyze the scaffold structure of the cytoplasmic and nuclear rings of the human nuclear pore complex. In this extra view, we highlight two specific aspects of this work: the power of electron microscopy for bridging different resolution regimes and the importance of post-translational modifications for regulating nucleoporin interactions. We review recent technological developments and give a perspective toward future structure determination approaches.
Nuclear pore complexes (NPCs) are huge macromolecular assemblies that span the inner and outer nuclear membranes and facilitate nucleocytoplasmic exchange of macromolecules. Due to their enormous size of about 120 nm in diameter, elucidating their structure is an enormous challenge. In the last decade, X-ray crystallography has provided major insights into the fold organization of various nucleoporins and the structure of many protein interfaces.Citation1 It has become clear that scaffold Nups are organized in a modular way and share α-helical repeat and β propeller domain folds.Citation2 Electron microscopy (EM) has revealed the overall shape of some of the subcomplexes, in particular the yeast Nup84 subcomplex.Citation3,Citation4 Taken together with structural modeling approaches, X-ray structures could be localized within the EM map of the yNup84 subcomplex.Citation4-Citation6 Such information, taken together with biochemical data, was integrated into models of the overall architectural organization of the NPC (reviewed, e.g., in ref. Citation1). Although electron tomography had previously provided density maps of fully assembled NPCs, structures of subcomplexes could not unambiguously be assigned into the structural framework. We have recently generated a tomographic map of the human NPC that is sufficiently resolved to detect structural signatures of larger subcomplexes.Citation7
A three-dimensional map was generated from cryo electron tomograms of purified HeLa cell nuclear envelopes that were analyzed by subtomogram averaging (). It shows that the nuclear and cytoplasmic rings of the nuclear pore are elevated from the outer and inner nuclear membranes by about 10 nm. Both rings are connected to the membrane by two rod-shaped elements per asymmetric unit (). Within each ring and asymmetric unit, these elements are positioned very close to each other. All four of these emanate into a Y-shaped element that we refer to as two pairs of the outer and inner vertices, respectively due to slightly different distances from the center. These show significant similarity to the structure of the isolated hNup107 subcomplex ().
Figure 1. Tomographic map of the human NPC. (A) Isosurface-rendered view of the human NPC as seen from the cytoplasm. The arrowhead indicates the membrane-touching point of the rod-shaped density connecting the cytoplasmic and spoke ring. Dotted and dashed lines mark density that could resemble the rough shape of Nups 155 and 205 as determined in isolation,Citation8 although an unambiguous assignment is not yet possible. (B) Same as (A) but seen from the luminal space. Arrowheads indicate the four membrane-touching points per asymmetric unit attributed to the four vertices. The positions of the two membrane-touching points of the two inner vertices rotationally register; the two corresponding points of the outer vertices do not. (C) Isosurface-rendered view of four consecutive slices through the cytoplasmic ring highlighting the similarity of the inner and outer vertex position with the structure of the isolated human Nup107 subcomplex (shown superimposed according to their optimized fit in bright blue in the right panels). The corresponding region is framed in (A). The two bottom panels have slightly different rotational states around the nucleocytoplasmic axis. The panel labeled “Inner vertex-outside view” also slices through the outer vertex of the adjacent asymmetric unit in the right third of the image.
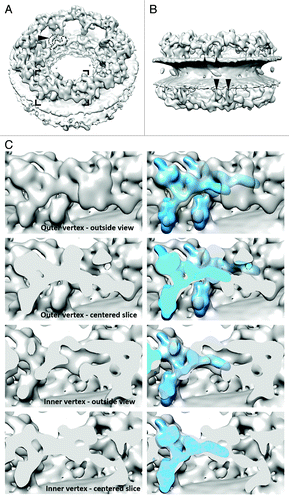
Another rod shaped element per asymmetric unit connects each, the cytoplasmic and nuclear ring, to the spoke ring. This connector element touches the membrane right at its merging point into the spoke ring (). One might speculate that Nup53 is positioned there, while the adjacent shapes could be attributed to Nups 155 and 205 (). Such an assignment, although intriguing, is however speculative at this point, since the crescent shapes typical for Nups 205 and 188Citation8-Citation10 are observed in multiple orientations and positions throughout the entire structure and the entire mid-plane of the spoke ring, which contributes a considerable amount of density, would remain unexplained.
The improved resolution of the tomographic structure was primarily enabled by the development of automatized data acquisition protocols that have dramatically improved the data acquisition throughput. Nowadays, the collection of about 50 tomograms per day without any user interference has become feasible. It has recently been shown that subtomogram averaging holds the potential to resolve secondary structure.Citation11 Whether this will be achieved for nuclear pores in the near future depends on several conditions: (1) Structure determination of membrane embedded, intact NPCs relies on relatively thick specimen, such as nuclear envelopes. It is thus dependent on energy-filtered electron detectors that reduce the contribution of inelastic electron scattering events observed on the camera. Next generation direct electron detectors coupled to energy filters have recently become available and should be explored for structure determination of NPCs. They promise to increase the signal to noise ratio of the primary data at least 2-fold.Citation12 (2) The data acquisition throughput might be even further increased, although this requires considerable infrastructure investments into CPU cycles and hard disk space. (3) However, the flexibility of the nuclear pore structure itself or even compositional heterogeneity across asymmetric units, might limit the attainable resolution. If so, this intrinsic property will be very difficult to overcome but might be tackled with further development of image classification algorithms.
An important caveat about tomographic structures is that it is not entirely clear which parts of the nuclear pore are averaged out during the structure determination process because they are flexible. On the other hand, even non-nucleoporins might contribute to observed electron optical density. One might assume that intrinsically disordered domains (IDPs) are averaged out while structured scaffold and anchoring domains are retained. However, scaffold Nups might be also flexible to quite some degree, while IDPs might generate structured elements. The latter is nicely illustrated by the fact that the dynein light chain-interacting (DID) domain of yNup159 that is classified as an IDP, forms a highly structured rod when interacting with dynein.Citation13
In order to interpret the tomographically observed electron optical density, it will be of utmost importance to further characterize isolated subcomplexes by single particle EM. Unfortunately, this has turned out challenging for Nups. Because of their membrane-associated nature, Nup subcomplexes often assume a preferred orientation on EM grids and may even disintegrate during the preparation of grids. To the best of our knowledge, no cryoEM structures of subcomplexes have been reported thus far. Although negative staining EM has revealed very important insights into subcomplex structures, one needs to bear in mind that the available negative staining structures might have been flatted along one spatial direction during the staining procedure. This problem can potentially be addressed by exposing the sample to less favorable conditions for surface adherence during EM grid preparation and the application of thicker embedded staining as we have done for the hNup107 subcomplex.Citation7 A promising alternative is the use of functionalized surfacesCitation14,Citation15 or even membranes that were successfully used for elucidating structures of coated vesicles using tomographic approaches.Citation16
Another important aspect to take into account when studying the structure of nuclear pores and their subcomplexes is that they are quite heavily post-transnationally modified. Prominent post-translational modifications (PTMs) of Nups are phosphorylation,Citation17-Citation20 O-glycosylation,Citation21-Citation23 N-glycosylation,Citation24 sumoylation,Citation25 ubiquintination,Citation26 and acetylation.Citation27 Advances in the mass spectrometry hardware, such as, e.g., the increased scanning speed of Orbitrap instruments or the introduction of electron dissociation transfer (ETD) fragmentation together with specific peptide enrichment strategies have permitted the large-scale identification of PTMs. Recent large scale studies have discovered a considerable number of PTM sites of Nups, see, e.g., references Citation20,Citation23,Citation25,Citation27. To the best of our knowledge, the regulatory function of at least some of PTMs abundant in Nups, e.g., acetylation, remain largely understudied. Interestingly, some of these, e.g., O-glycosylation and phosphorylation, have a high likelihood to co-occur at neighboring residues and to co-evolveCitation28 and might even interplay at the same residueCitation23 such as, e.g., acetylation and sumoylation.Citation27 These PTMs modulate various functional aspects of the nucleocytoplasmic transport system. For example, it is known that vertebrate FG Nups are modified by O-linked glycosylation,Citation22 and this may regulate the vertebrate NPC permeability barrier.Citation29 Phosphorylation of Nup98 is a key step during mitotic disassembly of NPCsCitation30 and high-throughput studies have demonstrated that various Nups are heavily phosphorylated during mitosis.Citation20 One might thus assume that phosphorylation is important to regulate inter-subcomplex interfaces of nucleoporins and that the hNup107 subcomplex is potentially a key player in the events of open mitosis. In line with this hypothesis, we have recently shown that phosphorylation sites map in potential mitotically regulated inter-subcomplex interfaces of the overall Y subcomplex, Phosphorylation sites within ELYS, Nup133 and Nup96 localize into these essential inter-subcomplex contact sites, further underlining the hypothesis that such areas are prime targets for investigating the disassembly and re-assembly mechanism of temporal cell cycle intermediates.Citation7 We mapped several phosphorylation sites in the pertinent sections of Nup160 that might be functionally relevant for NPC assembly. Also the distal N-terminus of Nup133 is heavily phosphorylated, likely to control the head-to-tail interaction of the hNup107 subcomplex in this flexible region. The N-terminal part of Nup96, which appears highly flexible in isolated subcomplexes, is heavily phosphorylated suggesting that it might assume a different conformation in assembled NPCs, where it localizes into the vertex interface.Citation7 These regions are prime for regulatory activity.
Confirmed in our work are 48 previously not annotated serine/threonine phosphorylation sites within the hNup107 subcomplex. By combining affinity isolation of mitotic Nups with the enrichment of phosphorylated peptides, we were able to identify a considerably larger number of phospho-peptides within the hNup107 subcomplex than aforementioned high-throughput studies that solely relied on a phospho-capturing strategy. This example demonstrates that similar biochemical strategies might be employed for various PTMs and subcomplexes in order to more comprehensively map the PTM landscape of Nups across different biological states. Such data will be crucial to understand how PTMs modulate NPC structure. Furthermore, it will be important to conduct such studies in vertebrate and mammalian cells since PTMs seem to play important roles in biological processes that are absent in yeast, such as mitotic nuclear envelope breakdown and embryonic development. Notably, phosphorylation sites are also observed in yeast Nups and likely have other important regulatory roles.
Advancing toward a high-resolution structure of the vertebrate NPC clearly requires progress on various different experimental fronts other than discussed above. For example, some domain folds and a large number of protein interfaces await high-resolution structural analysis. Separate targeting of poorly or non-conserved nucleoporins from lower and higher eukaryotes seems necessary. The fact that some nucleoporin domains are obviously engaged in multiple distinct protein interactions thereby imposes an additional layer of complexity. A better understanding of protein-lipid interactions as well as the contribution of IDPs to the scaffold architecture is needed as well. Finally, joint efforts of various laboratories would be the appropriate way to tackle the data integration challenge, since the weight of different types of data is often difficult to judge individually.
Disclosure of Potential Conflicts of Interest
No potential conflict of interest was disclosed.
Acknowledgments
M.B. acknowledges funding by the European Research Council (309271-NPCAtlas).
References
- Hoelz A, Debler EW, Blobel G. The structure of the nuclear pore complex. Annu Rev Biochem 2011; 80:613 - 43; http://dx.doi.org/10.1146/annurev-biochem-060109-151030; PMID: 21495847
- Schwartz TU. Modularity within the architecture of the nuclear pore complex. Curr Opin Struct Biol 2005; 15:221 - 6; http://dx.doi.org/10.1016/j.sbi.2005.03.003; PMID: 15837182
- Flemming D, Thierbach K, Stelter P, Böttcher B, Hurt E. Precise mapping of subunits in multiprotein complexes by a versatile electron microscopy label. Nat Struct Mol Biol 2010; 17:775 - 8; http://dx.doi.org/10.1038/nsmb.1811; PMID: 20512149
- Kampmann M, Blobel G. Three-dimensional structure and flexibility of a membrane-coating module of the nuclear pore complex. Nat Struct Mol Biol 2009; 16:782 - 8; http://dx.doi.org/10.1038/nsmb.1618; PMID: 19503077
- Fernandez-Martinez J, Phillips J, Sekedat MD, Diaz-Avalos R, Velazquez-Muriel J, Franke JD, Williams R, Stokes DL, Chait BT, Sali A, et al. Structure-function mapping of a heptameric module in the nuclear pore complex. J Cell Biol 2012; 196:419 - 34; http://dx.doi.org/10.1083/jcb.201109008; PMID: 22331846
- Nagy V, Hsia KC, Debler EW, Kampmann M, Davenport AM, Blobel G, Hoelz A. Structure of a trimeric nucleoporin complex reveals alternate oligomerization states. Proc Natl Acad Sci U S A 2009; 106:17693 - 8; http://dx.doi.org/10.1073/pnas.0909373106; PMID: 19805193
- Bui KH, von Appen A, DiGuilio AL, Ori A, Sparks L, Mackmull MT, Bock T, Hagen W, Andrés-Pons A, Glavy JS, et al. Integrated structural analysis of the human nuclear pore complex scaffold. Cell 2013; 155:1233 - 43; http://dx.doi.org/10.1016/j.cell.2013.10.055; PMID: 24315095
- Amlacher S, Sarges P, Flemming D, van Noort V, Kunze R, Devos DP, Arumugam M, Bork P, Hurt E. Insight into structure and assembly of the nuclear pore complex by utilizing the genome of a eukaryotic thermophile. Cell 2011; 146:277 - 89; http://dx.doi.org/10.1016/j.cell.2011.06.039; PMID: 21784248
- Andersen KR, Onischenko E, Tang JH, Kumar P, Chen JZ, Ulrich A, Liphardt JT, Weis K, Schwartz TU. Scaffold nucleoporins Nup188 and Nup192 share structural and functional properties with nuclear transport receptors. Elife 2013; 2:e00745; http://dx.doi.org/10.7554/eLife.00745; PMID: 23795296
- Sampathkumar P, Kim SJ, Upla P, Rice WJ, Phillips J, Timney BL, Pieper U, Bonanno JB, Fernandez-Martinez J, Hakhverdyan Z, et al. Structure, dynamics, evolution, and function of a major scaffold component in the nuclear pore complex. Structure 2013; 21:560 - 71; http://dx.doi.org/10.1016/j.str.2013.02.005; PMID: 23499021
- Schur FK, Hagen WJ, de Marco A, Briggs JA. Determination of protein structure at 8.5Å resolution using cryo-electron tomography and sub-tomogram averaging. J Struct Biol 2013; 184:394 - 400; http://dx.doi.org/10.1016/j.jsb.2013.10.015; PMID: 24184468
- Ruskin RS, Yu Z, Grigorieff N. Quantitative characterization of electron detectors for transmission electron microscopy. J Struct Biol 2013; 184:385 - 93; http://dx.doi.org/10.1016/j.jsb.2013.10.016; PMID: 24189638
- Stelter P, Kunze R, Flemming D, Höpfner D, Diepholz M, Philippsen P, Böttcher B, Hurt E. Molecular basis for the functional interaction of dynein light chain with the nuclear-pore complex. Nat Cell Biol 2007; 9:788 - 96; http://dx.doi.org/10.1038/ncb1604; PMID: 17546040
- Kelly DF, Abeyrathne PD, Dukovski D, Walz T. The Affinity Grid: a pre-fabricated EM grid for monolayer purification. J Mol Biol 2008; 382:423 - 33; http://dx.doi.org/10.1016/j.jmb.2008.07.023; PMID: 18655791
- Kelly DF, Dukovski D, Walz T. Monolayer purification: a rapid method for isolating protein complexes for single-particle electron microscopy. Proc Natl Acad Sci U S A 2008; 105:4703 - 8; http://dx.doi.org/10.1073/pnas.0800867105; PMID: 18347330
- Faini M, Prinz S, Beck R, Schorb M, Riches JD, Bacia K, Brügger B, Wieland FT, Briggs JA. The structures of COPI-coated vesicles reveal alternate coatomer conformations and interactions. Science 2012; 336:1451 - 4; http://dx.doi.org/10.1126/science.1221443; PMID: 22628556
- Favreau C, Worman HJ, Wozniak RW, Frappier T, Courvalin JC. Cell cycle-dependent phosphorylation of nucleoporins and nuclear pore membrane protein Gp210. Biochemistry 1996; 35:8035 - 44; http://dx.doi.org/10.1021/bi9600660; PMID: 8672508
- Glavy JS, Krutchinsky AN, Cristea IM, Berke IC, Boehmer T, Blobel G, Chait BT. Cell-cycle-dependent phosphorylation of the nuclear pore Nup107-160 subcomplex. Proc Natl Acad Sci U S A 2007; 104:3811 - 6; http://dx.doi.org/10.1073/pnas.0700058104; PMID: 17360435
- Lusk CP, Waller DD, Makhnevych T, Dienemann A, Whiteway M, Thomas DY, Wozniak RW. Nup53p is a target of two mitotic kinases, Cdk1p and Hrr25p. Traffic 2007; 8:647 - 60; http://dx.doi.org/10.1111/j.1600-0854.2007.00559.x; PMID: 17461799
- Olsen JV, Vermeulen M, Santamaria A, Kumar C, Miller ML, Jensen LJ, Gnad F, Cox J, Jensen TS, Nigg EA, et al. Quantitative phosphoproteomics reveals widespread full phosphorylation site occupancy during mitosis. Sci Signal 2010; 3:ra3; http://dx.doi.org/10.1126/scisignal.2000475; PMID: 20068231
- Finlay DR, Forbes DJ. Reconstitution of biochemically altered nuclear pores: transport can be eliminated and restored. Cell 1990; 60:17 - 29; http://dx.doi.org/10.1016/0092-8674(90)90712-N; PMID: 2295087
- Miller MW, Caracciolo MR, Berlin WK, Hanover JA. Phosphorylation and glycosylation of nucleoporins. Arch Biochem Biophys 1999; 367:51 - 60; http://dx.doi.org/10.1006/abbi.1999.1237; PMID: 10375398
- Wang Z, Udeshi ND, Slawson C, Compton PD, Sakabe K, Cheung WD, Shabanowitz J, Hunt DF, Hart GW. Extensive crosstalk between O-GlcNAcylation and phosphorylation regulates cytokinesis. Sci Signal 2010; 3:ra2; http://dx.doi.org/10.1126/scisignal.2000526; PMID: 20068230
- Wozniak RW, Bartnik E, Blobel G. Primary structure analysis of an integral membrane glycoprotein of the nuclear pore. J Cell Biol 1989; 108:2083 - 92; http://dx.doi.org/10.1083/jcb.108.6.2083; PMID: 2738089
- Golebiowski F, Matic I, Tatham MH, Cole C, Yin Y, Nakamura A, Cox J, Barton GJ, Mann M, Hay RT. System-wide changes to SUMO modifications in response to heat shock. Sci Signal 2009; 2:ra24; http://dx.doi.org/10.1126/scisignal.2000282; PMID: 19471022
- Hayakawa A, Babour A, Sengmanivong L, Dargemont C. Ubiquitylation of the nuclear pore complex controls nuclear migration during mitosis in S. cerevisiae. J Cell Biol 2012; 196:19 - 27; http://dx.doi.org/10.1083/jcb.201108124; PMID: 22213798
- Choudhary C, Kumar C, Gnad F, Nielsen ML, Rehman M, Walther TC, Olsen JV, Mann M. Lysine acetylation targets protein complexes and co-regulates major cellular functions. Science 2009; 325:834 - 40; http://dx.doi.org/10.1126/science.1175371; PMID: 19608861
- Minguez P, Parca L, Diella F, Mende DR, Kumar R, Helmer-Citterich M, Gavin AC, van Noort V, Bork P. Deciphering a global network of functionally associated post-translational modifications. Mol Syst Biol 2012; 8:599; http://dx.doi.org/10.1038/msb.2012.31; PMID: 22806145
- Labokha AA, Gradmann S, Frey S, Hülsmann BB, Urlaub H, Baldus M, Görlich D. Systematic analysis of barrier-forming FG hydrogels from Xenopus nuclear pore complexes. EMBO J 2013; 32:204 - 18; http://dx.doi.org/10.1038/emboj.2012.302; PMID: 23202855
- Laurell E, Beck K, Krupina K, Theerthagiri G, Bodenmiller B, Horvath P, Aebersold R, Antonin W, Kutay U. Phosphorylation of Nup98 by multiple kinases is crucial for NPC disassembly during mitotic entry. Cell 2011; 144:539 - 50; http://dx.doi.org/10.1016/j.cell.2011.01.012; PMID: 21335236