Abstract
Rad26p is a SWI/SNF-like ATPase in yeast, and is conserved among eukaryotes. Both Rad26p and its human homolog CSB (Cockayne syndrome group B) are involved in regulation of chromatin structure, transcription and DNA repair. Thus, mutations or malfunctions of these proteins have significant effects on cellular functions. Mutations in CSB are associated with Cockayne syndrome (CS) that is characterized by heterogeneous pathologies such as mental and physical retardation, sun sensitivity, premature aging, muscular and skeletal abnormalities, and progressive decline in neurological and cognitive functions. Therefore, many research groups focused their studies to understand the mechanisms of Rad26p/CSB functions to illuminate the molecular bases of CS. These studies have provided significant functional and mechanistic insights of Rad26p/CSB in regulation of gene expression and genome integrity as described here.
Introduction
Rad26p is a SWI/SNF-like ATPase in yeast, first identified in humans as ERCC6/CSB (Cockayne syndrome group B) in patients with Cockayne syndrome (CS). Both Rad26p and CSB are associated with the repair of actively transcribing strand (TS), a process termed as transcription-coupled repair (TCR).Citation1-5 Mutations in CSB lead to defective TCR, physiologically reflected as a severe disorder called CS.Citation6 Symptoms of CS include premature aging, photosensistivity, cataracts, retinal degeneration, dental caries, hearing loss, muscular and skeletal abnormalities, and progressive decline in neurological and cognitive functions.Citation6 Prognosis of CS in humans is degenerative and terminal illness with death by the average age of 12 years. The CSB gene, mutated in about 80% of the CS patients, plays an important role in TCR. Likewise, its yeast homolog Rad26p is involved in TCR.Citation2,3,5 Further, these proteins have also been implicated in regulation of chromatin structure and transcriptional elongation.Citation7-12 Such diverse functions are attributed to contribute to the wide-spectrum of clinical features of the CS patients with mutations in CSB. Here, we provide functional and mechanistic insights of Rad26p in regulation of chromatin structure, transcription, and DNA repair. In addition, we have also described below CSB regulation of these processes.
Regulation of TCR by Rad26p
Genomic DNA is continuously damaged by numerous factors and/or agents.Citation13-15 However, an intricate network of mechanisms has evolved to protect genomes in both prokaryotic and eukaryotic organisms.Citation16,17 The most versatile process for handling a large variety of structurally-unrelated DNA lesions (e.g., chemical adducts and UV-induced cyclobutane pyrimidine dimers or CPDs and 6–4 photoproducts) is nucleotide excision repair (NER). Other DNA damages such as oxidative lesions and small base alterations are handled by base excision repair (BER).Citation18,19 In addition, the cells also face more hazardous conditions where both the strands of DNA are simultaneously damaged, leading to DNA double-strand break (DSB). DSBs are repaired via non-homologous end-joining and/or homologous recombination.Citation20-24
When DNA damage blocks transcription, the lesion is removed by TCR. TCR was first evident about 30 years ago in a studyCitation25 that demonstrated faster DNA repair in an actively transcribing DHFR gene as compared with the genome overall in the Chinese hamster ovary cells. This was subsequently corroborated by another studyCitation26 that showed a preferential and efficient repair of CPDs in the TS of the mammalian DHFR gene in comparison to the repair at the non-transcribing strand (NTS). In agreement, the blocking of transcriptional elongation by α-amanitin inhibits DNA repair at the active gene in mammalian cells.Citation27 Similarly, the temperature-sensitive inactivation of Rpb1p, the largest subunit of RNA polymerase II, abolishes transcription, and reduces the rate of DNA repair at the TS to that of the NTS in yeast.Citation28 Likewise, TCR is also observed in bacteria.Citation29 Thus, TCR occurs in both prokaryotic and eukaryotic organisms.
TCR is one of the two sub-pathways of NER, and thus, is also known as TC-NER (transcription-coupled NER). The other sub-pathway of NER is the global genomic NER (GG-NER) that is responsible for removal of lesions throughout the genome. Both TC-NER and GG-NER follow common steps for repairing DNA lesion. However, the DNA damage recognition step differs between GG-NER and TC-NER. The DNA lesions in the active genes are recognized quickly in a transcription-dependent manner, resulting in faster TC-NER in comparison to GG-NER. Like NER, BER has also been recently shown to be facilitated by transcription, hence supporting the existence of TC-BER.Citation30 Similarly, our recent resultsCitation31 demonstrated that DSB at the active gene is repaired faster than that at the inactive gene, supporting TC-DSBR (transcription-coupled DSB repair). Thus, like NER, BER and DSBR are also coupled to transcription ().
Figure 1. Lesion at the active gene is repaired fast in comparison to the repair at the inactive gene or silent region of the genome. NER, nucleotide excision repair; BER, base excision repair; and DSBR, DNA double-strand break repair, are coupled to transcription, and known as TC-NER (transcription-coupled NER), TC-BER, and TC-DSBR, respectively.Citation4, Citation29-31
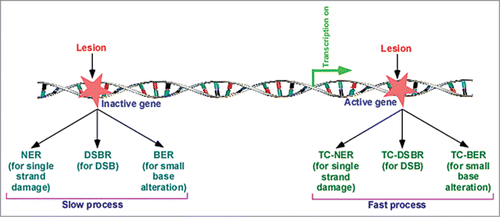
The defects in both TC-NER and GG-NER are associated with cellular pathophysiological states. An altered GG-NER manifests xeroderma pigmentosum (XP) that is characterized by abnormal skin pigmentation by sun, enhanced risk of developing skin cancer and propensity to develop internal tumors.Citation32 However, TCR-deficient CS patients do not have predisposition to cancer, but have other symptoms as mentioned above. Thus, the defects in TC-NER and GG-NER are associated with different pathological states. Such differential pathologies are attributed to different rates of TC-NER and GG-NER. TC-NER rapidly removes lesions from the coding regions. In the absence of TC-NER, GG-NER kicks in with slower repair kinetics. GG-NER repairs damage throughout the genome by the actions of XP proteins.Citation4,5,14, Citation32-34 TC-NER on the other hand is dedicated to repairing damage in the TS of active gene with the help of CS proteins, CSA (Cockayne syndrome group A) and CSB.Citation1,4,29 In addition to its role in TC-NER, CSB has also been implicated to regulate BER, transcription and chromatin structure.Citation35
An early event in TC-NER is the stalling of elongating RNA polymerase II at the DNA lesion.Citation4,5,27,28,36,37 In E. coli, Mfd (mutation frequency decline) or transcription repair-coupling factor (TRCF) links transcriptional halt (or RNA polymerase stalling) to DNA repair.Citation29,38 TRCF displaces stalled RNA polymerase from the lesion in an ATP-dependent manner, and targets repair factors for facilitating the formation of preincision-complex at the site of damage by binding to the repair factor, UvrA.Citation14,29,39 Both Rad26p and CSB have limited homology with TRCF, and thus, are not closely related. However, like TRCF, Rad26p has been implicated in displacing RNA polymerase II from the site of DNA lesion to allow the access of the repair factors to the lesion.Citation37,40 In addition, Rad26p also participates in the regulation of chromatin structure and transcriptional elongation as described below. Like Rad26p, CSB has been suggested to displace RNA polymerase II.Citation37 In addition, CSB regulates chromatin structure and transcription as described below.
Both Rad26p and its human homolog have been shown to play crucial roles in initial DNA damage recognition step in TC-NER.Citation4,5,37, Citation41-43 CSB associates with chromatin following UV-induced DNA damage, and such association is dependent on its ATPase activity or ATP hydrolysis.Citation44 Moreover, CSB preferentially associates with transcribing RNA polymerase II stalled at lesion.Citation45 Likewise, Rad26p travels with elongating RNA polymerase II.Citation11,12 Further, the ternary complex of RNA polymerase II-RNA-DNA serves as a preferred target for 26S proteasome-mediated degradation of the largest subunit of RNA polymerase II.Citation5,46 Although the stalling of RNA polymerase II at the DNA lesion is well-established, there are alternative outcomes of the stalled RNA polymerase II: (1) disassembly of RNA polymerase II via degradation of its largest subunit, Rpb1p and (2) displacement/backtracking of RNA polymerase II without degradation of Rpb1p. In case of Rpb1p degradation, Rpb1p undergoes polyubiquitination and subsequently degraded by the 26S proteasome. Polyubiquitination of Rpb1p is achieved through sequential actions of two E3 ubiquitin ligases, Rsp5p and Elc1p-Cul3p complex.Citation5,47 Rsp5p adds on lysine 63 (K63)-linked ubiquitin chain on Rpb1p. The K-63 polyubiquitin chain is then hydrolysed to monoubiquitin by the ubiquitin protease, Ubp2p.Citation5,47 Subsequently, Elc1p polyubiquitinates the monubiquitinated-Rpb1p, targeting it for the 26S proteasomal degradation. Such degradation of Rpb1p disassembles RNA polymerase II (as Rpb1p maintains the structural and functional integrity of RNA polymerase II) to allow the repair factors to access the lesion.Citation48 The incomplete transcript is discarded, and following repair, fresh transcription cycle starts. In another scenario, DNA repair occurs in the presence of stalled RNA polymerase II that resumes transcription after the repair is complete.Citation4,37,43, Citation49-51 In this case, Rpb1p degradation is inhibited, but rather RNA polymerase II is displaced and/or backtracked to allow repair factors to access the lesion for repair.
Rad26p protects Rpb1p from the 26S proteasomal degradation, while Rad26p-associated Def1p (RNA polymerase II degradation factor 1) facilitates the proteasomal degradation of Rpb1p.Citation5,37,46,52 Further, Rad26p has been implicated to displace RNA polymerase II or alter the DNA-RNA polymerase II interface to expose the lesion site for repair, and continue RNA synthesis following repair.Citation5,37,40,52 This is an energy saving process to repair DNA lesions at the active genes. However, when Rad26p fails to displace RNA polymerase II from the site of lesion, Def1p removes RNA polymerase II from the lesion via Rpb1p ubiquitination, 26S proteasomal degradation, and subsequent disassembly of RNA polymerase II. Thus, Rad26p and Def1p play important roles in providing two distinct fates on RNA polymerase II (i.e., displacement or disassembly). Such distinct outcomes of stalled RNA polymerase II by Rad26p and Def1p may be dictated by the types of DNA lesions, DNA sequences and chromatin modifications structures, as these factors influence the DNA conformation as well as DNA-binding strength of stalled-RNA polymerase II (and hence the actions of Rad26p and Def1p).
Although previous biochemical studies demonstrated global degradation of the Rpb1p subunit of RNA polymerase II following DNA damage,Citation37,50, Citation52-54 it was not clear whether transcriptionally engaged Rpb1p is targeted for degradation in vivo. Our recent results in yeastCitation48 demonstrated that the Rpb1p subunit of the elongating, but not initiating, form of RNA polymerase II is degraded at the active genes in response to DNA damage (). Such degradation of Rpb1p disassembles elongating RNA polymerase II at the lesion in the active coding sequence ().Citation48 However, other RNA polymerase II subunits are not degraded following DNA damage.Citation48 Consistent with our observations in yeast, another studyCitation55 also reported similar results in mammalian cells.
Figure 2. Rad26p is loaded to the site of DNA lesion in a transcription-dependent manner. Rad26p travels with elongating RNA polymerase II, and recognizes lesion.Citation11,12 Rpb1p is ubiquitinated and degraded after encountering the lesion at the active gene, and subsequently, RNA polymerase II is disassembled to favor the access of DNA repair factors to the lesion for repair.Citation11,12,48
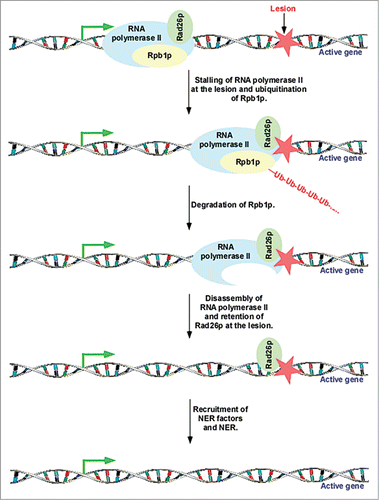
The disassembly of elongating RNA polymerase II following degradation of Rpb1p in response to DNA damage makes the lesion accessible to the repair factors.Citation4,5,27,28,48 Prior to the disassembly of RNA polymerase II, Rad26p is recruited to the lesion with the help of elongating RNA polymerase II ().Citation11 Once Rad26p recognizes the lesion at the active coding sequence, it does not depend on the retention of RNA polymerase II.Citation11 Following association, Rad26p contributes to DNA repair by facilitating the recruitment of the DNA repair factors.Citation56,57 In addition, Rad26p favors DNA repair at active gene via regulation chromatin structure as described below. Likewise, CSB regulates DNA repair at the active gene by interacting with DNA repair factors and altering chromatin structure.Citation35, Citation58-60
Regulation of Transcription by Rad26p
In addition to their functions in DNA repair, Rad26p and CSB also regulate transcription. The CS cells are unable to restore RNA synthesis after UV irradiation.Citation61,62 Further, cells lacking CSB have RNA polymerase II stalled at the UV-induced lesion and cannot continue to transcribe post UV-irradiation. However, after UV exposure, CSB-deficient cells cannot also transcribe many undamaged genes in addition to damaged templates.Citation63 Further, cells that do not have functional CSB have impaired RNA polymerase II recruitment to the promoters of the constitutively active genes such as DHFR and GAPDH.Citation63 These observations are in line with the biochemical findings that CSB resides in a complex with RNA polymerase II and interacts with basal transcription factors.Citation7,64 Consistently, lysates/extracts from the CS cells show low transcriptional activity.Citation8,65 These and other works firmly support the role of CSB in transcription. Likewise, CSB's yeast homolog promotes transcription.Citation9,10 Consistently, we have demonstrated that Rad26p interacts with RNA polymerase II, and associates specifically with the coding regions of the active genes even in the absence of DNA damage ().Citation11 Further, we find that Rad26p enhances the association of elongating RNA polymerase II with the active genes.Citation11,12 In agreement with these observations, Rad26p has been shown to promote transcriptional elongation.Citation9-12 Likewise, another NER factor Rad14p also associates with the active genes independently of DNA damage, and promotes transcription.Citation66
Regulation of Chromatin Structure by Rad26p
Although previous studies implicated Rad26p/CSB in stimulation of transcription, their mechanisms of actions were not clear. We have recently demonstrated that Rad26p regulates chromatin disassembly and/or reassembly that is correlated with transcriptional stimulation.Citation12,67 During transcriptional elongation, nucleosomes are disassembled for the passage of RNA polymerase II, followed by chromatin reassembly in the wake of elongating RNA polymerase II.Citation12,67,68 Intriguingly, we find for the first time the formation of an atypical histone H2A-H2B dimer-enriched chromatin at the active coding sequence in the absence of Rad26p.Citation12,67 Formation of such an atypical chromatin conformation is correlated with impaired RNA polymerase II association and hence transcription.Citation12,67 Importantly, our further analysisCitation12,67 deciphered how this atypical chromatin structure was formed in the absence Rad26p (). We find that Rad26p promotes the eviction of histone H2A-H2B dimer, and hence chromatin disassembly (as nucleosomal disassembly occurs via sequential evictions of histone H2A-H2B dimer and histone H3-H4 tetramer). This enhanced chromatin disassembly favors the passage of elongating RNA polymerase II, and thus transcription. However, nucleosomes are reassembled in the wake of elongating RNA polymerase II, and such chromatin reassembly plays important roles to regulate transcriptional elongation. During chromatin reassembly, histone H3-H4 tetramer first binds to naked DNA, and subsequently, histone H2A-H2B dimers associate to form canonical nucleosome. However, histone H2A-H2B dimer has a high affinity to naked DNA in comparison to histone H3-H4 tetramer.Citation69 Thus, histione H2A-H2B dimer competes with histone H3-H4 tetramer to impair the formation of canonical nucleosome.Citation69 Our data support that Rad26p prevents the reassociation of histone H2A-H2B dimer with naked DNA, and hence allows the association of histone H3-H4 tetramer with naked DNA to form connonical nucleosome in the wake of elongating RNA polymerase II.Citation12,67 However, histone H2A-H2B dimer reassociates with naked DNA in the absence of Rad26p, and hence competes out histone H3-H4 tetramer, leading to the formation of an atypical histone H2A-H2B dimer-enriched chromatin.Citation12,67 Thus, Rad26p regulates chromatin dynamics (and hence transcription) by facilitating the eviction of histone H2A-H2B dimer and preventing the reassociation of histone H2A-H2B dimer with naked DNA in the wake of elongating RNA polymerase II (). These functions of Rad26p in regulation of chromatin dynamics are likely to be mediated via its ATPase activity, as the eviction of histone H2A-H2B dimer is impaired in the ATPase mutant of Rad26p.Citation12 Like Rad26p, a histone chaperone Nap1p has also been recently shown to prevent the association of histone H2A-H2B dimer with DNA in favoring the formation of canonical nucleosome.Citation69 Thus, Rad26p and Nap1p are likely to have synergistic functions in regulation of chromatin dynamics at the active coding sequence and hence transcription. However, it is yet unknown whether CSB performs similar functions in regulation of chromatin dynamics and transcription. Nonetheless, CSB has been shown to possess chromatin remodeling activity.Citation37,59,60,70 Such activity has been implicated in regulation of transcription and DNA repair. Recently, CSB has been also shown to interact with NAP1-like chaperones to facilitate chromatin remodeling for efficient TC-NER.Citation58 Interestingly, CSB recruits the DNA repair factors independently of its chromatin remodeling activity.Citation58 Thus, CSB has separable domains and/or functions in mobilizing nucleosomes and recruiting proteins for efficient DNA repair.
Figure 3. Rad26p facilitates the eviction of histone H2A-H2B dimer and prevents the reassociation of histone H2A-H2B dimer with naked DNA in the wake of elongating RNA polymerse II.Citation11,12,67 “+”and “−” represent stimulation and prevention, respectively. H3/H4, histone H3-H4 tetramer; and H2A/H2B, histone H2A-H2B dimer.
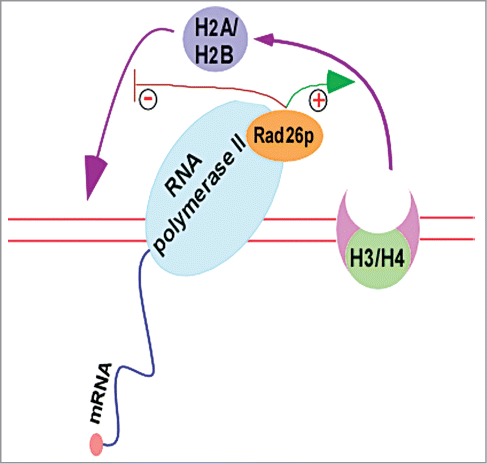
Concluding Remarks
Rad26p facilitates nucleosomal disassembly and canonical nucleosomal reassembly to favor the passage of RNA polymerase II through the coding sequence (and hence transcriptional elongation). Further, it interacts with RNA polymerase II and travels with elongating RNA polymerase II to regulate chromatin dynamics in facilitating transcription. Likewise, CSB may regulate chromatin structure and transcription in humans. In fact, CSB has been shown to promote transcription. However, it is yet unknown whether, like Rad26p, CSB is involved in chromatin disassembly/reassembly at the active genes.
Elongating RNA polymerase II carries Rad26p, and stalls at the lesion. Subsequently, Rad26p is loaded onto the lesion to initiate DNA repair by facilitating the recruitment of the DNA repair factors. Thus, elongating RNA polymerase II facilitates the recognition of DNA lesion by Rad26p. However, Rad26p does not recognize lesion at the inactive gene or silent areas of the genome, as Rad26p requires RNA polymerase II or active transcription machinery for its recruitment. This makes Rad26p a TC-NER-specific factor, and further explains why the recognition of DNA lesion occurs quickly at the active gene in comparison to the inactive gene, leading to faster TC-NER than GG-NER. Likewise, CSB interacts with RNA polymerase II, recognizes lesion at the active gene, and facilitates DNA repair by promoting the recruitment of the DNA repair factors. In addition, ATPase activity of CSB is required for its interaction with chromatin following DNA damage. Moreover, chromatin remodeling activity of CSB has been implicated in TC-NER. However, such chromatin remodeling activity has not yet been clearly analyzed for Rad26p.
Our results demonstrate that Rad26p favors chromatin disassembly and canonical chromatin reassembly in the wake of elongating RNA polymerase II, and hence transcriptional elongation and associated NER. Through such activities, Rad26p may also affect BER as well as DSBR at the active genes. In fact, Rad26p's human homolog has been shown to be involved in BER.Citation35,59,60 Further, mutations in CSB have been found to be sensitive to ionizing radiation.Citation35,59,60 Thus, CSB (as well as Rad26p) may be involved in TC-BER and TC-DSBR. However, these possibilities remain to be further elucidated.
Recently, Rad26p has been shown to be phosphorylated by a checkpoint kinase, Mec1p (yeast homolog of human ATR).Citation71 Such phosphorylation promotes TC-NER.Citation71 However, it is not clear how TC-NER is regulated by Rad26p phosphorylation. Further, it remains to be investigated whether CSB is similarly regulated by ATR to control TC-NER in humans. Moreover, it is yet unknown whether Mec1p-mediated phosphorylation of Rad26p has any effect on the regulation of chromatin structure and transcription.
In summary, a large number of studies implicated Rad26p/CSB in regulation of active genome integrity and expression with mechanistic insights. However, there are many important questions (as mentioned above) regarding the functions and mechanisms of Rad26p/CSB. In addition, it remains largely unknown whether CSB/Rad26p regulates TCR in collaboration with histone covalent modifications. Therefore, further investigations are needed to address these important questions to unveil yet unknown functional and mechanistic insights of Rad26p/CSB.
Disclosure of Potential Conflicts of Interest
No potential conflict of interest was disclosed.
Acknowledgments
We apologize to the authors whose work could not be cited owing to space limitations.
Funding
The work in the Bhaumik laboratory is supported by a National Institute of Health grant (2R15GM088798-02).
References
- Troelstra C, van Gool A, de Wit J, Vermeulen W, Bootsma D, Hoeijmakers JH. ERCC6, a member of a subfamily of putative helicases, is involved in Cockayne's syndrome and preferential repair of active genes. Cell 1992; 71:939-53; PMID:1339317; http://dx.doi.org/10.1016/0092-8674(92)90390-X
- van Gool AJ, Verhage R, Swagemakers SM, van de Putte P, Brouwer J, Troelstra C, Bootsma D, Hoeijmakers JH. RAD26, the functional S. cerevisiae homolog of the Cockayne syndrome B gene ERCC6. EMBO J 1994; 13:5361-9; PMID:7957102
- Jansen LE, den Dulk H, Brouns RM, de Ruijter M, Brandsma JA, Brouwer J. Spt4 modulates Rad26 requirement in transcription-coupled nucleotide excision repair. EMBO J 2000; 19:6498-507; PMID:11101522; http://dx.doi.org/10.1093/emboj/19.23.6498
- Hanawalt PC, Spivak G. Transcription-coupled DNA repair: two decades of progress and surprises. Nat Rev Mol Cell Biol 2008; 9:958-70; PMID:19023283; http://dx.doi.org/10.1038/nrm2549
- Wilson MD, Harreman M, Svejstrup JQ. Ubiquitylation and degradation of elongating RNA polymerase II: the last resort. Biochim Biophys Acta 2013; 1829:151-7; PMID:22960598; http://dx.doi.org/10.1016/j.bbagrm.2012.08.002
- Jaarsma D, van der Pluijm I, van der Horst GT, Hoeijmakers JH. Cockayne syndrome pathogenesis: lessons from mouse models. Mech Ageing Dev 2013; 134:180-95; PMID:23591128; http://dx.doi.org/10.1016/j.mad.2013.04.003
- Selby CP, Sancar A. Cockayne syndrome group B protein enhances elongation by RNA polymerase II. Proc Natl Acad Sci U S A 1997; 94:11205-9; PMID:9326587; http://dx.doi.org/10.1073/pnas.94.21.11205
- Dianov GL, Houle JF, Iyer N, Bohr VA, Friedberg EC. Reduced RNA polymerase II transcription in extracts of cockayne syndrome and xeroderma pigmentosum/Cockayne syndrome cells. Nucleic Acids Res 1997; 25:3636-42; PMID:9278484; http://dx.doi.org/10.1093/nar/25.18.3636
- Lee SK, Yu SL, Prakash L, Prakash S. Requirement for yeast RAD26, a homolog of the human CSB gene, in elongation by RNA polymerase II. Mol Cell Biol 2001; 21:8651-6; PMID:11713297; http://dx.doi.org/10.1128/MCB.21.24.8651-8656.2001
- Lee SK, Yu SL, Prakash L, Prakash S. Yeast RAD26, a homolog of the human CSB gene, functions independently of nucleotide excision repair and base excision repair in promoting transcription through damaged bases. Mol Cell Biol 2002; 22:4383-9; PMID:12024048; http://dx.doi.org/10.1128/MCB.22.12.4383-4389.2002
- Malik S, Chaurasia P, Lahudkar S, Durairaj G, Shukla A, Bhaumik SR. Rad26p, a transcription-coupled repair factor, is recruited to the site of DNA lesion in an elongating RNA polymerase II-dependent manner in vivo. Nucleic Acids Res 2010; 38:1461-77; PMID:20007604; http://dx.doi.org/10.1093/nar/gkp1147
- Malik S, Chaurasia P, Lahudkar S, Uprety B, Bhaumik SR. Rad26p regulates the occupancy of histone H2A-H2B dimer at the active genes in vivo. Nucleic Acids Res 2012; 40:3348-63; PMID:22199252; http://dx.doi.org/10.1093/nar/gkr1244
- Klaunig JE, Kamendulis LM, Hocevar BA. Oxidative stress and oxidative damage in carcinogenesis. Toxicol Pathol 2010; 38:96-109; PMID:20019356; http://dx.doi.org/10.1177/0192623309356453
- Sancar A, Lindsey-Boltz LA, Unsal-Kaçmaz K, Linn S. Molecular mechanisms of mammalian DNA repair and the DNA damage checkpoints. Annu Rev Biochem 2004; 73:39-85; PMID:15189136; http://dx.doi.org/10.1146/annurev.biochem.73.011303.073723
- Feuerhahn S, Egly JM. Tools to study DNA repair: what's in the box? Trends Genet 2008; 24:467-74; PMID:18675488; http://dx.doi.org/10.1016/j.tig.2008.07.003
- Friedberg EC, Walker GC, Siede W. DNA repair and mutagenesis. Washington DC: ASM Press (1995).
- Hoeijmakers JH. Genome maintenance mechanisms for preventing cancer. Nature 2001; 411:366-74; PMID:11357144; http://dx.doi.org/10.1038/35077232
- Seeberg E, Eide L, Bjørås M. The base excision repair pathway. Trends Biochem Sci 1995; 20:391-7; PMID:8533150; http://dx.doi.org/10.1016/S0968-0004(00)89086-6
- Krokan HE, Bjørås M. Base excision repair. Cold Spring Harb Perspect Biol 2013; 5:a012583; PMID:23545420; http://dx.doi.org/10.1101/cshpers-pect.a012583
- Kanaar R, Hoeijmakers JH, van Gent DC. Molecular mechanisms of DNA double strand break repair. Trends Cell Biol 1998; 8:483-9; PMID:9861670; http://dx.doi.org/10.1016/S0962-8924(98)01383-X
- Cromie GA, Connelly JC, Leach DR. Recombination at double-strand breaks and DNA ends: conserved mechanisms from phage to humans. Mol Cell 2001; 8:1163-74; PMID:11779493; http://dx.doi.org/10.1016/S1097-2765(01)00419-1
- Ohnishi T, Mori E, Takahashi A. DNA double-strand breaks: their production, recognition, and repair in eukaryotes. Mutat Res 2009; 669:8-12; PMID:19576233; http://dx.doi.org/10.1016/j.mrfmmm.2009.06.010
- Symington LS, Gautier J. Double-strand break end resection and repair pathway choice. Annu Rev Genet 2011; 45:247-71; PMID:21910633; http://dx.doi.org/10.1146/annurev-genet-110410-132435
- Cahill D, Connor B, Carney JP. Mechanisms of eukaryotic DNA double strand break repair. Front Biosci 2006; 11:1958-76; PMID:16368571; http://dx.doi.org/10.2741/1938
- Bohr VA, Smith CA, Okumoto DS, Hanawalt PC. DNA repair in an active gene: removal of pyrimidine dimers from the DHFR gene of CHO cells is much more efficient than in the genome overall. Cell 1985; 40:359-69; PMID:3838150; http://dx.doi.org/10.1016/0092-8674(85)90150-3
- Mellon I, Spivak G, Hanawalt PC. Selective removal of transcription-blocking DNA damage from the transcribed strand of the mammalian DHFR gene. Cell 1987; 51:241-9; PMID:3664636; http://dx.doi.org/10.1016/0092-8674(87)90151-6
- Christians FC, Hanawalt PC. Inhibition of transcription and strand-specific DNA repair by alpha-amanitin in Chinese hamster ovary cells. Mutat Res 1992; 274:93-101; PMID:1378211; http://dx.doi.org/10.1016/0921-8777(92)90056-9
- Sweder KS, Hanawalt PC. Preferential repair of cyclobutane pyrimidine dimers in the transcribed strand of a gene in yeast chromosomes and plasmids is dependent on transcription. Proc Natl Acad Sci U S A 1992; 89:10696-700; PMID:1438266; http://dx.doi.org/10.1073/pnas.89.22.10696
- Ganesan A, Spivak G, Hanawalt PC. Transcription-coupled DNA repair in prokaryotes. Prog Mol Biol Transl Sci 2012; 110:25-40; PMID:22749141; http://dx.doi.org/10.1016/B978-0-12-387665-2.00002-X
- Banerjee D, Mandal SM, Das A, Hegde ML, Das S, Bhakat KK, Boldogh I, Sarkar PS, Mitra S, Hazra TK. Preferential repair of oxidized base damage in the transcribed genes of mammalian cells. J Biol Chem 2011; 286:6006-16; PMID:21169365; http://dx.doi.org/10.1074/jbc.M110.198796
- Chaurasia P, Sen R, Pandita TK, Bhaumik SR. Preferential repair of DNA double-strand break at the active gene in vivo. J Biol Chem 2012; 287:36414-22; PMID:22910905; http://dx.doi.org/10.1074/jbc.M112.364661
- Hoeijmakers JH. DNA damage, aging, and cancer. N Engl J Med 2009; 361:1475-85; PMID:19812404; http://dx.doi.org/10.1056/NEJMra0804615
- Andressoo JO, Hoeijmakers JH. Transcription-coupled repair and premature ageing. Mutat Res 2005; 577:179-94; PMID:16009385; http://dx.doi.org/10.1016/j.mrfmmm.2005.04.004
- Diderich K, Alanazi M, Hoeijmakers JH. Premature aging and cancer in nucleotide excision repair-disorders. DNA Repair (Amst) 2011; 10:772-80; PMID:21680258; http://dx.doi.org/10.1016/j.dnarep.2011.04.025
- Khobta A, Epe B. Repair of oxidatively generated DNA damage in Cockayne syndrome. Mech Ageing Dev 2013; 134:253-60; PMID:23518175; http://dx.doi.org/10.1016/j.mad.2013.03.001
- Lee KB, Wang D, Lippard SJ, Sharp PA. Transcription-coupled and DNA damage-dependent ubiquitination of RNA polymerase II in vitro. Proc Natl Acad Sci U S A 2002; 99:4239-44; PMID:11904382; http://dx.doi.org/10.1073/pnas.072068399
- van den Boom V, Jaspers NG, Vermeulen W. When machines get stuck–obstructed RNA polymerase II: displacement, degradation or suicide. Bioessays 2002; 24:780-4; PMID:12210513; http://dx.doi.org/10.1002/bies.10150
- Selby CP, Sancar A. Molecular mechanism of transcription-repair coupling. Science 1993; 260:53-8; PMID:8465200; http://dx.doi.org/10.1126/science.8465200
- Selby CP, Sancar A. Human transcription-repair coupling factor CSB/ERCC6 is a DNA-stimulated ATPase but is not a helicase and does not disrupt the ternary transcription complex of stalled RNA polymerase II. J Biol Chem 1997; 272:1885-90; PMID:8999876; http://dx.doi.org/10.1074/jbc.272.3.1885
- Ghosh-Roy S, Das D, Chowdhury D, Smerdon MJ, Chaudhuri RN. Rad26, the transcription-coupled repair factor in yeast, is required for removal of stalled RNA polymerase-II following UV irradiation. PLoS One 2013; 8:e72090; PMID:23991048; http://dx.doi.org/10.1371/journal.pone.0072090
- Lainé JP, Egly JM. Initiation of DNA repair mediated by a stalled RNA polymerase IIO. EMBO J 2006; 25:387-97; PMID:16407975; http://dx.doi.org/10.1038/sj.emboj.7600933
- Svejstrup JQ. The interface between transcription and mechanisms maintaining genome integrity. Trends Biochem Sci 2010; 35:333-8; PMID:20194025; http://dx.doi.org/10.1016/j.tibs.2010.02.001
- Vermeulen W, Fousteri M. Mammalian transcription-coupled excision repair. Cold Spring Harb Perspect Biol 2013; 5:a012625; PMID:23906714; http://dx.doi.org/10.1101/cshperspect.a012625
- Lake RJ, Geyko A, Hemashettar G, Zhao Y, Fan HY. UV-induced association of the CSB remodeling protein with chromatin requires ATP-dependent relief of N-terminal autorepression. Mol Cell 2010; 37:235-46; PMID:20122405; http://dx.doi.org/10.1016/j.molcel.2009.10.027
- Tantin D, Kansal A, Carey M. Recruitment of the putative transcription-repair coupling factor CSB/ERCC6 to RNA polymerase II elongation complexes. Mol Cell Biol 1997; 17:6803-14; PMID:9372911
- Somesh BP, Reid J, Liu WF, Søgaard TM, Erdjument-Bromage H, Tempst P, Svejstrup JQ. Multiple mechanisms confining RNA polymerase II ubiquitylation to polymerases undergoing transcriptional arrest. Cell 2005; 121:913-23; PMID:15960978; http://dx.doi.org/10.1016/j.cell.2005.04.010
- Harreman M, Taschner M, Sigurdsson S, Anindya R, Reid J, Somesh B, Kong SE, Banks CA, Conaway RC, Conaway JW, et al. Distinct ubiquitin ligases act sequentially for RNA polymerase II polyubiquitylation. Proc Natl Acad Sci U S A 2009; 106:20705-10; PMID:19920177; http://dx.doi.org/10.1073/pnas.0907052106
- Malik S, Bagla S, Chaurasia P, Duan Z, Bhaumik SR. Elongating RNA polymerase II is disassembled through specific degradation of its largest but not other subunits in response to DNA damage in vivo. J Biol Chem 2008; 283:6897-905; PMID:18195014; http://dx.doi.org/10.1074/jbc.M707649200
- Daulny A, Tansey WP. Damage control: DNA repair, transcription, and the ubiquitin-proteasome system. DNA Repair (Amst) 2009; 8:444-8; PMID:19272841; http://dx.doi.org/10.1016/j.dnarep.2009.01.017
- Svejstrup JQ. Rescue of arrested RNA polymerase II complexes. J Cell Sci 2003; 116:447-51; PMID:12508106; http://dx.doi.org/10.1242/jcs.00271
- Tornaletti S. DNA repair in mammalian cells: Transcription-coupled DNA repair: directing your effort where it's most needed. Cell Mol Life Sci 2009; 66:1010-20; PMID:19153656; http://dx.doi.org/10.1007/s00018-009-8738-x
- Woudstra EC, Gilbert C, Fellows J, Jansen L, Brouwer J, Erdjument-Bromage H, Tempst P, Svejstrup JQ. A Rad26-Def1 complex coordinates repair and RNA pol II proteolysis in response to DNA damage. Nature 2002; 415:929-33; PMID:11859374; http://dx.doi.org/10.1038/415929a
- Luo Z, Zheng J, Lu Y, Bregman DB. Ultraviolet radiation alters the phosphorylation of RNA polymerase II large subunit and accelerates its proteasome-dependent degradation. Mutat Res 2001; 486:259-74; PMID:11516929; http://dx.doi.org/10.1016/S0921-8777(01)00097-0
- Ratner JN, Balasubramanian B, Corden J, Warren SL, Bregman DB. Ultraviolet radiation-induced ubiquitination and proteasomal degradation of the large subunit of RNA polymerase II. Implications for transcription-coupled DNA repair. J Biol Chem 1998; 273:5184-9; PMID:9478972; http://dx.doi.org/10.1074/jbc.273.9.5184
- Escargueil AE, Poindessous V, Soares DG, Sarasin A, Cook PR, Larsen AK. Influence of irofulven, a transcription-coupled repair-specific antitumor agent, on RNA polymerase activity, stability and dynamics in living mammalian cells. J Cell Sci 2008; 121:1275-83; PMID:18388315; http://dx.doi.org/10.1242/jcs.023259
- Ho Y, Gruhler A, Heilbut A, Bader GD, Moore L, Adams SL, Millar A, Taylor P, Bennett K, Boutilier K, et al. Systematic identification of protein complexes in Saccharomyces cerevisiae by mass spectrometry. Nature 2002; 415:180-3; PMID:11805837; http://dx.doi.org/10.1038/415180a
- Sung P, Prakash L, Matson SW, Prakash S. RAD3 protein of Saccharomyces cerevisiae is a DNA helicase. Proc Natl Acad Sci U S A 1987; 84:8951-5; PMID:2827162; http://dx.doi.org/10.1073/pnas.84.24.8951
- Cho I, Tsai PF, Lake RJ, Basheer A, Fan HY. ATP-dependent chromatin remodeling by Cockayne syndrome protein B and NAP1-like histone chaperones is required for efficient transcription-coupled DNA repair. PLoS Genet 2013; 9:e1003407; PMID:23637612; http://dx.doi.org/10.1371/journal.pgen.1003407
- Aamann MD, Muftuoglu M, Bohr VA, Stevnsner T. Multiple interaction partners for Cockayne syndrome proteins: implications for genome and transcriptome maintenance. Mech Ageing Dev 2013; 134:212-24; PMID:23583689; http://dx.doi.org/10.1016/j.mad.2013.03.009
- Vélez-Cruz R, Egly JM. Cockayne syndrome group B (CSB) protein: at the crossroads of transcriptional networks. Mech Ageing Dev 2013; 134:234-42; PMID:23562425; http://dx.doi.org/10.1016/j.mad.2013.03.004
- Lehmann AR. Three complementation groups in Cockayne syndrome. Mutat Res 1982; 106:347-56; PMID:6185841; http://dx.doi.org/10.1016/0027-5107(82)90115-4
- Mayne LV, Lehmann AR. Failure of RNA synthesis to recover after UV irradiation: an early defect in cells from individuals with Cockayne's syndrome and xeroderma pigmentosum. Cancer Res 1982; 42:1473-8; PMID:6174225
- Proietti-De-Santis L, Drané P, Egly JM. Cockayne syndrome B protein regulates the transcriptional program after UV irradiation. EMBO J 2006; 25:1915-23; PMID:16601682; http://dx.doi.org/10.1038/sj.emboj.7601071
- van Gool AJ, Citterio E, Rademakers S, van Os R, Vermeulen W, Constantinou A, Egly JM, Bootsma D, Hoeijmakers JH. The Cockayne syndrome B protein, involved in transcription-coupled DNA repair, resides in an RNA polymerase II-containing complex. EMBO J 1997; 16:5955-65; PMID:9312053; http://dx.doi.org/10.1093/emboj/16.19.5955
- Balajee AS, May A, Dianov GL, Friedberg EC, Bohr VA. Reduced RNA polymerase II transcription in intact and permeabilized Cockayne syndrome group B cells. Proc Natl Acad Sci U S A 1997; 94:4306-11; PMID:9113985; http://dx.doi.org/10.1073/pnas.94.9.4306
- Chaurasia P, Sen R, Bhaumik SR. Functional analysis of Rad14p, a DNA damage recognition factor in nucleotide excision repair, in regulation of transcription in vivo. J Biol Chem 2013; 288:793-806; PMID:23188830; http://dx.doi.org/10.1074/jbc.M112.413716
- Malik S, Bhaumik SR. Rad26p, a transcription-coupled repair factor, promotes the eviction and prevents the reassociation of histone H2A-H2B dimer during transcriptional elongation in vivo. Biochemistry 2012; 51:5873-5; PMID:22794311; http://dx.doi.org/10.1021/bi3005768
- Durairaj G, Chaurasia P, Lahudkar S, Malik S, Shukla A, Bhaumik SR. Regulation of chromatin assembly/disassembly by Rtt109p, a histone H3 Lys56-specific acetyltransferase, in vivo. J Biol Chem 2010; 285:30472-9; PMID:20668333; http://dx.doi.org/10.1074/jbc.M110.113225
- Andrews AJ, Chen X, Zevin A, Stargell LA, Luger K. The histone chaperone Nap1 promotes nucleosome assembly by eliminating nonnucleosomal histone DNA interactions. Mol Cell 2010; 37:834-42; PMID:20347425; http://dx.doi.org/10.1016/j.molcel.2010.01.037
- Citterio E, Van Den Boom V, Schnitzler G, Kanaar R, Bonte E, Kingston RE, Hoeijmakers JH, Vermeulen W. ATP-dependent chromatin remodeling by the Cockayne syndrome B DNA repair-transcription-coupling factor. Mol Cell Biol 2000; 20:7643-53; PMID:11003660; http://dx.doi.org/10.1128/MCB.20.20.7643-7653.2000
- Taschner M, Harreman M, Teng Y, Gill H, Anindya R, Maslen SL, Skehel JM, Waters R, Svejstrup JQ. A role for checkpoint kinase-dependent Rad26 phosphorylation in transcription-coupled DNA repair in Saccharomyces cerevisiae. Mol Cell Biol 2010; 30:436-46; PMID:19901073; http://dx.doi.org/10.1128/MCB.00822-09