Abstract
Members of the interferon-regulatory factor (IRF) proteins family were originally identified as transcriptional regulators of the Type I interferon system. Thanks to consistent advances made in our understanding of the immunobiology of innate receptors, it is now clear that several IRFs are critical for the elicitation of innate pattern recognition receptors, and—as a consequence—for adaptive immunity. In addition, IRFs have attracted great attentions as they modulate cellular responses that are involved in tumorigenesis. The regulation of oncogenesis by IRFs has important implications for understanding the host susceptibility to several Types of cancers, their progression, as well as the potential for therapeutic interventions.
Introduction
The discovery of interferon-regulatory factors (IRFs) dates back to 1988, when a cDNA clone encoding a mouse protein that binds to a virus-inducible enhancer element of the interferon (IFN) β-coding (IFNB) gene was identified.Citation1 In that period, no significant homology between this gene and other known proteins was recognized and hence the gene was named IFN-regulatory factor 1 (IRF1). The subsequent identification of a cDNA clone that cross-hybridizes to IRF1 cDNA, named IRF2, marked the official recognition of the IRF family.Citation2 Since then, the IRF family has been shown to include nine members, IRF1–9 (). Although it has become customary to refer to these proteins as to IRFs, some of them were originally identified with different acronyms, such as PIP/LSIRF/ICSAT for IRF4, ICSBP for IRF8, and IFN-stimulated gene factor 3γ (ISGF3γ) for IRF9 (refs. Citation3–Citation8). Virus-encoded homologs of IRFs, named viral IRFs (vIRFs), have also been characterized.Citation9,Citation10
Figure 1. Schematic illustration of human and viral IRF family members. Nine human IRF family members (IRF1 to IRF9) (upper) and three vIRFs (vIRF1, vIRF2, and vIRF3) (lower), all encoded by Kaposi sarcoma-associated herpesvirus (KSHV) are shown. Length (in amino acid) is reported. IRF family members possess an amino N-terminal DNA-binding domain (DBD) that is characterized by a series of five relatively well conserved tryptophan-rich repeats. All IRF family members, except for IRF1 and IRF2, contain an IRF association domain (IAD1). IRF1 and IRF2 share another IRF association domain (IAD2), which is structurally distinct from IAD1. IRF3 and IRF7 have multiple sites that—upon viral infection—are substrates for phosphorylation by the serine/threonine kinases TANK-binding kinase 1 (TBK1) and IκB kinase ε (IKKε). Phosphorylation at these sites is required for the nuclear translocation of IRF3 and IRF7. KSHV encodes a cluster of three viral vIRFs, all of which show homology in their N-terminal regions to the DBD of IRFs but lack several tryptophan residues. For this reason, vIRFs are believed to be unable to directly bind DNA. vIRF1 contains an IAD domain which enables vIRF1 to associate with other IRFs, such as IRF1. vIRF3 has an IAD-like domain which shows 32% identity with the IAD of IFR4, although the exact function of this domain remains elusive.
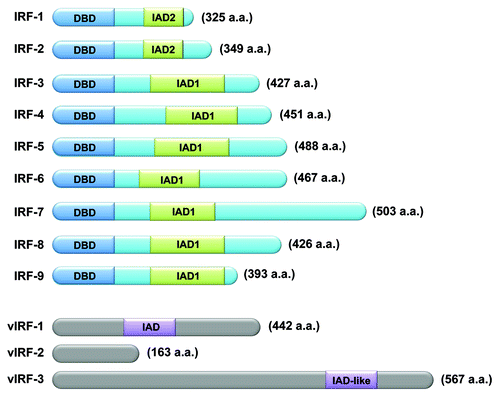
All IRF family members possess an N-terminal DNA-binding domain (DBD) that is characterized by a series of five relatively well-conserved tryptophan-rich repeatsCitation11,Citation12 (). The DBD forms a helix-turn-helix structure and recognizes a DNA sequence known as IFN-stimulated response element (ISRE)Citation13 which is characterized by the consensus sequence, 5′-AANNGAAA-3′.Citation14 The C-terminal region of IRFs is less well conserved and supposedly mediates the interactions of a specific IRF with other family members, other transcriptional factors, or cofactors, so as to confer specific activities upon each IRF. Even so, two Types of association modules have been identified within the C-terminal region of some IRFs:Citation15 IRF-associated domain 1 (IAD1),Citation16 which is conserved in all IRFs except IRF1 and IRF2 and is structurally similar to the Mad-homology 2 (MH2) domain of SMAD transcription factors; and IAD2,Citation12 which is shared by IRF1 and IRF2 only (). The nature of the protein-to-protein interactions dictated by these domains likely determines whether the resulting complex functions as a transcriptional activator or repressor, and define the nucleotide sequences adjacent to the core IRF-binding motif to which the transcriptional complex binds.Citation12,Citation16
There has been much progress in our understanding of the close connection between innate and adaptive immunity. Several IRFs play an essential role in this setting, by regulating the development and functions of various Types of immune cells (reviewed in refs. Citation12, Citation16–Citation21). In addition, accumulating evidence indicates that IRFs play a critical function in the regulation of cellular responses linked to oncogenesis, thereby connecting the mechanisms governing immunity and cancer. In this review, we discuss current knowledge on how each IRF participates in the regulation of oncogenesis (see for general overview). Although not extensively discussed in this review, all IRFs may play a broader and less direct role in the regulation of oncogenesis as they critically control the development and/or function of cells involved in antitumor immune responses.Citation11,Citation12,Citation22,Citation23 Such and other aspects of the IRF biology have been extensively discussed in several excellent and comprehensive reviews.Citation11,Citation12,Citation16-Citation21
Table 1. A summary of IRF family members and their functions in oncogenesis
IRF1
As IRF1 and IRF2 were the first IRF family members identified, studies on the regulation of oncogenesis by IRFs were initiated on these factors. The notion that IRFs participate in the regulation of oncogenesis originally came from studies demonstrating that the phenoType of NIH3T3 cells that underwent transformation upon IRF2 overexpression was reverted by the overexpression of IRF1 (ref. Citation24). Subsequent studies using Irf1−/− mouse embryonic fibroblasts (MEFs) revealed that these cells do not undergo a normal DNA damage-induced cell cycle arrest.Citation25 Together with the oncosuppressor protein p53, IRF1 transcriptionally activates the gene encoding the cyclin-dependent kinase (CDK) inhibitor p21WAF1/CIP1 (official name CDKNA1) (ref. Citation25). Upon DNA damage, IRF1 protein levels normally increase as a result of increased mRNA expression and protein stability, so as to act on the CDKNA1 promoter region containing the IRF1- and p53-binding sites.Citation25,Citation26 In the context of DNA repair, IRF1 positively regulates the expression of the DNA repair protein BRIP1, also known as Fanconi anemia gene J (FANCJ), and cells lacking IRF1 expression are hypersensitive to the DNA crosslinking agent melphalan, a characteristic phenoType of FANCJ-deficient cells.Citation27
Apoptosis is a mechanism by which pre-cancerous cells are actively eliminated from the host. In the context of an activated oncogene, such as c-Ha-RAS, wild-Type (WT) MEFs undergo apoptosis rather than cell cycle arrest in response to anticancer drugs or ionizing radiation. This sign of tumor suppression was found to depend on both IRF1 and p53 (ref. Citation28). While DNA damage-induced apoptosis relies on IRF1 but not on p53 in activated mature T lymphocytes,Citation29 it depends on p53 but not IRF1 in thymocytes. Thus, depending on the cell Type and differentiation stage, IRF1 and p53 regulate DNA damage-induced apoptosis cooperatively or independently. Interestingly, a transcriptional activator of both IRF1 and p53, GAAP-1, has been shown to mediate pro-apoptotic effects.Citation30 In addition, IRF1 is important for apoptosis as activated (or enhanced) by other stimuli, such as IFNγ.Citation31-Citation33 The IRF1target gene(s) that are responsible for apoptotic responses have not yet been firmly identified, but may include genes coding for caspases and tumor necrosis factor-related apoptosis-inducing ligand (TRAIL).Citation11,Citation23 The lysyl oxidase-encoding (LOX) gene is also a transcriptional target of IRF1, and is involved in its tumor suppressive activity.Citation34 LOX plays a critical role in the biogenesis of connective tissue and de facto corresponds to the independently discovered Ras recision gene (Rrg).Citation35 Rrg has been implicated in the reversion of Ras-transformed NIH 3T3 cells, owing to its ability of preventing the activation of NF-κB.Citation35
Consistent with these observations, an oncogenic transformation assay in which the introduction of a single oncogene, activated c-Ha-Ras, into Irf1−/− MEFs was sufficient to transform these cells.Citation28 This does not apply to WT cells, whose transformation require the activation of at least two oncogenes, indicating a tumor suppressor-like activity for IRF1 Moreover, conditions in which activated Ras paradoxically inhibits the growth of myeloid cells were found to involve IRF1 and the induction of p21WAF1/CIP1 (ref. Citation36). The ectopic expression of IRF1 suppresses the malignant properties of cancer cell lines and oncogene-transformed cell lines in vitro and in vivo.Citation37 Although the loss of IRF1 alone rarely promotes tumor development in mice, IRF1 deficiency dramatically exacerbates the incidence of tumors caused by the expression of a c-Ha-Ras transgene or by Tp53 deletion.Citation38 Thus, IRF1 operates as a tumor suppressor whose loss, in combination with other genetic alterations, significantly increases the tumor incidence in vivo.
Mechanisms other than mutations can lead to the loss of IRF1 function in cancer. For instance, an elevated level of SUMOylated IRF1 in tumor cells interferes with IRF1-mediated apoptosis.Citation39 Splicing aberrations of IRF1 mRNA cause the loss of functional IRF1 in myelodysplastic syndrome (MDS) and leukemia.Citation40,Citation41 Also, a putative ribosome assembly factor that is often overexpressed in leukemic cells, nucleophosmin, binds to IRF1 and inhibits its function.Citation42 Other IRF1 repressors, Y-box protein (YB-1) and tripartite motif-containing 28 (TRIM-28), both of which are overexpressed in various cancer Types, have been identified.Citation43
A number of clinical studies have shown a correlation between the loss of IRF1 expression or function and human malignancies. IRF1 maps to chromosome 5q31.1, a genomic region frequently affected by cytogenetic abnormalities in MDS and leukemia, and IRF1 is consistently deleted at one or both alleles in patients with aberrations of 5q31 (ref. Citation44). The heterozygous loss of IRF1 has also been reported in esophageal and gastric cancer patients,Citation45,Citation46 and in one out of four cases of gastric cancers examined the deletion of one allele of IRF1 is accompanied by an inactivating point mutation in the other allele.Citation47 It has been reported that approximately 11% of sporadic breast cancer patients exhibit the loss of chromosome 5q12–31 (ref. Citation48) and approximately 30% of neoplastic breast tissues lack IRF1 (ref. Citation49). In woman with breast cancer, the relatively frequent allelic loss of IRF1 is associated with an increased risk of recurrence and death, underscoring the relevance of the tumor suppressive role of IRF1 in this clinical setting.Citation50 The mechanisms by which IRF1 contributes to tumor suppression has not yet been fully clarified.
IRF2
IRF2 is well known for its ability to exert pro-oncogenic activities. As mentioned above, the overexpression of IRF2 in NIH3T3 cells causes oncogenic transformation.Citation24 Consistently, elevated IRF2 expression levels have been observed in pancreatic cancer patients and are associated with an increased proliferative potential of pancreatic cancer cells.Citation51 A genetic screen of a retroviral library identified IRF2 as an inhibitor of activated N-RAS-induced growth suppression in leukemic cells.Citation52 The pro-oncogenic functions of IRF2 appear to be mediated—at least in part—by its ability to transcriptionally interfere with IRF1 and/or other IRF family members that bind to the same ISRE elements.Citation53 In addition, IRF2 can also regulate transcription under specific conditions,Citation54 for example by stimulating the expression of genes involved in oncogenesis such as histone H4 (refs. Citation55, Citation56). It has also been reported that IRF2 is post-translationally regulated in a cell growth-dependent manner. In particular, it appeas that acetylated IRF2 preferentially binds to the H4 promoter in proliferating cells only.Citation57
Interestingly, IRF2 has been shown to interact with murine double minute 2 (MDM2), the enzyme that catalyzes the ubiquitination of p53, targeting it to proteasomal degradation.Citation58 In this context, an oncosuppressive role of IRF2 has recently been reported. Indeed, IRF2 has been found to be inactivated specifically in hepatitis B virus (HBV)-related hepatocellular carcinoma patients. The lack of IRF2 may impair p53 function, since IRF2 silencing decreased p53 protein levels and p53 target gene expression.Citation59 Thus, IRF2 may be bifunctional in the regulation of oncogenesis and its function may be highly cell Type- and context-dependent.
IRF3
IRF3 is best known for its critical role as a transcription factor promoting Type I IFN expression,Citation18,Citation60 and its contribution to oncogenesis has not yet been extensively studied. IRF3 expression has been found to be significantly higher in the neoplastic lesions of non-small cell lung carcinoma patients surviving Stage I disease than in the tumors of patients who succumbed from cancer.Citation61 In human primary lung cancer, two novel protein variants of IRF3 have been identified.Citation62 One of these variants carries the A208(GCC) → D208(GAC) substitution, which was also found in squamous cell carcinoma cases, and exhibits reduced nuclear translocation in response to activation by IκB kinase ε (IKKε). It remains to be determined whether this mutant contributes to the etiology of primary lung cancer.Citation62
Apoptosis in response to viral infection may be mediated by IRF3 activation, as the expression of a constitutively active mutant of IRF3 triggers apoptosis, while dominant negative mutants of IRF3 strongly inhibit Sendai virus- and Newcastle disease virus (NDV)-induced apoptosis.Citation63,Citation64 Interestingly, IRF3-mediated apoptosis appears to be independent of p53 as well as Type I IFN,Citation64 but to involves the gene encoding TRAIL, which is transcriptionally activated by the ectopic expression of IRF3 (ref. Citation65). However, the fact that vesicular stomatitis virus (VSV) infected Irf3−/− MEFs undergo apoptosis as efficiently as WT cells indicates that IRF3 does not mediate apoptosis as induced by all viruses.Citation66
IRF3 also participates in a putative apoptotic instance that is promoted by bacterial infection and mediated by Toll-like receptor (TLR) activation. Some bacteria induce indeed the apoptotic demise of macrophages by producing virulence factors that inhibit cell survival pathways such as those mediated by p38 and NF-κB, and this apoptotic response appears to require IRF3 along with protein kinase, RNA-activated (PKR) and TLR4 (ref. Citation67). Finally, IRF3 is suspected to play a role in DNA damage-induced apoptosis as it is phosphorylated and translocates from the cytoplasm to the nucleus in response to DNA damaging agents.Citation64,Citation68 Of interest, DNA-dependent protein kinase (DNA-PK) is capable of phosphorylating human IRF3 at Thr135, a residue that is distinct from the site phosphorylated by the serine/threonine kinase TANK-binding kinase 1 (TBK1).Citation69 Moreover, IRF3 has a potential to inhibit the growth of cancer cell lines in vitro and in vivo.Citation70,Citation71 Taken together, these studies suggest that IRF3 may also function as a tumor suppressor protein.
IRF4
IRF4 is a key regulator of several steps of lymphoid, myeloid and dendritic cell (DC) differentiation. Particular attention has recently been dedicated to the role of IRF4 in B-cell differentiation and function.Citation72 The functions of IRF4 in oncogenesis and tumor progression, in particular in relationship to hematopoietic malignancies, has been extensively studied. The expression of IRF4 mRNA is induced upon human T cell leukemia virus-1 (HTLV-1) infection.Citation8 Moreover, in Jurkat T cells, the overexpression of the HTLV-1 oncoprotein Tax induces the upregulation of IRF4, while constitutive expression levels limit the expression of the G2-M checkpoint gene cyclin B1 and several DNA repair genes. These transcriptional changes are strikingly similar to those that occur in HTLV-1-infected T cells,Citation73,Citation74 suggesting a possible involvement of IRF4 in HTLV-1-induced leukemogenesis. Translocations involving IRF4 have been detected in 12 out of 169 cases of peripheral T-cell lymphoma.Citation75 In some patients with multiple myeloma as well as in cell lines derived from this tumor, the chromosomal translocation t(p25;q32) juxtaposes the immunoglobulin heavy-chain locus to IRF4/MUM1 (multiple myeloma 1), resulting in the overexpression of IRF4 (ref. Citation76). Furthermore, IRF4 mRNA expression levels are a prognostic marker for poor survival in patients with multiple myeloma.Citation77 Recently, IRF4 has emerged as a master regulator of an aberrant and malignancy-specific gene expression program in this disease.Citation78 In fact, IRF4 is required for the survival of multiple myeloma cell lines. This is because IRF4 transactivates the MYC gene while MYC activates IRF4, thereby establishing a positive feedforward loop. Although IRF4 is not genetically altered in most myelomas, this positive feedback loop is likely to be triggered by the initial oncogenic activation of MYC, which is often amplified and inserted at ectopic genomic locations in this disease. The overexpression of IRF4 alone in lymphocytes, however, is not sufficient for the development of T-cell leukemia and multiple myeloma in transgenic mice,Citation79 suggesting that additional factors are required for the development of these neoplasms. Since interfering with IRF4 expression is lethal for multiple myeloma cells, it has been argued that IRF4 may constitute an “Achilles’ heel” of multiple myeloma that may be exploited therapeutically.Citation72
In the activated B cell-like (ABC) subType of diffuse large B cell lymphoma (DLBCL), oncogenic mutations activate the B-cell receptor (BCR) and myeloid differentiation primary response gene 88 (MYD88) pathways, engaging NFκB and IFNβ signaling. Lenalidomide, a drug showing clinical activity against DLBCL, antagonizes a central regulatory hub in ABC DLBCL governed by IRF4 and SPIB, which together suppress IFNβ while augmenting NFκB activity. In this setting, the oncogenic signaling of BCR to NFκB induces IRF4 expression. Inhibition of the BCR-IRF4 signaling axis by the Bruton’s tyrosine kinase (BTK) inhibitor ibrutinib coupled to lenalidomide kills ABC DLBCL cells, suggesting that this pathway may constitute an attractive therapeutic target.Citation80
Interestingly, a tumor suppressive role of IRF4 has also been reported. In particular, c-Myc-induced leukemia appears to be greatly accelerated in IRF4 heterozygous mice. Evidence has also been provided that IRF4 functions as a classical tumor suppressor gene to inhibit c-Myc-induced leukemogenesis. Thus, IRF4 deficiency appears to accelerate the loss of p27KIP1 and reconstitution of IRF4 expression in leukemic cells restores p27KIP1 expression and inhibits their proliferation in vivo.Citation81
IRF5
IRF5 has emerged as another IRF family member that possesses tumor suppressor activity. It has been reported that IRF5 expression is reduced in human leukemia and human ductal carcinoma and that this correlates with disease stage.Citation82,Citation83 In addition, a single point missense mutation (G202C), leading to the IRF5 variant called IRF-5P68, was identified in peripheral blood mononuclear cells from patients with adult T-cell leukemia/lymphoma (ATL) and chronic lymphocytic leukemia (CLL).Citation84 IRF-5P68 acts as a dominant negative protein and hence interferes with IRF5 activity. Taken together, these reports suggest that IRF5 inactivation relates to the development of human cancer. In nude mice, activated c-Ha-Ras-expressing Irf5−/− MEFs fail to efficiently die of apoptosis in response to DNA damage but undergo neoplastic transformation.Citation66 Irf5−/− MEFs are resistant to VSV-induced apoptosis, resulting in enhanced viral propagation, although they produce normal levels of Type I IFNs and interleukin-6 (IL-6) in response to infection.Citation66 The IRF5 mRNA is induced upon viral infection through Type I IFN signaling and upon DNA damage by p53 (refs. Citation66, Citation85). Because several p53 targets, such as the genes coding for PUMA and NOXA, are induced even in Irf5−/− MEFs, it seems that IRF5 may operate in an apoptotic pathway that is distinct from that controlled by p53 (ref. Citation66). Indeed, overexpression of IRF5 inhibits in vitro and in vivo B-cell lymphoma growth in the absence of WT p53 (ref. Citation82). Furthermore, the ectopic expression of IRF5 sensitizes p53-proficient and p53-deficient colon cancer cells to DNA damage-induced apoptosis.Citation86 IRF5 has been involved in FAS/CD95-induced apoptosis, which typically occurs in a p53-independent manner.Citation87 Irf5−/− mice are resistant to liver apoptosis as well as to death induced by the administration of a FAS-activating monoclonal antibody. IRF5 is also required for FAS-induced apoptosis in DCs activated by hypomethylated CpG oligonucleotides, but not in thymocytes and MEFs. Thus, IRF5 is required for the death receptor-induced apoptosis in a cell Type-dependent manner. Interestingly, IRF5 expression is suppressed in human leukemia cells, pointing to a possible involvement of IRF5 gene inactivation in human leukemogenesis.Citation82 Further studies are required to clarify the transcriptional pathway(s) by which IRF5 stimulates apoptosis.
IRF6
Unlike all other IRFs, IRF6 is not involved in innate immunity, but is rather essential for the normal development and differentiation of the epidermis. Mutations of IRF6 have been found in patients affected by the Van der Woude and popliteal pterygium syndromes, which are characterized by cleft palate and lip pits, skin folds, syndactyly and oral adhesions.Citation88 In mice, loss of IRF6 results in severe defects in limb and skin development with compromised differentiation of keratinocytes in the interfollicular epidermis.Citation89,Citation90 It has been shown that p63 (a member of the p53 tumor suppressor family) binds to an IRF6 enhancer to stimulate gene expression, while IRF6 negatively regulates p63 levels, hence limiting growth of keratinocytes.Citation91 Furthermoe, IRF6 has been shown to constitute a primary target of Notch signaling in keratinocytes and to exert pro-differentiation and tumor suppression functions in this setting.Citation92
IRF6 may also act as a tumor suppressor via its interaction with the mammary serine proteinase inhibitor (maspin), a known tumor suppressor gene.Citation93 Similar to maspin, IRF6 expression inversely correlates with breast cancer invasiveness. IRF6 is unphosphorylated in quiescent mammary epithelial cells, but during cell division IRF6 becomes phosphorylated and undergoes proteasome-dependent degradation.Citation94 Ectopic expression of IRF6 results in cell cycle arrest, a process that is exacerbated by maspin.Citation94
IRF7
IRF7 is best known as a master regulator of Type I IFN-dependent innate immune responses.Citation95 Most studies on the role of IRF7 in the regulation of oncogenesis have been perfomed in the context of Type I IFN responses. In a mouse model of spontaneous bone metastasis, a substantial number of genes suppressed in metastatic lesions are regulated by IRF7, and restoration of IRF7 in tumor cells has been shown to limit metastatic dissemination. Furthermore, in > 800 breast cancer patients, elevated expression of IRF7-regulated genes in the primary lesion has been associated with prolonged metastasis-free survival, suggesting that Type I IFN-mediated immunity is critical for preventing metastasis.Citation96 Lenalidomide has been shown to kill ABC DLBCL cells by augmenting Type I IFN production. This drug apparently downregulates IRF4 and SPIB, transcription factors that together prevent Type I IFN production by repressing IRF7 and amplify pro-survival NF-κB signaling.Citation80 Furthermore, disruption of the IFN pathway in lung cancer cell lines and primary tumor tissues appears to be often caused by the epigenetic silencing of the critical IFN-responsive transcription factors IRF7 and/or IRF5.Citation97
IRF8
IRF8 is a key transcription factor for myeloid cell differentiation, and its expression is frequently lost in hematopoietic cells obtained from myeloid leukemia patients. Accumulating evidence indicates an antagonizing relationship between IRF8 and myeloid leukemia, in particular chronic myelogenous leukemia (CML). It has been shown that Irf8−/− mice develop a CML-like syndrome.Citation98 In CML and acute myelogenous leukemia patients, IRF8 transcripts are absent and a number of the expression of IRF8 target genes, such as BCL2 and PML, is limited.Citation99,Citation100 Conversely, the ectopic expression of IRF8 is able to override the mitogenic activity of BCR-ABL (the etiological determinant of a vast majority of human CML cases), in vitro, by activating several proteins that interfere with the c-Myc pathway (a downstream target of BCR-ABL)Citation101 and to ameliorate BCR-ABL-mediated murine myeloid leukemia in vivo.Citation102 In addition, the administration of IFNα to CML patients induces IRF8 expression in vivo,Citation103 and—in this setting—IRF8 expression levels positively correlated with pre-treatment risk factors as well as with cytogenetic responses to IFNα.Citation104 Thus, IRF8 expression may be a major factor against the development of human CML. Interestingly, IRF4 transcript levels are also significantly reduced in CML patients,Citation105,Citation106 suggesting that IRF4 may have an activity similar to that of IRF8 in myeloid cell development and CML pathogenesis, as it is the case for DC and B-cell development.
It is likely that IRF8 exerts its anti-leukemic activity not only by direct controling cell growth, differentiation and apoptosis but also by modulating anti-tumor immunity. Since human CML cells are susceptible to T cell-mediated immune responses, the ability of IRF8 to support the differentiation and function of professional antigen-presenting cells (APCs) such as macrophages (MΦs), DCs and B cells may be important for the elimination of CML cells by the immune system. In mice, the expression of IRF8 in BCR-ABL-transformed pro-B cells causes a CD8+ cytotoxic T-cell response that prevents the establishment of leukemia in vivo.Citation107 Given the efficacy of IFNα-based therapy for human CML, it is also interesting to note that IRF8 is required for the development of plasmacytoid DCs (pDCs), which produce high levels of Type I IFNs, and that IRF8 is a transcriptional activator of Type I IFN genes.Citation108 In addition, acid ceramidase (A-CDase) has been identified as a general transcription target of IRF8. IRF8 expression is regulated by promoter DNA methylation in myeloid leukemia cells and restoration of IRF8 expression has been shown to repress A-CDase expression, resulting in C16-ceramide accumulation and increased sensitivity of CML cells to FASL-induced apoptosis.Citation109
IRF8 has been reported to exert anti-tumor activity even in non-hematopoietic tumors. IFNγ-induced IRF8 sensitizes human colon carcinoma cells to FAS-mediated apoptosis,Citation110 and IRF8 represses PTPN13, a gene that encodes a ubiquitously expressed protein-tyrosine phosphatase, FAS-associated phosphatase 1 (ref. Citation111). IRF8 expression is repressed by DNA methylation in human metastatic colon carcinoma cell lines and lung metastasis of murine mammary carcinoma, in vivo.Citation112 IRF8 localizes to 16q24, a chromosomal region that is frequently deleted in several Type of solid tumor. In 78% of primary nasopharyngeal carcinoma and in 36–71% of other carcinoma samples, IRF8 is associated with transcriptional silencing and promoter methylation.Citation113
IRF9
IRF9 is best known for its integral role in Type I IFN-mediated cellular responses, to which it contributed by forming a trimeric complex, termed ISGF3, with STAT1 and STAT2 (refs. Citation5, Citation12, Citation114). A critical link between Type I IFNs and the p53 pathway, which is required for virus-induced apoptosis, has been established by the finding that Type I IFNs transcriptionally activate TP53 via ISGF3 binding to ISREs that are located in TP53 promoter and first intron.Citation115 As a component of ISGF3, IRF9 stimulates the p53 pathway when cells are exposed to endogenously induced or exogenously administered Type I IFNs. Accordingly, Irf9−/− MEFs fail to upregulate p53 upon IFNβ stimulation,Citation115 a setting in which IFNβ normally suppresses oncogene-induced malignant cell transformation and enhance DNA damage-induced apoptosis. The link between Type I IFNs and p53 also exemplifies the relationship between tumor suppression and antiviral immunity. On another note, it has been reported that IRF9 is directly activated by c-Myc, and a cell line lacking IRF9 expression is more susceptible to cytotoxic chemotherapeutic drugs,Citation116 suggesting an hitherto elusive role of IRF9 in cell cycle regulation. Additional research is required to get further insights into these functions of IRF9.
Viruses and IRFs in the Regulation of Oncogenesis
Many viruses have evolved mechanisms to counteract the activity of the host immune system. Given the important role of IRFs in the regulation of immune responses as well as their prominent tumor suppressive functions, it is not surprising that these transcription factors and their activation pathways are targeted by many viral factors.
The vaccinia virus-encoded proteins N1L and K7 antagonize TLR signaling at the level of IκB kinases (IKKs) plus TBK1 and DEAD box protein 3 (Ddx3), respectively.Citation117,Citation118 Hepatitis C virus (HCV) encodes the nonstructural proteins 3 and 4A (NS3/4A), which function as protease and cleave IFNβ promoter stimulator-1 (IPS-1) and TIR domain-containing adaptor-inducing IFNβ (TRIF), thereby inhibiting the activation of IRF3 and/or IRF7 during HCV infection.Citation119,Citation120 The rotavirus nonstructural protein 1 (NSP1) mediates the degradation of IRF3, IRF5 and IRF7 (refs. Citation121, Citation122). Kaposi’s sarcoma-associated herpesvirus (KSHV)/human herpes virus 8 (HHV8) encodes replication and transcription activator (RTA), an ubiquitin E3 ligase that promotes IRF7 ubiquitination and proteasome-mediated degradation.Citation123 Furthermore, KSHV encodes a cluster of three viral IRFs (vIRFs), vIRF1, vIRF2, and vIRF3/latency-associated nuclear antigen 2 (LANA2) ().Citation21,Citation124 The N-terminal region of all vIRFs is homologous of the DBD of mammalian IRFs but lacks several of the tryptophan residues that are essential for DNA binding. Thus, vIRFs are believed to be unable to directly bind DNA. Although the underlying mechanism has not yet been precisely elucidated, vIRF1 has been shown to function as a repressor of virus-mediated induction of Type I IFN genes in a transient transfection assay.Citation125,Citation126 Additionally, vIRF2 and vIRF3/LANA2 reportedly inhibit the transactivation of Type I IFN genes, perhaps by interfering with host IRFs. Finally, vIRF3/LANA2 has been demonstrated to bind to and therefore inhibit the DNA binding activity of IRF7 and IRF5 (refs. Citation127–Citation129).
These viral proteins not only contribute to the persistence of viral infection, but also they may constitute risk factors for virus-induced carcinogenesis. Epstein-Barr virus (EBV) latency has been associated with various human cancers.Citation130 The EBV-encoded latent membrane protein 1 (LMP-1) transforms B lymphocytes into a proliferating lymphoblastoid cell line. LMP-1 has been demonstrated to induce the expression of IRF7 and to activate IRF7 through receptor-interacting protein kinase 1 (RIPK1) and TNF receptor-associated factor 6 (TRAF6).Citation131-Citation133 Since IRF7 has been shown to promote the anchorage-independent growth of NIH3T3 cells and LMP-1 has an additive effect on the growth of these cells, the LMP-1-mediated activation of IRF7 is thought to potentiate the transformation process driven by EBV.Citation133
Human papillomavirus (HPV) is a causative agent of cervical dysplasia and cervical cancer.Citation134 The high-risk Types of HPV (HPV-16 and HPV-18) encode two viral oncogenes, E6 and E7, which inactivate cellular tumor suppressor proteins. E6 binds to p53 and promotes its proteolysis, whereas E7 binds to the hypophosphorylated form of RB and interferes with its binding to E2F.Citation134 Furthermore, these HPV oncoproteins target IRF family members and inhibit their activities. Thus, both E6 and E7 interfere with IRF3-mediated Type I IFN gene induction and IRF1-mediated tumor suppressor activity, respectively, hence promoting the development of cervical tumors.Citation135,Citation136
Implications and Future Prospects
Since the discovery of IRF1, a number of critical roles for the IRF family have been revealed. Although this review focused on the regulation of oncogenesis, IRF members impact a number of aspects of the host defense system, ranging from the activation or attenuation of immune responses (essentially all IRFs) to the regulation of immune cell differentiation (IRF1, IRF2, IRF4 and IRF8) as well as to the regulation of cell growth or death (again, essentially all IRFs).Citation11,Citation12,Citation16,Citation18,Citation20,Citation21 While each member of the IRF family may be assigned a specific function, considerable overlapping exists between the functional profile of distinct IRFs. For instance, many IRFs are activated by pattern recognition receptors (PRRs) during innate immune responses. This occurs because many IRFs (i.e., IRF1, IRF4, IRF5, IRF7 and IRF8) share the ability to interact with the common adaptor protein MYD88 and/or TRAF6 in the TLR-MYD88 pathways, and also because IRF3 and IRF7 can be activated by the nucleic acid-sensing cytosolic receptors and TLRs.Citation11,Citation18,Citation20,Citation21 Active IRFs stimulate an overlapping but distinct set of target genes to shape the appropriate immune response. In this regard, IRFs appear to form a “hub” that integrates and relay signals originating from PRR stimulation. In another example of overlapping and distinct activities, IRF8 and IRF4 contribute to the development of multiple DC subsets, thereby contributing to the functional heterogeneity of DCs.Citation11,Citation20
Of note, an IRF-related gene has been identified in Schistosoma mansoni, a digenetic platyhelminth trematode.Citation137 Hence,IRFs may have an ancient evolutionary origin and may have been lost in Ecdysozoa, such as Drosophila melanogaster and Caenorhabditis elegans, while they persisted in Lophotrochozoa and probably even in Ctenophora and Cnidaria.Citation137,Citation138 Given that the Type I IFN genes have been found so far in vertebrates only, IRFs may have arisen much earlier than this cytokine system. Thus, one may speculate that the Type I IFN system was acquired by vertebrates together with the adaptive immune system, and since then it shaped the complex and efficient defense system of the host against viruses and cancer.
Further molecular insights into the versatile functions of each IRF are needed. Indeed, in spite of the extensive body of knowledge that scientific research has generated on the function of IRFs, critical questions remain. For example, the mechanisms accounting for the oncosuppressive activity of IRFs remain to be fully clarified. Also, as our understanding of the roles of IRFs in the regulation of innate immunity improves, the broader view becomes increasingly complicated by the interrelationships between IRFs and other regulatory systems. The cooperation and antagonism between IRFs and NFκB are particularly intriguing. Both are activated by a remarkably common set of stimuli, such as pathogen associated molecular patterns (PAMPs) and DNA damage, and cooperatively regulate the expression of many cytokines. Still, IRFs and NFκB appear to exert opposite effects on cell growth and survival. In contrast to the tumor suppressive effects of several IRFs, NFκB acts as a potent pro-survival transcription factor and contributes to oncogenesis and tumor progression, in particular of inflammation-associated cancers.Citation139 Therefore, precisely how and to what extent these two transcription factor families cooperate or antagonize each other is an important issue to be addressed. Ultimately, a comprehensive understanding of interacting partners and target genes whereby IRFs and NFκB cross-talk in various Types of cells and in response to multiple stimuli must be achieved.
Since IRFs are critical for two aspects of host defense, i.e., immunity against pathogens and tumor suppression, the IRF family may constitute an attractive therapeutic target for the treatment of malignancies, infectious diseases as well as other disorders with an immune component.
Acknowledgments
We apologize to all of the authors whose invaluable work we could not discuss or cite in this review due to space constraints. This work was supported in part by a Grant-In-Aid for Scientific Research on Innovative Areas from the Ministry of Education, Culture, Sports, Science, and Technology of Japan, and by Core Research for Evolutional Science and Technology (CREST) of the Japan Science and Technology Agency (JST).
References
- Miyamoto M, Fujita T, Kimura Y, Maruyama M, Harada H, Sudo Y, et al. Regulated expression of a gene encoding a nuclear factor, IRF-1, that specifically binds to IFN-β gene regulatory elements. Cell 1988; 54:903 - 13; http://dx.doi.org/10.1016/S0092-8674(88)91307-4; PMID: 3409321
- Harada H, Fujita T, Miyamoto M, Kimura Y, Maruyama M, Furia A, et al. Structurally similar but functionally distinct factors, IRF-1 and IRF-2, bind to the same regulatory elements of IFN and IFN-inducible genes. Cell 1989; 58:729 - 39; http://dx.doi.org/10.1016/0092-8674(89)90107-4; PMID: 2475256
- Driggers PH, Ennist DL, Gleason SL, Mak WH, Marks MS, Levi BZ, et al. An interferon gamma-regulated protein that binds the interferon-inducible enhancer element of major histocompatibility complex class I genes. Proc Natl Acad Sci U S A 1990; 87:3743 - 7; http://dx.doi.org/10.1073/pnas.87.10.3743; PMID: 2111015
- Eisenbeis CF, Singh H, Storb U. Pip, a novel IRF family member, is a lymphoid-specific, PU.1-dependent transcriptional activator. Genes Dev 1995; 9:1377 - 87; http://dx.doi.org/10.1101/gad.9.11.1377; PMID: 7797077
- Levy DE, Kessler DS, Pine R, Darnell JE Jr.. Cytoplasmic activation of ISGF3, the positive regulator of interferon-α-stimulated transcription, reconstituted in vitro. Genes Dev 1989; 3:1362 - 71; http://dx.doi.org/10.1101/gad.3.9.1362; PMID: 2606351
- Matsuyama T, Grossman A, Mittrücker HW, Siderovski DP, Kiefer F, Kawakami T, et al. Molecular cloning of LSIRF, a lymphoid-specific member of the interferon regulatory factor family that binds the interferon-stimulated response element (ISRE). Nucleic Acids Res 1995; 23:2127 - 36; http://dx.doi.org/10.1093/nar/23.12.2127; PMID: 7541907
- Weisz A, Marx P, Sharf R, Appella E, Driggers PH, Ozato K, et al. Human interferon consensus sequence binding protein is a negative regulator of enhancer elements common to interferon-inducible genes. J Biol Chem 1992; 267:25589 - 96; PMID: 1460054
- Yamagata T, Nishida J, Tanaka S, Sakai R, Mitani K, Yoshida M, et al. A novel interferon regulatory factor family transcription factor, ICSAT/Pip/LSIRF, that negatively regulates the activity of interferon-regulated genes. Mol Cell Biol 1996; 16:1283 - 94; PMID: 8657101
- Moore PS, Boshoff C, Weiss RA, Chang Y. Molecular mimicry of human cytokine and cytokine response pathway genes by KSHV. Science 1996; 274:1739 - 44; http://dx.doi.org/10.1126/science.274.5293.1739; PMID: 8939871
- Russo JJ, Bohenzky RA, Chien MC, Chen J, Yan M, Maddalena D, et al. Nucleotide sequence of the Kaposi sarcoma-associated herpesvirus (HHV8). Proc Natl Acad Sci U S A 1996; 93:14862 - 7; http://dx.doi.org/10.1073/pnas.93.25.14862; PMID: 8962146
- Tamura T, Yanai H, Savitsky D, Taniguchi T. The IRF family transcription factors in immunity and oncogenesis. Annu Rev Immunol 2008; 26:535 - 84; http://dx.doi.org/10.1146/annurev.immunol.26.021607.090400; PMID: 18303999
- Taniguchi T, Ogasawara K, Takaoka A, Tanaka N. IRF family of transcription factors as regulators of host defense. Annu Rev Immunol 2001; 19:623 - 55; http://dx.doi.org/10.1146/annurev.immunol.19.1.623; PMID: 11244049
- Darnell JE Jr., Kerr IM, Stark GR. Jak-STAT pathways and transcriptional activation in response to IFNs and other extracellular signaling proteins. Science 1994; 264:1415 - 21; http://dx.doi.org/10.1126/science.8197455; PMID: 8197455
- Fujii Y, Shimizu T, Kusumoto M, Kyogoku Y, Taniguchi T, Hakoshima T. Crystal structure of an IRF-DNA complex reveals novel DNA recognition and cooperative binding to a tandem repeat of core sequences. EMBO J 1999; 18:5028 - 41; http://dx.doi.org/10.1093/emboj/18.18.5028; PMID: 10487755
- Meraro D, Hashmueli S, Koren B, Azriel A, Oumard A, Kirchhoff S, et al. Protein-protein and DNA-protein interactions affect the activity of lymphoid-specific IFN regulatory factors. J Immunol 1999; 163:6468 - 78; PMID: 10586038
- Mamane Y, Heylbroeck C, Génin P, Algarté M, Servant MJ, LePage C, et al. Interferon regulatory factors: the next generation. Gene 1999; 237:1 - 14; http://dx.doi.org/10.1016/S0378-1119(99)00262-0; PMID: 10524230
- Colonna M. TLR pathways and IFN-regulatory factors: to each its own. Eur J Immunol 2007; 37:306 - 9; http://dx.doi.org/10.1002/eji.200637009; PMID: 17273997
- Honda K, Taniguchi T. IRFs: master regulators of signalling by Toll-like receptors and cytosolic pattern-recognition receptors. Nat Rev Immunol 2006; 6:644 - 58; http://dx.doi.org/10.1038/nri1900; PMID: 16932750
- Nguyen H, Hiscott J, Pitha PM. The growing family of interferon regulatory factors. Cytokine Growth Factor Rev 1997; 8:293 - 312; http://dx.doi.org/10.1016/S1359-6101(97)00019-1; PMID: 9620643
- Ozato K, Tailor P, Kubota T. The interferon regulatory factor family in host defense: mechanism of action. J Biol Chem 2007; 282:20065 - 9; http://dx.doi.org/10.1074/jbc.R700003200; PMID: 17502370
- Paun A, Pitha PM. The IRF family, revisited. Biochimie 2007; 89:744 - 53; http://dx.doi.org/10.1016/j.biochi.2007.01.014; PMID: 17399883
- Savitsky D, Tamura T, Yanai H, Taniguchi T. Regulation of immunity and oncogenesis by the IRF transcription factor family. Cancer Immunol Immunother 2010; 59:489 - 510; http://dx.doi.org/10.1007/s00262-009-0804-6; PMID: 20049431
- Takaoka A, Tamura T, Taniguchi T. Interferon regulatory factor family of transcription factors and regulation of oncogenesis. Cancer Sci 2008; 99:467 - 78; http://dx.doi.org/10.1111/j.1349-7006.2007.00720.x; PMID: 18190617
- Harada H, Kitagawa M, Tanaka N, Yamamoto H, Harada K, Ishihara M, et al. Anti-oncogenic and oncogenic potentials of interferon regulatory factors-1 and -2. Science 1993; 259:971 - 4; http://dx.doi.org/10.1126/science.8438157; PMID: 8438157
- Tanaka N, Ishihara M, Lamphier MS, Nozawa H, Matsuyama T, Mak TW, et al. Cooperation of the tumour suppressors IRF-1 and p53 in response to DNA damage. Nature 1996; 382:816 - 8; http://dx.doi.org/10.1038/382816a0; PMID: 8752276
- Pamment J, Ramsay E, Kelleher M, Dornan D, Ball KL. Regulation of the IRF-1 tumour modifier during the response to genotoxic stress involves an ATM-dependent signalling pathway. Oncogene 2002; 21:7776 - 85; http://dx.doi.org/10.1038/sj.onc.1205981; PMID: 12420214
- Frontini M, Vijayakumar M, Garvin A, Clarke N. A ChIP-chip approach reveals a novel role for transcription factor IRF1 in the DNA damage response. Nucleic Acids Res 2009; 37:1073 - 85; http://dx.doi.org/10.1093/nar/gkn1051; PMID: 19129219
- Tanaka N, Ishihara M, Kitagawa M, Harada H, Kimura T, Matsuyama T, et al. Cellular commitment to oncogene-induced transformation or apoptosis is dependent on the transcription factor IRF-1. Cell 1994; 77:829 - 39; http://dx.doi.org/10.1016/0092-8674(94)90132-5; PMID: 8004672
- Tamura T, Ishihara M, Lamphier MS, Tanaka N, Oishi I, Aizawa S, et al. An IRF-1-dependent pathway of DNA damage-induced apoptosis in mitogen-activated T lymphocytes. Nature 1995; 376:596 - 9; http://dx.doi.org/10.1038/376596a0; PMID: 7637809
- Lallemand C, Palmieri M, Blanchard B, Meritet JF, Tovey MG. GAAP-1: a transcriptional activator of p53 and IRF-1 possesses pro-apoptotic activity. EMBO Rep 2002; 3:153 - 8; http://dx.doi.org/10.1093/embo-reports/kvf032; PMID: 11818340
- Kano A, Haruyama T, Akaike T, Watanabe Y. IRF-1 is an essential mediator in IFN-γ-induced cell cycle arrest and apoptosis of primary cultured hepatocytes. Biochem Biophys Res Commun 1999; 257:672 - 7; http://dx.doi.org/10.1006/bbrc.1999.0276; PMID: 10208842
- Kim EJ, Lee JM, Namkoong SE, Um SJ, Park JS. Interferon regulatory factor-1 mediates interferon-gamma-induced apoptosis in ovarian carcinoma cells. J Cell Biochem 2002; 85:369 - 80; http://dx.doi.org/10.1002/jcb.10142; PMID: 11948692
- Tomita Y, Bilim V, Hara N, Kasahara T, Takahashi K. Role of IRF-1 and caspase-7 in IFN-γ enhancement of Fas-mediated apoptosis in ACHN renal cell carcinoma cells. Int J Cancer 2003; 104:400 - 8; http://dx.doi.org/10.1002/ijc.10956; PMID: 12584735
- Tan RS, Taniguchi T, Harada H. Identification of the lysyl oxidase gene as target of the antioncogenic transcription factor, IRF-1, and its possible role in tumor suppression. Cancer Res 1996; 56:2417 - 21; PMID: 8625321
- Jeay S, Pianetti S, Kagan HM, Sonenshein GE. Lysyl oxidase inhibits ras-mediated transformation by preventing activation of NF-κ B. Mol Cell Biol 2003; 23:2251 - 63; http://dx.doi.org/10.1128/MCB.23.7.2251-2263.2003; PMID: 12640111
- Passioura T, Dolnikov A, Shen S, Symonds G. N-ras-induced growth suppression of myeloid cells is mediated by IRF-1. Cancer Res 2005; 65:797 - 804; PMID: 15705876
- Tanaka N, Ishihara M, Taniguchi T. Suppression of c-myc or fosB-induced cell transformation by the transcription factor IRF-1. Cancer Lett 1994; 83:191 - 6; http://dx.doi.org/10.1016/0304-3835(94)90318-2; PMID: 8062214
- Nozawa H, Oda E, Nakao K, Ishihara M, Ueda S, Yokochi T, et al. Loss of transcription factor IRF-1 affects tumor susceptibility in mice carrying the Ha-ras transgene or nullizygosity for p53. Genes Dev 1999; 13:1240 - 5; http://dx.doi.org/10.1101/gad.13.10.1240; PMID: 10346812
- Park J, Kim K, Lee EJ, Seo YJ, Lim SN, Park K, et al. Elevated level of SUMOylated IRF-1 in tumor cells interferes with IRF-1-mediated apoptosis. Proc Natl Acad Sci U S A 2007; 104:17028 - 33; http://dx.doi.org/10.1073/pnas.0609852104; PMID: 17942705
- Harada H, Kondo T, Ogawa S, Tamura T, Kitagawa M, Tanaka N, et al. Accelerated exon skipping of IRF-1 mRNA in human myelodysplasia/leukemia; a possible mechanism of tumor suppressor inactivation. Oncogene 1994; 9:3313 - 20; PMID: 7936656
- Tzoanopoulos D, Speletas M, Arvanitidis K, Veiopoulou C, Kyriaki S, Thyphronitis G, et al. Low expression of interferon regulatory factor-1 and identification of novel exons skipping in patients with chronic myeloid leukaemia. Br J Haematol 2002; 119:46 - 53; http://dx.doi.org/10.1046/j.1365-2141.2002.03829.x; PMID: 12358902
- Kondo T, Minamino N, Nagamura-Inoue T, Matsumoto M, Taniguchi T, Tanaka N. Identification and characterization of nucleophosmin/B23/numatrin which binds the anti-oncogenic transcription factor IRF-1 and manifests oncogenic activity. Oncogene 1997; 15:1275 - 81; http://dx.doi.org/10.1038/sj.onc.1201286; PMID: 9315094
- Narayan V, Halada P, Hernychová L, Chong YP, Žáková J, Hupp TR, et al. A multiprotein binding interface in an intrinsically disordered region of the tumor suppressor protein interferon regulatory factor-1. J Biol Chem 2011; 286:14291 - 303; http://dx.doi.org/10.1074/jbc.M110.204602; PMID: 21245151
- Willman CL, Sever CE, Pallavicini MG, Harada H, Tanaka N, Slovak ML, et al. Deletion of IRF-1, mapping to chromosome 5q31.1, in human leukemia and preleukemic myelodysplasia. Science 1993; 259:968 - 71; http://dx.doi.org/10.1126/science.8438156; PMID: 8438156
- Ogasawara S, Tamura G, Maesawa C, Suzuki Y, Ishida K, Satoh N, et al. Common deleted region on the long arm of chromosome 5 in esophageal carcinoma. Gastroenterology 1996; 110:52 - 7; http://dx.doi.org/10.1053/gast.1996.v110.pm8536888; PMID: 8536888
- Tamura G, Ogasawara S, Nishizuka S, Sakata K, Maesawa C, Suzuki Y, et al. Two distinct regions of deletion on the long arm of chromosome 5 in differentiated adenocarcinomas of the stomach. Cancer Res 1996; 56:612 - 5; PMID: 8564980
- Nozawa H, Oda E, Ueda S, Tamura G, Maesawa C, Muto T, et al. Functionally inactivating point mutation in the tumor-suppressor IRF-1 gene identified in human gastric cancer. Int J Cancer 1998; 77:522 - 7; http://dx.doi.org/10.1002/(SICI)1097-0215(19980812)77:4<522::AID-IJC8>3.0.CO;2-W; PMID: 9679752
- Tirkkonen M, Tanner M, Karhu R, Kallioniemi A, Isola J, Kallioniemi OP. Molecular cytogenetics of primary breast cancer by CGH. Genes Chromosomes Cancer 1998; 21:177 - 84; http://dx.doi.org/10.1002/(SICI)1098-2264(199803)21:3<177::AID-GCC1>3.0.CO;2-X; PMID: 9523192
- Doherty GM, Boucher L, Sorenson K, Lowney J. Interferon regulatory factor expression in human breast cancer. Ann Surg 2001; 233:623 - 9; http://dx.doi.org/10.1097/00000658-200105000-00005; PMID: 11323500
- Cavalli LR, Riggins RB, Wang A, Clarke R, Haddad BR. Frequent loss of heterozygosity at the interferon regulatory factor-1 gene locus in breast cancer. Breast Cancer Res Treat 2010; 121:227 - 31; http://dx.doi.org/10.1007/s10549-009-0509-8; PMID: 19697121
- Cui L, Deng Y, Rong Y, Lou W, Mao Z, Feng Y, et al. IRF-2 is over-expressed in pancreatic cancer and promotes the growth of pancreatic cancer cells. Tumour Biol 2012; 33:247 - 55; http://dx.doi.org/10.1007/s13277-011-0273-3; PMID: 22119988
- Passioura T, Shen S, Symonds G, Dolnikov A. A retroviral library genetic screen identifies IRF-2 as an inhibitor of N-ras-induced growth suppression in leukemic cells. Oncogene 2005; 24:7327 - 36; http://dx.doi.org/10.1038/sj.onc.1208877; PMID: 16007130
- Nguyen H, Mustafa A, Hiscott J, Lin R. Transcription factor IRF-2 exerts its oncogenic phenoType through the DNA binding/transcription repression domain. Oncogene 1995; 11:537 - 44; PMID: 7630638
- Yamamoto H, Lamphier MS, Fujita T, Taniguchi T, Harada H. The oncogenic transcription factor IRF-2 possesses a transcriptional repression and a latent activation domain. Oncogene 1994; 9:1423 - 8; PMID: 8152803
- Vaughan PS, Aziz F, van Wijnen AJ, Wu S, Harada H, Taniguchi T, et al. Activation of a cell-cycle-regulated histone gene by the oncogenic transcription factor IRF-2. Nature 1995; 377:362 - 5; http://dx.doi.org/10.1038/377362a0; PMID: 7566094
- Vaughan PS, van der Meijden CM, Aziz F, Harada H, Taniguchi T, van Wijnen AJ, et al. Cell cycle regulation of histone H4 gene transcription requires the oncogenic factor IRF-2. J Biol Chem 1998; 273:194 - 9; http://dx.doi.org/10.1074/jbc.273.1.194; PMID: 9417064
- Masumi A, Yamakawa Y, Fukazawa H, Ozato K, Komuro K. Interferon regulatory factor-2 regulates cell growth through its acetylation. J Biol Chem 2003; 278:25401 - 7; http://dx.doi.org/10.1074/jbc.M213037200; PMID: 12738767
- Pettersson S, Kelleher M, Pion E, Wallace M, Ball KL. Role of Mdm2 acid domain interactions in recognition and ubiquitination of the transcription factor IRF-2. Biochem J 2009; 418:575 - 85; http://dx.doi.org/10.1042/BJ20082087; PMID: 19032150
- Guichard C, Amaddeo G, Imbeaud S, Ladeiro Y, Pelletier L, Maad IB, et al. Integrated analysis of somatic mutations and focal copy-number changes identifies key genes and pathways in hepatocellular carcinoma. Nat Genet 2012; 44:694 - 8; http://dx.doi.org/10.1038/ng.2256; PMID: 22561517
- Sato M, Suemori H, Hata N, Asagiri M, Ogasawara K, Nakao K, et al. Distinct and essential roles of transcription factors IRF-3 and IRF-7 in response to viruses for IFN-α/β gene induction. Immunity 2000; 13:539 - 48; http://dx.doi.org/10.1016/S1074-7613(00)00053-4; PMID: 11070172
- Oshita F, Sekiyama A, Ito H, Kameda Y, Miyagi Y. Genome-wide cDNA microarray screening of genes related to survival in patients after curative resection of non-small cell lung cancer. Oncol Rep 2006; 16:817 - 21; PMID: 16969500
- Tokunaga T, Naruke Y, Shigematsu S, Kohno T, Yasui K, Ma Y, et al. Aberrant expression of interferon regulatory factor 3 in human lung cancer. Biochem Biophys Res Commun 2010; 397:202 - 7; http://dx.doi.org/10.1016/j.bbrc.2010.05.085; PMID: 20501320
- Heylbroeck C, Balachandran S, Servant MJ, DeLuca C, Barber GN, Lin R, et al. The IRF-3 transcription factor mediates Sendai virus-induced apoptosis. J Virol 2000; 74:3781 - 92; http://dx.doi.org/10.1128/JVI.74.8.3781-3792.2000; PMID: 10729153
- Weaver BK, Ando O, Kumar KP, Reich NC. Apoptosis is promoted by the dsRNA-activated factor (DRAF1) during viral infection independent of the action of interferon or p53. FASEB J 2001; 15:501 - 15; http://dx.doi.org/10.1096/fj.00-0222com; PMID: 11156966
- Kirshner JR, Karpova AY, Kops M, Howley PM. Identification of TRAIL as an interferon regulatory factor 3 transcriptional target. J Virol 2005; 79:9320 - 4; http://dx.doi.org/10.1128/JVI.79.14.9320-9324.2005; PMID: 15994827
- Yanai H, Chen HM, Inuzuka T, Kondo S, Mak TW, Takaoka A, et al. Role of IFN regulatory factor 5 transcription factor in antiviral immunity and tumor suppression. Proc Natl Acad Sci U S A 2007; 104:3402 - 7; http://dx.doi.org/10.1073/pnas.0611559104; PMID: 17360658
- Hsu LC, Park JM, Zhang K, Luo JL, Maeda S, Kaufman RJ, et al. The protein kinase PKR is required for macrophage apoptosis after activation of Toll-like receptor 4. Nature 2004; 428:341 - 5; http://dx.doi.org/10.1038/nature02405; PMID: 15029200
- Kim T, Kim TY, Song YH, Min IM, Yim J, Kim TK. Activation of interferon regulatory factor 3 in response to DNA-damaging agents. J Biol Chem 1999; 274:30686 - 9; http://dx.doi.org/10.1074/jbc.274.43.30686; PMID: 10521456
- Karpova AY, Trost M, Murray JM, Cantley LC, Howley PM. Interferon regulatory factor-3 is an in vivo target of DNA-PK. Proc Natl Acad Sci U S A 2002; 99:2818 - 23; http://dx.doi.org/10.1073/pnas.052713899; PMID: 11867762
- Duguay D, Mercier F, Stagg J, Martineau D, Bramson J, Servant M, et al. In vivo interferon regulatory factor 3 tumor suppressor activity in B16 melanoma tumors. Cancer Res 2002; 62:5148 - 52; PMID: 12234977
- Kim TY, Lee KH, Chang S, Chung C, Lee HW, Yim J, et al. Oncogenic potential of a dominant negative mutant of interferon regulatory factor 3. J Biol Chem 2003; 278:15272 - 8; http://dx.doi.org/10.1074/jbc.M205792200; PMID: 12582166
- Shaffer AL, Emre NC, Romesser PB, Staudt LM. IRF4: Immunity. Malignancy! Therapy?. Clin Cancer Res 2009; 15:2954 - 61; http://dx.doi.org/10.1158/1078-0432.CCR-08-1845; PMID: 19383829
- Mamane Y, Grandvaux N, Hernandez E, Sharma S, Innocente SA, Lee JM, et al. Repression of IRF-4 target genes in human T cell leukemia virus-1 infection. Oncogene 2002; 21:6751 - 65; http://dx.doi.org/10.1038/sj.onc.1205843; PMID: 12360402
- Mamane Y, Loignon M, Palmer J, Hernandez E, Césaire R, Alaoui-Jamali M, et al. Repression of DNA repair mechanisms in IRF-4-expressing and HTLV-I-infected T lymphocytes. J Interferon Cytokine Res 2005; 25:43 - 51; http://dx.doi.org/10.1089/jir.2005.25.43; PMID: 15684621
- Feldman AL, Law M, Remstein ED, Macon WR, Erickson LA, Grogg KL, et al. Recurrent translocations involving the IRF4 oncogene locus in peripheral T-cell lymphomas. Leukemia 2009; 23:574 - 80; http://dx.doi.org/10.1038/leu.2008.320; PMID: 18987657
- Iida S, Rao PH, Butler M, Corradini P, Boccadoro M, Klein B, et al. Deregulation of MUM1/IRF4 by chromosomal translocation in multiple myeloma. Nat Genet 1997; 17:226 - 30; http://dx.doi.org/10.1038/ng1097-226; PMID: 9326949
- Heintel D, Zojer N, Schreder M, Strasser-Weippl K, Kainz B, Vesely M, et al. Expression of MUM1/IRF4 mRNA as a prognostic marker in patients with multiple myeloma. Leukemia 2008; 22:441 - 5; http://dx.doi.org/10.1038/sj.leu.2404895; PMID: 17690696
- Shaffer AL, Emre NC, Lamy L, Ngo VN, Wright G, Xiao W, et al. IRF4 addiction in multiple myeloma. Nature 2008; 454:226 - 31; http://dx.doi.org/10.1038/nature07064; PMID: 18568025
- Saito T, Yamagata T, Takahashi T, Honda H, Hirai H. ICSAT overexpression is not sufficient to cause adult T-cell leukemia or multiple myeloma. Biochem Biophys Res Commun 1999; 260:329 - 31; http://dx.doi.org/10.1006/bbrc.1999.0911; PMID: 10403770
- Yang Y, Shaffer AL 3rd, Emre NC, Ceribelli M, Zhang M, Wright G, et al. Exploiting synthetic lethality for the therapy of ABC diffuse large B cell lymphoma. Cancer Cell 2012; 21:723 - 37; http://dx.doi.org/10.1016/j.ccr.2012.05.024; PMID: 22698399
- Pathak S, Ma S, Trinh L, Eudy J, Wagner KU, Joshi SS, et al. IRF4 is a suppressor of c-Myc induced B cell leukemia. PLoS One 2011; 6:e22628; http://dx.doi.org/10.1371/journal.pone.0022628; PMID: 21818355
- Barnes BJ, Kellum MJ, Pinder KE, Frisancho JA, Pitha PM. Interferon regulatory factor 5, a novel mediator of cell cycle arrest and cell death. Cancer Res 2003; 63:6424 - 31; PMID: 14559832
- Bi X, Hameed M, Mirani N, Pimenta EM, Anari J, Barnes BJ. Loss of interferon regulatory factor 5 (IRF5) expression in human ductal carcinoma correlates with disease stage and contributes to metastasis. Breast Cancer Res 2011; 13:R111; http://dx.doi.org/10.1186/bcr3053; PMID: 22053985
- Yang L, Zhao T, Shi X, Nakhaei P, Wang Y, Sun Q, et al. Functional analysis of a dominant negative mutation of interferon regulatory factor 5. PLoS One 2009; 4:e5500; http://dx.doi.org/10.1371/journal.pone.0005500; PMID: 19430534
- Mori T, Anazawa Y, Iiizumi M, Fukuda S, Nakamura Y, Arakawa H. Identification of the interferon regulatory factor 5 gene (IRF-5) as a direct target for p53. Oncogene 2002; 21:2914 - 8; http://dx.doi.org/10.1038/sj.onc.1205459; PMID: 11973653
- Hu G, Mancl ME, Barnes BJ. Signaling through IFN regulatory factor-5 sensitizes p53-deficient tumors to DNA damage-induced apoptosis and cell death. Cancer Res 2005; 65:7403 - 12; http://dx.doi.org/10.1158/0008-5472.CAN-05-0583; PMID: 16103093
- Couzinet A, Tamura K, Chen HM, Nishimura K, Wang Z, Morishita Y, et al. A cell-Type-specific requirement for IFN regulatory factor 5 (IRF5) in Fas-induced apoptosis. Proc Natl Acad Sci U S A 2008; 105:2556 - 61; http://dx.doi.org/10.1073/pnas.0712295105; PMID: 18268344
- Kondo S, Schutte BC, Richardson RJ, Bjork BC, Knight AS, Watanabe Y, et al. Mutations in IRF6 cause Van der Woude and popliteal pterygium syndromes. Nat Genet 2002; 32:285 - 9; http://dx.doi.org/10.1038/ng985; PMID: 12219090
- Ingraham CR, Kinoshita A, Kondo S, Yang B, Sajan S, Trout KJ, et al. Abnormal skin, limb and craniofacial morphogenesis in mice deficient for interferon regulatory factor 6 (Irf6). Nat Genet 2006; 38:1335 - 40; http://dx.doi.org/10.1038/ng1903; PMID: 17041601
- Richardson RJ, Dixon J, Malhotra S, Hardman MJ, Knowles L, Boot-Handford RP, et al. Irf6 is a key determinant of the keratinocyte proliferation-differentiation switch. Nat Genet 2006; 38:1329 - 34; http://dx.doi.org/10.1038/ng1894; PMID: 17041603
- Moretti F, Marinari B, Lo Iacono N, Botti E, Giunta A, Spallone G, et al. A regulatory feedback loop involving p63 and IRF6 links the pathogenesis of 2 genetically different human ectodermal dysplasias. J Clin Invest 2010; 120:1570 - 7; http://dx.doi.org/10.1172/JCI40267; PMID: 20424325
- Restivo G, Nguyen BC, Dziunycz P, Ristorcelli E, Ryan RJ, Özuysal OY, et al. IRF6 is a mediator of Notch pro-differentiation and tumour suppressive function in keratinocytes. EMBO J 2011; 30:4571 - 85; http://dx.doi.org/10.1038/emboj.2011.325; PMID: 21909072
- Bailey CM, Hendrix MJ. IRF6 in development and disease: a mediator of quiescence and differentiation. Cell Cycle 2008; 7:1925 - 30; http://dx.doi.org/10.4161/cc.7.13.6221; PMID: 18604160
- Bailey CM, Abbott DE, Margaryan NV, Khalkhali-Ellis Z, Hendrix MJ. Interferon regulatory factor 6 promotes cell cycle arrest and is regulated by the proteasome in a cell cycle-dependent manner. Mol Cell Biol 2008; 28:2235 - 43; http://dx.doi.org/10.1128/MCB.01866-07; PMID: 18212048
- Honda K, Yanai H, Negishi H, Asagiri M, Sato M, Mizutani T, et al. IRF-7 is the master regulator of Type-I interferon-dependent immune responses. Nature 2005; 434:772 - 7; http://dx.doi.org/10.1038/nature03464; PMID: 15800576
- Bidwell BN, Slaney CY, Withana NP, Forster S, Cao Y, Loi S, et al. Silencing of Irf7 pathways in breast cancer cells promotes bone metastasis through immune escape. Nat Med 2012; In Press http://dx.doi.org/10.1038/nm.2830; PMID: 22820642
- Li Q, Tainsky MA. Epigenetic silencing of IRF7 and/or IRF5 in lung cancer cells leads to increased sensitivity to oncolytic viruses. PLoS One 2011; 6:e28683; http://dx.doi.org/10.1371/journal.pone.0028683; PMID: 22194884
- Holtschke T, Löhler J, Kanno Y, Fehr T, Giese N, Rosenbauer F, et al. Immunodeficiency and chronic myelogenous leukemia-like syndrome in mice with a targeted mutation of the ICSBP gene. Cell 1996; 87:307 - 17; http://dx.doi.org/10.1016/S0092-8674(00)81348-3; PMID: 8861914
- Burchert A, Cai D, Hofbauer LC, Samuelsson MK, Slater EP, Duyster J, et al. Interferon consensus sequence binding protein (ICSBP; IRF-8) antagonizes BCR/ABL and down-regulates bcl-2. Blood 2004; 103:3480 - 9; http://dx.doi.org/10.1182/blood-2003-08-2970; PMID: 14656881
- Dror N, Rave-Harel N, Burchert A, Azriel A, Tamura T, Tailor P, et al. Interferon regulatory factor-8 is indispensable for the expression of promyelocytic leukemia and the formation of nuclear bodies in myeloid cells. J Biol Chem 2007; 282:5633 - 40; http://dx.doi.org/10.1074/jbc.M607825200; PMID: 17189268
- Tamura T, Kong HJ, Tunyaplin C, Tsujimura H, Calame K, Ozato K. ICSBP/IRF-8 inhibits mitogenic activity of p210 Bcr/Abl in differentiating myeloid progenitor cells. Blood 2003; 102:4547 - 54; http://dx.doi.org/10.1182/blood-2003-01-0291; PMID: 12933588
- Hao SX, Ren R. Expression of interferon consensus sequence binding protein (ICSBP) is downregulated in Bcr-Abl-induced murine chronic myelogenous leukemia-like disease, and forced coexpression of ICSBP inhibits Bcr-Abl-induced myeloproliferative disorder. Mol Cell Biol 2000; 20:1149 - 61; http://dx.doi.org/10.1128/MCB.20.4.1149-1161.2000; PMID: 10648600
- Schmidt M, Nagel S, Proba J, Thiede C, Ritter M, Waring JF, et al. Lack of interferon consensus sequence binding protein (ICSBP) transcripts in human myeloid leukemias. Blood 1998; 91:22 - 9; PMID: 9414265
- Schmidt M, Hochhaus A, Nitsche A, Hehlmann R, Neubauer A. Expression of nuclear transcription factor interferon consensus sequence binding protein in chronic myeloid leukemia correlates with pretreatment risk features and cytogenetic response to interferon-alpha. Blood 2001; 97:3648 - 50; http://dx.doi.org/10.1182/blood.V97.11.3648; PMID: 11369663
- Ortmann CA, Burchert A, Hölzle K, Nitsche A, Wittig B, Neubauer A, et al. Down-regulation of interferon regulatory factor 4 gene expression in leukemic cells due to hypermethylation of CpG motifs in the promoter region. Nucleic Acids Res 2005; 33:6895 - 905; http://dx.doi.org/10.1093/nar/gki1001; PMID: 16396836
- Schmidt M, Hochhaus A, König-Merediz SA, Brendel C, Proba J, Hoppe GJ, et al. Expression of interferon regulatory factor 4 in chronic myeloid leukemia: correlation with response to interferon alfa therapy. J Clin Oncol 2000; 18:3331 - 8; PMID: 11013272
- Deng M, Daley GQ. Expression of interferon consensus sequence binding protein induces potent immunity against BCR/ABL-induced leukemia. Blood 2001; 97:3491 - 7; http://dx.doi.org/10.1182/blood.V97.11.3491; PMID: 11369642
- Tailor P, Tamura T, Kong HJ, Kubota T, Kubota M, Borghi P, et al. The feedback phase of Type I interferon induction in dendritic cells requires interferon regulatory factor 8. Immunity 2007; 27:228 - 39; http://dx.doi.org/10.1016/j.immuni.2007.06.009; PMID: 17702615
- Hu X, Yang D, Zimmerman M, Liu F, Yang J, Kannan S, et al. IRF8 regulates acid ceramidase expression to mediate apoptosis and suppresses myelogeneous leukemia. Cancer Res 2011; 71:2882 - 91; http://dx.doi.org/10.1158/0008-5472.CAN-10-2493; PMID: 21487040
- Liu K, Abrams SI. Coordinate regulation of IFN consensus sequence-binding protein and caspase-1 in the sensitization of human colon carcinoma cells to Fas-mediated apoptosis by IFN-γ. J Immunol 2003; 170:6329 - 37; PMID: 12794166
- Huang W, Zhu C, Wang H, Horvath E, Eklund EA. The interferon consensus sequence-binding protein (ICSBP/IRF8) represses PTPN13 gene transcription in differentiating myeloid cells. J Biol Chem 2008; 283:7921 - 35; http://dx.doi.org/10.1074/jbc.M706710200; PMID: 18195016
- Yang D, Thangaraju M, Greeneltch K, Browning DD, Schoenlein PV, Tamura T, et al. Repression of IFN regulatory factor 8 by DNA methylation is a molecular determinant of apoptotic resistance and metastatic phenoType in metastatic tumor cells. Cancer Res 2007; 67:3301 - 9; http://dx.doi.org/10.1158/0008-5472.CAN-06-4068; PMID: 17409439
- Lee KY, Geng H, Ng KM, Yu J, van Hasselt A, Cao Y, et al. Epigenetic disruption of interferon-gamma response through silencing the tumor suppressor interferon regulatory factor 8 in nasopharyngeal, esophageal and multiple other carcinomas. Oncogene 2008; 27:5267 - 76; http://dx.doi.org/10.1038/onc.2008.147; PMID: 18469857
- Kessler DS, Veals SA, Fu XY, Levy DE. Interferon-alpha regulates nuclear translocation and DNA-binding affinity of ISGF3, a multimeric transcriptional activator. Genes Dev 1990; 4:1753 - 65; http://dx.doi.org/10.1101/gad.4.10.1753; PMID: 2249773
- Takaoka A, Hayakawa S, Yanai H, Stoiber D, Negishi H, Kikuchi H, et al. Integration of interferon-alpha/beta signalling to p53 responses in tumour suppression and antiviral defence. Nature 2003; 424:516 - 23; http://dx.doi.org/10.1038/nature01850; PMID: 12872134
- Weihua X, Lindner DJ, Kalvakolanu DV. The interferon-inducible murine p48 (ISGF3γ) gene is regulated by protooncogene c-myc. Proc Natl Acad Sci U S A 1997; 94:7227 - 32; http://dx.doi.org/10.1073/pnas.94.14.7227; PMID: 9207073
- DiPerna G, Stack J, Bowie AG, Boyd A, Kotwal G, Zhang Z, et al. Poxvirus protein N1L targets the I-kappaB kinase complex, inhibits signaling to NF-kappaB by the tumor necrosis factor superfamily of receptors, and inhibits NF-kappaB and IRF3 signaling by toll-like receptors. J Biol Chem 2004; 279:36570 - 8; http://dx.doi.org/10.1074/jbc.M400567200; PMID: 15215253
- Schröder M, Baran M, Bowie AG. Viral targeting of DEAD box protein 3 reveals its role in TBK1/IKKepsilon-mediated IRF activation. EMBO J 2008; 27:2147 - 57; http://dx.doi.org/10.1038/emboj.2008.143; PMID: 18636090
- Li K, Foy E, Ferreon JC, Nakamura M, Ferreon AC, Ikeda M, et al. Immune evasion by hepatitis C virus NS3/4A protease-mediated cleavage of the Toll-like receptor 3 adaptor protein TRIF. Proc Natl Acad Sci U S A 2005; 102:2992 - 7; http://dx.doi.org/10.1073/pnas.0408824102; PMID: 15710891
- Seth RB, Sun L, Chen ZJ. Antiviral innate immunity pathways. Cell Res 2006; 16:141 - 7; http://dx.doi.org/10.1038/sj.cr.7310019; PMID: 16474426
- Barro M, Patton JT. Rotavirus NSP1 inhibits expression of Type I interferon by antagonizing the function of interferon regulatory factors IRF3, IRF5, and IRF7. J Virol 2007; 81:4473 - 81; http://dx.doi.org/10.1128/JVI.02498-06; PMID: 17301153
- Graff JW, Mitzel DN, Weisend CM, Flenniken ML, Hardy ME. Interferon regulatory factor 3 is a cellular partner of rotavirus NSP1. J Virol 2002; 76:9545 - 50; http://dx.doi.org/10.1128/JVI.76.18.9545-9550.2002; PMID: 12186937
- Yu Y, Wang SE, Hayward GS. The KSHV immediate-early transcription factor RTA encodes ubiquitin E3 ligase activity that targets IRF7 for proteosome-mediated degradation. Immunity 2005; 22:59 - 70; http://dx.doi.org/10.1016/j.immuni.2004.11.011; PMID: 15664159
- Offermann MK. Kaposi sarcoma herpesvirus-encoded interferon regulator factors. Curr Top Microbiol Immunol 2007; 312:185 - 209; http://dx.doi.org/10.1007/978-3-540-34344-8_7; PMID: 17089798
- Burýsek L, Yeow WS, Lubyová B, Kellum M, Schafer SL, Huang YQ, et al. Functional analysis of human herpesvirus 8-encoded viral interferon regulatory factor 1 and its association with cellular interferon regulatory factors and p300. J Virol 1999; 73:7334 - 42; PMID: 10438822
- Li M, Damania B, Alvarez X, Ogryzko V, Ozato K, Jung JU. Inhibition of p300 histone acetyltransferase by viral interferon regulatory factor. Mol Cell Biol 2000; 20:8254 - 63; http://dx.doi.org/10.1128/MCB.20.21.8254-8263.2000; PMID: 11027294
- Burysek L, Yeow WS, Pitha PM. Unique properties of a second human herpesvirus 8-encoded interferon regulatory factor (vIRF-2). J Hum Virol 1999; 2:19 - 32; PMID: 10200596
- Joo CH, Shin YC, Gack M, Wu L, Levy D, Jung JU. Inhibition of interferon regulatory factor 7 (IRF7)-mediated interferon signal transduction by the Kaposi’s sarcoma-associated herpesvirus viral IRF homolog vIRF3. J Virol 2007; 81:8282 - 92; http://dx.doi.org/10.1128/JVI.00235-07; PMID: 17522209
- Wies E, Hahn AS, Schmidt K, Viebahn C, Rohland N, Lux A, et al. The Kaposi’s Sarcoma-associated Herpesvirus-encoded vIRF-3 Inhibits Cellular IRF-5. J Biol Chem 2009; 284:8525 - 38; http://dx.doi.org/10.1074/jbc.M809252200; PMID: 19129183
- Pagano JS. Epstein-Barr virus: the first human tumor virus and its role in cancer. Proc Assoc Am Physicians 1999; 111:573 - 80; http://dx.doi.org/10.1046/j.1525-1381.1999.t01-1-99220.x; PMID: 10591086
- Ning S, Campos AD, Darnay BG, Bentz GL, Pagano JS. TRAF6 and the three C-terminal lysine sites on IRF7 are required for its ubiquitination-mediated activation by the tumor necrosis factor receptor family member latent membrane protein 1. Mol Cell Biol 2008; 28:6536 - 46; http://dx.doi.org/10.1128/MCB.00785-08; PMID: 18710948
- Song YJ, Izumi KM, Shinners NP, Gewurz BE, Kieff E. IRF7 activation by Epstein-Barr virus latent membrane protein 1 requires localization at activation sites and TRAF6, but not TRAF2 or TRAF3. Proc Natl Acad Sci U S A 2008; 105:18448 - 53; http://dx.doi.org/10.1073/pnas.0809933105; PMID: 19017798
- Zhang L, Zhang J, Lambert Q, Der CJ, Del Valle L, Miklossy J, et al. Interferon regulatory factor 7 is associated with Epstein-Barr virus-transformed central nervous system lymphoma and has oncogenic properties. J Virol 2004; 78:12987 - 95; http://dx.doi.org/10.1128/JVI.78.23.12987-12995.2004; PMID: 15542650
- zur Hausen H. Papillomaviruses and cancer: from basic studies to clinical application. Nat Rev Cancer 2002; 2:342 - 50; http://dx.doi.org/10.1038/nrc798; PMID: 12044010
- Park JS, Kim EJ, Kwon HJ, Hwang ES, Namkoong SE, Um SJ. Inactivation of interferon regulatory factor-1 tumor suppressor protein by HPV E7 oncoprotein. Implication for the E7-mediated immune evasion mechanism in cervical carcinogenesis. J Biol Chem 2000; 275:6764 - 9; http://dx.doi.org/10.1074/jbc.275.10.6764; PMID: 10702232
- Ronco LV, Karpova AY, Vidal M, Howley PM. Human papillomavirus 16 E6 oncoprotein binds to interferon regulatory factor-3 and inhibits its transcriptional activity. Genes Dev 1998; 12:2061 - 72; http://dx.doi.org/10.1101/gad.12.13.2061; PMID: 9649509
- Venancio TM, DeMarco R, Almeida GT, Oliveira KC, Setubal JC, Verjovski-Almeida S. Analysis of Schistosoma mansoni genes shared with Deuterostomia and with possible roles in host interactions. BMC Genomics 2007; 8:407; http://dx.doi.org/10.1186/1471-2164-8-407; PMID: 17996068
- Nehyba J, Hrdlicková R, Bose HR. Dynamic evolution of immune system regulators: the history of the interferon regulatory factor family. Mol Biol Evol 2009; 26:2539 - 50; http://dx.doi.org/10.1093/molbev/msp167; PMID: 19638535
- Ben-Neriah Y, Karin M. Inflammation meets cancer, with NF-κB as the matchmaker. Nat Immunol 2011; 12:715 - 23; http://dx.doi.org/10.1038/ni.2060; PMID: 21772280