Abstract
The mature renal medulla, the inner part of the kidney, consists of the medullary collecting ducts, loops of Henle, vasa recta and the interstitium. The unique spatial arrangement of these components is essential for the regulation of urine concentration and other specialized kidney functions. Thus, the proper and timely assembly of medulla constituents is a crucial morphogenetic event leading to the formation of a functioning metanephric kidney. Mechanisms that direct renal medulla formation are poorly understood. This review describes the current understanding of the key molecular and cellular mechanisms underlying morphological aspects of medulla formation. Given that hypoplasia of the renal medulla is a common manifestation of congenital obstructive nephropathy and other types of congenital anomalies of the kidney and urinary tract (CAKUT), better understanding of how disruptions in medulla formation are linked to CAKUT will enable improved diagnosis, treatment and prevention of CAKUT and their associated morbidity.
Introduction
Congenital hydronephrosis, defined as increased diameter of the renal pelvis, is the most frequently identifiable anomaly by prenatal ultrasound with an overall prevalence of 0.5 to 1%.Citation1,Citation2 Moreover, congenital obstructive nephropathy, frequently accompanied by hydronephrosis, is a common cause of renal failure in children.Citation3 In turn, hydronephrosis is frequently associated with effacement of the renal medulla, the inner zone of the kidney.Citation4 Small medulla may accompany renal hypodysplasia, a form of congenital anomaly of the kidney and urinary tract (CAKUT) characterized by reduced number of ureteric bud (UB) branches, presence of undifferentiated mesenchymal and stromal cells, cysts and cartilage, which is observed in 0.027% of fetuses by ultrasonography.Citation5 For example, renal medullary hypodysplasia is observed in patients with Simpson-Golabi-Behmel (OMIM# 312870) and Beckwith-Wiedeman (OMIM# 130650) syndromes.Citation6,Citation7 Although hypoplastic renal medulla may result from backward pressure to the renal parenchyma due to urinary stasis,Citation8 emerging evidence indicates that it may be also due to an intrinsic defect in medullary morphogenesis.Citation9
Major Steps in Kidney Organogenesis
On the fifth week of gestation in humans (embryonic day E10.5 in mice), the caudal portion of the nephric duct forms an epithelial outgrowth called the UB, which invades the metanephric mesenchyme.Citation10,Citation11 UB branches repeatedly within the mesenchyme to form the renal collecting system (the ureter, pelvis, calyces and collecting ducts).Citation10,Citation11 Proximal ureter subsequently translocates from the nephric duct to fuse with the bladder which originates from the urogenital sinus.Citation12 As distal UBs branch, the mesenchymal cells condense around the UB tips to form nephrons (the glomerulus, proximal and distal tubules and loop of Henle). Kidney vascularization is synchronized with nephrogenesis and occurs by vasculogenesis (formation of new blood vessels from endothelial cell precursors) and angiogenesis (sprouting of new capillaries from pre-existing blood vessels).Citation13,Citation14 The stromal mesenchyme or stroma is a complex mixture of cell types which surround nascent UBs and nephrons, do not differentiate into nephrons or the UBs/collecting ducts and give rise to renal interstitium.Citation15
Brief Overview of Morphology and Function of Mature Renal Medulla
The medulla of the adult kidney has a modified cone shape with a broad base adjacent to renal cortex and the narrow apex termed papilla. The mature renal medulla consists of the medullary collecting ducts, loops of Henle, vasa recta (straight capillaries) and the interstitium (lipid-laden interstitial cells, lymphocyte-like cells and pericytes).Citation16 The main function of the medulla is to regulate concentration of the urine. The urine flows from the collecting ducts into the renal calyces and pelvis, which undergoes unidirectional peristaltic movements to allow drainage of the urine into the downstream ureter and bladder.Citation8 In addition, the renal papilla is a niche for adult kidney stem cells which may play a role in repair after ischemic kidney injury.Citation17,Citation18
Developmental Aspects of Medullary Morphogenesis
Development of collecting ducts
Morphologic development of collecting ducts
Collecting ducts and other components of the renal collecting system (calyces, pelvis and the ureter) are formed during kidney development as a result of sequential branching, elongation and patterning (gradual acquisition of structure characteristic of mature organ) of the UB, driven by reciprocal inductive interactions with the metanephric mesenchyme and stroma.Citation10,Citation19 In the mouse, renal medulla becomes identifiable morphologically on E15.5, expands longitudinally (along the corticomedullary axis), increases in length 4.5-fold from E15.5 to birth (mouse gestation period is 20 d) and represents 30% of total kidney volume at birth ().Citation20 The papilla grows through proliferation of collecting duct cells in a distal-to-proximal fashion, with cell proliferation arresting in the distal papilla before E17.5, a time when extensive proliferative growth continues in the proximal papilla.Citation21 In the human kidney, once UB branching is completed by 20–22 weeks of gestation, subsequent morphogenesis of collecting ducts occurs by extension and patterning of peripheral branch segments.Citation19 Initial generations of UB branches undergo remodeling (change in structure or form) by dilatation and growth to form the ureter, renal pelvis and calyces.Citation11 The 6th–8th generations of UB branches give rise to the long unbranched outer medullary collecting ducts in mice.Citation20 While significant longitudinal elongation of the medullary collecting ducts accompanies renal medulla formation,Citation20 the mechanisms by which linear arrays of inner medullary collecting ducts converge centrally to form the papilla and how many collecting ducts enter the tip of the papilla remains unknown.
Figure 1. Renal medulla development in the mouse. (A) Whole mount immunohistochemistry of E13.5 kidney. Ureteric buds (UB) and their derivatives (ureter, Ur) are visualized with anti-pancytokeratin antibody (green). Medulla is not identifiable morphologically at this stage. (B) Longitudinal section through a newborn (postnatal day P1) kidney. Medulla and papilla (Pa) are identifiable morphologically. UB-derived collecting ducts (CDs) are visualized with anti-pancytokeratin antibody (green). (C) Transverse section through P14 kidney stained with hematoxylin and eosin. Long papilla (Pa) is present at this stage.
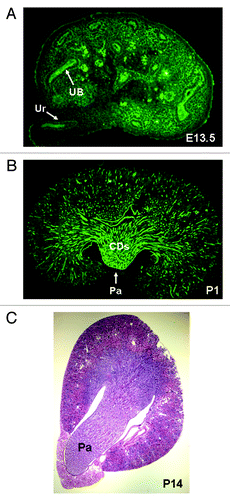
Genetic control of collecting duct morphogenesis
Recent genetic studies in mice demonstrate that medullary hypoplasia may be due, in part, to an intrinsic defect in medullary morphogenesis normally driven by longitudinal elongation of UB-derived collecting ducts (). Global deletion of cell surface heparan sulfate proteoglycan glypican3 (Gpc3), expressed in the UB, in mice causes renal medullary cystic dysplasia due to increased apoptosis of medullary collecting duct cells.Citation22,Citation23 Global genetic inactivation of α3 integrin, expressed in the UB and their derivative collecting ducts, in mice results in hypoplastic medulla due to decreased branching of the medullary collecting ducts.Citation24 Genetic removal of estrogen-related receptor gamma (Esrrg), expressed in the UB and UB-derived collecting ducts, in mice results in medullary hypoplasia without detectable morphological changes in the renal pelvis or the ureter.Citation25 Targeted removal of bone morphogenic protein (BMP) receptor Alk3 in the UB reduces the number of UB branches and medullary collecting ducts resulting in medullary hypoplasia.Citation26 UB-specific inactivation of the fibroblast growth factor receptor (FGFR) in mice decreases UB branching and leads to abnormally shaped and hydronephrotic kidneys.Citation27 Deletion of the epidermal growth factor receptor (EGFR) in the UB in mice, normally expressed in the UB trunk domain, does not appear to alter UB branching, but results in moderate dilation of the medullary collecting ducts, thin medulla and reduced urine-concentrating ability.Citation28 Absence of Wnt7b, a secreted protein which is also normally expressed in UB trunk, is accompanied by dilated collecting ducts that have reduced number of branch points and leads to a failure of medullary development.Citation9 These changes in Wnt7b mutants are likely due to an abnormal plane of collecting duct cell division where cell division occurs orthogonal to the longitudinal axis of the collecting ducts. In contrast, inactivation of Dickkopf1 (Dkk1), a secreted Wnt antagonist, in the UB lineage in mice leads to overgrowth of the whole papilla resulting from enhanced proliferation of the collecting duct and loop of Henle cells.Citation29 This effect of absent Dkk1 may be due to induction of Wnt7b signaling from the collecting duct cells to the stroma.Citation29 The findings that ureter and renal pelvis are comparable between Wnt7b-null and wild-type kidneysCitation9 indicate that medullary hypoplasia may occur in the presence of normally structured pelvis and ureter. Moreover, the findings that selective removal of β-catenin, a key mediator of canonical Wnt signaling, from the interstitium phenocopies the medullary hypoplasia of Wnt7b mutants underscores the importance of cross-talk between nascent collecting ducts and stroma in medulla formation.Citation9 Together, these findings demonstrate that defects in UB branching and collecting duct growth may be causally linked to medullary hypodysplasia in mice.
Table 1. Gene mutations leading to defects in morphogenesis of renal medulla in mice
Development of the loop of Henle (LOH)
Anatomical development of the LOH
The LOH is the anatomical basis for the countercurrent multiplier system that maintains the osmotic gradient in the renal medulla. Two types of LOH are distinguished in the mature kidney: short and long. Short loops make a turn in the outer medulla and long loops in the inner medulla. The outer medulla contains thick descending and ascending (TAL) limbs and descending thin limbs.Citation16 The inner medulla contains descending and ascending thin limbs. Long loops loop back at successive levels in the medulla and only few penetrate into the tip of the papilla.Citation16 In the adult rat kidney, about 1,500 of 10,000 loops that enter the inner medulla reach the second half of the inner medulla and only about 250 enter the last millimeter of the papilla.Citation16 In the newborn rat kidney, renal medulla is not separated into an outer and inner zone, lacks ascending thin limbs and has the structural composition characteristic of the mature inner stripe of the outer medulla.Citation30 During the first 2 weeks of the postnatal life (when nephrogenesis continues in the rat), immature TALs in the renal papilla are transformed into thin limbs by apoptotic deletion of cells in thick limbs and the growth of the loops of Henle occurs mainly at the corticomedullary junction.Citation21,Citation30,Citation31 Low levels of circulating glucocorticoids promote proliferation of TAL cells and elongation of the outer medulla during postnatal development in the rat.Citation32
Genetic control of LOH morphogenesis
Recent studies showed that several genes are essential in the formation of the LOH (). Studies in Xenopus demonstrated that Iroquois (Irx) 3 is necessary for intermediate tubule formation.Citation33 Intermediate tubule progenitors give rise to epithelia of LOH in mammals. Generation of conditional Irx3-mutant mice will clarify the role of Irx3 in LOH development in mammals. In the developing mouse kidney, Adamts-1 (disintegrin and metalloproteinase with thrombospondin motifs) expression is specifically detected in the LOH.Citation34 Adamts-1-null mice display thin medulla and hydronephrosis in the absence of morphological alterations in the renal pelvis or ureter.Citation34 Given that Adamts-1 is a secreted zinc metalloproteinase, it is conceivable that it plays a role in the remodeling of the ECM in medullary interstitium to facilitate elongation of maturing LOH. Mice deficient in POU transcription factor Brn1, expressed in the TAL, exhibit decreased number and length of LOH, enhanced apoptosis of LOH cells and reduced expression of TAL-specific genes.Citation35 Thus, Brn1 is required for growth and differentiation of the loop of Henle. One of the mechanisms by which Brn1 promotes elongation of the LOH may involve suppression of cell apoptosis in primitive loop. To this end, emerging evidence demonstrates that defects in growth and elongation of the developing LOH may account for medullary hypodysplasia. While growth, elongation and remodeling of LOH can be explained by segment-specific cell proliferation, apoptosis and differentiation, the mechanisms directing bending and turning of the loop remain to be elucidated. Other challenges include identification of molecular networks that regulate morphogenesis of LOH and their cross-talk among the loop, stroma/interstitium, collecting ducts and vasculature.
Development of medullary vasculature
Anatomical development of vasa recta
Vasa recta are straight capillaries that branch off the efferent arterioles of juxtamedullary nephrons, enter the medulla and surround the LOH. These vessels play an important role in the maintenance of mass balance and osmotic gradient in the medulla by returning the NaCl and water reabsorbed in the LOH and medullary collecting tubule to the systemic circulation. The vasa recta function as countercurrent exchangers to delay the washout of NaCl and urea and prevent the excess accumulation of water in the inner medulla.Citation36 In the developing rat kidney, vasa recta bundles grow centrally into the medulla around the previously developed collecting ducts.Citation37
Factors that control angiogenesis of vasa recta
Angiogenic factors
Angiogenic factors not only direct developmental angiogenesis, but provide fundamental cues for cell fate specification, embryo patterning, organ differentiation and postnatal tissue remodeling.Citation38 Recent data demonstrate that impaired development of medullary microcirculation may be causally linked to medullary hypoplasia observed in AT1R-mutant mice.Citation39,Citation40 Treatment of newborn rats with the specific AT1R antagonist reduces length, volume and surface area of capillaries in the renal medulla, and inhibits cell proliferation and organization of the developing vasa recta bundles.Citation41 These changes are accompanied by decreased expression of angiogenic growth factors such as vascular endothelial growth factor (VEGF), angiopoietins 1 and 2, Flk1/Flt1 and Tie1 in the medulla, and lead to decreased renal blood flow later in life. Moreover, administration of AT1R antagonist during pregnancy in humans results in atrophy of the renal medulla and poorly developed vasa recta in the fetal kidney.Citation42 Double-mutant mice deficient in high mobility group (HMG) box transcription factor genes Sox17/Sox18, show reduced neovascularization in the outer medulla vasa recta on postnatal day 7.Citation43 Notably, AT1R or Sox17 mutations are causative factors in CAKUT in humans.Citation44,Citation45 Whether structural maldevelopment of the medulla affects vasa recta formation or defects in vascular development account for decreased size of the medulla remains to be determined. Regardless, aberrant medullary microcirculation may have a major role in how fetal reprogramming results in postnatal diseases such as hypertension and progressive kidney disease.
Embryonic blood flow and oxygenation
The role of embryonic blood supply in the development of medullary vasculature in vivo and the mechanisms by which aberrant blood flow in the embryonic kidney may affect medulla formation are poorly understood. It is plausible that the inflow of blood into the renal medulla, with the resulting increase in oxygen tension, may regulate both the development of vasa recta and medullary morphogenesis. This possibility is supported by the findings that mice deficient in Cited1, a transcriptional co-factor expressed in the induced metanephric mesenchyme of the embryonic kidney, have renal medullary dysplasia that does not result from defects in UB branching or lower urinary tract obstruction, but may be due to intrauterine hypoxia resulting from placental insufficiency.Citation46 Hypoxia-inducible factors (HIF) HIF-1 and HIF-2 are transcription factors induced in hypoxia to alleviate hypoxic stress, in part by promoting neovascularization.Citation47 HIF-1 and HIF-2 mRNAs are highly expressed in the medulla, and particularly in the medullary collecting ducts, of the newborn mouse kidney in vivo.Citation48 In addition, VEGF mRNA is detected in the outer medullary collecting ducts dissected from the developing rat kidney on P14.Citation41 Moreover, the expression of HIF-1, HIF-2 and VEGF, a crucial regulator of vascular development, is induced in embryonic kidneys maintained in hypoxic organ cultures.Citation48 These data suggest that HIF stabilization by hypoxia may be critical for VEGF production and kidney vascular development. Potential role for HIF-1 in the regulation of medulla growth is supported by the findings that administration of dominant-negative HIF-1 induces severe damage in the medulla of normal adult rats.Citation49
Recent studies using in utero intracardiac injection of vascular tracer to living mouse embryos demonstrate that perfusion of embryonic pancreas correlates with pancreatic cell differentiation in the region of the blood flow in vivo.Citation50 Given that the regions adjacent to perfused vessels have more oxidized thiols, the authors propose that enhanced oxygen delivery after the inflow of blood is a likely permissive factor for initiating cell differentiation.Citation50 Thus, vascular flow, rather than vascular endothelium alone, may provide specific signals necessary for regional organ differentiation and complete vessel morphogenesis (e.g., development of anastomoses between the main vessels that carry blood flow and small vessels that do not have blood flow) during early embryogenesis. In accord with this hypothesis, cardiac-specific deletion of a key angiogenic factor angiopoietin 1 in mice recapitulates kidney vascular defects observed in global angiopoietin 1 knockout.Citation51 Application of in utero intracardiac injection of vascular tracer to living embryos in model organisms to measure maturation of the embryonic medullary perfusion may provide correlative evidence that enhanced blood flow underlies normal morphogenesis of the renal medulla.
Development of interstitium
Anatomical development of medullary interstitium
The medullary interstitium of the adult kidney consists of lipid-laden interstitial cells, lymphocyte-like cells, pericytes and extracellular matrix (ECM), and provides a structural supportive framework around collecting ducts, loops of Henle and vasa recta.Citation16,Citation52 Other possible functions of mature medullary interstitial cells include production of ECM and prostaglandins.Citation16 The interstitium of the adult kidney originates from the renal stroma of the developing metanephros.Citation15,Citation53 During early metanephric development, fibroblast-like spindle-shaped stromal cells are detected around Pax2-positive cell of the cap mesenchyme and later on around nascent UBs and nephrons.Citation54 These stromal cells specifically express forkhead box transcription factor Foxd1.Citation54 Later in kidney development, stroma is divided into two distinct populations: cortical and medullary. Cortical stroma is characterized by the expression of Foxd1, retinoic acid (RA) receptors (RAR) α and β2, and retinaldehyde dehydrogenase 2, an enzyme required for most fetal RA synthesis. Medullary stroma expresses fibroblast growth factor 7 (FGF7), basic helix-loop-helix transcription factor Pod1 and bone morphogenic protein 4 (BMP4).Citation55-Citation57 Whether molecular differences between these two stromal populations imply a lineage relationship is currently unknown. While medullary stromal cells eventually differentiate into mature interstitial cells, pericytes or neurons,Citation58 it is conceivable that a subpopulation of stromal cells that do not differentiate into other cell types may undergo apoptosis during late gestation to free space for LOH as they extend toward the tip of the papilla.Citation59 Whether apoptosis of medullary stromal cells indeed promotes elongation of LOH or collecting ducts during later stages of kidney development warrants further investigations.
Developmental functions of the metanephric stroma
Besides forming adult interstitium, stroma modulates glomerulogenesis and UB branching in the developing metanephros.Citation54,Citation60,Citation61 The contribution of stroma to morphogenesis of renal medulla is demonstrated by aberrant medullary development in mice that are deficient in genes expressed exclusively in the stroma (). Kidneys of Foxd1-null mice have a reduced number of UB branches and ectopic mesenchymal condensates in the medulla.Citation54 Genetic inactivation of FGF7 in mice causes a decrease in the size of the UB compartment and in total kidney size.Citation62 BMP4 mutants manifest hypoplastic-dysplastic kidneys, duplex kidneys with bifid ureters and hydronephrosis.Citation57 Combined absence of RA receptors RAR α and β2 or retinaldehyde dehydrogenase 2 causes formation of small kidneys with reduced number of UB branches.Citation61,Citation63 In Pod1-null mice, fibroblast-like spindle-shaped stromal cells are absent and renal medulla does not form.Citation64 Similar phenotype is observed in mice that lack a homeodomain transcription factor Pbx1.Citation65 Notably, Pod1 expressed in the stromal, but not in the metanephric, mesenchyme is critical for the proper UB and glomerular development.Citation64 Mutations in cell cycle regulatory gene p57KIP2, expressed in embryonic renal stroma, in mice leads to medullary hypodysplasia, a phenotype very similar to that of patients with Beckwith-Wiedemann syndrome (OMIM# 130650).Citation66 Simpson-Golabi-Behmel syndrome (OMIM# 312870), caused by mutations of heparin sulfate proteoglycan GPC3, leads to dysplastic renal medulla secondary to increased proliferation of UB cells followed by enhanced apoptosis of medullary collecting ducts.Citation7 These observations suggest that balance of cell proliferation and apoptosis is essential during morphogenesis of the renal medulla. Collectively, these findings indicate that aberrant stromal signaling is causally linked to medullary hypodysplasia.
Development of renal neuronal cells
Anatomical development of renal nerves
Despite recognition of the important role of the renal sympathetic nerve activity in the regulation of renin secretion, sodium reabsorption and vascular tone,Citation67 our knowledge of the role of the renal neuronal cells during metanephric development is still rudimentary. Sympathetic nerves grow into the embryonic kidney following the track of blood vessels.Citation68,Citation69 Terminal axon arborization to allow innervation of multiple target cells also parallels vessel sprouting. Nerve fibers are first detected in the fetal rat kidney on the outer aspect of the main branches of the renal artery at E17.Citation69 The density of nerve fibers increases progressively with age and extends to afferent and efferent arterioles. On postnatal day 15 of life in the rat, nerve fibers are also detected in the medulla where vasa recta are developing.Citation68 The human fetal kidney, including renal medulla, is richly innervated during the 2nd and 3rd trimesters when collecting duct patterning and nephrogenesis continue.Citation70 In the mature rabbit kidney, neuropeptide Y-positive neurons are detected in the medulla where they regulate renal medullary perfusion.Citation71
Factors that control development of renal nerves
The guidance of axons is directed by guidance molecules such as semaphorin 3A, Slits and their Robo receptors, Ephrins and their Eph receptors and others.Citation69 In the rat kidney, neurofilament-, L1 neural cell adhesion protein- and neurotrophin 3 nerve growth factor-positive neuronal precursor cells are present early in embryonic development.Citation72 Functionally, neurotrophin 3 promotes survival and induces differentiation of embryonic renal neuronal cells.Citation72 Of interest, L1 is expressed in the collecting ducts but not in the renal stroma. Expression of neurofilament in stromal cells raises the possibility that they may derive from the neural crest rather than from the mesoderm. In the central nervous system (CNS), AT2R promotes neurite outgrowth and elongation.Citation73 Since AT2R is expressed in the medullary stroma of the embryonic mouse kidney,Citation74 AT2R may influence nerve growth in the forming renal medulla. In addition to a potential role in the regulation of medulla morphogenesis per se, aberrant innervation of the renal medulla may be causally linked to development of programmed hypertension in the offspring in later life.Citation75
Impact of lower urinary tract obstruction on structural development of the renal medulla
Murine models of renal hypodysplasia demonstrate that blunting of renal papilla and medulla may result from anatomical or functional obstruction of the lower urinary tract at the level of the renal pelvis or the ureter. Targeted deletion of calcineurin b1 (Cnb1) or hedgehog effector smoothened (Smo) in periureteral mesenchyme in mice causes nonobstructive hydronephrosis, hydroureter and medullary hypoplasia not associated with abnormal collecting duct morphogenesis.Citation76,Citation77 Thus, medullary hypoplasia observed in these mice may result from backward pressure to the renal parenchyma due to urinary stasis. The mechanisms that have been implicated in functional ureteral obstruction in these mice include defective ureteral peristalsis (failure of ureteral peristalsis to propagate in a sequential proximal-distal direction), reduced number of ureteral pacemaker cells and decreased proliferation of periureteral mesenchymal cells.Citation76,Citation77 Although medullary hypoplasia and hydronephrosis observed in angiotensin (Ang) II AT1 receptor (AT1R)-deficient mice may result from impaired ureteral peristalsis due to hypoplastic ureteral smooth muscle layer,Citation78 our previous studies suggest that aberrant branching morphogenesis of the UB may also contribute to medullary defects observed in these mutants.Citation79 The possibility that medullary hypoplasia may be due in some cases to an intrinsic defect in medullary morphogenesis, rather than to urinary tract obstruction, is supported by the findings that Wnt7b- and Adamts 1/4- or Esrrg-null mice exhibit hypoplastic renal medulla at birth in the absence of structural abnormalities of the lower urinary tract.Citation9,Citation25,Citation34 Of interest, renal tubular dysgenesis, a congenital and frequently fatal human disease due to mutations in angiotensinogen, renin, angiotensin-converting enzyme or AT1R genes is characterized by collapsed medullary collecting ducts and abundant interstitial fibrosis that occur in the absence of papillary hypoplasia or hydronephrosis.Citation80 The reasons for occurance of papillary hypoplasia and hydronephrosis in mice, but not in humans, with AT1R gene mutations remain to be determined. Defining embryonic origin and lineage of renal pacemaker cells and the mechanisms that regulate differentiation of these cells could lead to the discovery of new treatments for congenital functional obstruction of the urinary tract.
Conclusions and Perspectives
Although the renal medulla is essential for normal renal physiology, more work is needed to develop an integrated mechanistic understanding of its morphogenesis. A novel emerging paradigm suggests that the cross-talk among renal stroma, collecting ducts, LOH and vasculature is essential in spatiotemporal development of the renal medulla. Understanding the molecular cues for progenitor differentiation, directional elongation, patterning and remodeling, blood flow and blood-borne oxygen delivery, developmental transport physiology and collecting system contractility are fundamental biologic questions with applicability to multiple renal and extrarenal disease processes. Defining the mechanisms that direct morphogenesis of medulla offers opportunity to understand pathogenesis of CAKUT, identify biomarkers of risk and to conceive new preventive and therapeutic strategies. Future studies investigating the effect of spatiotemporal compartment/cell type-specific inactivation and epigenetic imprinting of the genes that govern morphogenesis of the medulla, identification of the association of known genetic mutations linked to medullary hypodysplasia with human CAKUT will provide essential information regarding the mechanisms whereby critical signaling networks direct formation of the medulla.
Abbreviations: | ||
Ang II | = | angiotensin II |
CAKUT | = | congenital anomalies of the kidney and urinary tract |
E | = | embryonic |
LOH | = | loop of Henle |
TAL | = | thick ascending loop of Henle |
UB | = | ureteric bud |
References
- Dudley JA, Haworth JM, McGraw ME, Frank JD, Tizard EJ. Clinical relevance and implications of antenatal hydronephrosis. Arch Dis Child Fetal Neonatal Ed 1997; 76:F31 - 4; http://dx.doi.org/10.1136/fn.76.1.F31; PMID: 9059183
- Garne E, Loane M, Wellesley D, Barisic I, Eurocat Working Group. Congenital hydronephrosis: prenatal diagnosis and epidemiology in Europe. J Pediatr Urol 2009; 5:47 - 52; http://dx.doi.org/10.1016/j.jpurol.2008.08.010; PMID: 18977697
- Chevalier RL. Pathophysiology of obstructive nephropathy in the newborn. Semin Nephrol 1998; 18:585 - 93; PMID: 9819149
- Harold C. Renal and urological disorders. In: Williams L, Staff W, eds. Professional guide to diseases. 9. Springhouse: Springhouse Corp, 2009: 403-4.
- Wiesel A, Queisser-Luft A, Clementi M, Bianca S, Stoll C, EUROSCAN Study Group. Prenatal detection of congenital renal malformations by fetal ultrasonographic examination: an analysis of 709,030 births in 12 European countries. Eur J Med Genet 2005; 48:131 - 44; http://dx.doi.org/10.1016/j.ejmg.2005.02.003; PMID: 16053904
- Hatada I, Ohashi H, Fukushima Y, Kaneko Y, Inoue M, Komoto Y, et al. An imprinted gene p57KIP2 is mutated in Beckwith-Wiedemann syndrome. Nat Genet 1996; 14:171 - 3; http://dx.doi.org/10.1038/ng1096-171; PMID: 8841187
- Pilia G, Hughes-Benzie RM, MacKenzie A, Baybayan P, Chen EY, Huber R, et al. Mutations in GPC3, a glypican gene, cause the Simpson-Golabi-Behmel overgrowth syndrome. Nat Genet 1996; 12:241 - 7; http://dx.doi.org/10.1038/ng0396-241; PMID: 8589713
- Constantinou CE. Renal pelvic pacemaker control of ureteral peristaltic rate. Am J Physiol 1974; 226:1413 - 9; PMID: 4833997
- Yu J, Carroll TJ, Rajagopal J, Kobayashi A, Ren Q, McMahon APA. A Wnt7b-dependent pathway regulates the orientation of epithelial cell division and establishes the cortico-medullary axis of the mammalian kidney. Development 2009; 136:161 - 71; http://dx.doi.org/10.1242/dev.022087; PMID: 19060336
- Costantini F, Kopan R. Patterning a complex organ: branching morphogenesis and nephron segmentation in kidney development. Dev Cell 2010; 18:698 - 712; http://dx.doi.org/10.1016/j.devcel.2010.04.008; PMID: 20493806
- Song R, Yosypiv IV. Genetics of congenital anomalies of the kidney and urinary tract. Pediatr Nephrol 2011; 26:353 - 64; http://dx.doi.org/10.1007/s00467-010-1629-4; PMID: 20798957
- Mendelsohn C. Using mouse models to understand normal and abnormal urogenital tract development. Organogenesis 2009; 5:306 - 14; PMID: 19568352
- Gomez RA, Norwood VF, Tufro-McReddie A. Development of the kidney vasculature. Microsc Res Tech 1997; 39:254 - 60; http://dx.doi.org/10.1002/(SICI)1097-0029(19971101)39:3<254::AID-JEMT5>3.0.CO;2-K; PMID: 9372498
- Sequeira Lopez ML, Gomez RA. Development of the Renal Arterioles. J Am Soc Nephrol 2011; 22:2156 - 65; PMID: 22052047
- Cullen-McEwen LA, Caruana G, Bertram JF. The where, what and why of the developing renal stroma. Nephron Exp Nephrol 2005; 99:e1 - 8; http://dx.doi.org/10.1159/000081792; PMID: 15637462
- Kriz W. Structural organization of the renal medulla: comparative and functional aspects. Am J Physiol 1981; 241:R3 - 16; PMID: 7018270
- Oliver JA, Maarouf O, Cheema FH, Martens TP, Al-Awqati Q. The renal papilla is a niche for adult kidney stem cells. J Clin Invest 2004; 114:795 - 804; PMID: 15372103
- Oliver JA, Klinakis A, Cheema FH, Friedlander J, Sampogna RV, Martens TP, et al. Proliferation and migration of label-retaining cells of the kidney papilla. J Am Soc Nephrol 2009; 20:2315 - 27; http://dx.doi.org/10.1681/ASN.2008111203; PMID: 19762493
- Rosenblum ND. Developmental biology of the human kidney. Semin Fetal Neonatal Med 2008; 13:125 - 32; http://dx.doi.org/10.1016/j.siny.2007.10.005; PMID: 18096451
- Cebrín C, Borodo K, Charles N, Herzlinger DA. Morphometric index of the developing murine kidney. Dev Dyn 2004; 231:601 - 8; http://dx.doi.org/10.1002/dvdy.20143; PMID: 15376282
- Adams DC, Oxburgh L. The long-term label retaining population of the renal papilla arises through divergent regional growth of the kidney. Am J Physiol Renal Physiol 2009; 297:F809 - 15; http://dx.doi.org/10.1152/ajprenal.90650.2008; PMID: 19535568
- Cano-Gauci DF, Song HH, Yang H, McKerlie C, Choo B, Shi W, et al. Glypican-3-deficient mice exhibit developmental overgrowth and some of the abnormalities typical of Simpson-Golabi-Behmel syndrome. J Cell Biol 1999; 146:255 - 64; PMID: 10402475
- Grisaru S, Cano-Gauci D, Tee J, Filmus J, Rosenblum ND. Glypican-3 modulates BMP- and FGF-mediated effects during renal branching morphogenesis. Dev Biol 2001; 231:31 - 46; http://dx.doi.org/10.1006/dbio.2000.0127; PMID: 11180950
- Kreidberg JA, Donovan MJ, Goldstein SL, Rennke H, Shepherd K, Jones RC, et al. Alpha 3 beta 1 integrin has a crucial role in kidney and lung organogenesis. Development 1996; 122:3537 - 47; PMID: 8951069
- Berry R, Harewood L, Pei L, Fisher M, Brownstein D, Ross A, et al. Esrrg functions in early branch generation of the ureteric bud and is essential for normal development of the renal papilla. Hum Mol Genet 2011; 20:917 - 26; http://dx.doi.org/10.1093/hmg/ddq530; PMID: 21138943
- Hartwig S, Bridgewater D, Di Giovanni V, Cain J, Mishina Y, Rosenblum ND. BMP receptor ALK3 controls collecting system development. J Am Soc Nephrol 2008; 19:117 - 24; http://dx.doi.org/10.1681/ASN.2007010080; PMID: 18178801
- Zhao H, Kegg H, Grady S, Truong HT, Robinson ML, Baum M, et al. Role of fibroblast growth factor receptors 1 and 2 in the ureteric bud. Dev Biol 2004; 276:403 - 15; http://dx.doi.org/10.1016/j.ydbio.2004.09.002; PMID: 15581874
- Zhang Z, Pascuet E, Hueber PA, Chu L, Bichet DG, Lee TC, et al. Targeted inactivation of EGF receptor inhibits renal collecting duct development and function. J Am Soc Nephrol 2010; 21:573 - 8; http://dx.doi.org/10.1681/ASN.2009070719; PMID: 20133479
- Pietilä I, Ellwanger K, Railo A, Jokela T, Barrantes IdelB, Shan J, et al. Secreted Wnt antagonist Dickkopf-1 controls kidney papilla development coordinated by Wnt-7b signalling. Dev Biol 2011; 353:50 - 60; http://dx.doi.org/10.1016/j.ydbio.2011.02.019; PMID: 21354128
- Cha JH, Kim YH, Jung JY, Han KH, Madsen KM, Kim J. Cell proliferation in the loop of henle in the developing rat kidney. J Am Soc Nephrol 2001; 12:1410 - 21; PMID: 11423570
- Neiss WF. Histogenesis of the loop of Henle in the rat kidney. Anat Embryol (Berl) 1982; 164:315 - 30; http://dx.doi.org/10.1007/BF00315754; PMID: 7137581
- Stubbe J, Madsen K, Nielsen FT, Skøtt O, Jensen BL. Glucocorticoid impairs growth of kidney outer medulla and accelerates loop of Henle differentiation and urinary concentrating capacity in rat kidney development. Am J Physiol Renal Physiol 2006; 291:F812 - 22; http://dx.doi.org/10.1152/ajprenal.00477.2005; PMID: 16638911
- Reggiani L, Raciti D, Airik R, Kispert A, Brändli AW. The prepattern transcription factor Irx3 directs nephron segment identity. Genes Dev 2007; 21:2358 - 70; http://dx.doi.org/10.1101/gad.450707; PMID: 17875669
- Mittaz L, Ricardo S, Martinez G, Kola I, Kelly DJ, Little MH, et al. Neonatal calyceal dilation and renal fibrosis resulting from loss of Adamts-1 in mouse kidney is due to a developmental dysgenesis. Nephrol Dial Transplant 2005; 20:419 - 23; http://dx.doi.org/10.1093/ndt/gfh603; PMID: 15615810
- Nakai S, Sugitani Y, Sato H, Ito S, Miura Y, Ogawa M, et al. Crucial roles of Brn1 in distal tubule formation and function in mouse kidney. Development 2003; 130:4751 - 9; http://dx.doi.org/10.1242/dev.00666; PMID: 12925600
- Pannabecker TL, Dantzler WH. Three-dimensional architecture of inner medullary vasa recta. Am J Physiol Renal Physiol 2006; 290:F1355 - 66; http://dx.doi.org/10.1152/ajprenal.00481.2005; PMID: 16380456
- Speller AM, Moffat DB. Tubulo-vascular relationships in the developing kidney. J Anat 1977; 123:487 - 500; PMID: 858697
- Crivellato E. The role of angiogenic growth factors in organogenesis. Int J Dev Biol 2011; 55:365 - 75; http://dx.doi.org/10.1387/ijdb.103214ec; PMID: 21858761
- Oliverio MI, Kim HS, Ito M, Le T, Audoly L, Best CF, et al. Reduced growth, abnormal kidney structure, and type 2 (AT2) angiotensin receptor-mediated blood pressure regulation in mice lacking both AT1A and AT1B receptors for angiotensin II. Proc Natl Acad Sci U S A 1998; 95:15496 - 501; http://dx.doi.org/10.1073/pnas.95.26.15496; PMID: 9860997
- Tsuchida S, Matsusaka T, Chen X, Okubo S, Niimura F, Nishimura H, et al. Murine double nullizygotes of the angiotensin type 1A and 1B receptor genes duplicate severe abnormal phenotypes of angiotensinogen nullizygotes. J Clin Invest 1998; 101:755 - 60; http://dx.doi.org/10.1172/JCI1899; PMID: 9466969
- Madsen K, Marcussen N, Pedersen M, Kjaersgaard G, Facemire C, Coffman TM, et al. Angiotensin II promotes development of the renal microcirculation through AT1 receptors. J Am Soc Nephrol 2010; 21:448 - 59; http://dx.doi.org/10.1681/ASN.2009010045; PMID: 20056745
- Daïkha-Dahmane F, Levy-Beff E, Jugie M, Lenclen R. Foetal kidney maldevelopment in maternal use of angiotensin II type I receptor antagonists. Pediatr Nephrol 2006; 21:729 - 32; http://dx.doi.org/10.1007/s00467-006-0070-1; PMID: 16565869
- Matsui T, Kanai-Azuma M, Hara K, Matoba S, Hiramatsu R, Kawakami H, et al. Redundant roles of Sox17 and Sox18 in postnatal angiogenesis in mice. J Cell Sci 2006; 119:3513 - 26; http://dx.doi.org/10.1242/jcs.03081; PMID: 16895970
- Gribouval O, Gonzales M, Neuhaus T, Aziza J, Bieth E, Laurent N, et al. Mutations in genes in the renin-angiotensin system are associated with autosomal recessive renal tubular dysgenesis. Nat Genet 2005; 37:964 - 8; http://dx.doi.org/10.1038/ng1623; PMID: 16116425
- Gimelli S, Caridi G, Beri S, McCracken K, Bocciardi R, Zordan P, et al. Mutations in SOX17 are associated with congenital anomalies of the kidney and the urinary tract. Hum Mutat 2010; 31:1352 - 9; http://dx.doi.org/10.1002/humu.21378; PMID: 20960469
- Sparrow DB, Boyle SC, Sams RS, Mazuruk B, Zhang L, Moeckel GW, et al. Placental insufficiency associated with loss of Cited1 causes renal medullary dysplasia. J Am Soc Nephrol 2009; 20:777 - 86; http://dx.doi.org/10.1681/ASN.2008050547; PMID: 19297558
- Freeburg PB, Robert B, St John PL, Abrahamson DR. Podocyte expression of hypoxia-inducible factor (HIF)-1 and HIF-2 during glomerular development. J Am Soc Nephrol 2003; 14:927 - 38; http://dx.doi.org/10.1097/01.ASN.0000059308.82322.4F; PMID: 12660327
- Freeburg PB, Abrahamson DR. Hypoxia-inducible factors and kidney vascular development. J Am Soc Nephrol 2003; 14:2723 - 30; http://dx.doi.org/10.1097/01.ASN.0000092794.37534.01; PMID: 14569081
- Manotham K, Tanaka T, Ohse T, Kojima I, Miyata T, Inagi R, et al. A biologic role of HIF-1 in the renal medulla. Kidney Int 2005; 67:1428 - 39; http://dx.doi.org/10.1111/j.1523-1755.2005.00220.x; PMID: 15780095
- Shah SR, Esni F, Jakub A, Paredes J, Lath N, Malek M, et al. Embryonic mouse blood flow and oxygen correlate with early pancreatic differentiation. Dev Biol 2011; 349:342 - 9; http://dx.doi.org/10.1016/j.ydbio.2010.10.033; PMID: 21050843
- Jeansson M, Gawlik A, Anderson G, Li C, Kerjaschki D, Henkelman M, et al. Angiopoietin-1 is essential in mouse vasculature during development and in response to injury. J Clin Invest 2011; 121:2278 - 89; http://dx.doi.org/10.1172/JCI46322; PMID: 21606590
- Klein G, Ekblom P. Extracellular matrix composition during kidney development. Contrib Nephrol 1990; 80:17 - 31; PMID: 2282818
- Alcorn D, Maric C, McCausland J. Development of the renal interstitium. Pediatr Nephrol 1999; 13:347 - 54; http://dx.doi.org/10.1007/s004670050624; PMID: 10454789
- Hatini V, Huh SO, Herzlinger D, Soares VC, Lai E. Essential role of stromal mesenchyme in kidney morphogenesis revealed by targeted disruption of Winged Helix transcription factor BF-2. Genes Dev 1996; 10:1467 - 78; http://dx.doi.org/10.1101/gad.10.12.1467; PMID: 8666231
- Mason IJ, Fuller-Pace F, Smith R, Dickson C. FGF-7 (keratinocyte growth factor) expression during mouse development suggests roles in myogenesis, forebrain regionalisation and epithelial-mesenchymal interactions. Mech Dev 1994; 45:15 - 30; http://dx.doi.org/10.1016/0925-4773(94)90050-7; PMID: 8186145
- Quaggin SE, Schwartz L, Cui S, Igarashi P, Deimling J, Post M, et al. The basic-helix-loop-helix protein pod1 is critically important for kidney and lung organogenesis. Development 1999; 126:5771 - 83; PMID: 10572052
- Miyazaki Y, Oshima K, Fogo A, Hogan BL, Ichikawa I. Bone morphogenetic protein 4 regulates the budding site and elongation of the mouse ureter. J Clin Invest 2000; 105:863 - 73; http://dx.doi.org/10.1172/JCI8256; PMID: 10749566
- Humphreys BD, Lin SL, Kobayashi A, Hudson TE, Nowlin BT, Bonventre JV, et al. Fate tracing reveals the pericyte and not epithelial origin of myofibroblasts in kidney fibrosis. Am J Pathol 2010; 176:85 - 97; http://dx.doi.org/10.2353/ajpath.2010.090517; PMID: 20008127
- Bard JB. The metanephros. In, Vice P, Woolf A, Bard J: The kidney: from normal development to congenital disease. London, Academic Press 2003; 25: 181-193.
- Schmidt-Ott KM, Chen X, Paragas N, Levinson RS, Mendelsohn CL, Barasch J. c-kit delineates a distinct domain of progenitors in the developing kidney. Dev Biol 2006; 299:238 - 49; http://dx.doi.org/10.1016/j.ydbio.2006.07.026; PMID: 16942767
- Rosselot C, Spraggon L, Chia I, Batourina E, Riccio P, Lu B, et al. Non-cell-autonomous retinoid signaling is crucial for renal development. Development 2010; 137:283 - 92; http://dx.doi.org/10.1242/dev.040287; PMID: 20040494
- Qiao J, Uzzo R, Obara-Ishihara T, Degenstein L, Fuchs E, Herzlinger D. FGF-7 modulates ureteric bud growth and nephron number in the developing kidney. Development 1999; 126:547 - 54; PMID: 9876183
- Mendelsohn C, Batourina E, Fung S, Gilbert T, Dodd J. Stromal cells mediate retinoid-dependent functions essential for renal development. Development 1999; 126:1139 - 48; PMID: 10021334
- Cui S, Schwartz L, Quaggin SE. Pod1 is required in stromal cells for glomerulogenesis. Dev Dyn 2003; 226:512 - 22; http://dx.doi.org/10.1002/dvdy.10244; PMID: 12619136
- Zhang P, Liégeois NJ, Wong C, Finegold M, Hou H, Thompson JC, et al. Altered cell differentiation and proliferation in mice lacking p57KIP2 indicates a role in Beckwith-Wiedemann syndrome. Nature 1997; 387:151 - 8; http://dx.doi.org/10.1038/387151a0; PMID: 9144284
- Schnabel CA, Godin RE, Cleary ML. Pbx1 regulates nephrogenesis and ureteric branching in the developing kidney. Dev Biol 2003; 254:262 - 76; http://dx.doi.org/10.1016/S0012-1606(02)00038-6; PMID: 12591246
- Yosypiv IV. Vasoactive Factors and Blood Pressure in Children. In: Pediatric hypertension, 3rd edition. Edited by: J. T. Flynn. Springer, 23-40, 2010.
- Pupilli C, Gomez RA, Tuttle JB, Peach MJ, Carey RM. Spatial association of renin-containing cells and nerve fibers in developing rat kidney. Pediatr Nephrol 1991; 5:690 - 5; http://dx.doi.org/10.1007/BF00857873; PMID: 1768580
- Carmeliet P, Tessier-Lavigne M. Common mechanisms of nerve and blood vessel wiring. Nature 2005; 436:193 - 200; http://dx.doi.org/10.1038/nature03875; PMID: 16015319
- Tiniakos D, Anagnostou V, Stavrakis S, Karandrea D, Agapitos E, Kittas C. Ontogeny of intrinsic innervation in the human kidney. Anat Embryol (Berl) 2004; 209:41 - 7; http://dx.doi.org/10.1007/s00429-004-0420-3; PMID: 15480775
- Eppel GA, Luff SE, Denton KM, Evans RG. Type 1 neuropeptide Y receptors and alpha1-adrenoceptors in the neural control of regional renal perfusion. Am J Physiol Regul Integr Comp Physiol 2006; 290:R331 - 40; http://dx.doi.org/10.1152/ajpregu.00317.2005; PMID: 16195497
- Karavanov A, Sainio K, Palgi J, Saarma M, Saxen L, Sariola H. Neurotrophin 3 rescues neuronal precursors from apoptosis and promotes neuronal differentiation in the embryonic metanephric kidney. Proc Natl Acad Sci U S A 1995; 92:11279 - 83; http://dx.doi.org/10.1073/pnas.92.24.11279; PMID: 7479979
- Gendron L, Payet MD, Gallo-Payet N. The angiotensin type 2 receptor of angiotensin II and neuronal differentiation: from observations to mechanisms. J Mol Endocrinol 2003; 31:359 - 72; http://dx.doi.org/10.1677/jme.0.0310359; PMID: 14664700
- Song R, Spera M, Garrett C, El-Dahr SS, Yosypiv IV. Angiotensin II AT2 receptor regulates ureteric bud morphogenesis. Am J Physiol Renal Physiol 2010; 298:F807 - 17; http://dx.doi.org/10.1152/ajprenal.00147.2009; PMID: 20032120
- Kett MM, Denton KM. Renal programming: cause for concern?. Am J Physiol Regul Integr Comp Physiol 2011; 300:R791 - 803; http://dx.doi.org/10.1152/ajpregu.00791.2010; PMID: 21191002
- Chang CP, McDill BW, Neilson JR, Joist HE, Epstein JA, Crabtree GR, et al. Calcineurin is required in urinary tract mesenchyme for the development of the pyeloureteral peristaltic machinery. J Clin Invest 2004; 113:1051 - 8; PMID: 15057312
- Cain JE, Islam E, Haxho F, Blake J, Rosenblum ND. GLI3 repressor controls functional development of the mouse ureter. J Clin Invest 2011; 121:1199 - 206; http://dx.doi.org/10.1172/JCI45523; PMID: 21339645
- Miyazaki Y, Tsuchida S, Nishimura H, Pope JC 4th, Harris RC, McKanna JM, et al. Angiotensin induces the urinary peristaltic machinery during the perinatal period. J Clin Invest 1998; 102:1489 - 97; http://dx.doi.org/10.1172/JCI4401; PMID: 9788961
- Yosypiv IV, Schroeder M, El-Dahr SS. Angiotensin II type 1 receptor-EGF receptor cross-talk regulates ureteric bud branching morphogenesis. J Am Soc Nephrol 2006; 17:1005 - 14; http://dx.doi.org/10.1681/ASN.2005080803; PMID: 16495379
- Lacoste M, Cai Y, Guicharnaud L, Mounier F, Dumez Y, Bouvier R, et al. Renal tubular dysgenesis, a not uncommon autosomal recessive disorder leading to oligohydramnios: Role of the Renin-Angiotensin system. J Am Soc Nephrol 2006; 17:2253 - 63; http://dx.doi.org/10.1681/ASN.2005121303; PMID: 16790508
- Song R, Van Buren T, Yosypiv IV. Histone Deacetylases are Critical Regulators of the Renin-Angiotensin System During Ureteric Bud Branching Morphogenesis. Pediatric Research 2010; 67:573 - 8; http://dx.doi.org/10.1203/PDR.0b013e3181da477c; PMID: 20496471
- Nagata M, Tanimoto K, Fukamizu A, Kon Y, Sugiyama F, Yagami K, et al. Nephrogenesis and renovascular development in angiotensinogen-deficient mice. Lab Invest 1996; 75:745 - 53; PMID: 8941219
- Esther CR Jr, Howard TE, Marino EM, Goddard JM, Capecchi MR, Bernstein KE. Mice lacking angiotensin-converting enzyme have low blood pressure, renal pathology, and reduced male fertility. Lab Invest 1996; 74:953 - 65; PMID: 8642790
- Nishimura H, Yerkes E, Hohenfellner K, Miyazaki Y, Ma J, Hunley TE, et al. Role of the angiotensin type 2 receptor gene in congenital anomalies of the kidney and urinary tract, CAKUT, of mice and men. Mol Cell 1999; 3:1 - 10; http://dx.doi.org/10.1016/S1097-2765(00)80169-0; PMID: 10024874