Abstract
Stem cells in vivo are housed within a functional microenvironment termed the “stem cell niche.” As the niche components can modulate stem cell behaviors like proliferation, migration and differentiation, evaluating these components would be important to determine the most optimal platform for their maintenance or differentiation. In this review, we have discussed methods and technologies that have aided in the development of high throughput screening assays for stem cell research, including enabling technologies such as the well-established multiwell/microwell plates and robotic spotting, and emerging technologies like microfluidics, micro-contact printing and lithography. We also discuss the studies that utilized high throughput screening platform to investigate stem cell response to extracellular matrix, topography, biomaterials and stiffness gradients in the stem cell niche. The combination of the aforementioned techniques could lay the foundation for new perspectives in further development of high throughput technology and stem cell research.
Introduction
Stem cells have the ability to self-renew and differentiate into progenitors or differentiated cells. The factors affecting the stem cell niche include neighboring stem cells or differentiated cells, extracellular matrix, growth factors, and cytokines.Citation1 Recent works have shown that physical factors like topography (architecture, geometry and size), stiffness and shear stress can also affect the stem cell behavior and its fate to a particular lineage (refer to the review by Yim and SheetzCitation2). To have a better understanding of factors influencing and mimicking the stem cell niche in the in vitro tissue engineered platform, it would be valuable to screen several factors simultaneously. Researchers have devised new techniques that could analyze the collective complexity of both physicalCitation3,Citation4 and biochemical cues for stem cell expansion or differentiation on the same platform. Currently, most studies focus on evaluating 1–2 factors at a given time, which is expensive, labor intensive and time consuming. This unmet need motivated us to review the current status of high throughput technologies for the study of stem cell behavior. The history of high throughput screening dates back to 1960s when the first microtiter plate was developed.Citation5 This development led to the rapid and reliable screening of soluble molecules such as drugs, small molecule inhibitors, siRNA that affect stem cell expansion and differentiation. Conventional strategies like robotic spotting could be combined with lithography to develop chips having an array of combinatorial factors (biochemical and biophysical cues) for stem cell based high throughput screening. These technologies enabled the development of complex in vitro stem cell niche by the combination of several critical components like biomaterial, topography and ECM. This review will first discuss the various technologies that have the potential for designing arrays of ECM, biomaterials, topography and stiffness. The repository of intriguing results will then be discussed with a focus on stem cell behaviors like adhesion, proliferation and differentiation in the second section.
Technologies with Potential for the Development and Application of High Throughput Screening
Microwell plates and robotic spotting are examples of established technologies for high-throughput applications. They are commercially available and can be customizable according to the end user’s requirements. New bioengineering techniques like lithography and printing have previously been successfully used for applications in the field of microelectronics. Both the commercially available and the newer techniques could potentially be applied for high throughput studies.
Multiwell/microwell plate
The multiwell plate also known as “microtiter plate” was first manufactured in the 1960s by Dynatech, and the plate consisted of ten test tubes arranged in ten rows.Citation5 Microwell plates were made of plastic or polymers. The design of the multiwell plate has evolved over the years to have a variety of options: flat or convex bottom plate, with or without surface treatment for tissue culture or protein adsorption, black, white, colored or transparent multiwell plates and so on. Currently, more than 20 companies manufacture microwell plates for various high throughput applications. The standard and most commonly used format for multiwell plates is the 96- and 384-well plate format. An increasing availability of plate readers, robotic dispensers, multi-channel electronic pipettes and other supporting devices further enhance and assist the high-throughput experiments with the microwell plates.Citation6 In addition to the commercially available microwell plates, we have reviewed a few recent works that have incorporated new design, biomaterials or technique to fabricate microwells for high throughput stem cell studies.
Application of new designs to conventional multiwell plates
As a breakthrough of the conventional microplate design, Lindstrom et al. made slight modifications of the microwell design to include sloped walls which enabled uniform seeding of single cells (). Low volumes (500 nL) were sufficient to fill the well diameter (1360 μm) and have enough number of cells for stem cell analysis.Citation7,Citation8 The microwell chip was made on a thin glass slide and hence supported high quality imaging. A more recent study by Wen and co-authors aimed at miniaturising bioreactors into a microwell plate. A 24-well microwell plate was designed with static mixers and gas permeable lids for reduced evaporation for high throughput cell culture. These well plates gave results equivalent to spinner flask culture or bioreactor, so it was similar to having 24 bioreactors in a multiwell plate.Citation9 Such a system is inexpensive and does not require complex equipment or robotics.
Figure 1. Photographs of the microplate showing sloped walls for efficient cell seeding (Courtesy: Lindstrom et al., 2008).Citation7
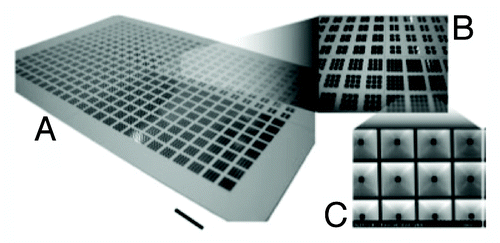
Novel biomaterials for multiwell plates
New materials for studying cell interactions with biomaterials are being considered to improve the in vitro stem cell niche. Microwells were also fabricated using new materials like the polyethylene glycol (PEG)-based hydrogels as opposed to the conventional polystyrene for cell based applications. With the advent of lithography techniques, polydimethylsiloxane (PDMS) mold were easy to fabricate by soft lithography and used repeatedly as the master mold to transfer the microwell patterns of different shapes and sizes onto the biomaterial substrate. Moeller et al. patterned PEG based hydrogels using PDMS stamps and photo-crosslinkable curing agent.Citation10,Citation11 By extending this method of using PDMS mold to fabricate microwells, Tekin et al. imprinted microwells onto a temperature sensitive material, PNIPAAm (poly N-isopropyl acrylamide). This novel temperature sensitive multi-well array was hydrophilic and swollen at 24 °C while they became hydrophobic and shrunk at 37 °C.
New techniques to simplify the fabrication procedure
Fabrication of PDMS mold still requires expensive silica or glass master molds for synthesis of the microwell arrays. In an interestingly simple and cost efficient technique devised by Liu et al., 10–20 μm polystyrene beads were assembled onto a glass slide. The beads were melted onto the glass slide, which was then used as the master mold to fabricate PDMS containing rounded microwells. The advantages of this method include inexpensive equipment and can be performed easily in a cost-efficient manner with common laboratory equipment.Citation12
Here, we have looked briefly into the history of microplate design and how novel microwells were designed. Robotic spotters are the next most commonly used equipment and well-established method for generating chips containing small volumes of biochemical components laid out in many permutations and combinations.
Printing-based technologies for high-throughput screening
The printing methods can be divided into contact and non-contact printing methods, depending on the physical contact between substrate and the printing device. Roth et al. developed a cell printing technology by using a commercially available desktop printer.Citation13 Patterns were designed by Microsoft PowerPoint software and collagen was printed on the cell repellent substrate prior to cell seeding with a modified inkjet printer. Attachment of dorsal root ganglia neurons and smooth muscle cells were observed at different time points.
Contact printing methods include robotic spotting, which is one of the most established fabrication technologies employed in high-throughput screening, and other direct contact printing techniques using easily available printers, which is cost effective as compared with the specialized robotic spotters. However, robotic spotters have the advantages of precision and automation.
Electrodynamic jet printing
Another novel high resolution electrodynamic jet-printing (e-jet printing) method developed by Park et al., also has potential for high throughput applications ().Citation14 This method was based on the electrodynamically induced flow of fluid through microcapillary nozzles that enabled submicron patterning. Poellman et al. applied this method to print 5-μm spots of rabbit immunoglobulin-G and fibronectin on polyacrylamide hydrogel coated on a glass slide.Citation15 Voltage, substrate alignment, 5-axis stage and nozzle distance for printing were controlled by custom designed software. Cell adhesion and cytoskeletal structure were examined for mouse mesenchymal stem cells (MSCs) and rat MSCs grown on these patterned substrates.
Figure 2. The Printer set-up is illustrated. It consists of a gold-coated nozzle located above a substrate that rests on a grounded electrode. The substrate/electrode combination is mounted on a 5-axis stage for printing (Courtesy: Park et al., 2007).Citation14
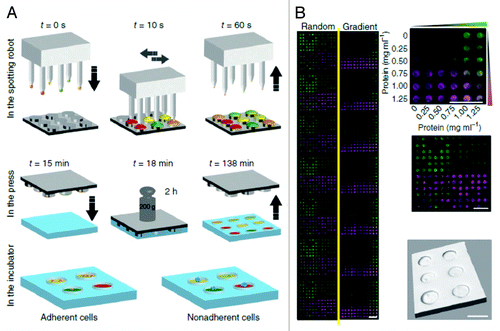
Pulsed laser-based printing technique
Another printing method is the Laser Induced Forward Transfer (LIFT), in which pulsed laser was used to induce the transfer of material from a source film (spread onto an optically transparent quartz support) to a substrate in close proximity with the source film.Citation16 This printing method was demonstrated by Gaebel et al. to pattern human MSCs and endothelial cells for cardiac regeneration on polyester urethane urea cardiac patches.Citation17
Guillemot et al. explored the option of using an infrared pulsed laser (Biological Laser Printing, BioLP) for high throughput printing of human endothelial cells, sodium alginate and hydroxyapatite patterns in the nanoscale. Using this method, deposition of 5 to 7 live cells onto the hydrogel for further studies was possible.Citation18
Direct contact printing
While the above examples described non-contact printing methods, a direct contact printing process was used in an intriguing study by Frimat et al. for analyzing the neurite outgrowth.Citation19 PDMS was inked on a PDMS master mold by spin coating followed by microcontact printing onto glass or TCPS. A variety of patterns with different dimensions and geometries were printed to demonstrate the high potential and applicability of this method for stem cell-based assays. Refer to Ringeisen et al.Citation20 and Stratakis et al.Citation21 for more detailed review on the various printing methods.
Robotic spotting and its application for high throughput studies
High-throughput screening has the ability to scale up the number of tests samples, allowing the simultaneous analysis of various important parameters and components including biophysical factors, biochemical factors including small molecules, genes and pharmacological drugs. Among the various common techniques developed for in vitro high-throughput screening (reviewed in Barrett et al., 2003Citation22), robotic spotting remains as one of the most preferred and applied technology for manufacturing DNA, siRNA or protein microarrays. Most robotic spotted microarrays require the immobilization of biomolecules onto chemically modified surfaces. The pins of the robotic spotters are first dipped into a solution containing the compound of interest, before bringing it into contact with the substrate surface that is to be printed, to produce a desired number of nano-liter sized spots on this single surface in a spatially controlled manner. As robotic spotting technology was first conceived in the 1990s,Citation23 advancements have made this technology advantageous in terms of the high efficiency by multiplexing the analysis of different test parameters, ease of availability, the low cost due to the nano-liter dispensing of test reagents and the versatility to spot a large variety of biomolecules, compounds and, more recently, even stem cells. The main drawbacks of this technology are the expensive instrumentation, the need for high throughput advanced image or data processing techniques and the variability in spot sizes.
The advent of robotic spotting spurred the improvement of this platform for many applications. In addition to conventional small molecule screening and toxicology analysis,Citation24-Citation26 the technology has also been harnessed to include target protein screeningCitation24,Citation27 and to allow distinct multiple parallel infections in different types of cells for mimicking a particular genetic disease.Citation28
Protein spotting
Modifications to robotic spotting technology for high throughput stem cell studies were made mainly through the use of novel materials and protein spotting techniques. A study by Gobaa et al. demonstrated the development of a robotic spotting technique to combine a hydrogel microwell arrays with ECM protein spotting to functionalize these new material surfaces.Citation29 Partially cross-linked PEG hydrogels were first attached to the bottom of a 4-well culture plate. Using conventional soft lithography, silicon PDMS micropillar stamps were first fabricated to be used for imprinting biomolecules on the inert PEG hydrogel substrates. The biomolecules of choice were then robotically spotted using an existing DNA spotter (Genetix QArray Mini) onto these micropillars, where the micropillar layout was designed to match exactly the spotting layout of this current spotter. When the micropillar stamps were brought into contact with the hydrogel substrates, it enabled the simultaneous embossing of the hydrogel as well as the tethering of biomolecules from the silicon stamp to the bottom of embossed microwells ().
Figure 3. Schematic diagram showing the fabrication of stem cell niche microarray using a combination of photolithography and robotic spotting developed by Gobaa et al. (Courtesy: Gobaa et al., 2011).Citation29
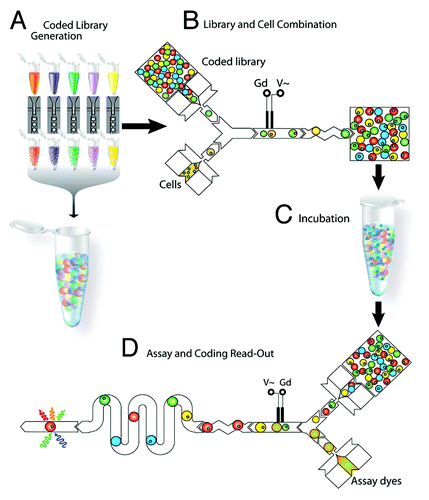
The use of robotic spotting technology was generally incompatible for ECM protein deposition until Flaim et al. adopted the technology using a different spotting buffer that allows for ECM protein spotting using a standard DNA spotter.Citation30 Using a custom fabricated acrylamide gel pad slide, a microarray with a combination of 32 different ECM proteins, including collagen I, III, IV, laminin and fibronectin with 8 replicates, was robotically printed onto the slide. This group optimized the printing buffer, microarray surface and deposition method for use with a contact style arrayer capable of depositing 150-μm spots, which could accommodate at least 20 cells, using 1–2 nL of ECM, greatly reducing the cost of reagents used.
The microengineered platform was used to probe different stem cell functions including the effect of cell–cell interactions, substrate elasticity and combinatorial protein microenvironments on adherent or non-adherent stem cells. The fabricated microwells used in the system also enabled the trapping of non-adherent cells in different wells of combinatorial protein tethered to the base of these structures. Although the effect of substrate stiffness was probed in the study, it was limited to a range of stiffness determined by the crosslinking density, where a larger range or a gradient of stiffness could prove to be more informative about this essential biophysical parameter. The authors also mindfully pointed out the need to temporally modulate the cellular microenvironment (i.e., changing the exposure of cells to various combinations of growth factors at several time points) and the possible paracrine effects from inter cellular communications because they were cultured in the same chamber. With the advancement of image and data processing techniques, coupling this novel platform with a high throughput image acquisition system will allow for a more efficient data mining.
Robotic spotting technology has enabled the high throughput screening of various biophysical and biochemical modulators of stem cell behavior. While the potential of robotic spotting is undoubtedly high to allow the systematic screening of stem cell niches parameters, it is still limited by the presence of inter cellular paracrine and autocrine signals that are present when cells are probed on the same microarray. While the presentation of ECM approach gives specific control during the early phases of cell attachment or signaling, the importance of this synthetically deposited protein conformation should be better characterized. How these deposited matrices are remodeled and its effect on eventual stem cell behavior remains important questions to be answered using such microarrays. As with every aspiring technology, robotic spotting still emerges as one of the most promising technologies for high throughput in vitro screening with specific applications to stem cells.
Microfluidic based chips
Microfluidics based systems are characterized by their small reaction volumes, short analysis time and precise control of flow parameters. PDMS base microfluidic devices are transparent thereby allowing the visualization and analysis using microscope.Citation31 Current microfluidics based research is focused on developing lab-on-a-chip devices using this technology, which could allow the study of stem cells under controlled environmental conditions in high throughput.Citation32 The microfluidic systems for high throughput applications are based on two main techniques, using channel arrays to generate several combinations of desired chemicals on a glass substrate or by optically coding drugs and cells into droplets (refer to ) for further screening.Citation34
Figure 4. The schematic of droplet generation is shown. (A) generation of drug library, (B) merge the drugs with cells, (C) incubation of the droplets, (D) analysis of the results (Courtesy: Brouzes et al., 2009).Citation33
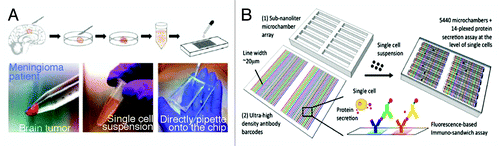
Droplet microfluidics
Brouzes and coworkers developed a microfluidic chip for high throughput drug screening of single cells using droplet microfluidic technology.Citation33 The chip consisted of three main parts: cell merge chip, incubator and analysis in an assay reader (). In a two-way channel, the drug was mixed with the cells to merge and form cell-containing droplets (700 pL, ~110 μm). These merged droplets were passed through a mixing module on the same chip to facilitate rapid and thorough mixing of drugs with cells. They were then collected in a syringe and incubated for 24 h before being injected into an assay plate to perform the viability test. This system has high reproducibility, robust performance and low standard deviations. The authors suggested the use of this chip for cell sorting,Citation35 DNA or siRNA screening. The cell survivability in droplets was for 2–3 days as compared with hours shown in most literature using the droplet microfluidic technology. The throughput could also be increased by multiplexing with multi-colored beadsCitation36 and could be potentially extended to study the stem cell or cancer cell viability in response to new drugs.
Serpentine channel-based microfluidics
The second most commonly used technique in microfluidics is the use of series of channels with programmed flow systems to control the release of chemicals used for studying stem cells. Secretome analysis of single cells using microfluidics was shown by Lu et al.Citation37 Antibody bar codes were created using serpentine microfluidic channels. Low volumes of 1 μl antibody solution were used to print up to 20 different antibodies in a single column to create a high density antibody bar code similar to the protein or DNA barcodes used in other studies.Citation38 Up to 30 repeats of the barcodes were created, and each barcode was separated by 1 mm (). The authors also used a microarray chamber onto which the antibody barcode is placed and incubated with single cell suspension of brain tumor cells for further analysis. Automated phase-contrast imaging and image analysis systems were used to analyze the protein secretion and to determine the cell population heterogeneity. Proteins secreted were detected by biotinylated detection antibodies followed by incubation with fluorescently-tagged streptavidin. The drawback of this system is that a very high starting concentration of cell suspension is needed to get at least 1000 wells with single cells. However, it can help to identify the heterogeneity of a populationCitation39 and also to screen for factors produced in single cells vs. cells in clusters.
Figure 5. The illustration shows a high-density antibody bar code array chip and the process of isolating primary tumor cells from patients for further secretomic analysis on the antibody arrays (Courtesy: Lu et al., 2013).Citation37
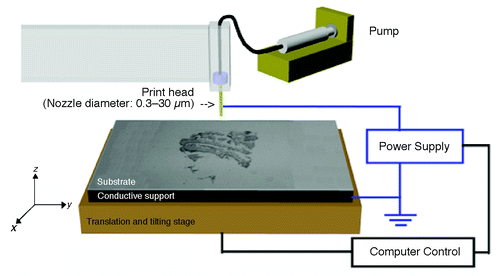
Microfluidics holds great potential for high throughput analysis of single cell or cell co-culture and their response to drugs at small volumes. These microfluidic lab-on-a-chip devices require a diligent design of the device and sophisticated, expensive equipment to control the fluid flow. However, the advantages of microfluidics make it a versatile technology to study stem cell behavior in a high throughput manner, with promises for commercialisation.
Two-dimensional gradient of polyelectrolyte multilayer to mimic the extracellular matrix
Studies have shown that ECM is an important component of the stem cell niche that can modulate the behavior of stem cells. Based on the need to recreate the stem cell niche in vitro for efficient generation of tissue engineered implants, a number of research is focused on simulating the ECM properties using a layer-by-layer combination of polyelectrolytes to form a two-dimensional combinational polyelectrolyte multilayer (PEM).Citation40 Water-soluble polymers could be used to fabricate many layers using PEM technique. In addition, every layer can have a tuneable internal architecture and density. The two-dimensional combinations of PEMs provide various ECM conditions with different surface energy, modulus and surface charge. This PEM was used for high throughput cell survival and proliferation assays of human embryonic kidney 293 (HEK293) cells and embryonic spinal commissural neurons.
Lithography-based technologies for high-throughput screening
Micro-electro-mechanical systems (MEMS) also known as micromachines is a technology of fabrication of devices, which involves the process of lithography and etching. The MEMS-based microfabrication has been used in enormous biological and medical applications. In the development of high throughput test for stem cell research, a number of substrates or arrays could be created using individual or combination of the micro-mechanical or microelectronic components to study cellular behavior (refer to the review by Ingham and van Hylckama VliegCitation41). In a recent study, Chen et al. demonstrated the use of MEMS based microwell array on a glass substrate for high throughput screening of HeLa cell’s transfection efficiency.Citation42 Agudelo et al. combined lithography with microfluidic technology to fabricate the TipChip.Citation43 This chip contained serially arranged microchannels for the study of cell response to chemical gradients, topographical features and directional triggers.
Photolithography techniques to design in vitro stem cell niche
Lithography is a common process used to recapitulate the topography and architecture of the stem cell niche by micro- or nano-patterning biocompatible substrates. With the advanced development of MEMS technology, different variations of lithography, such as photolithography, nanoimprinted lithography or soft lithography, provide fabrication process that suit the different needs of biological studies. The processes can also be combined to create complex structures. For example, Park et al. used a novel combination of photolithography and PDMS membrane to create a perforated mask with microwells. This thin PDMS membrane was pressed onto a perforated substrate using air pressure to create a complex mix of concave and convex microstructures.Citation44 The height or depth was adjustable by varying the pressure used to create the vacuum. These structures were seeded either with murine fibroblasts or human mesenchymal stem cells to study their response to the geometry. This system could be useful for various applications including stem cell differentiation, understanding the mechanobiology of their behavior.
Soft-lithography
Another lithographic process, soft-lithography, was used by Khetani et al. to fabricate reusable PDMS stencil membranes, which were placed at the bottom of each well in a 24-well plate.Citation45 The PDMS stencil was then sealed to the well plate with gentle pressure (). The exposed tissue culture polystyrene (TCPS) surface of the wells was coated with different concentrations of fluorescently labeled collagen-I to form micropatterned arrays. Co-culture of human or rat hepatocytes, which were seeded onto the collagen-I islands, and fibroblasts, which were seeded between the collagen-I islands, could be performed using this array. It was successfully used to screen the susceptibility of cells to hepatotoxins, metabolism and secretion of specific products in this hepatocyte-fibroblast co-culture for several weeks.
Figure 6. Soft lithographic process to fabricate a multiwell format is shown. Polydimethylsiloxane (PDMS) consisting of membranes with through-holes were placed at the bottom of each followed by incubation with extracellular matrix protein (ECM). The stencil is then peeled off leaving micropatterned ECM protein on the substrate (Courtesy: Khetani et al., 2008).Citation45
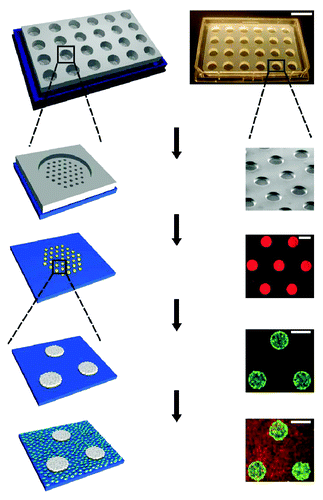
The extracellular microenvironment comprises of a reservoir of sub-micron topographical architecture. An increasing number of studies showed that when the nanotopography was replicated in synthetic substrates, the substrate topography could modulate cell behaviors. Thus, topography would be one of the important components of the stem cell niche to be investigated. (The topic would be discussed in a latter section. Refer to the review by Teo et al.Citation46). Most of the microfabrication techniques either involve expensive processes or mold. A more efficient and high throughput method of studying the stem cell-topography interaction will be highly desired; however, only a few high throughput topography arrays have been developed to date.
Markert et al. and Unadkat et al. designed the Bio surface structure arraysCitation47 (BSSA containing 169 patterns) and TopoChipCitation48 (housing 2176 distinct topographical units), respectively, using standard lithographic processes. The BSSA comprised of combination of micro-pillars of different shapes and spacing. In the TopoChip, Unadkat and coworkers used mathematical algorithms as a design tool, to design arrays of patterns onto a single chip with distinct combinations on each field. Nonetheless, due to the limitation of the lithographic process, the topographical features could only be at the same height on the same chip. Also, the size of the patterns fabricated was in the micron range.
Hybrid techniques combining lithography and etching
The limitations posed by lithography techniques include challenges in micro and nano-sized fabrication of topographical patterns especially the combination of structures to derive 3-dimensional or curved structures. Methods like electron-beam lithography could be coupled with optical and isotropic etching for fabricating curved features in the nano-scale dimensions. But combining several techniques to produce an array of topographies on the same chip is challenging.
In order to overcome these limitations, our group designed the Multiarchitecture (MARC) chip using a two-stage method: (1) patterning of topographies by nano-imprint lithography (NIL) and (2) assembly of topographies onto a single chip.Citation49,Citation50 Thermal NIL was used to fabricate individual topographies onto thermoplastic polycarbonate (PC) sheet using silicon master molds, which were fabricated by photo- or e-beam lithography. Parts of the imprinted PC sheet were cut into “fields” of 2 mm × 2 mm. In the second stage, these distinct topographies were assembled onto a single chip in a customized layout. This two-step fabrication enabled the inclusion of complex hierarchical structures and lens topographies onto the same topography chip and allowed the easy replication of these structures onto PDMS using soft lithography or onto TCPS using heat embossing. We could easily customize the chip in terms of geometry, height, size of the topography and arrangement and size of the fields on the MARC chip. For more detailed reviews on photolithography techniques for study of stem cell behavior, refer to the article by Melchels et al.Citation51
The enabling and emerging technologies based on microwells, robotic spotters, microfluidics and lithography holds promise in identification of the critical niche components and their incorporation into high throughput assays for stem cell based studies. However, there is scope for more development in each of these fields.
High Throughput Screening of Factors Affecting Stem Cell Interaction with Extracellular Environment
The term “stem cell niche” refers to the functionally specific microenvironment of stem cells.Citation52 Spatiotemporal interaction of the stem cells with their niche microenvironment is essential to maintain a balance between stem cell quiescence and activity.Citation53 It is indeed challenging to identify the right combination of factors that affect the stem cell niche. Previous reports have mentioned some of the factors affecting the stem cell niche include the surrounding cells (differentiated somatic cells, stem or progenitor cells), growth factors or cytokines (fibroblast growth factor, epidermal growth factor) and adhesion molecules (cadherin, cell adhesion molecules). The development of sophisticated and precise tools to analyze, measure and evaluate stem cell behavior has resulted in the identification of other niche modifying factors—physical factors like stress, stiffness, geometry, shape and size. Incorporating several of these identified factors into a biomaterial substrate to create the ideal platform for their expansion and differentiation in vitro adds another dimension of complexity. Having reviewed the commercially available technologies in the previous section, we will review the use of these methods to recreate a stem cell niche for the high throughput study of stem cell behavior.
ECM arrays
Stem cell behaviors in response to various extracellular molecules have been studied in a high throughput fashion using ECM arrays (). One such array fabricated by Ceriotti et al. was used to study the effect of ECM on adhesion of human umbilical cord blood neural stem cells (HUCB-NSCs).Citation54,Citation55 Fibronectin, laminin, collagen I, III and V, bovine serum albumin (BSA) and poly-l-lysine (PLL) spots were made on these slides using a piezoelectric printing technology (sciFLEXARRAYER S3). Based on the number of adherent cells, collagen III was determined to be the least suitable while collagen V was the most suitable for cell adhesion. In the spots having a combination of laminin and collagen V, the cells tended to attach to the boundary of the spot while on fibronectin spots, the cells attached over the entire surface area. Cells did not adhere on BSA, which was a negative control, but actively attached on PLL. Unlike other arrays which did not support stem cell growth for extended periods, the HUCB-NSCs could be grown for 7 d. This is a robust platform for precise determination of the minimum ECM concentration required for stem cell attachment.
Several researches were conducted on hybrid arrays, which contained several combinations of ECM as well as growth factors (immobilized or soluble). Lin et al. fabricated the microenvironment array (MEarray) composed of ECM and signaling molecules (EGFR, P-cadherin, E-cadherin, SHH, TGFα, epimorphin, integrin β4, β1 or α6) to study the maintenance of human mammary progenitor derived cell line, D920.Citation56,Citation57 The cells showed a decreased BrdU incorporation when grown on laminin, which also helped to maintain the progenitors in a quiescent and growth supressed state. Laminin was also essential for the maintenance of mammary progenitor state while P-cadherin could induce differentiation to a myoepithelial cell phenotype.
Brafman et al. conducted a high throughput study to evaluate the role of ECM and growth factors on hESC proliferation and maintenance. Their results suggested that single ECM component was sufficient to support either hESC proliferation or maintenance but not both. This study identified that laminin and fibronectin were essential for proliferation of hESCs, while collagen I, laminin and fibronectin individually could aid in maintaining the pluripotency of hESCs.Citation58
In addition to the growth and pluripotency maintenance, ECM also plays an important role in the differentiation. Soen et al. studied the differentiation fate of primary human neural precursor cells in response to ECM and immobilized growth factors. The cells were immunostained for neuronal, Tuj1, and glial markers, GFAP, after 3 d of culture on the protein microenvironment array. Combination of notch ligand, jagged-1, rhDLL4 (recombinant human delta like ligand 4), TGFβ, CNTF (ciliary neurotrophic factor), BMP4 encouraged glial morphology while a combination of wnt3a and laminin had a neurogenic effect on the neural precursor cells.Citation59 Generally, growth factors are supplemented in the differentiation media in a soluble form but in this study ECM and growth factors were immobilized onto the substrate. This technique could have the advantage of sustained exposure to growth factors but also the disadvantage that the signaling molecules were not presented in the functional configuration to cells. It would be interesting to compare the effect of ECM in the presence of either soluble growth factors or immobilized growth factors simultaneously for stem cell differentiation.
Flaim et al. addressed the effect of ECM on stem cell differentiation in the presence of soluble cues in a study of the cardiac and early hepatic differentiation of mouse ESCs on ECM arrays. The readout was based on the expression of green fluorescent protein (GFP)-tagged myosin heavy chain, a marker of cardiac cells, or relative expression of β-galactosidase reporter fused to a fetal liver specific gene. The differentiation efficiency was decreased by wnt3A while BMP-4 and activin A promoted the cardiac differentiation.Citation60 Highest β-galactosidase expression was seen in cells grown on matrices containing collagen I, while the lowest signals were obtained from cells grown on matrices lacking fibronectin.Citation30 In this study, the ECM microarray was coupled with a laser scanning confocal microscope and a software that was previously used to analyze DNA microarrays, resulting in higher resolution of images and a 10-times faster analysis. It was possible to screen potential ECM molecules that were either essential for differentiation (fibronectin) or to increase the differentiation efficiency.
The above examples discussed the study of stem cell behavior like adhesion, proliferation, maintenance and differentiation in response to various ECM molecules in the presence or absence of growth factors. summarizes the key achievements of various groups using various technologies to develop ECM arrays for stem cell research. The huge library of results enable us to precisely determine the concentration of ECM to be used, and the most essential components of the niche for stem cell expansion or differentiation. The use of fluorescently tagged proteins to the protein of interest eliminates the need for immunostaining.
Table 1. Summary of ECM arrays developed for stem cell research
Biomaterial arrays
Though ECM is one of the most important components of the stem cell niche, the choice of biomaterials is important for the in vitro expansion and differentiation of stem cells and for the design of tissue engineering scaffolds or biomedical devices. The choice of biomaterials depends on few of the following factors such as biocompatibility, ease of fabrication, mechanical properties, non-toxicity or biodegradability. However, very few studies have shown the fabrication of biomaterial arrays that could be used to understand stem cell interaction with materials, for identification of the suitable materials to be used for a biomedical device.
Anderson et al. designed an efficient nanoliter scale system to study the attachment of human mesenchymal stem cells (hMSCs)61 and hESCs,62 using a modified fluid handling system to deposit 1728 or 3456 individual spots onto a polyhydroxyethylmethacrylate (pHEMA) coated slide. Only polyethylene glycol (PEG) could inhibit hMSC attachment while just 30% of polylactic-co-glycolic acid (PLGA) could reverse this condition.Citation61 This was one of the earliest studies showing an on-chip polymerisation of biomaterial library as high throughput screening of biomaterials supporting stem cell adhesion.
In another massive library of biomaterials created by Neuss and coworkers to study the maintenance of stem cell morphology, proliferation and viability, a wide range of polymers (alginate, collagen, fibrin, hyaluronic acid) and stem cells (hMSCs, human preadipocytes, human dental pulp stem cells, human endothelial progenitor cells, mouse MSCs, mouse ESCs) were used.Citation62 The viability and attachment to a particular substrate was found to be cell type dependent. Both the chemical property of the substrate and the topography of the material were shown to be important determinants of stem cell morphology. Multiplex assays like this study help to better understand the interaction of various stem cells with the biomaterial.
Human ESCs and induced pluripotent stem cells (iPSCs) are grown in clumps but a defined system for clonal growth of hPSCs is not very well studied. In a recent work by Mei et al., the structure-function relationship of hPSCs and biomaterial substrate was studied using a high throughput biomaterial array. The effect of material properties like wettability, surface chemistry and elastic modulus on clonal growth of hPSCs was investigated. This study showed that the surface roughness did not have any effect on colony formation of PSCs.Citation63 This study has paved a new direction increasing the scope and possibilities for pluripotent stem cell maintenance.
Derda et al. showed that with the addition of photolithography to robotic spotting, the size and shape of the spotted peptide arrays (18 different laminin derived peptides) could be precisely controlled.Citation64 Human ESC lines, H1 and H9 were tested for cell attachment and proliferation in the presence of mouse embryonic fibroblast (MEF) conditioned media after 7 d of culture on these arrays. It was inferred that the ligand must be presented in high density for the hESCs to adhere and proliferate into a colony. Using a similar technique, Nakajima et al., studied the attachment, proliferation and differentiation of NSCs on the biomaterial in the presence of ECM and growth factors.Citation65 Cells were attached to spots containing immobilized FN, LN and PEI-0.8 while very few cells adhered to spots with collagen I, collagen-IV and PLL. Moreover, even on the same kind of ECM, the proliferation varied depending on the growth factors that were co-immobilized. Epidermal growth factor (EGF) was identified as the most potent growth factor for maintenance of NSC independent of the underlying ECM. Distinct from previous studies, this group combined biomaterial array with ECM and growth factors thereby creating a complex stem cell niche environment in vitro for the study of neural stem cells.
The above discussed studies showed that it is possible to create a hybrid biomaterial + ECM/growth factor array to identify the most important components regulating a particular stem cell adhesion, proliferation, migration and differentiation. summarizes the key techniques used to evaluate the biomaterials for stem cell adhesion and proliferation. Automated imaging and analysis software could potentially increase the screening throughput of these hybrid biomaterial arrays.
Table 2. Summary of biomaterial arrays developed for stem cell research
Stiffness arrays
Stem cells are receptive and sensitive to the extracellular microenvironment. One important aspect of the stem cell niche is the matrix elasticity.Citation67 To understand the effects of both ECM proteins and substrate stiffness on stem cell behavior, Gobaa et al. fabricated a microengineered PEG based hydrogel microwell arrays with biologically relevant stiffness (shear moduli ranging from 1–50 kPa and elastic moduli of approximately 3–150 kPa assuming a Poisson ratio of 0.5) combined with different ECM proteins.Citation29 These microfabricated arrays were used for testing the effect of cell–cell interactions on adipogenic differentiation of adherent hMSCs, the effect of substrate elasticity on osteogenic differentiation of single MSCs and the effect of combinatorial protein microenvironments on the proliferation of non-adherent NSCs. By changing the PEG precursor concentrations, the PEG hydrogel cross linking density was modified, thus giving a range of different hydrogel stiffness with constant biochemical functionalization, allowing the effect of substrate stiffness to be decoupled from surface functionalization. When arrays of variable stiffness with eight different concentrations of FN9–10 (fibronectin fragment 9–10) were investigated for their effects on MSC osteogenic differentiation, it was observed that the stem cell differentiation was independent of FN9–10 concentration, while the increase in substrate elastic modulus increased MSC osteogenic differentiation (). This platform allows for the high throughput screening of biophysical and biochemical factors that regulates stem cell proliferation and lineage determination. It is however important to note that the softest gels used in this study were limited in the amount of protein to be tethered onto these substrate surfaces because of the dependence on free functional groups present on the termini of PEG macromers in these microarrays.
Figure 7. The combinatorial effect of substrate elasticity and cell adhesion on mesenchymal stem cell osteogenic differentiation. Different concentrations of FN9–10 (Fibronectin fragment 9–10) was tethered onto different hydrogel stiffness to fabricate a microarray to study the combinatorial effects by controlling both cell adhesion and substrate elastic modulus (Courtesy: Gobaa et al., 2011).Citation29
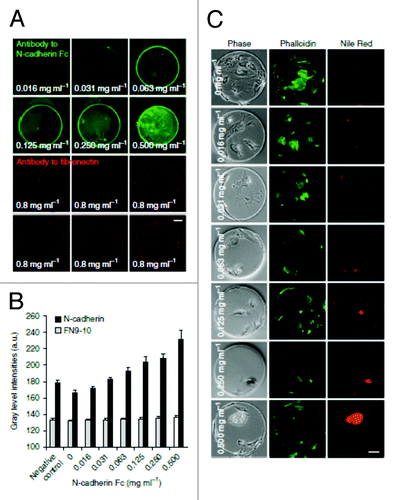
Another group attempted to develop a protocol for the rapid fabrication of 96 and 384 well platforms for the high throughput screening of cells on different elasticity.Citation68 Using polyacrylamide gels, substrates with Young’s modulus ranging from 0.3–55 kPa were fabricated and functionalized with collagen (). These arrays were then seeded with 7 different cell types (including both primary and immortalized cell lines) and fluorescently labeled for f-actin and nuclei before being automatically imaged to identify morphological transitions. In this study, proliferation and apoptosis assays were also performed. The authors were able to profile the cell growth as a function of substrate stiffness for various cell types using this stiffness array. To further demonstrate the versatility of their system, the authors then performed a combinatorial screen of small molecules with substrate stiffness and their effects on cell growth. Interestingly, the use of blebbistatin was able to improve reduced cell growth of lung fibroblast at low substrate stiffness.
Figure 8. Overview of the fabrication of different stiffness polyacrylamide gels on the bottom of 384 glass bottom well plates. To make a thin layer of polyacrylamide (PAA) gel, the glass array was inserted to sandwich uncrosslinked PAA solutions. Gels were then cross-linked using UV (UV) light and coated with collagen before cell seeding (Courtesy: Mih et al., 2011).Citation68
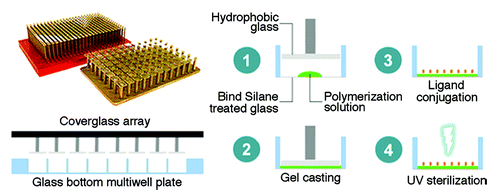
Although substrate elasticity is an important determinant of stem cell behavior, the number of high throughput methods applicable to elasticity appears to be limited. While the reviewed methods (the summary of key results is shown in ) allowed for a certain degree of stiffness screening, the range of stiffness was often limited due to the choice of polymers that must be complementary to the choice of technology and cell culture. Future advancements in fabrication methods for stiffness arrays will allow different materials with a stiffness gradient to be tested with regards to the control of stem cell behavior.
Table 3. Summary of stiffness arrays developed for stem cell research
Topography arrays
Topography is one of the key biophysical components of the stem cell niche.Citation69 Stem cell differentiation into various lineages including epithelial,Citation70 cardiomyocyte,Citation71 osteoblasts,Citation72 mesenchymalCitation73 and neuronalCitation74 was demonstrated by several groups using topographically patterned substrates. These studies highlighted the importance of substrate topography and the need to provide an artificial niche for stem cells that closely mimic the in vivo microenvironment of the desired cell types. The geometry, size and curvature of the substrate topography and architecture are important design parameters for a tissue engineering scaffold. Geometry could provide important signal to cell behavior. For example, grating like structures can provide contact guidance for stem cells thereby facilitating neuronal differentiation or cell elongation.Citation75-Citation77 Most of the studies were focused on a limited number of geometries and size, rather than a systematic screening through a wide range of shapes, size and arrangement, resulting in the current limitation in the research of stem cell-topography interaction. Here we discussed few studies that pioneered the fabrication of topography libraries using lithographic techniques to understand the independent and synergistic effects of topographies and biochemical cues on stem cell adhesion, proliferation, migration and differentiation.
BioSurface Structure Array (BSSA) developed by Lovmand et al. could incorporate both micron size range of pillars on a silicon wafer.Citation78 These pillars varied in shape (circle, square), placement (aligned, staggered), feature size (1, 2, 4, 6 μm), spacing distance (1, 2, 4, 6 μm) and feature height (0.6, 1.6, 2.4 μm). Markert et al. used the BSSA to screen for topographies promoting differentiation of mouse embryonic stem cells.Citation47 Their results showed that the “shark skin-like” arrangement of the topographies (round or square pillars) supported the highest levels of mESC differentiation independent of geometry and size. On the other hand, the submicron structures were evaluated to be the most suitable platforms for expansion and proliferation of undifferentiated ESCs. The disadvantage of this study was that the authors explored only pillar structures and the height of the topographies could not be varied on the same chip because of limitations in fabrication technology. Stiff substrates like tantalum were used for fabricating these patterns; thus, the technology does not allow flexibility in design of arrays with varying stiffness.
A systematic study conducted by Unadkat et al. resulted in the development of TopoChip consisting an array of 2176 different topographies.Citation48 In this study, they used the silicon master mold to imprint the patterns onto biocompatible substrates like PLA, showing the flexibility in design of topographies onto different biomaterial substrates. They made use of three basic structures—circles, triangles and thin rectangles, which were subjected to a mathematical algorithm to derive designs of complex shapes. Human MSCs were screened in a high- throughput manner for osteogenic differentiation, which was indicated by the alkaline phosphatase expression. The images were captured using an automated microscope for fast capture of all the topographies. This group incorporated the highest number of topographies onto a single chip but were still unable to fabricate nanoscale structures of varying heights. Also, both the BSSA and TopoChip did not include continuous structures like gratings for their design.
To overcome the limitation of fabricating a topography array containing different geometries of varying height, a two-step fabrication method was employed by our group to develop the Multi Architectural (MARC) chip. The MARC chip included topographies ranging from micro- to nano-dimensions with varying heights and complexity. Grounded on previous studies, a combination of anisotropic patterns (the gratingsCitation76,Citation77) and isotropic patterns (wellsCitation79 and pillarsCitation80) along with hierarchical structures was chosen to be assembled on the MARC chip. The latest generation of MARC chip has up to 49 different topographies including complex structures like concave and convex wells and hierarchical structures (dimples in between gratings). It is of imminent interest to study the effect of mechanical forces arising from the topographies on stem cell behavior like adhesion, proliferation, migration and differentiation. Using a single platform, MARC chip, it is possible to screen the effect of all three factors; geometry, size and arrangement of topographies on the differentiation fate or pluripotency maintenance of stem cells.
Ankam et al. studied the neural fate determination of hESCs in response to topographically patterned substrates grown on anisotropic patterns like gratings have a bias toward neuronal differentiation.Citation49 The grating size (micron vs. nano) also influenced the number of neurons (Tuj1 positive cells) positive for mature neuronal marker (microtubule associated protein 2, MAP2). Anisotropy topographies could improve maturation of the neurons and enable elongation and alignment of the neurites along the grating axis. Contrastingly, the hESCs grown on isotropic patterns like pillars and wells preferentially differentiated into astrocytes (GFAP positive population). We were able to compare the neuronal differentiation and extent of neurite elongation obtained by our method termed “direct differentiation” with the conventional methods of neuronal differentiation (through embryoid body formation). Despite previous studies showing that gratings could provide contact guidance to stem cells thereby directing their differentiation, this was the first study to conduct a systematic screening of different geometries and analysis for both glial and neuronal differentiation. Our results agreed with previous works that gratings enhanced the rate of neuronal differentiation. It was also one of the first work showing the selective expression of higher number of glial markers on isotropic patterns as compared with grating structures. This screen resulted in the identification of topographies that directed the neural fate of hESCs in a short duration (7 d) using minimal neuronal supplements (N2 and B27 supplements) ().
Figure 9. The schematic of neural differentiation on the Multi Architecture (MARC) chip by the direct (blue arrows) or conventional method (red arrows) is shown. The MARC chip has duplicates and also includes unpatterned surfaces as control (Courtesy: Ankam et al., 2013).Citation49
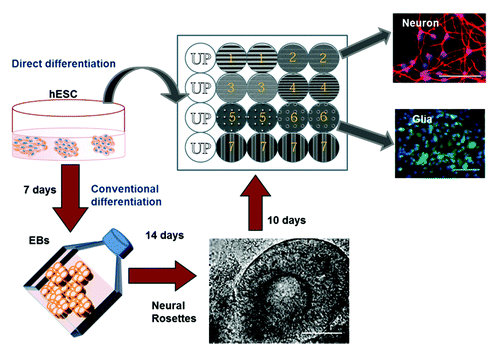
Similar work was performed by Moe et al. on the second generation MARC chip containing up to 18 different topographies to identify the optimal topographies suitable for neuronal differentiation of mouse neural progenitor cells (mNPCs).Citation50 Anisotropic patterns like the micron-sized gratings supported higher neuronal differentiation as observed from the higher neuron: astrocyte ratio and elongation of neurite along the grating axis, while the isotropic patterns like pillars and wells supported the glial differentiation (higher expression of GFAP), neurite branching and increase in area of the cell body.
These studies allow us to rethink the role of biophysical factors over biochemical factors in modulating the stem cell niche. Our group has successfully been able to design complex geometries of varying dimensions onto a single customizable chip. However, the coupling with mathematical algorithms to design new topographical structures, a faster image acquisition system to avoid photo bleaching and to improve test efficiency and a powerful software to analyze the vast set of data will still be necessary for further improvement. Summary of various topography chips employed for stem cell applications is shown in .
Table 4. Summary of Topography arrays developed for stem cell research
Conclusion
Stem cell fate and behaviors can be controlled by changing the properties of their substrate niche in vitro. Thus far, the development of sophisticated equipment to create multiwell plates and robotic spotters has aided the screening of cell behavior in response to biomolecules. Until recently, biochemical factors were the only components that were of interest. However, with the development of technologies like microfluidics and lithography, it allowed us to experiment and study the role of the mechanical signal via the biophysical extracellular components on stem cell behaviors. The development of arrays with various combinations of topography, microenvironment and stiffness of the underlying substrate has created a new dimension for analysis and screening of factors affecting the stem cell niche (). These high throughput methods are still in the early stages that must be complemented with fast imaging and analysis software. Researchers would be interested to investigate a few interesting questions in the near future: How will more sophisticated data analysis software improve the efficiency of high-throughput? How can we create an in vitro stem cell niche array that could incorporate multiple components such as ECM + topography + biomaterial + stiffness along with growth factors and signaling molecules, with the multi-variant data analysis to enable complex high throughput screening? Will these technologies be commercially available at a reasonable price? Should we expect more advanced and biocompatible materials to be used for these HTS chips? However, the design and development of next generation combinatorial arrays containing both biochemical factors (ECM, growth factors and ligand) and physical factors (stiffness, geometry and size) could give us a better understanding of the complex in vivo stem cell niche.
Figure 10. Overview of the various technologies available for identifying and evaluating the stem cell niche. Technologies like robotic spotting, printing, lithography and microfluidics could be combined with in vitro stem cell niche modulating components like extracellular matrix, biomaterials, topographies and materials of varying stiffness to analyze the behavior of somatic or stem cells (adhesion, proliferation, migration, differentiation, response to drugs) in a high throughput fashion.
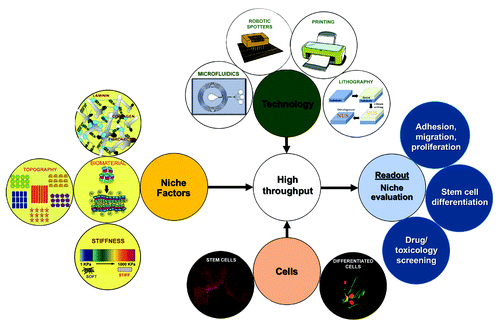
Abbreviations: | ||
BioLP | = | biological laser printing |
BMP | = | bone morphogenetic protein |
ECM | = | extracellular matrix |
EGF | = | epidermal growth factor |
FGF | = | fibroblast growth factor |
GFAP | = | glial fibrillary acidic protein |
HEK293 | = | human embryonic kidney 293 |
hESCs | = | human embryonic stem cells |
HTS | = | high throughput screening |
HUCB | = | human umbilical cord blood |
iPSCs | = | induced pluripotent stem cells |
LIFT | = | Laser Induced Forward Transfer |
MAP2 | = | microtubule associated protein |
MARC | = | multi-architecture |
MEF | = | mouse embryonic fibroblasts |
MEMS | = | micro electro mechanical systems |
MSCs | = | mesenchymal stem cells |
NSCs | = | neural stem cells |
PDMS | = | polydimethylsiloxane |
PEG | = | polyethylene glycol |
PEM | = | polyelectrolyte multilayer |
PNIPAAm | = | poly N isopropyl acrylamide |
SHH | = | sonic hedgehog |
TCPS | = | tissue culture polystyrene |
TGFβ | = | transforming growth factor β |
Tuj1 | = | beta tubulin III |
UV | = | ultraviolet |
Acknowledgments
This work was supported by the Singapore National Research foundation under its Competitive Research Program (NRF-CRP002-082) and Research Center of Excellence Mechanobiology Institute Singapore at the National University of Singapore. The authors would like to thank Chun Xi Wong for his help with illustrations. S.A. and B.K.K.T. thank the Ministry of Education and Department of Bioengineering and M.K. thanks Mechanobiology Institute Singapore for the scholarship.
Submitted
03/26/2013
Revised
05/19/2013
Accepted
06/15/2013
Disclosure of Potential Conflicts of Interest
No potential conflicts of interest were disclosed.
References
- De Souza N. In vitro niches. Nat Methods 2013; 10:37; http://dx.doi.org/10.1038/nmeth.2296
- Yim EK, Sheetz MP. Force-dependent cell signaling in stem cell differentiation. Stem Cell Res Ther 2012; 3:41; http://dx.doi.org/10.1186/scrt132; PMID: 23114057
- Nava MM, Raimondi MT, Pietrabissa R. Controlling self-renewal and differentiation of stem cells via mechanical cues. J Biomed Biotechnol 2012; 2012:797410; http://dx.doi.org/10.1155/2012/797410; PMID: 23091358
- Discher DE, Janmey P, Wang YL. Tissue cells feel and respond to the stiffness of their substrate. Science 2005; 310:1139 - 43; http://dx.doi.org/10.1126/science.1116995; PMID: 16293750
- Manns R. Microplate History. Presented at MipTec-ICAR'99, May 17–21, 1999, Montreux, Switzerland 1999; 5.
- Astle TW. Recollections of Early Microplate Automation. J Assoc Lab Autom 2000; 5:30 - 1; http://dx.doi.org/10.1016/S1535-5535(04)00103-0
- Lindström S, Larsson R, Svahn HA. Towards high-throughput single cell/clone cultivation and analysis. Electrophoresis 2008; 29:1219 - 27; http://dx.doi.org/10.1002/elps.200700536; PMID: 18288779
- Lindström S, Eriksson M, Vazin T, Sandberg J, Lundeberg J, Frisén J, et al. High-density microwell chip for culture and analysis of stem cells. PLoS One 2009; 4:e6997; http://dx.doi.org/10.1371/journal.pone.0006997; PMID: 19750008
- Wen Y, Zang R, Zhang XD, Yang ST. A 24-microwell plate with improved mixing and scalable performance for high throughput cell cultures. Process Biochem 2012; 47:612 - 8; http://dx.doi.org/10.1016/j.procbio.2011.12.023
- Moeller HC, Mian MK, Shrivastava S, Chung BG, Khademhosseini A. A microwell array system for stem cell culture. Biomaterials 2008; 29:752 - 63; http://dx.doi.org/10.1016/j.biomaterials.2007.10.030; PMID: 18001830
- Cordey M, Limacher M, Kobel S, Taylor V, Lutolf MP. Enhancing the reliability and throughput of neurosphere culture on hydrogel microwell arrays. Stem Cells 2008; 26:2586 - 94; http://dx.doi.org/10.1634/stemcells.2008-0498; PMID: 18669905
- Liu C, Liu J, Gao D, Ding M, Lin JM. Fabrication of microwell arrays based on two-dimensional ordered polystyrene microspheres for high-throughput single-cell analysis. Anal Chem 2010; 82:9418 - 24; http://dx.doi.org/10.1021/ac102094r; PMID: 20958018
- Roth EA, Xu T, Das M, Gregory C, Hickman JJ, Boland T. Inkjet printing for high-throughput cell patterning. Biomaterials 2004; 25:3707 - 15; http://dx.doi.org/10.1016/j.biomaterials.2003.10.052; PMID: 15020146
- Park JU, Hardy M, Kang SJ, Barton K, Adair K, Mukhopadhyay DK, et al. High-resolution electrohydrodynamic jet printing. Nat Mater 2007; 6:782 - 9; http://dx.doi.org/10.1038/nmat1974; PMID: 17676047
- Poellmann MJ, Barton KL, Mishra S, Johnson AJ. Patterned hydrogel substrates for cell culture with electrohydrodynamic jet printing. Macromol Biosci 2011; 11:1164 - 8; http://dx.doi.org/10.1002/mabi.201100004; PMID: 21656685
- Ringeisen BR, Kim H, Barron JA, Krizman DB, Chrisey DB, Jackman S, et al. Laser printing of pluripotent embryonal carcinoma cells. Tissue Eng 2004; 10:483 - 91; http://dx.doi.org/10.1089/107632704323061843; PMID: 15165465
- Gaebel R, Ma N, Liu J, Guan J, Koch L, Klopsch C, et al. Patterning human stem cells and endothelial cells with laser printing for cardiac regeneration. Biomaterials 2011; 32:9218 - 30; http://dx.doi.org/10.1016/j.biomaterials.2011.08.071; PMID: 21911255
- Guillemot F, Souquet A, Catros S, Guillotin B, Lopez J, Faucon M, et al. High-throughput laser printing of cells and biomaterials for tissue engineering. Acta Biomater 2010; 6:2494 - 500; http://dx.doi.org/10.1016/j.actbio.2009.09.029; PMID: 19819356
- Frimat JP, Sisnaiske J, Subbiah S, Menne H, Godoy P, Lampen P, et al. The network formation assay: a spatially standardized neurite outgrowth analytical display for neurotoxicity screening. Lab Chip 2010; 10:701 - 9; http://dx.doi.org/10.1039/b922193j; PMID: 20221557
- Ringeisen BR, Othon CM, Barron JA, Young D, Spargo BJ. Jet-based methods to print living cells. Biotechnol J 2006; 1:930 - 48; http://dx.doi.org/10.1002/biot.200600058; PMID: 16895314
- Stratakis E, Ranella A, Farsari M, Fotakis C. Laser-based micro/nanoengineering for biological applications. Prog Quantum Electron 2009; 33:127 - 63; http://dx.doi.org/10.1016/j.pquantelec.2009.06.001
- Barrett JC, Kawasaki ES. Microarrays: the use of oligonucleotides and cDNA for the analysis of gene expression. Drug Discov Today 2003; 8:134 - 41; http://dx.doi.org/10.1016/S1359-6446(02)02578-3; PMID: 12568783
- Schena M, Shalon D, Davis RW, Brown PO. Quantitative monitoring of gene expression patterns with a complementary DNA microarray. Science 1995; 270:467 - 70; http://dx.doi.org/10.1126/science.270.5235.467; PMID: 7569999
- Bailey SN, Sabatini DM, Stockwell BR. Microarrays of small molecules embedded in biodegradable polymers for use in mammalian cell-based screens. Proc Natl Acad Sci U S A 2004; 101:16144 - 9; http://dx.doi.org/10.1073/pnas.0404425101; PMID: 15534212
- Lee MY, Park CB, Dordick JS, Clark DS. Metabolizing enzyme toxicology assay chip (MetaChip) for high-throughput microscale toxicity analyses. Proc Natl Acad Sci U S A 2005; 102:983 - 7; http://dx.doi.org/10.1073/pnas.0406755102; PMID: 15657119
- Lee MY, Kumar RA, Sukumaran SM, Hogg MG, Clark DS, Dordick JS. Three-dimensional cellular microarray for high-throughput toxicology assays. Proc Natl Acad Sci U S A 2008; 105:59 - 63; http://dx.doi.org/10.1073/pnas.0708756105; PMID: 18160535
- Fernandes TG, Kwon SJ, Lee MY, Clark DS, Cabral JM, Dordick JS. On-chip, cell-based microarray immunofluorescence assay for high-throughput analysis of target proteins. Anal Chem 2008; 80:6633 - 9; http://dx.doi.org/10.1021/ac800848j; PMID: 18656951
- Bailey SN, Ali SM, Carpenter AE, Higgins CO, Sabatini DM. Microarrays of lentiviruses for gene function screens in immortalized and primary cells. Nat Methods 2006; 3:117 - 22; http://dx.doi.org/10.1038/nmeth848; PMID: 16432521
- Gobaa S, Hoehnel S, Roccio M, Negro A, Kobel S, Lutolf MP. Artificial niche microarrays for probing single stem cell fate in high throughput. Nat Methods 2011; 8:949 - 55; http://dx.doi.org/10.1038/nmeth.1732; PMID: 21983923
- Flaim CJ, Chien S, Bhatia SN. An extracellular matrix microarray for probing cellular differentiation. Nat Methods 2005; 2:119 - 25; http://dx.doi.org/10.1038/nmeth736; PMID: 15782209
- van Noort D, Ong SM, Zhang C, Zhang S, Arooz T, Yu H. Stem cells in microfluidics. Biotechnol Prog 2009; 25:52 - 60; http://dx.doi.org/10.1002/btpr.171; PMID: 19205022
- Gupta K, Kim D-H, Ellison D, Smith C, Kundu A, Tuan J, et al. Lab-on-a-chip devices as an emerging platform for stem cell biology. Lab Chip 2010; 10:2019 - 31; http://dx.doi.org/10.1039/c004689b; PMID: 20556297
- Brouzes E, Medkova M, Savenelli N, Marran D, Twardowski M, Hutchison JB, et al. Droplet microfluidic technology for single-cell high-throughput screening. Proc Natl Acad Sci U S A 2009; 106:14195 - 200; http://dx.doi.org/10.1073/pnas.0903542106; PMID: 19617544
- Thorsen TA. Microfluidic tools for high-throughput screening. Biotechniques 2004; 36:197 - 9; PMID: 14989081
- Ahn K, Agresti J, Chong H, Marquez M, Weitz DA. Electrocoalescence of drops synchronized by size-dependent flow in microfluidic channels. Appl Phys Lett 2006; 88:264105; http://dx.doi.org/10.1063/1.2218058
- Han MY, Gao XH, Su JZ, Nie S. Quantum-dot-tagged microbeads for multiplexed optical coding of biomolecules. Nat Biotechnol 2001; 19:631 - 5; http://dx.doi.org/10.1038/90228; PMID: 11433273
- Lu Y, Chen JJ, Mu L, Xue Q, Wu Y, Wu PH, et al. High-throughput secretomic analysis of single cells to assess functional cellular heterogeneity. Anal Chem 2013; 85:2548 - 56; http://dx.doi.org/10.1021/ac400082e; PMID: 23339603
- Fan R, Vermesh O, Srivastava A, Yen BK, Qin L, Ahmad H, et al. Integrated barcode chips for rapid, multiplexed analysis of proteins in microliter quantities of blood. Nat Biotechnol 2008; 26:1373 - 8; http://dx.doi.org/10.1038/nbt.1507; PMID: 19029914
- Bhatia S, Frangioni JV, Hoffman RM, Iafrate AJ, Polyak K. The challenges posed by cancer heterogeneity. Nat Biotechnol 2012; 30:604 - 10; http://dx.doi.org/10.1038/nbt.2294; PMID: 22781679
- Sailer M, Lai Wing Sun K, Mermut O, Kennedy TE, Barrett CJ. High-throughput cellular screening of engineered ECM based on combinatorial polyelectrolyte multilayer films. Biomaterials 2012; 33:5841 - 7; http://dx.doi.org/10.1016/j.biomaterials.2012.05.001; PMID: 22632764
- Ingham CJ, van Hylckama Vlieg JE. MEMS and the microbe. Lab Chip 2008; 8:1604 - 16; http://dx.doi.org/10.1039/b804790a; PMID: 18813380
- Chen PC, Huang YY, Juang JL. MEMS microwell and microcolumn arrays: novel methods for high-throughput cell-based assays. Lab Chip 2011; 11:3619 - 25; http://dx.doi.org/10.1039/c0lc00696c; PMID: 21904742
- Agudelo CG, Sanati Nezhad A, Ghanbari M, Naghavi M, Packirisamy M, Geitmann A. TipChip: a modular, MEMS-based platform for experimentation and phenotyping of tip-growing cells. Plant J 2013; 73:1057 - 68; http://dx.doi.org/10.1111/tpj.12093; PMID: 23217059
- Park JY, Lee DH, Lee EJ, Lee SH. Study of cellular behaviors on concave and convex microstructures fabricated from elastic PDMS membranes. Lab Chip 2009; 9:2043 - 9; http://dx.doi.org/10.1039/b820955c; PMID: 19568673
- Khetani SR, Bhatia SN. Microscale culture of human liver cells for drug development. Nat Biotechnol 2008; 26:120 - 6; http://dx.doi.org/10.1038/nbt1361; PMID: 18026090
- Teo BK, Ankam S, Yim EF. Stem Cell Interaction with Topography. In: Roy K, ed. Biomaterials as Stem Cell Niche: Springer Berlin Heidelberg, 2010:61-87.
- Markert LDA, Lovmand J, Foss M, Lauridsen RH, Lovmand M, Füchtbauer E-M, et al. Identification of distinct topographical surface microstructures favoring either undifferentiated expansion or differentiation of murine embryonic stem cells. Stem Cells Dev 2009; 18:1331 - 42; http://dx.doi.org/10.1089/scd.2009.0114; PMID: 19508153
- Unadkat HV, Hulsman M, Cornelissen K, Papenburg BJ, Truckenmüller RK, Carpenter AE, et al. An algorithm-based topographical biomaterials library to instruct cell fate. Proc Natl Acad Sci U S A 2011; 108:16565 - 70; http://dx.doi.org/10.1073/pnas.1109861108; PMID: 21949368
- Ankam S, Suryana M, Chan LY, Moe AA, Teo BK, Law JB, et al. Substrate topography and size determine the fate of human embryonic stem cells to neuronal or glial lineage. Acta Biomater 2013; 9:4535 - 45; http://dx.doi.org/10.1016/j.actbio.2012.08.018; PMID: 22906625
- Moe AA, Suryana M, Marcy G, Lim SK, Ankam S, Goh JZ, et al. Microarray with micro- and nano-topographies enables identification of the optimal topography for directing the differentiation of primary murine neural progenitor cells. Small 2012; 8:3050 - 61; http://dx.doi.org/10.1002/smll.201200490; PMID: 22807278
- Melchels FP, Feijen J, Grijpma DW. A review on stereolithography and its applications in biomedical engineering. Biomaterials 2010; 31:6121 - 30; http://dx.doi.org/10.1016/j.biomaterials.2010.04.050; PMID: 20478613
- Schofield R. The relationship between the spleen colony-forming cell and the haemopoietic stem cell. Blood Cells 1978; 4:7 - 25; PMID: 747780
- Moore KA, Lemischka IR. Stem cells and their niches. Science 2006; 311:1880 - 5; http://dx.doi.org/10.1126/science.1110542; PMID: 16574858
- Ceriotti L, Buzanska L, Rauscher H, Mannelli I, Sirghi L, Gilliland D, et al. Fabrication and characterization of protein arrays for stem cell patterning. Soft Matter 2009; 5:1406 - 16; http://dx.doi.org/10.1039/b814616k
- Brétagnol F, Lejeune M, Papadopoulou-Bouraoui A, Hasiwa M, Rauscher H, Ceccone G, et al. Fouling and non-fouling surfaces produced by plasma polymerization of ethylene oxide monomer. Acta Biomater 2006; 2:165 - 72; http://dx.doi.org/10.1016/j.actbio.2005.11.002; PMID: 16701874
- LaBarge MA, Nelson CM, Villadsen R, Fridriksdottir A, Ruth JR, Stampfer MR, et al. Human mammary progenitor cell fate decisions are products of interactions with combinatorial microenvironments. Integr Biol (Camb) 2009; 1:70 - 9; http://dx.doi.org/10.1039/b816472j; PMID: 20023793
- Lin C-H, Lee JK, LaBarge MA. Fabrication and use of microenvironment microarrays (MEArrays). J Vis Exp 2012; e4152; PMID: 23093325
- Brafman DA, Shah KD, Fellner T, Chien S, Willert K. Defining long-term maintenance conditions of human embryonic stem cells with arrayed cellular microenvironment technology. Stem Cells Dev 2009; 18:1141 - 54; http://dx.doi.org/10.1089/scd.2008.0410; PMID: 19327010
- Soen Y, Mori A, Palmer TD, Brown PO. Exploring the regulation of human neural precursor cell differentiation using arrays of signaling microenvironments. Mol Syst Biol 2006; 2:37; http://dx.doi.org/10.1038/msb4100076; PMID: 16820778
- Flaim CJ, Teng D, Chien S, Bhatia SN. Combinatorial signaling microenvironments for studying stem cell fate. Stem Cells Dev 2008; 17:29 - 39; http://dx.doi.org/10.1089/scd.2007.0085; PMID: 18271698
- Anderson DG, Putnam D, Lavik EB, Mahmood TA, Langer R. Biomaterial microarrays: rapid, microscale screening of polymer-cell interaction. Biomaterials 2005; 26:4892 - 7; http://dx.doi.org/10.1016/j.biomaterials.2004.11.052; PMID: 15763269
- Neuss S, Apel C, Buttler P, Denecke B, Dhanasingh A, Ding X, et al. Assessment of stem cell/biomaterial combinations for stem cell-based tissue engineering. Biomaterials 2008; 29:302 - 13; http://dx.doi.org/10.1016/j.biomaterials.2007.09.022; PMID: 17935776
- Mei Y, Saha K, Bogatyrev SR, Yang J, Hook AL, Kalcioglu ZI, et al. Combinatorial development of biomaterials for clonal growth of human pluripotent stem cells. Nat Mater 2010; 9:768 - 78; http://dx.doi.org/10.1038/nmat2812; PMID: 20729850
- Derda R, Li L, Orner BP, Lewis RL, Thomson JA, Kiessling LL. Defined substrates for human embryonic stem cell growth identified from surface arrays. ACS Chem Biol 2007; 2:347 - 55; http://dx.doi.org/10.1021/cb700032u; PMID: 17480050
- Nakajima M, Ishimuro T, Kato K, Ko IK, Hirata I, Arima Y, et al. Combinatorial protein display for the cell-based screening of biomaterials that direct neural stem cell differentiation. Biomaterials 2007; 28:1048 - 60; http://dx.doi.org/10.1016/j.biomaterials.2006.10.004; PMID: 17081602
- Anderson DG, Levenberg S, Langer R. Nanoliter-scale synthesis of arrayed biomaterials and application to human embryonic stem cells. Nat Biotechnol 2004; 22:863 - 6; http://dx.doi.org/10.1038/nbt981; PMID: 15195101
- Engler AJ, Sen S, Sweeney HL, Discher DE. Matrix elasticity directs stem cell lineage specification. Cell 2006; 126:677 - 89; http://dx.doi.org/10.1016/j.cell.2006.06.044; PMID: 16923388
- Mih JD, Sharif AS, Liu F, Marinkovic A, Symer MM, Tschumperlin DJ. A multiwell platform for studying stiffness-dependent cell biology. PLoS One 2011; 6:e19929; http://dx.doi.org/10.1371/journal.pone.0019929; PMID: 21637769
- Teo BKK, Ankam S, Chan LY, Yim EKF. Chapter 11 - Nanotopography/Mechanical Induction of Stem-Cell Differentiation. In: Shivashankar GV, ed. Methods in Cell Biology: Academic Press, 2010:241-94.
- Teixeira AI, McKie GA, Foley JD, Bertics PJ, Nealey PF, Murphy CJ. The effect of environmental factors on the response of human corneal epithelial cells to nanoscale substrate topography. Biomaterials 2006; 27:3945 - 54; http://dx.doi.org/10.1016/j.biomaterials.2006.01.044; PMID: 16580065
- Kim DH, Kim P, Suh K, Kyu Choi S, Ho Lee S, Kim B. Modulation of adhesion and growth of cardiac myocytes by surface nanotopography. Conf Proc IEEE Eng Med Biol Soc 2005; 4:4091 - 4; PMID: 17281132
- Biggs MJ, Richards RG, Gadegaard N, McMurray RJ, Affrossman S, Wilkinson CD, et al. Interactions with nanoscale topography: adhesion quantification and signal transduction in cells of osteogenic and multipotent lineage. J Biomed Mater Res A 2009; 91:195 - 208; http://dx.doi.org/10.1002/jbm.a.32196; PMID: 18814275
- Dalby MJ, Gadegaard N, Tare R, Andar A, Riehle MO, Herzyk P, et al. The control of human mesenchymal cell differentiation using nanoscale symmetry and disorder. Nat Mater 2007; 6:997 - 1003; http://dx.doi.org/10.1038/nmat2013; PMID: 17891143
- Cecchini M, Bumma G, Serresi M, Beltram F. PC12 differentiation on biopolymer nanostructures. Nanotechnology 2007; 18:505103; http://dx.doi.org/10.1088/0957-4484/18/50/505103
- Christopherson GT, Song H, Mao H-Q. The influence of fiber diameter of electrospun substrates on neural stem cell differentiation and proliferation. Biomaterials 2009; 30:556 - 64; http://dx.doi.org/10.1016/j.biomaterials.2008.10.004; PMID: 18977025
- Yim EK, Pang SW, Leong KW. Synthetic nanostructures inducing differentiation of human mesenchymal stem cells into neuronal lineage. Exp Cell Res 2007; 313:1820 - 9; http://dx.doi.org/10.1016/j.yexcr.2007.02.031; PMID: 17428465
- Lee MR, Kwon KW, Jung H, Kim HN, Suh KY, Kim K, et al. Direct differentiation of human embryonic stem cells into selective neurons on nanoscale ridge/groove pattern arrays. Biomaterials 2010; 31:4360 - 6; http://dx.doi.org/10.1016/j.biomaterials.2010.02.012; PMID: 20202681
- Lovmand J, Justesen J, Foss M, Lauridsen RH, Lovmand M, Modin C, et al. The use of combinatorial topographical libraries for the screening of enhanced osteogenic expression and mineralization. Biomaterials 2009; 30:2015 - 22; http://dx.doi.org/10.1016/j.biomaterials.2008.12.081; PMID: 19178942
- Sasaki D, Shimizu T, Masuda S, Kobayashi J, Itoga K, Tsuda Y, et al. Mass preparation of size-controlled mouse embryonic stem cell aggregates and induction of cardiac differentiation by cell patterning method. Biomaterials 2009; 30:4384 - 9; http://dx.doi.org/10.1016/j.biomaterials.2009.05.003; PMID: 19487020
- Migliorini E, Grenci G, Ban J, Pozzato A, Tormen M, Lazzarino M, et al. Acceleration of neuronal precursors differentiation induced by substrate nanotopography. Biotechnol Bioeng 2011; 108:2736 - 46; http://dx.doi.org/10.1002/bit.23232; PMID: 21656711