Abstract
It is recognized that, as the bladder fills, there is a corresponding increase in sensation. This awareness of the volume in the bladder is then used in a complex decision making process to determine if there is a need to void. It is also part of everyday experience that, when the bladder is full and sensations strong, these sensations can be suppressed and the desire to void postponed. The obvious explanation for such altered perceptions is that they occur centrally. However, this may not be the only mechanism. There are data to suggest that descending neural influences and local factors might regulate the sensitivity of the systems within the bladder wall generating afferent activity. Specifically, evidence is accumulating to suggest that the motor-sensory system within the bladder wall is influenced in this way. The motor-sensory system, first described over 100 years ago, appears to be a key component in the afferent outflow, the afferent “noise,” generated within the bladder wall. However, the presence and possible importance of this complex system in the generation of bladder sensation has been overlooked in recent years. As the bladder fills the motor activity increases, driven by cholinergic inputs and modulated, possibly, by sympathetic inputs. In this way information on bladder volume can be transmitted to the CNS. It can be argued that the ability to alter the sensitivity of the mechanisms generating the motor component of this motor-sensory system represents a possible indirect way to influence afferent activity and so the perception of bladder volume centrally. Furthermore, it is emerging that the apparent modulation of sensation by drugs to alleviate the symptoms of overactive bladder (OAB), the anti-cholinergics and the new generation of drugs the β3 sympathomimetics, may be the result of their ability to modulate the motor component of the motor sensory system. The possibility of controlling sensation, physiologically and pharmacologically, by influencing afferent firing at its point of origin is a “new” concept in bladder physiology. It is one that deserves careful consideration as it might have wider implications for our understanding of bladder pathology and in the development of new therapeutic drugs. In this overview, evidence for the concept peripheral modulation of bladder afferent outflow is explored.
Introduction
The sensations we associate with the volume in our bladder are part of everyday life. As the bladder fills, sensations grow. However, the perception of bladder volume is only one of many factors that we take into consideration when deciding where and when to void. We constantly integrate our bladder sensations into calculations of the proximity of an appropriate place to void, possible delays in getting there, a knowledge of recent fluid intake and practical issues involving propriety and safety. For the vast majority, this is done automatically with little conscious thought. It is part of a complex learned behavior. The generation, perception and control of bladder sensations are, without doubt, key factors. Our understanding of how this information is generated and how it is processed is still rudimentary. Critically, it is now clear that the bladder is not just a passive bag that has stretch receptors in the wall, which, when activated, trigger the coordinated reflexes of voiding. The sensory systems in the bladder wall are varied and complex with the potential for even greater complexity and subtlety.
As the bladder begins to fill we are not normally aware of it. However, as filling continues, sensations increase and this begins to encroach into consciousness and to influence behavior. At this stage decisions are made to take no action or to seek to void. If a decision to void is made, sensations are heightened focusing attention on finding a safe and appropriate place to void.Citation1-Citation6 These sensations alone do not trigger a reflex void. Rather the information appears to be used in a complex decision making process.Citation4 The sensation of bladder “awareness” during filling has been shown to increase progressively as the bladder is filled at a constant rate.Citation5,Citation6 This pattern of growing awareness correlates well with electrophysiological data recording from bladder afferents.Citation7-Citation11 Thus, the CNS is constantly receiving information on the state of filling of the bladder.
However, when the bladder is near capacity and awareness is high, it is possible to defer voiding. The strong sensations of bladder awareness can appear to be suppressed and effectively lost from consciousness for short periods of time.Citation12 This waxing and waning of strong bladder sensations are indeed part of everyday experience. The value of this ability to suppress sensation is obvious, we can defer voiding to a more convenient time, within reason. Also, the opposite can occur. When approaching a toilet, sensation can increase in preparation, focusing attention on the processes of voiding, the so-called “latch key phenomenon.” However, despite the importance of these sensory adaptations of increased and decreased sensation, the physiological mechanisms underlying such gains and losses are not known.
One obvious explanation for changes in the perception of bladder fullness is that this occurs within the CNS, as the result of information processing within the spinal cord or higher centers. Central processing and modulation of sensation is well recognized in many other sensory systems, for example altering perceptions of pain [for a review see ref. Citation13]. Ideas are now emerging in relation to the areas of the CNS that might be involved in processing such information from the bladder.Citation14,Citation15 These ideas are not reviewed here. However, briefly, as the bladder fills areas in the midbrain are progressively activated including the insula, periaqueductal gray, anterior cingulate gyrus, locus coeruleus, prefrontal cortex, and Barrington’s nucleus. These areas are almost certainly associated with progressive awareness of bladder volume as it fills. When a decision to void is made, those regions associated with behavioral control are activated and then the coordinated voiding reflexes within the pons in Barrington’s nuclei control voiding. Within such complexity of central sensory processing, it is almost certain that the system would allow the perception of sensation to be altered. Central processing of information from the bladder must be a component in the modulation of bladder sensations. But is it the only one?
An intriguing and alternative possibility is that the afferent outflow from the bladder can be modulated directly within the bladder or within the accessory systems involved in the generation of bladder sensation. If afferent outflow were to be affected by such peripheral mechanisms, the perception of bladder sensation could be modified. Sensations could either be depressed or increased, temporarily diminishing or enhancing the need to void. Such a peripheral modulation of bladder sensation has not been considered a possibility. However, there are some basic observations and emerging data that support this idea. In other sensory systems, the peripheral modulation of sensory outflow is recognized and has been well characterized. For example, in the somatic nervous system, the gain of the muscle spindle, the complex receptor mechanism involved in determining muscle length, can be increased or decreased by a specific and specialized efferent innervation, γ motor neurones.Citation16 Similar regulatory events are seen at the invertebrate equivalent stretch receptor.Citation17 So, peripheral regulation of afferent activity is known.
The purpose of this overview is to explore the concept of peripheral regulation of the afferent outflow from the bladder and to consider possible mechanisms that might underpin such a system. If this type of sensory regulation does occur it might be an important new insight into our understanding the physiology and pathology of voiding.
Perspectives—A Classical View
The classical view of the efferent and afferent innervation of the bladder is based on observations made over 100 years ago.Citation18-Citation24 Basically, the neural connections and sensory elements controlling the lower urinary tract (LUT) were considered to involve multiple reflex arcs operating at the levels of the spinal cord and brain stem. Of the three major nerves supplying the bladder, urethra and sphincters, the pelvic, hypogastric contain both afferent and efferent fibers, both sympathetic and parasympathetic,Citation22,Citation23 while the pudendal nerve carries somatic efferent and afferent fibers. Transection of the pelvic nerves removed central control of voiding contractions, suggesting that the major efferent motor component of reflex nerves were carried there. In contrast, sectioning the hypogastric nerves did not affect voiding efficiency, but voiding frequency was increased and the voided volume decreased.Citation20 Barrington deduced that the efferents in the hypogastric, principally sympathetic fibers, have a profound regulatory role in determining the timing of voiding, a result since repeated by others.Citation25-Citation27 Specifically how this was brought about was not considered directly. Thus, simplistically, the parasympathetic and sympathetic arms of the autonomic nervous system had defined effects on the bladder: parasympathetics were motor and sympathetics regulatory. Peripheral control of voiding frequency by sympathetic efferent outflow is perhaps the first indication of peripheral control of afferent function.
With respect to the afferent limb, Sherrington pointed out that the afferent nerves leaving an organ might be of two types, those which contributed to sensation and those that did not result in sensation but which were involved in basic reflex activity.Citation19,Citation28 Therefore, in any experiment recording afferent nerve activity, it was impossible to determine if this was involved in generating sensations or contributing to a reflex. So, it is not straight forward to study neuro-physiologically sensations generated in the bladder in animal models using electrical recording from afferent nerves. The problem of linking afferent fibers to sensation is further compounded by the fact that the very nature of the sensations resulting from the afferents that reached consciousness is very difficult to describe.Citation1,Citation3,Citation29 Thus, questions relating to which fibers contribute to particular bladder sensations have been put to one side.
Complex Afferent Outflow from the Bladder
Despite these difficulties, attempts have been made to characterize the basic electrophysiological properties of the general population of afferent fibers. This task was begun by Iggo in 1955Citation30 who described fibers that responded to stretch and contraction: in series and in parallel fibers. Also, based on conduction velocity and myelination, two broad groups were identified: myelinated Aδ fibers (conduction velocity > 1.3 m/sec) and un-myelinated C fibers (conduction velocity < 1.3 m/sec).Citation30-Citation34 Afferent fibers of both types have been found in both major nerves innervating the bladder, the hypogastric and pelvic nerves.Citation35 The properties of the different afferent fiber types have been the subject of intensive investigation. The situation is complex and is, as yet, not fully understood. In general, the small myelinated Aδ fibers are thought to be located in the detrusor and respond to both distension and contraction.Citation30 The functional properties and the anatomical location of the population of C fibers is more complex, with the majority of the C fibers thought to be located within the mucosal layer of the bladder, although this is by no means certain.Citation34 Morrison proposed, based on their functional properties, that there were at least 4 distinct sub-groups of mucosal fibers that: (1) responded to stretch of the mucosa, (2) were silent in normal conditions but which were activated by excessive distension, termed “pain fibers,” (3) were insensitive to distension but which responded to cold stimuli and (4) were normally insensitive to distension but which could become active in pathological conditions such as bladder inflammation
Based on morphological and molecular criteria, different sub-types of afferent nerves originate from within the bladder wall. In a key early study, it was shown that different afferent fibers had different expression profiles for characteristic markers (: adapted from ref. Citation34). Adding to this, it is now recognized that dorsal root ganglion (DRG) neurones can also express different markers, ChAT (choline acetyl-transferase),Citation37 sodium channel sub-types,Citation38 and lectin binding,Citation39 suggesting multiple types of afferent nerve. In the rat it is clear that a significant number of afferent fibers (Aδ and C fibers) express CGRP (calcitonin gene related peptide) and substance-P immuno-reactivity while in contrast C fibers express little NF (neurofilament) while all the Aδ fibers do.Citation36 These observations are very important as they strongly suggest that there are different and discrete types of afferent fiber and that classifications based purely on myelination and conduction velocity are an oversimplification. Indeed, there is now a significant literature demonstrating quite elegantly the complexity and distribution of afferent nerves in the bladder wall and the different modalities to which they are sensitive (see refs Citation14,Citation15,Citation40, and Citation41 for an overview) ().
Table 1. Neurofiliament and peptide content of DRG neurones with identified C, Aδ or Aα/β fiber conduction velocities (data extracted from ref. Citation36)
Using CGRP as a marker for a sub-population of afferent fibers, it has been shown that all muscle bundles in the bladder wall (rat) show CGRP immuno-reactive (CGRP-IR) nerves.Citation42 In contrast, CGRP-IR afferent innervation of the mucosa is not uniform throughout the bladder, being highest at the bladder base. There are few, if any, CGRP-IR fibers in the lateral wall or bladder dome.Citation42 This latter observation is of fundamental importance. It implies that the urothelium, in those regions with no nerve fibers, cannot be operating as a stretch sensor for an afferent sensory system: there are no nerves. Therefore, the complex functions of the urothelium, in these non-innervated regions, needs to be better understood.
By way of an intermediate summary, the current state of our understanding of the afferent system in the bladder is rudimentary. It is clear that there are morphologically and anatomically distinct types of afferent fiber, but we do not know what these different subtypes do. Importantly, we do not know which fibers project to consciousness and contribute to bladder sensation. The afferent system is regarded simply as a sensing and output system: as the bladder fills the output increases. With this simple model there is no obvious component or element to suggest that sensation might be modulated in the periphery. The conclusion might be that any modulation of sensation would occur centrally.
“Afferent Noise” and the Concept of the Peripheral Regulation of Sensation
It would appear that we are no further forward in considering the mechanisms of sensation or their modulation. In an attempt to simplify the complexity of the afferent systems in the bladder, a basic conceptual model has been proposed: the concept of “afferent noise.”Citation43 Here it was suggested that information from the bladder, “afferent noise,” can be separated into discrete physiological systems. There are at least 4 components: (1) a system registering pain, (2) a mechano-sensory system activated by simple stretch, (3) a urothelial system activated by signals released from the urothelium, and (4) a motor-sensory system activated by micro-contractions and stretches in the bladder wall. The elements within these systems have been identified and characterized using both functional and morphological approaches ().Citation14,Citation15,Citation40,Citation41,Citation43-Citation45 The value of this “systems physiology” approach allows us to examine the properties and regulation of each system, without need for a full understanding of intrinsic cellular and molecular mechanisms.
Figure 1. The components of afferent noise in the guinea pig. (A) Illustrates the broad elements of the afferent systems associated with the bladder: components of afferent noise. Four are identified: pain, mechano-sensory, urothelial and motor-sensory. Each sends afferent information to the CNS but only the motor-sensory system has the potential for an output from the CNS and inputs from peripheral afferent fibers. (B) Illustrates in more detail some of the component parts of the systems making up afferent noise. The afferent out flow to the CNS can again be seen for each system: 1, pain; 2, mechano-sensitive (stretch); 3, urothelial; 4, motor-sensory. For the motor-sensory system, part of the complex regulatory systems involved in the regulation of motor activity may be occurring via the intra-mural ganglia and local neural circuits within the bladder wall. Reprinted from reference Citation43 with permission.
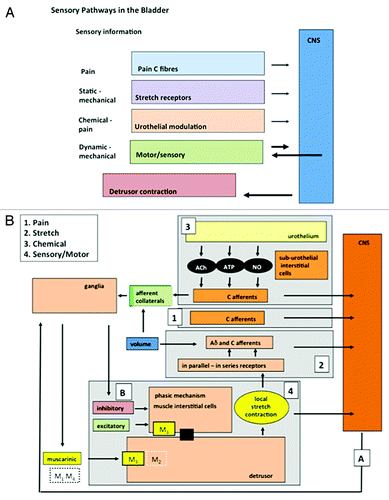
What is emerging is that there may be components within the afferent noise systems that can be influenced by external inputs. If this is so, then we might have evidence for a modulation of bladder sensation at the sites where the afferent outflow originates. Such mechanisms would contribute to an explanation for the perception of waxing and waning of sensations from the bladder without any alterations in bladder volume.
The following sections explore the possible regulation of elements of afferent noise in relation to the physiological modulation of perceived bladder sensations.
Pain afferent noise
Pain is a critical component of the pathological afferent outflow from the bladder. The ability to modulate the sensation of pain is well recognized.Citation41,Citation46 It is known, that this can occur centrally, within the cord and higher centers, where sensations can be increased or decreased. Sensitization of afferent nerves by inflammation is well recognized to occur as a result of modulation of excitatory mechanisms linked to expression of voltage gated ion channels. Such changes are recognized in many systems including the bladder.Citation13 Sensitization of C fibers could occur anywhere within the bladder wall. However, the urothelium might, in certain circumstances, be involved specifically in the sensitization of C fiber afferents linked to pain, for example, in interstitial cystitis.
Although pain, in all systems studied, can be modulated, the peripheral mechanisms associated with these events appear to be exclusively associated with the intensification of sensations by the pathological sensitization of the afferent nerves. It is likely that the bladder is similar, with centrally modulated and peripherally sensitized elements. These are pathological situations and are not involved in a minute by minute regulation of sensation. Thus, the pain system within afferent noise is unlikely to be involved in the waxing and waning of physiological bladder sensations as it fills and approaches maximum volume.
Urothelial afferent noise
Ideas that the urothelium was more than a simple barrier preventing penetration of urine into the bladder wall were formulated over 50 years ago. A great deal has been written on this in recent years and needs not be reiterated here. However, it was a series of discoveries that the urothelium could synthesize and release a range of substances (prostaglandins, nitric oxide, ATP, and acetylcholine) in response to stretch, that led to the concept that the urothelium is a key accessory element of an afferent system transducing bladder volume.Citation47-Citation53 When released, these agents activate (ATPCitation54) or decrease (NOCitation55) afferent firing and so contribute to afferent noise (urothelial afferent noise).Citation51-Citation53 Urothelial signal production and interaction between the signals have been the subject of many papers and reviews in recent years.Citation51-Citation53 So the urothelial system, urothelial afferent noise, clearly has the potential to modulate afferent firing.
Recently, observations have been made showing that these urothelial signals interact with each other in the absence of stretch, such that the net signal output of the urothelium appears to be an integrated response.Citation56 In the guinea pig, certain signals are stimulatory, e.g., acetylcholine and ATP increase prostaglandin production, while some are inhibitory, nitric oxide decreases prostaglandin output.Citation56 The importance of these interactions is not fully understood, but their presence raises the possibility that the final activation of the afferent fibers might occur only after a considerable degree of signal and information processing within the urothelium. If this is so, then mechanisms such as this might represent means whereby sensation is modulated locally, both increased and decreased.
However, there is no evidence so far, demonstrating a direct efferent control of the urothelial system of afferent noise. Until this lack of knowledge is addressed this element may not be a candidate for the modulation of sensation as perceived by higher centers. The urothelium seems to function only to send information not to respond to descending neural control. Furthermore, it must be borne in mind that the urothelial mechanisms may not be operating under physiological conditions, but may only come into play when the bladder is excessively distended, inflamed or damaged.
Motor-sensory afferent noise
The existence of a motor-sensory system in the bladder was initially proposed at the turn of the 20th century ().Citation18,Citation57 Local contractions not associated with voiding, non-voiding activity (NVA), and the local stretches that accompany them were recorded during bladder filling. These contractions increased in amplitude and frequency as the bladder filled (see ).Citation10,Citation18,Citation59,Citation60 It was suggested that this motor activity is directly coupled to afferent activity (),Citation7-Citation9,Citation11,Citation30 sending information to the CNS regarding bladder volume.Citation57 The motor-sensory system is a component of afferent noise that can inform the CNS on the state of bladder filling. This afferent outflow does not appear to be part of the autonomic reflexes involved in the control of the coordination of micturition (Barrington’s reflexes). For reasons that will be argued below, it is possible that this system is a reasonable candidate to contribute to the sensations of “awareness” as the bladder fills.Citation4,Citation6 It is also a candidate for modulation.
Figure 2. Motor activity and the generation of afferent firing. (A) Illustrates one of the original records of non-voiding motor activity recorded in the cat from where it was first proposed that this activity was associated with afferent firing and the control of micturition. (B) Illustrates confirmation of this concept showing the motor activity in the cat (upper record) with associated bursts of afferent nerve activity (lower record) (reprinted from reference Citation58 with permission). (C) Shows a cystometric record from a conscious rat during bladder filling. Voids can be seen at the beginning and end of the recording. During the filling phase small non-voiding contractions appear, progressively increasing in amplitude and frequency as the bladder fills. The progressive increase in motor activity implies that the intensity of afferent activity is also increasing during filling (reprinted from reference Citation59 with permission).
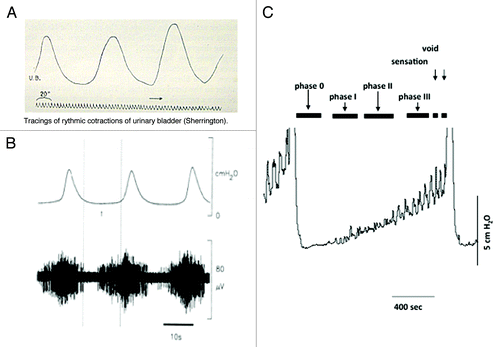
It has also been known for over 100 years, that the sympathetic innervation to the bladder exerts a profound effect on voiding behavior: section of the sympathetic nerves (hypogastric nerve) to the bladder in the cat, doubled the frequency of voiding, with the animals voiding reduced volumes.Citation20,Citation61 Stimulation of sympathetic nerves has also been shown to have profound effects on NVA.Citation25,Citation26,Citation62 Thus, the output of the motor-sensory system appears to be under direct CNS control. By reducing the afferent output in this way, bladder sensation may be depressed at the site of origin within the bladder wall. At times of stress (sympathetic activation), bladder afferent outflow, possibly sensation, could be reduced, leaving the individual free to concentrate on other things. Thus, sympathetic control of bladder afferent outflow, operating via the motor-sensory system, may be involved in the waxing and waning of sensations experienced in everyday life.
An unexpected link, supporting the idea for a sympathetic regulation of the motor-sensory system, has come from experiments with drugs that mimic sympathetic nerve action. Recently, β3-specific adrenoceptor agonists have been suggested to be effective in reducing the frequency of voiding in patients diagnosed with the overactive bladder symptom complex (OABSC).Citation63 In an obstructed rat model, where NVA is dramatically increased during bladder filling, direct pharmacological activation of β3 adrenoceptors by mirabegron reduces both the amplitude and frequency of the NVA ().Citation10 This adrenergic inhibition of motor-sensory noise suggests that adrenergic nerves might be involved in the system controlling NVA. Thus, sympathetic reduction in motor-sensory noise reduces sensation and allows deferment of the decision to void.
Figure 3. The effects of the β3 specific agonist mirabegron on motor sensory noise in the conscious partially obstructed rat (modifed from reference Citation10). (A) Illustrates an original record showing 4 filling and voiding cycles during cystometry in a conscious rat. The micturition contractions are easily seen. The first 2 cycles are under control conditions and the motor component of the motor-sensory system, the non-voiding activity, is apparent. This is more clearly seen in section (B) where the non-voiding activity has been isolated by filtering. The β3 specific agonist (YM-178, mirabegron) was then added and the effects on the following filling and voiding cycles noted. The drug clearly affects the non-voiding activity but has little effect on the amplitude of the voiding contraction. (Reprinted from reference Citation10 with permission). (B) Shows a cartoon proposing how on-voiding activity and micturition activity is generated in the rat. The accepted parasympathetic motor system is there to initiate the large voiding contraction. In addition the system generating and modulating the motor component of the motor-sensory noise behaves as though it had a “pacemaker” controlled by cholinergic (excitatory) and adrenergic (inhibitory) inputs. Afferent fibers (green) respond to the local contractions and stretches sending information related to bladder volume to the CNS.
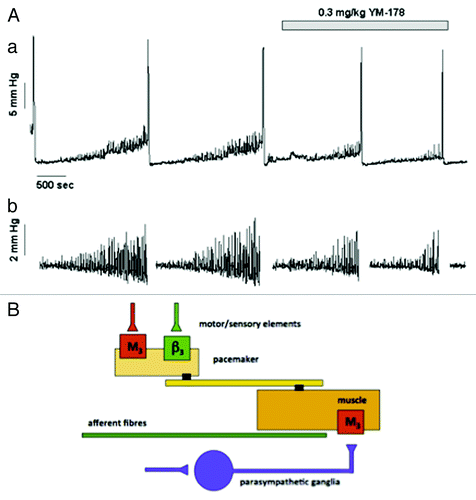
A parallel argument can be used for a cholinergic regulation of NVA. Anti-cholinergic drugs reduce sensations during the filling phase. These drugs do so at plasma concentrations that do not have a major effect on the detrusor neuromuscular junction.Citation64,Citation65 Furthermore, at therapeutic concentrations, they do not affect the overt overactive bladder contractions. Critically, they are able to alleviate symptoms in patients that do not have overt bladder contractions during cystometric investigationCitation66 suggesting that they do not have a motor effect but act on sensory mechanisms. In search for an alternative site of action, it has been shown, in the outflow obstructed rat, that the non-voiding activity is affected at therapeutic concentrations.Citation10 It was therefore argued that the mechanisms generating NVA were the therapeutic target for these drugs also.
The data accumulating so far is suggesting the presence of a motor-sensory system that is augmented by cholinergic and inhibited by adrenergic inputs. Thus, we have evidence, in animal models at least, for a component of afferent noise that is clearly capable of modulation. Since the anticholinergic and sympathomimetic drugs affect sensation during filling and also NVA, we have circumstantial evidence to suggest that the NVA element of afferent noise is indeed linked to the perception of bladder volume.
The mechanisms involved in the regulation of NVA may be more complicated. When the bladder is isolated and maintained in vitro, spontaneous activity, autonomous activity, can readily be recorded in nearly all species examined.Citation18,Citation58,Citation67 It has been speculated that this autonomous activity represents the systems in the bladder wall that underlie or contribute to NVA.Citation68 Studies on the pharmacological modulation of this autonomous activity suggests that it can be increased by cholinergicCitation64 and purinergic agonistsCitation67 and prostanoidsCitation68 and decreased by nitrergic,Citation69 peptidergic (calcitonin gene related peptide [CGRP])Citation70 and adrenergicCitation71 stimulation. This has led to the suggestion that this mechanism is the target for multiple systems that can lead to modulation of bladder sensation.Citation58 Inhibition of autonomous activity by CGRP has also led to the idea that this system may be modulated by local neural reflexes within the bladder wall (). Citation72,Citation73 Collaterals of afferent fibers expressing CGRP are seen to make contact with neurones in intra-mural ganglia.Citation69 Stimulation of these afferents either associated with the urothelium or muscle layers appear to modulate autonomous activity. In this way sensation may be further modulated, but in these cases by mechanisms originating within the bladder wall.
Figure 4. Images of afferent nerves and intra-mural ganglia in the guinea pig bladder. The sections were stained for choline-acetyltransferase (ChAT) (red) detecting the enzyme responsible for synthesising acetylcholine. The sections were also stained with an antibody to calcitonin gene related peptide (CGRP) (green). Almost certainly these CGRP fibers are afferent fibers. (A and B) Show images of sub-urothelial cholinergic (A) and peptidergic (CGRP) (B) nerves indicating two distinct populations of afferent fiber. (C and D) Show cholinergic terminals (C, red) within the intra-mural-ganglia as are CGRP terminals (C and D, green). These observations suggest the possibility of integration of different inputs into these neurons. Panel E shows a cartoon illustrating the possible interactions of afferent collaterals, both peptidergic (CGRP) and cholinergic with the intra-mural ganglia. An output to the muscle is postulated that is in addition to the conventional parasympathetic system involved in the initiation of the voiding contraction (reprinted from reference Citation73 with permission).
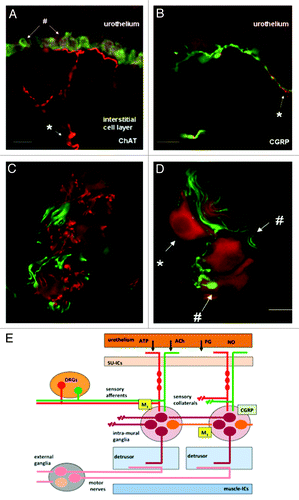
Intra-mural ganglia are found in many species, but not all. For example, they are absent in the mouse and rat.Citation74 Therefore, if some form of neural circuitry is involved in regulating bladder motor-sensory activity, what happens in the mouse and rat? In all species, nerves leaving the spinal cord travel to the periphery, transiting and making contacts in peripheral ganglia.Citation75 The neural elements, directed to the bladder, reproductive organs and colon, are contained in a distributed network of ganglia in the pelvis: the pelvic plexus. It has been suggested that the intra-mural ganglia in the bladder are a sub-set of this network.Citation76 In the male rat there is a particularly large single peripheral ganglion, the major pelvic ganglion (MPG) that receives inputs from the pelvic and hypogastric nerves and connects to the bladder. It is possible that this structure sub-serves some of the complex functional interactions and modulations that might be ascribed to the intra-mural ganglia. Indeed there is a great deal of literature discussing the potential integrative functions in these pelvic ganglia (see ref. Citation76 for a review and refs. Citation77-Citation80). The vast majority of this work has focused on the possible modulation of efferent function, with little consideration that processing in the ganglia might have a sensory element. The possibility, put forward here, is that the elements for control and modulation of the motor-sensory system might reside in these intra-mural and extra-mural ganglia.
The motor-sensory system in vivo is augmented by a cholinergic input as deduced from the fact that it is inhibited by muscarinic antagonists.Citation10 It has been reported that a sub-population of neurones in the pelvic ganglia express muscarinic receptors.Citation76 It is therefore possible that it is this sub-population of neurones that could be linked to the generation of motor-sensory activity. The concept for a functionally different sub-set of ganglionic motor neurones is also suggested by the distribution of other regulatory inputs. As in the intra-mural ganglia in the guinea pig, CGRP and substance P afferent collateral fibers are seen to form basket like structures on a sub-population of cholinergic neurones in the rat major pelvic ganglion and in the female equivalent ganglion, the paracervical ganglion.Citation76 Furthermore, noradrenergic terminals, also forming basket like structures, have also been observed around a sub-population of MPG neurones pointing to a possible descending sympathetic regulatory input within the ganglia.Citation76,Citation81-Citation84 It is not known if these sub-populations converge, representing a single population of ganglion cells or whether they might connect via interneurons. It is therefore tempting to speculate that, within these peripheral ganglia, we have two functional physiological systems: the classical nicotinic parasympathetic relay system for activating the detrusor during voiding and a further system, involving a sub-set of neurones, receiving muscarinic, peptidergic and adrenergic inputs, linked to the regulation of the motor component of motor-sensory afferent noise.
Afferent noise, generated from NVA, may be an important system involved in the perception of bladder volume. The complexity and regulation of motor-sensory noise points to its basic physiological importance. First, it appears to have extrinsic (cholinergic and sympathomimetic) excitatory and inhibitory inputs. Second, it is also influenced by local reflexes and local mediators residing within the bladder wall. Third, it is altered in conditions of bladder pathology where there is likely to be increased bladder sensations. Finally, motor-sensory noise appears to be the site of therapeutic action for the major drugs used to treat bladder over activity and incontinence.
Thus, there may indeed be evidence for the modulation of sensation by the regulation of peripheral sensory systems.
Conclusions
The control of the bladder and the lower urinary tract is much more complex than commonly acknowledged. Also, key basic and critical observations about bladder function and bladder sensations are yet to be made. In attempts to address such questions we are now discovering, or re-discovering, ideas and phenomena that have lain dormant in the literature for many years. The motor-sensory system is one such element. In addition, re-examination of the structures within the bladder wall, the intra mural ganglia, and the pelvic plexus reveals elements that might contribute to the peripheral regulation of afferent mechanisms. These systems appear to involve excitatory and inhibitory synaptic input. This, in turn, raises the possibility of pharmacological manipulation of bladder function, specifically sensation. This may indeed be the mode of action of drugs used to treat bladder dysfunction. Finally, and critically, an understanding of systems regulating sensation may provide and alternative basis with which to understand and interpret the pathologies of the LUT. Thus, with a deeper understanding of basic physiology, novel systems targeted by specific pharmacology and new insights into pathology, the future holds the promise of new and exciting discoveries that will ultimately benefit those who suffer from the disabling pathologies of the bladder.
06/28/13
07/17/13
07/23/13
Disclosure of Potential Conflicts of Interest
No potential conflicts of interest were disclosed.
References
- Denny-Brown D, Robertson EG. On the physiology of micturition. Brain 1933; 56:149 - 90; http://dx.doi.org/10.1093/brain/56.2.149
- Nathan PW. Sensations associated with micturition. Br J Urol 1956; 28:126 - 31; http://dx.doi.org/10.1111/j.1464-410X.1956.tb04744.x; PMID: 13342451
- De Wachter SGG, Heeringa R, van Koeveringe GA, Gillespie JI. On the nature of bladder sensation: the concept of sensory modulation. Neurourol Urodyn 2011; 30:1220 - 6; PMID: 21661031
- Harvey J, Finney S, Stewart L, Gillespie JI. The relationship between cognition and sensation in determining when and where to void: the concept of cognitive voiding. BJU Int 2012; 110:1756 - 61; http://dx.doi.org/10.1111/j.1464-410X.2012.11078.x; PMID: 22642959
- Wyndaele JJ. The normal pattern of perception of bladder filling during cystometry studied in 38 young healthy volunteers. J Urol 1998; 160:479 - 81; http://dx.doi.org/10.1016/S0022-5347(01)62929-X; PMID: 9679902
- Gillespie JI, Eastham J, de Wachter S. The development of bladder sensation during filling and when preparing to void. Abstract, ICS Barcelona, 2013.
- Shea VK, Cai R, Crepps B, Mason JL, Perl ER. Sensory fibers of the pelvic nerve innervating the Rat’s urinary bladder. J Neurophysiol 2000; 84:1924 - 33; PMID: 11024085
- De Wachter S, De Laet K, Wyndaele J-J. Does the cystometric filling rate affect the afferent bladder response pattern? A study on single fibre pelvic nerve afferents in the rat urinary bladder. Neurourol Urodyn 2006; 25:162 - 7; http://dx.doi.org/10.1002/nau.20157; PMID: 16372317
- De Wachter S. Afferent signaling from the bladder: species differences evident from extracellular recordings of pelvic and hypogastric nerves. Neurourol Urodyn 2011; 30:647 - 52; http://dx.doi.org/10.1002/nau.21135; PMID: 21661008
- Gillespie JI, Palea S, Guilloteau V, Guerard M, Lluel P, Korstanje C. Modulation of non-voiding activity by the muscarinergic antagonist tolterodine and the β(3)-adrenoceptor agonist mirabegron in conscious rats with partial outflow obstruction. BJU Int 2012; 110:2 Pt B E132 - 42; http://dx.doi.org/10.1111/j.1464-410X.2012.11240.x; PMID: 22734512
- Aizawa N, Homma Y, Igawa Y. Characteristics of lower urinary tract dysfunction and bladder afferent nerve properties in type 2 diabetic goto-kakizaki rats. J Urol 2013; 189:1580 - 7; http://dx.doi.org/10.1016/j.juro.2012.10.060; PMID: 23103236
- Chapple CR, Artibani W, Cardozo LD, Castro-Diaz D, Craggs M, Haab F, et al. The role of urinary urgency and its measurement in the overactive bladder symptom syndrome: current concepts and future prospects. BJU Int 2005; 95:335 - 40; http://dx.doi.org/10.1111/j.1464-410X.2005.05294.x; PMID: 15679789
- Ossipov MH, Dussor GO, Porreca F. Central modulation of pain. J Clin Invest 2010; 120:3779 - 87; http://dx.doi.org/10.1172/JCI43766; PMID: 21041960
- Zagorodnyuk VP, Gibbins IL, Costa M, Brookes SJ, Gregory SJ. Properties of the major classes of mechanoreceptors in the guinea pig bladder. J Physiol 2007; 585:147 - 63; http://dx.doi.org/10.1113/jphysiol.2007.140244; PMID: 17916614
- Zagorodnyuk VP, Brookes SJ, Spencer NJ, Gregory S. Mechanotransduction and chemosensitivity of two major classes of bladder afferents with endings in the vicinity to the urothelium. J Physiol 2009; 587:3523 - 38; http://dx.doi.org/10.1113/jphysiol.2009.172577; PMID: 19470774
- Proske U, Gandevia SC. The proprioceptive senses: their roles in signaling body shape, body position and movement, and muscle force. Physiol Rev 2012; 92:1651 - 97; http://dx.doi.org/10.1152/physrev.00048.2011; PMID: 23073629
- Cattaert D, Le Bon M, Le Ray D. Efferent controls in crustacean mechanoreceptors. Microsc Res Tech 2002; 58:312 - 24; http://dx.doi.org/10.1002/jemt.10139; PMID: 12214298
- Sherrington CS. Notes on the arrangement of motor fibres in the lumbo-sacral plexus. J Physiol 1892; 13:621 - , 17; PMID: 16992009
- Sherrington CS. Cutaneous sensations. In Schäffer EA ed. Textbook of Physiology. Edinburgh: Pentland Press, 1900: 920–1001.
- Barrington FJ. The effect of division of the hypogastric nerves on frequency of micturition. Exp Physiol 1915; 9:261 - 4
- Barrington FJ. The effect of lesions of the hind- and mid-brain on micturition in the cat. Quart J Exp Physiol Cogn Med 1925; 15:81 - 102
- Barrington FJ. The component reflexes of micturition in the cat. Part I. Brain 1931; 54:177 - 88; http://dx.doi.org/10.1093/brain/54.2.177
- Barrington FJ. The component reflexes of micturition in the cat. Part II. Brain 1941; 64:239 - 43; http://dx.doi.org/10.1093/brain/64.4.239
- Garry RC, Roberts TD, Todd JK. Reflexes involving the external urethral sphincter in the cat. J Physiol 1959; 149:653 - 65; PMID: 13826682
- Gjone R. Peripheral autonomic influence on the motility of the urinary bladder in the cat. I. Rhythmic contractions. Acta Physiol Scand 1965; 65:370 - 7; http://dx.doi.org/10.1111/j.1748-1716.1965.tb04287.x; PMID: 5880505
- Satchell P, Vaughan C. Hypogastric nerve activity to the feline bladder during slow filling. J Auton Nerv Syst 1988; 25:41 - 7; http://dx.doi.org/10.1016/0165-1838(88)90006-9; PMID: 3225381
- Mitsui T, Kakizaki H, Matsuura S, Ameda K, Yoshioka M, Koyanagi T. Afferent fibers of the hypogastric nerves are involved in the facilitating effects of chemical bladder irritation in rats. J Neurophysiol 2001; 86:2276 - 84; PMID: 11698518
- Cervero F. Sensory innervation of the viscera: peripheral basis of visceral pain. Physiol Rev 1994; 74:95 - 138; PMID: 8295936
- De Wachter S, Wyndaele JJ. How sudden is a compelling desire to void? An observational cystometric study on the suddenness of this sensation. BJU Int 2008; 101:1000 - 3; http://dx.doi.org/10.1111/j.1464-410X.2007.07422.x; PMID: 18218058
- Iggo A. Tension receptors in the stomach and the urinary bladder. J Physiol 1955; 128:593 - 607; PMID: 13243351
- Morrison J. The activation of bladder wall afferent nerves. Exp Physiol 1999; 84:131 - 6; PMID: 10081713
- Häbler HJ, Jänig W, Koltzenburg M. Myelinated primary afferents of the sacral spinal cord responding to slow filling and distension of the cat urinary bladder. J Physiol 1993; 463:449 - 60; PMID: 8246192
- Janig CH,. Maziéres L, Lindström S. Cold- and menthol-sensitive C afferents of cat urinary bladder. J Physiol 2002; 543:211 - 20; http://dx.doi.org/10.1113/jphysiol.2002.019042; PMID: 12181293
- Morrison J, Steers WD, Brading A, Blok B, Fry C, de Groat WC, et al. 2002. Neurophysiology and neuropharmacology. In Abrams P,Cardozo L, Khoury S, Wein A (Eds) Incontinence health Publications Ltd, Jersey pp 83-164.
- Bahns EU, Ernsberger JW, Nelke A. Functional- characteristics of lumbar visceral afferent- fibers from the urinary bladder and the urethra in the cat. Pflugers Archiv- European. J Physiol 1986; 407:510 - 8
- Lawson SN, Perry MJ, Prabhakar E, McCarthy PW. Primary sensory neurones: neurofilament, neuropeptides, and conduction velocity. Brain Res Bull 1993; 30:239 - 43; http://dx.doi.org/10.1016/0361-9230(93)90250-F; PMID: 7681350
- Sann H, McCarthy PW, Mäder M, Schemann M. Choline acetyltransferase-like immunoreactivity in small diameter neurones of the rat dorsal root ganglion. Neurosci Lett 1995; 198:17 - 20; http://dx.doi.org/10.1016/0304-3940(95)11951-R; PMID: 8570086
- Ho C, Zhao J, Malinowski S, Chahine M, O’Leary ME. Differential expression of sodium channel β subunits in dorsal root ganglion sensory neurons. J Biol Chem 2012; 287:15044 - 53; http://dx.doi.org/10.1074/jbc.M111.333740; PMID: 22408255
- Mori K. Lectin Ulex europaeus agglutinin I specifically labels a subset of primary afferent fibers which project selectively to the superficial dorsal horn of the spinal cord. Brain Res 1986; 365:404 - 8; http://dx.doi.org/10.1016/0006-8993(86)91659-8; PMID: 3081237
- Zagorodnyuk VP, Costa M, Brookes SJ. Major classes of sensory neurons to the urinary bladder. Auton Neurosci 2006; 126-127:390 - 7; http://dx.doi.org/10.1016/j.autneu.2006.02.007; PMID: 16581309
- Xu L, Gebhart GF. Characterization of mouse lumbar splanchnic and pelvic nerve urinary bladder mechanosensory afferents. J Neurophysiol 2008; 99:244 - 53; http://dx.doi.org/10.1152/jn.01049.2007; PMID: 18003875
- Gabella G, Davis C. Distribution of afferent axons in the bladder of rats. J Neurocytol 1998; 27:141 - 55; http://dx.doi.org/10.1023/A:1006903507321; PMID: 10640174
- Gillespie JI, van Koeveringe GA, de Wachter SG, de Vente J. On the origins of the sensory output from the bladder: the concept of afferent noise. BJU Int 2009; 103:1324 - 33; http://dx.doi.org/10.1111/j.1464-410X.2009.08377.x; PMID: 19344428
- Fowler CJ, Griffiths D, de Groat WC. The neural control of micturition. Nat Rev Neurosci 2008; 9:453 - 66; http://dx.doi.org/10.1038/nrn2401; PMID: 18490916
- Griffiths D. Imaging bladder sensations. Neurourol Urodyn 2007; 26:Suppl 899 - 903; http://dx.doi.org/10.1002/nau.20488; PMID: 17654564
- Melzack R, Wall PD. Pain mechanisms: a new theory. Science 1965; 150:971 - 9; http://dx.doi.org/10.1126/science.150.3699.971; PMID: 5320816
- Gilmore NJ, Vane JR. Hormones released into the circulation when the urinary bladder of the anaesthetized dog is distended. Clin Sci 1971; 41:69 - 83; PMID: 5559114
- Ferguson DR, Kennedy I, Burton TJ. ATP is released from rabbit urinary bladder epithelial cells by hydrostatic pressure changes--a possible sensory mechanism?. J Physiol 1997; 505:503 - 11; http://dx.doi.org/10.1111/j.1469-7793.1997.503bb.x; PMID: 9423189
- Birder LA, Apodaca G, De Groat WC, Kanai AJ. Adrenergic- and capsaicin-evoked nitric oxide release from urothelium and afferent nerves in urinary bladder. Am J Physiol 1998; 275:F226 - 9; PMID: 9691011
- Yoshida M, Miyamae K, Iwashita H, Otani M, Inadome A. Management of detrusor dysfunction in the elderly: changes in acetylcholine and adenosine triphosphate release during aging. Urology 2004; 63:Suppl 1 17 - 23; http://dx.doi.org/10.1016/j.urology.2003.11.003; PMID: 15013648
- Birder LA. Role of the urothelium in urinary bladder dysfunction following spinal cord injury. Prog Brain Res 2006; 152:135 - 46; http://dx.doi.org/10.1016/S0079-6123(05)52009-0; PMID: 16198698
- Apodaca G, Balestreire E, Birder LA. The uroepithelial-associated sensory web. Kidney Int 2007; 72:1057 - 64; http://dx.doi.org/10.1038/sj.ki.5002439; PMID: 17667988
- Birder LA. Urothelial signaling. Auton Neurosci 2010; 153:33 - 40; http://dx.doi.org/10.1016/j.autneu.2009.07.005; PMID: 19666243
- Rong W, Spyer KM, Burnstock G. Activation and sensitisation of low and high threshold afferent fibres mediated by P2X receptors in the mouse urinary bladder. J Physiol 2002; 541:591 - 600; http://dx.doi.org/10.1113/jphysiol.2001.013469; PMID: 12042363
- Persson K, Igawa Y, Mattiasson A, Andersson KE. Effects of inhibition of the L-arginine/nitric oxide pathway in the rat lower urinary tract in vivo and in vitro. Br J Pharmacol 1992; 107:178 - 84; http://dx.doi.org/10.1111/j.1476-5381.1992.tb14483.x; PMID: 1422571
- Nile CJ, Gillespie JI. Interactions between cholinergic and prostaglandin signaling elements in the urothelium: role for muscarinic type 2 receptors. Urology 2012; 79:e17 - 23; http://dx.doi.org/10.1016/j.urology.2011.08.029; PMID: 22055690
- Starling EH. Elements of Human Physiology, Seventh edition, Churchill, London, 1905.
- Gillespie JI. The autonomous bladder: a view of the origin of bladder overactivity and sensory urge. BJU Int 2004; 93:478 - 83; http://dx.doi.org/10.1111/j.1464-410X.2003.04667.x; PMID: 15008713
- Streng T, Hedlund P, Talo A, Andersson K-E, Gillespie JI. Phasic non-micturition contractions in the bladder of the anaesthetized and awake rat. BJU Int 2006; 97:1094 - 101; http://dx.doi.org/10.1111/j.1464-410X.2006.06137.x; PMID: 16643498
- Klevmark B. Motility of the urinary bladder in cats during filling at physiological rates. I. Intravesical pressure patterns studied by a new method of cystometry. Acta Physiol Scand 1974; 90:565 - 77; http://dx.doi.org/10.1111/j.1748-1716.1974.tb05621.x; PMID: 4830420
- Langworthy OR. Innervation of the pelvic organs of the rat. Invest Urol 1965; 2:491 - 511; PMID: 14261791
- Vaughan CW, Satchell PM. Urine storage mechanisms. Prog Neurobiol 1995; 46:215 - 37; PMID: 7568914
- Tyagi P, Tyagi V. Mirabegron, a β₃-adrenoceptor agonist for the potential treatment of urinary frequency, urinary incontinence or urgency associated with overactive bladder. IDrugs 2010; 13:713 - 22; PMID: 20878594
- Finney SM, Andersson K-E, Gillespie JI, Stewart LH. Antimuscarinic drugs in detrusor overactivity and the overactive bladder syndrome: motor or sensory actions?. BJU Int 2006; 98:503 - 7; http://dx.doi.org/10.1111/j.1464-410X.2006.06258.x; PMID: 16925744
- Finney SM, Stewart LH, Gillespie JI. Cholinergic activation of phasic activity in the isolated bladder: possible evidence for M3- and M2-dependent components of a motor/sensory system. BJU Int 2007; 100:668 - 78; http://dx.doi.org/10.1111/j.1464-410X.2007.07021.x; PMID: 17627783
- Malone-Lee J, Henshaw DJ, Cummings K. Urodynamic verification of an overactive bladder is not a prerequisite for antimuscarinic treatment response. BJU Int 2003; 92:415 - 7; http://dx.doi.org/10.1046/j.1464-410X.2003.04351.x; PMID: 12930431
- Gillespie JI. Modulation of autonomous contractile activity in the isolated whole bladder of the guinea pig. BJU Int 2004; 93:393 - 400; http://dx.doi.org/10.1111/j.1464-410X.2003.04624.x; PMID: 14764145
- de Jongh R, van Koeveringe GA, van Kerrebroeck PE, Markerink-van Ittersum M, de Vente J, Gillespie JI. The effects of exogenous prostaglandins and the identification of constitutive cyclooxygenase I and II immunoreactivity in the normal guinea pig bladder. BJU Int 2007; 100:419 - 29; http://dx.doi.org/10.1111/j.1464-410X.2007.07011.x; PMID: 17617145
- Gillespie JI, Drake MJ. The actions of sodium nitroprusside and the phosphodiesterase inhibitor dipyridamole on phasic activity in the isolated guinea-pig bladder. BJU Int 2004; 93:851 - 8; http://dx.doi.org/10.1111/j.1464-410X.2003.04727.x; PMID: 15050004
- Gillespie JI. Inhibitory actions of calcitonin gene-related peptide and capsaicin: evidence for local axonal reflexes in the bladder wall. BJU Int 2005; 95:149 - 56; http://dx.doi.org/10.1111/j.1464-410X.2005.05268.x; PMID: 15638914
- Gillespie JI. Noradrenaline inhibits autonomous activity in the isolated guinea pig bladder. BJU Int 2004; 93:401 - 9; http://dx.doi.org/10.1111/j.1464-410X.2003.04626.x; PMID: 14764146
- Lagou M, Drake MJ, Gillespie JI. Volume-induced effects on the isolated bladder: a possible local reflex. BJU Int 2004; 94:1356 - 65; http://dx.doi.org/10.1111/j.1464-410X.2004.05113.x; PMID: 15610121
- Gillespie JI, Markerink-van Ittersum M, de Vente J. Sensory collaterals, intramural ganglia and motor nerves in the guinea-pig bladder: evidence for intramural neural circuits. Cell Tissue Res 2006; 325:33 - 45; http://dx.doi.org/10.1007/s00441-006-0166-8; PMID: 16525831
- Gabella G. Intramural neurons in the urinary bladder of the guinea-pig. Cell Tissue Res 1990; 261:231 - 7; http://dx.doi.org/10.1007/BF00318664; PMID: 2401001
- Langley JN, Anderson HK. The innervations of the pelvic and adjoining viscera-2-The bladder. J Physiol 1895; a 19:71 - 84; PMID: 16992335
- de Groat WC, Booth AM. Synaptic transmission in pelvic ganglia. Nervous Control of the Urogenital System. Ed Maggi CA, Harwood Academic Publishers; 1993; 291-347.
- Dail WG, Dziurzynski R. Substance P immunoreactivity in the major pelvic ganglion of the rat. Anat Rec 1985; 212:103 - 9; http://dx.doi.org/10.1002/ar.1092120115; PMID: 2416248
- Papka RE. Some nerve endings in the rat pelvic paracervical autonomic ganglia and varicosities in the uterus contain calcitonin gene-related peptide and originate from dorsal root ganglia. Neuroscience 1990; 39:459 - 70; http://dx.doi.org/10.1016/0306-4522(90)90282-9; PMID: 2128374
- Keast JR. Visualization and immunohistochemical characterization of sympathetic and parasympathetic neurons in the male rat major pelvic ganglion. Neuroscience 1995; 66:655 - 62; http://dx.doi.org/10.1016/0306-4522(94)00595-V; PMID: 7644029
- Keast JR, Booth AM, de Groat WC. Distribution of neurons in the major pelvic ganglion of the rat which supply the bladder, colon or penis. Cell Tissue Res 1989; 256:105 - 12; http://dx.doi.org/10.1007/BF00224723; PMID: 2713886
- Hamberger B, Norberg KA. Adrenergic synaptic terminals and nerve cells in bladder ganglia of the cat. Int J Neuropharmacol 1965; 4:41 - 5; http://dx.doi.org/10.1016/0028-3908(65)90045-6; PMID: 14346149
- Félix B, Catalin D, Miolan JP, Niel JP. Integrative properties of the major pelvic ganglion in the rat. J Auton Nerv Syst 1998; 69:6 - 11; http://dx.doi.org/10.1016/S0165-1838(97)00133-1; PMID: 9672118
- Marrazzi AS, Marrazzi RN. Further localization and analysis of adrenergic synaptic inhibition. J Neurophysiol 1947; 10:167 - 78; PMID: 20239771
- Blackman JG, Crowcroft PJ, Devine CE, Holman ME, Yonemura K. Transmission from pregnanglionic fibres in the hypogastric nerve to peripheral ganglia of male guinea-pigs. J Physiol 1969; 201:723 - 43; PMID: 4305798