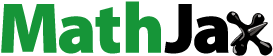
Abstract
The present report is an extension of our preceding publication in Biomaterials (2013) entitled “Effect of RGD nanospacing on differentiation of stem cells.” Cell-adhesive peptide arginine-glycine-aspartate (RGD) was nanopatterned on a non-fouling poly(ethylene glycol) (PEG) hydrogel, and mesenchymal stem cells (MSCs) derived from rat bone marrow were cultured on the patterned surfaces at nanospacings from 37 to 124 nm. Cell adhesion parameters such as spreading areas varied with RGD nanospacings significantly. The differences were well observed at both the first and eighth days, which confirmed the persistence of this nanospacing effect on our nanopatterns. The proliferation rate also varied with the nanospacings. Osteogenic and adipogenic inductions were undertaken, and a significant influence of RGD nanospacing on stem cell differentiation was found. The effect on differentiation cannot be simply interpreted by differences in cell adhesion and proliferation. We further calculated the fractions of single, coupled, and multiple cells on those nanopatterns, and ruled out the possibility that the extent of cell-cell contact determined the different differentiation fractions. Accordingly, we reinforced the idea that RGD nanospacing might directly influence stem cell differentiation.
Introduction
Stem cells play a vital role in organogenesis.Citation1,Citation2 In tissue engineering and regenerative medicine, stem cells are usually loaded into biomaterials instead of being used alone.Citation3–Citation8 Ideally, biomaterials should, to a certain extent, mimic the extracellular matrix (ECM) to promote cell adhesion, proliferation, and differentiation.Citation9–Citation13 Hence, interactions between cells and materials become a key fundamental topic.Citation14–Citation16 One of the core peptide sequences in ECM proteins is arginine-glycine-aspartate (RGD), which can be specifically bound to integrin, a receptor across the membrane.Citation17 The RGD motif has been usefully employed in cell research and biomaterial modification.Citation18–Citation21
Considering that the size of integrin is about 8 to 12 nm,Citation22 patterns with RGD peptides grafted onto nanodots of about 10 nm diameter are important when investigating the effect of spatial distribution of RGD ligands on cell behavior.Citation23 An ideal RGD nanopattern should be based upon a perfect non-fouling background. A hydrogel of poly(ethylene glycol) (PEG) affords such a background, and a transfer lithography technique has been proposed to generate gold patterns on PEG hydrogels.Citation24,Citation25 After grafting RGD-thiol ligands onto gold domains embedded in the PEG hydrogel, RGD patterns on a non-fouling background were obtained.
Although cell adhesion and migration on RGD nanopatterns have been extensively investigated,Citation23,Citation25 the behaviors of stem cells on those patterns have not yet been reported until 2013. Our group examined osteogenic and adipogenic differentiations of mesenchymal stem cells (MSCs) derived from the bone marrow of rats and found an underlying nanospacing effect.Citation26 The present report is an extension of our preceding publication.Citation26 A schematic presentation of this study is given in . Novelty is claimed as follows: (1) besides cell differentiation, adhesion of stem cells will be analyzed, and besides average values, the distributions of spreading area, circularity, and aspect ratio of cells on nanopatterns of varied nanospacings will be reported; (2) proliferation rates of stem cells on RGD nanopatterns will be examined; (3) we will compile statistics about single, coupled, and multiple cells on nanopatterns, to check whether or not cell-cell contact on nanopatterns of varied nanospacings can account for the origins of the nanospacing effect on stem cell differentiation.
Figure 1. Schematic presentation of stem cells on RGD nanopatterns with a non-fouling background. RGD ligands are grafted onto gold nanodots, which are covalently bound to a PEG hydrogel. Integrins as the receptors of RGD will be linked to the nanodots after cell culture. The pre-designed nanospacing then determines the nanospacing of integrins, and might regulate adhesion, proliferation, and differentiation of MSCs.
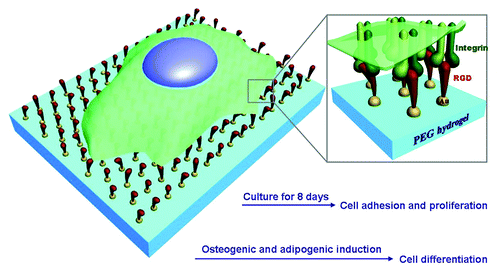
Preparation of RGD Nanopatterns on PEG Hydrogels
First, gold nanopatterns were prepared by block copolymer micelle nanolithography, and characterized by atomic force microscopy (AFM) (). The average size of gold particles was measured using their heights as a proxy, resulting in approximately 10 nm.
Figure 2. AFM images of gold nanopatterns on glass. The lower row shows the height profiles with respect to the lines in the upper images, indicating that the particle size was around 10 nm. From the left to right, the average nanospacings of gold particles are 34 nm, 48 nm, 70 nm, 79 nm, and 113 nm, respectively. Note: after the nanoarrays were transferred onto the surfaces of PEG hydrogels and then soaked in an aqueous environment to culture cells, the average nanospacings were enlarged to 37 nm, 53 nm, 77 nm, 87 nm, and 124 nm, respectively, considering the swelling ratio of the hydrogels as 1.1.
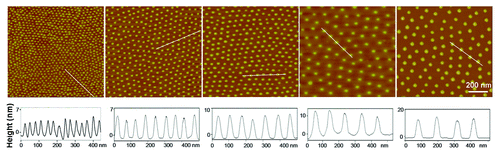
Then, the gold nanoarrays were transferred to the surfaces of PEG hydrogels, which provide a non-adhesive background. Finally, the RGD motifs were grafted onto the gold particles by way of an S-Au bond to obtain nanopatterns of RGD motifs. Since the size of an integrin molecule is about 10 nm,Citation22 this technique might achieve a one-to-one correspondence between a nanodot and an integrin receptor. The RGD patterns can thus regulate the spatial distribution of their receptor integrins.
Strong and Persistent Non-fouling of the PEG Hydrogel in Cell Culture Medium
The process of cell adhesion includes specific, and non-specific, adhesion.Citation27 To guarantee that cells adhere to the RGD nanopatterned surfaces mainly through bioconjugation of RGD ligands to integrins across the cell membrane, namely, specific adhesion, one should first confirm the persistent resistance of the non-specific adhesion of the substrate background. However, ensuring non-fouling conditions in the culture medium with serum for several days presents technical difficulties.
Herein, we took advantage of the strong and persistent non-fouling property of PEG hydrogels, which has been confirmed both in vitro and in vivo.Citation28–Citation32 The persistent non-fouling property of the nanopatterned substrate could be well reflected by avoiding cell adhesion beyond what we termed the dip-line in our experiments. Here the dip-line is the border between regions with and without gold nanoarrays formed during the dip-coating process, in which glass coverslips were dipped into, and then pulled out of, a solution of block copolymer micelles loaded with HAuCl4. The resulting substrate surface thus contained a nanopatterned region and a non-nanopatterned region separated by the dip-line. Fluorescence images of cells revealed that cells preferred to adhere to the nanopatterned region (). Even after eight days of cell culture, MSCs still stayed inside the patterned region and rarely migrated to the unpatterned region. It indicated that the PEG hydrogel afforded a strong and persistent anti-adhesive background, and therefore cells were immobilised on the RGD-nanopatterned region just by bioconjugation between RGD ligands on the substrate and integrins in the cell membrane.
Distributions of Adhesion Parameters of Stem Cells on RGD Nanopatterns
As the RGD nanospacing increased, the mean of the spreading area of the stem cells decreased, the mean cell circularity increased, and the mean cell aspect ratio did not change in any regular way according to our previous report.Citation26 Herein, their distributions were further calculated. We measured over one hundred cells from each nanopattern of different nanospacings using an image processing program (ImageJ, freely available from: www.nih.gov). The resultant distributions of cell spreading parameters are shown in . The peak values of cell area, cell circularity, and aspect ratio changed with the same trend as their average values. The effect of RGD nanospacing on stem cell adhesion was thus strengthened.
Proliferation of Stem Cells on RGD Nanopatterns
While all previous studies of non-stem cells on RGD nanopatterns were examined within 24 h, we checked the adhesion until the eighth day, by which time there were large numbers of cells. Some of cells even peeled off if overcrowded, which led to difficulty when examining cell differentiation. So, we added a cell-division inhibitor (aphidicolin, 0.5 μg/mL) to our cell culture. Nevertheless, cell proliferation could still be observed, as seen from the comparison between densities of cells cultured in growth medium for 8 d (GM 8 d) and 1 d (GM 1 d), and also from the corresponding data in the insert to . The proliferation rate decreased slightly with RGD nanospacing except for a significant increase at very large nanospacings, where the low initial density of the adherent cells may play a role.
Figure 5. Densities of MSCs adhering on nanopatterned surfaces of indicated RGD nanospacings. Cells were cultured in growth medium for 1 d (GM 1 d) or 8 d (GM 8 d), or cells underwent one-day culture in GM followed by 7 d in osteogenic medium (OM 1+7 d) or adipogenic medium (AM 1 + 7 d). In all cases, the cell-division inhibitor aphidicolin was added at a concentration of 0.5 μg/mL. Insets show proliferation rates calculated by cell density at day 8 over that at day 1. The error bars of the proliferation rates were obtained by an error transfer formula (see Materials and methods).
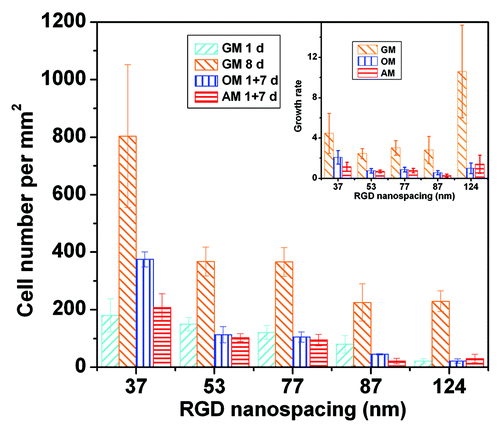
The presence of induction agents slowed down cell proliferation, as also seen in . The trend is consistent with the well-known principle that a stem cell does not proliferate and undergo differentiation simultaneously.Citation33–Citation36 The proliferation rates were quite small in presence of both induction medium and 0.5 μg/mL aphidicolin, which made the forthcoming examination of cell differentiation less subject to interference and thus more convincing.
Differentiation of Stem Cells on RGD Nanopatterns of Different Nanospacings
After one day of adhesion in growth medium, osteogenic, and adipogenic inductions of stem cells lasted for 7 d. Cells were then stained to enable later counting to establish the extent of differentiation. Osteoblasts expressing alkaline phosphatase (ALP) were stained in blue, and adipocytes in red. Due to space limitations we do not show all images of the stained cells experiencing osteogenic or adipogenic induction on different RGD nanopatterns. Typical images of stained cells on nanopatterns with RGD nanospacings of 37 nm and 87 nm are shown in . Relatively more stem cells were committed to osteoblasts and adipocytes on 37 nm-spaced nanopatterns than on 87 nm-spaced nanopatterns. The differentiation fractions at different nanospacings have been reported elsewhere.Citation26 Larger RGD nanospacings induced greater extents of both osteogenic and adipogenic differentiation.
Figure 6. Bright-field micrographs (upper row) and fluorescence micrographs (lower row) of MSCs on RGD nanopatterns of indicated nanospacings in osteogenic or adipogenic medium for 7 d. Before observation, the cells upon osteogenic induction were stained for ALP and nuclei, and those upon adipogenesis for oil microdroplets and nuclei. Both osteogenesis and adipogenesis were enhanced with RGD nanospacing.
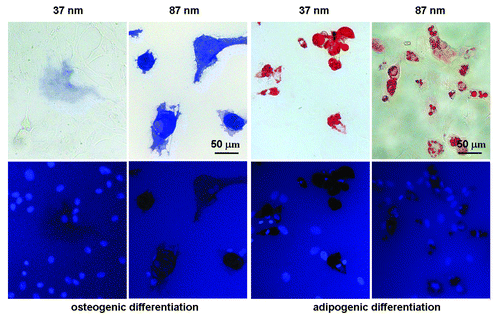
Cell Adhesion and Clustering Do Not Fully Explain the Nanospacing Effect on Cell Differentiation
It has been known from our examination of MSCs on microscale RGD patterns that adhesion parameters including cell density, spreading areas, and cell shape can influence cell differentiation.Citation15,Citation28,Citation37,Citation38 These factors have been excluded as the reasons to account for the nanospacing effect on differentiation of MSCs according to our previous analysis.Citation26
Besides adhesion parameters, cell-cell contact has also been recognized as regulating stem cell differentiation.Citation28,Citation39 Herein, cells on patterns of large RGD nanospacings did not spread well and may even shrink and aggregate into cell clusters. So the extent of cell-cell contact changed with RGD nanospacing. We counted the numbers of isolated single cells and clustered cells that came into contacted with others. The statistical results indicated that the fraction of single and coupled cell numbers increased with the RGD nanospacing, the fraction of clusters containing three or more cells decreased, and cell clusters were predominant under our experimental conditions (). So, the extent of cell-cell contact became weaker with the increase of RGD nanospacing. While cell-cell contact is beneficial for both osteogenic and adipogenic differentiations,Citation39 the increase of nanospacing enhanced the two lineage commitments. Thus the change of cell-cell contact extent cannot explain the differentiation trend of stem cells. The present study implies that the RGD nanospacing inherently regulates stem cell differentiation beyond cell adhesion.
Concluding Remarks and Perspectives
In conclusion, a series of nanopatterns of RGD ligands were fabricated on a non-fouling PEG hydrogel: stem cells on the patterns of varied RGD nanospacings exhibited different adhesion, proliferation, and differentiation behaviors. The increased nanospacing led to a smaller cell density and less cell spreading. Cell proliferation decelerated in the presence of the induction agent and division inhibitor. A larger RGD nanospacing was beneficial for both osteogenesis and adipogenesis of MSCs. The differences in cell differentiation can be explained by neither cell spreading, shaping, adherent density, nor cell clustering. RGD nanospacing may directly regulate cell differentiation.
The universality of this principle is ready to be checked by examination of other stem cell types and even some non-stem cells with differentiation abilities such as pre-osteoblasts. The mechanism of the nano-phenomenon remains a challenging topic. The nanospacing effects on cell adhesion, proliferation, and differentiation are potentially stimulating for the precise design of new-generation biomaterials.
Materials and Methods
Preparation and characterization of nanopatterns
Gold nanopatterns on glass surfaces were prepared following our previous protocol.Citation26 Before being transferred to PEG hydrogels, the gold nanoarrays on glass were characterized with AFM (Multimode IV, Bruker) under tapping mode. For each group of patterns of a given nanospacing, six samples were randomly selected from the population of 100 samples. For each sample, three regions were randomly selected for scanning, and the nanodot spacings were measured and averaged. Before cell culture, c(-RGDfK-)-thiol ligands (f: d-phenylalanine, K: l-lysine; Peptides International) were grafted onto gold nanodots.
Culture and induction of stem cells
Primary MSCs were isolated from marrows of tibia and femur of 7-d Sprague-Dawley (SD) rats. The MSCs of the second passage were seeded on RGD nanopatterned surfaces at a density of 6600 cells per cm2. We cultured the cells in the growth medium (GM) for both 1 d and 8 d to test cell densities and spreading parameters. The GM was composed of low-glucose Dulbecco’s modified Eagle medium (DMEM, Gibco) supplemented with 10% fetal bovine serum (FBS, Gibco). In the process of 8-d culture, the culture medium was changed on the first, fourth, and sixth days. For osteogenic and adipogenic inductions, the culture medium was changed to the induction medium after cells adhered in the GM for 1 d. The following induction lasted for 7 d. The osteogenic induction medium (OM) was composed of high-glucose DMEM, 10% FBS, 50 μM ascorbic acid-2-phosphate, 10 mM β-glycerophosphate, and 100 nM dexamethasone (Sigma). The OM was changed on the third and fifth days of induction. The adipogenic induction medium (AM) was composed of high-glucose DMEM, 10% FBS, 1 mM dexamethasone, 200 mM indomethacin, 10 mg/mL insulin, and 0.5 mM methylisobutylxanthine (Sigma). On the fourth and fifth days of the adipogenic induction, cells were cultured in a maintenance medium composed of high-glucose DMEM, 10% FBS, and 10 mg/mL insulin. In all cases, aphidicolin was added from the fourth to fifth day of cell culture or cell induction to diminish interference with cell proliferation.
Staining of cells and statistics of differentiation extents
After 1 d and 8 d of culture, cytoskeletons and nuclei of cells were fluorescently stained, with F-actins labeled by phalloidin-TRITC (Sigma) and nuclei by 4´,6-diamidino-2-phenylindole (DAPI, Sigma).
For osteogenically induced cells, ALP as an indicator of osteoblasts was stained with Fast Blue RR (Sigma) to reflect the extent of osteogenic differentiation; for adipogenically induced cells, lipid droplets as an indicator of adipocytes were stained with Oil Red O (Sigma) to characterize adipogenic differentiation.
All the stained cells were observed under an inverted microscope (Axiovert 200, Zeiss). Images at different magnifications were taken with a charge coupled device (CCD, AxioCam HRC, Zeiss).
The positively stained cells were counted. Then, the osteogenic and adipogenic differentiation extents of MSCs on nanopatterned surfaces were calculated.
Statistics of cell densities and spreading parameters
Cell densities were obtained by counting the numbers of cell nuclei in fluorescence images of cells stained by DAPI. Cell areas, aspect ratios (ARs), and circularities were measured by outlining cells in fluorescence images with ImageJ (freely available from: www.nih.gov). AR is defined as the ratio between the two principal axes, which were obtained from the two eigenvalues of the corresponding cell profile calculated by the ImageJ software. Circularity is quantified by area multiplied by 4π divided by the square of the perimeter.
Calculation of proliferation rates
The proliferation rate or growth rate G was reflected simply by cell density at day 8 (D8) over that at day 1 (D1).Citation40,Citation41 The error bound on G was obtained by the following transfer formula
where s denotes the corresponding standard deviation.
Abbreviations: | ||
RGD | = | arginine-glycine-aspartate |
PEG | = | poly(ethylene glycol) |
MSC | = | mesenchymal stem cells |
ECM | = | extracellular matrix |
AFM | = | atomic force microscope |
ALP | = | alkaline phosphatase |
DAPI | = | 4´,6-diamidino-2-phenylindole |
PS-b-P2VP | = | polystyrene-block-poly(2-vinylpyridine) |
F-actins | = | filamentous actins |
CCD | = | charge coupled device |
Disclosure of Potential Conflicts of Interest
No potential conflicts of interest were disclosed.
Acknowledgments
The authors are grateful for financial support from The Chinese Ministry of Science and Technology (973 Program No. 2009CB930000 and No. 2011CB606203), The NSF of China (Grants No. 21034002, No. 91127028, and No. 51273046), and The Science and Technology Developing Foundation of Shanghai (Grant No. 13XD1401000).
References
- Thirumala S, Goebel WS, Woods EJ. Clinical grade adult stem cell banking. Organogenesis 2009; 5:143 - 54; http://dx.doi.org/10.4161/org.5.3.9811; PMID: 20046678
- Klemmt P. Application of amniotic fluid stem cells in basic science and tissue regeneration. Organogenesis 2012; 8:76; http://dx.doi.org/10.4161/org.23023; PMID: 23187723
- Lagasse E, Connors H, Al-Dhalimy M, Reitsma M, Dohse M, Osborne L, Wang X, Finegold M, Weissman IL, Grompe M. Purified hematopoietic stem cells can differentiate into hepatocytes in vivo. Nat Med 2000; 6:1229 - 34; http://dx.doi.org/10.1038/81326; PMID: 11062533
- Orlic D, Kajstura J, Chimenti S, Limana F, Jakoniuk I, Quaini F, Nadal-Ginard B, Bodine DM, Leri A, Anversa P. Mobilized bone marrow cells repair the infarcted heart, improving function and survival. Proc Natl Acad Sci U S A 2001; 98:10344 - 9; http://dx.doi.org/10.1073/pnas.181177898; PMID: 11504914
- Karageorgiou V, Kaplan D. Porosity of 3D biomaterial scaffolds and osteogenesis. Biomaterials 2005; 26:5474 - 91; http://dx.doi.org/10.1016/j.biomaterials.2005.02.002; PMID: 15860204
- Rustad KC, Sorkin M, Levi B, Longaker MT, Gurtner GC. Strategies for organ level tissue engineering. Organogenesis 2010; 6:151 - 7; http://dx.doi.org/10.4161/org.6.3.12139; PMID: 21197216
- Pan Z, Ding JD. Poly(lactide-co-glycolide) porous scaffolds for tissue engineering and regenerative medicine. Interface Focus 2012; 2:366 - 77; http://dx.doi.org/10.1098/rsfs.2011.0123; PMID: 23741612
- Park D, Spencer JA, Koh BI, Kobayashi T, Fujisaki J, Clemens TL, Lin CP, Kronenberg HM, Scadden DT. Endogenous bone marrow MSCs are dynamic, fate-restricted participants in bone maintenance and regeneration. Cell Stem Cell 2012; 10:259 - 72; http://dx.doi.org/10.1016/j.stem.2012.02.003; PMID: 22385654
- Langer R, Tirrell DA. Designing materials for biology and medicine. Nature 2004; 428:487 - 92; http://dx.doi.org/10.1038/nature02388; PMID: 15057821
- Lutolf MP, Hubbell JA. Synthetic biomaterials as instructive extracellular microenvironments for morphogenesis in tissue engineering. Nat Biotechnol 2005; 23:47 - 55; http://dx.doi.org/10.1038/nbt1055; PMID: 15637621
- Graeter SV, Huang JH, Perschmann N, López-García M, Kessler H, Ding JD, Spatz JP. Mimicking cellular environments by nanostructured soft interfaces. Nano Lett 2007; 7:1413 - 8; http://dx.doi.org/10.1021/nl070098g; PMID: 17394372
- Nichols JE, Niles JA, Cortiella J. Design and development of tissue engineered lung: Progress and challenges. Organogenesis 2009; 5:57 - 61; http://dx.doi.org/10.4161/org.5.2.8564; PMID: 19794900
- Zhang G. Biomimicry in biomedical research. Organogenesis 2012; 8:101 - 2; http://dx.doi.org/10.4161/org.23395; PMID: 23275257
- Sun JG, Tang J, Ding JD. Cell orientation on a stripe-micropatterned surface. Chin Sci Bull 2009; 54:3154 - 9; http://dx.doi.org/10.1007/s11434-009-0240-1
- Peng R, Yao X, Ding JD. Effect of cell anisotropy on differentiation of stem cells on micropatterned surfaces through the controlled single cell adhesion. Biomaterials 2011; 32:8048 - 57; http://dx.doi.org/10.1016/j.biomaterials.2011.07.035; PMID: 21810538
- Pan Z, Yan C, Peng R, Zhao YC, He Y, Ding JD. Control of cell nucleus shapes via micropillar patterns. Biomaterials 2012; 33:1730 - 5; http://dx.doi.org/10.1016/j.biomaterials.2011.11.023; PMID: 22133552
- Humphries JD, Byron A, Humphries MJ. Integrin ligands at a glance. J Cell Sci 2006; 119:3901 - 3; http://dx.doi.org/10.1242/jcs.03098; PMID: 16988024
- Hersel U, Dahmen C, Kessler H. RGD modified polymers: biomaterials for stimulated cell adhesion and beyond. Biomaterials 2003; 24:4385 - 415; http://dx.doi.org/10.1016/S0142-9612(03)00343-0; PMID: 12922151
- Zhang Z, Lai YX, Yu L, Ding JD. Effects of immobilizing sites of RGD peptides in amphiphilic block copolymers on efficacy of cell adhesion. Biomaterials 2010; 31:7873 - 82; http://dx.doi.org/10.1016/j.biomaterials.2010.07.014; PMID: 20674012
- Bellis SL. Advantages of RGD peptides for directing cell association with biomaterials. Biomaterials 2011; 32:4205 - 10; http://dx.doi.org/10.1016/j.biomaterials.2011.02.029; PMID: 21515168
- Zhang Z, Ni J, Chen L, Yu L, Xu JW, Ding JD. Encapsulation of cell-adhesive RGD peptides into a polymeric physical hydrogel to prevent postoperative tissue adhesion. J Biomed Mater Res B Appl Biomater 2012; 100:1599 - 609; http://dx.doi.org/10.1002/jbm.b.32728; PMID: 22692943
- Xiong JP, Stehle T, Zhang RG, Joachimiak A, Frech M, Goodman SL, Arnaout MA. Crystal structure of the extracellular segment of integrin alpha Vbeta3 in complex with an Arg-Gly-Asp ligand. Science 2002; 296:151 - 5; http://dx.doi.org/10.1126/science.1069040; PMID: 11884718
- Huang JH, Ding JD. Nanostructured interfaces with RGD arrays to control cell-matrix interaction. Soft Matter 2010; 6:3395 - 401; http://dx.doi.org/10.1039/b927168f
- Sun JG, Graeter SV, Yu L, Duan SF, Spatz JP, Ding JD. Technique of surface modification of a cell-adhesion-resistant hydrogel by a cell-adhesion-available inorganic microarray. Biomacromolecules 2008; 9:2569 - 72; http://dx.doi.org/10.1021/bm800477s; PMID: 18646821
- Huang JH, Grater SV, Corbellini F, Rinck S, Bock E, Kemkemer R, Kessler H, Ding J, Spatz JP. Impact of order and disorder in RGD nanopatterns on cell adhesion. Nano Lett 2009; 9:1111 - 6; http://dx.doi.org/10.1021/nl803548b; PMID: 19206508
- Wang X, Yan C, Ye K, He Y, Li ZH, Ding JD. Effect of RGD nanospacing on differentiation of stem cells. Biomaterials 2013; 34:2865 - 74; http://dx.doi.org/10.1016/j.biomaterials.2013.01.021; PMID: 23357372
- Lai YX, Xie C, Zhang Z, Lu WY, Ding JD. Design and synthesis of a potent peptide containing both specific and non-specific cell-adhesion motifs. Biomaterials 2010; 31:4809 - 17; http://dx.doi.org/10.1016/j.biomaterials.2010.02.064; PMID: 20346502
- Peng R, Yao X, Cao B, Tang J, Ding JD. The effect of culture conditions on the adipogenic and osteogenic inductions of mesenchymal stem cells on micropatterned surfaces. Biomaterials 2012; 33:6008 - 19; http://dx.doi.org/10.1016/j.biomaterials.2012.05.010; PMID: 22681981
- Kingshott P, Griesser HJ. Surfaces that resist bioadhesion. Curr Opin Solid State Mater Sci 1999; 4:403 - 12; http://dx.doi.org/10.1016/S1359-0286(99)00018-2
- Yu L, Ding JD. Injectable hydrogels as unique biomedical materials. Chem Soc Rev 2008; 37:1473 - 81; http://dx.doi.org/10.1039/b713009k; PMID: 18648673
- Zhang Z, Ni J, Chen L, Yu L, Xu JW, Ding JD. Biodegradable and thermoreversible PCLA-PEG-PCLA hydrogel as a barrier for prevention of post-operative adhesion. Biomaterials 2011; 32:4725 - 36; http://dx.doi.org/10.1016/j.biomaterials.2011.03.046; PMID: 21482434
- Yan C, Sun JG, Ding JD. Critical areas of cell adhesion on micropatterned surfaces. Biomaterials 2011; 32:3931 - 8; http://dx.doi.org/10.1016/j.biomaterials.2011.01.078; PMID: 21356556
- Walsh K, Perlman H. Cell cycle exit upon myogenic differentiation. Curr Opin Genet Dev 1997; 7:597 - 602; http://dx.doi.org/10.1016/S0959-437X(97)80005-6; PMID: 9388774
- Jakoby M, Schnittger A. Cell cycle and differentiation. Curr Opin Plant Biol 2004; 7:661 - 9; http://dx.doi.org/10.1016/j.pbi.2004.09.015; PMID: 15491914
- Grossel MJ, Hinds PW. From cell cycle to differentiation: an expanding role for cdk6. Cell Cycle 2006; 5:266 - 70; http://dx.doi.org/10.4161/cc.5.3.2385; PMID: 16410727
- Buttitta LA, Edgar BA. Mechanisms controlling cell cycle exit upon terminal differentiation. Curr Opin Cell Biol 2007; 19:697 - 704; http://dx.doi.org/10.1016/j.ceb.2007.10.004; PMID: 18035529
- McBeath R, Pirone DM, Nelson CM, Bhadriraju K, Chen CS. Cell shape, cytoskeletal tension, and RhoA regulate stem cell lineage commitment. Dev Cell 2004; 6:483 - 95; http://dx.doi.org/10.1016/S1534-5807(04)00075-9; PMID: 15068789
- Yao X, Peng R, Ding JD. Effects of aspect ratios of stem cells on lineage commitments with and without induction media. Biomaterials 2013; 34:930 - 9; http://dx.doi.org/10.1016/j.biomaterials.2012.10.052; PMID: 23140997
- Tang J, Peng R, Ding JD. The regulation of stem cell differentiation by cell-cell contact on micropatterned material surfaces. Biomaterials 2010; 31:2470 - 6; http://dx.doi.org/10.1016/j.biomaterials.2009.12.006; PMID: 20022630
- Allred DC, Clark GM, Elledge R, Fuqua SAW, Brown RW, Chamness GC, Osborne CK, McGuire WL. Association of p53 protein expression with tumor cell proliferation rate and clinical outcome in node-negative breast cancer. J Natl Cancer Inst 1993; 85:200 - 6; http://dx.doi.org/10.1093/jnci/85.3.200; PMID: 8423624
- Peister A, Mellad JA, Larson BL, Hall BM, Gibson LF, Prockop DJ. Adult stem cells from bone marrow (MSCs) isolated from different strains of inbred mice vary in surface epitopes, rates of proliferation, and differentiation potential. Blood 2004; 103:1662 - 8; http://dx.doi.org/10.1182/blood-2003-09-3070; PMID: 14592819