Abstract
The intrasynovial bone-tendon interface is a gradual transition from soft tissue to bone, with two intervening zones of uncalcified and calcified fibrocartilage. Following injury, the native anatomy is not restored, resulting in inferior mechanical properties and an increased risk of re-injury. Recent in vivo studies provide evidence of improved healing when surgical repair of the bone-tendon interface is augmented with cells capable of undergoing chondrogenesis. In particular, cellular therapy in bone-tendon healing can promote fibrocartilage formation and associated improvements in mechanical properties. Despite these promising results in animal models, cellular therapy in human patients remains largely unexplored. This review highlights the development and structure-function relationship of normal bone-tendon insertions. The natural healing response to injury is discussed, with subsequent review of recent research on cellular approaches for improved healing. Finally, opportunities for translating in vivo findings into clinical practice are identified.
Introduction
Now in the third decade following its introduction by YC Fung and the subsequent publication of the seminal paper of Langer and Vacanti,Citation1 the field of tissue engineering still offers tremendous promise in allaying or eliminating multiple diseases. Perhaps no organ system is closer to broad application of tissue engineering strategies than that of the musculoskeletal system,Citation2 due in part to the relatively well-characterized structure and function of the these tissues, coupled with the prevalence of musculoskeletal disease. Annually, there are 32 million musculoskeletal injuries in the United States, of which 45% involve tendons or ligaments (T/L).Citation3 Multiple researchers have already demonstrated the ability to engineer T/L that possess similar structure, function, and cellular behavior as normal tissue,Citation4 but the in vivo integration of these engineered constructs with the surrounding normal tissues is a persistent challenge. In particular, the apposition of T/L against their bone insertion site fails to restore the complex anatomy found at the native bone-ligament or bone-tendon interface, commonly known as the enthesis. As a result, the repaired tissue possesses inferior mechanical and biochemical properties, increasing the risk of re-injury and continued disability. Therefore, restoration of the complex structure and function of the enthesis is the next step in bringing the promise of T/L tissue engineering to fruition. While several approaches have been explored, including the application of growth factors, scaffolds, bone cements, and inhibitors of matrix metalloproteinases,Citation5 recapitulation of the fibrocartilaginous layer interposed between bone and tendon in the native enthesis will require guided chondrogenesis of a cell source, whether exogenously-applied or endogenously-derived.
Given the central role of cells in enthesis repair, this review examines the in vivo evidence to support cellular therapy in bone-tendon interface regeneration. We first describe the complex anatomical and biomechanical characteristics of the bone-tendon interface, and then present the embryonic development and postnatal maturation of the enthesis, with special emphasis on the cellular origin of interfacial tissues and the biophysical cues that coordinate their differentiation. We next discuss the pathophysiology of bone-tendon injury and the subsequent intrinsic healing cascade, and how its insufficiency in restoring native structure and function has led to the development of current tissue engineering strategies, specifically cellular therapies. In closing, the regulatory and scientific hurdles impeding the adoption of cellular therapy in the clinical care of bone-tendon injuries are presented and potential solutions are offered.
Structure-Function Relationship of the Bone-Tendon Interface
While tendons and ligaments connect muscle-to-bone and bone-to-bone, respectively, they share a similar hierarchical structure of collagen fibers and resulting mechanical properties. Furthermore, the interface between T/L and bone are otherwise indistinguishable, although a large degree of variability exists depending on the location of the attachment site. Therefore, tendons and ligaments will be considered interchangeable for the purpose of this review. In general, T/L that attach to the bone diaphysis display fibers inserting directly into cortical bone or periosteum, and are known as fibrous entheses.Citation6 Conversely, T/L that insert into bone epiphyses, often within or around a synovial joint, possess a gradual transition from connective tissue to bone with two intervening layers of fibrocartilage.Citation7 Interfaces that possess this four-zone structure are known as fibrocartilaginous entheses.Citation6 As the vast majority of injuries of the bone-tendon interface involve fibrocartilaginous entheses, with surgical repair failing to restore this native structure, no further discussion on the anatomy and healing potential of fibrous entheses will be performed. For a detailed description on the latter type of bone-tendon insertion, please refer to references Citation7 and Citation8.
In the typical fibrocartilaginous enthesis, there are four discernible zones—dense connective tissue (T/L), uncalcified fibrocartilage (UF), calcified fibrocartilage (CF), and bone ().Citation6 The first zone (T/L) is similar to the midsubstance of these tissues in terms of architecture and extracellular matrix protein composition, with aligned collagen type I fibers and small amounts of the proteoglycans decorin and biglycan.Citation8,Citation9 The UF zone possesses columns of oval chondrocytes embedded in a matrix rich in collagen types II and III, with small amounts of collagen types I, IX, and X, and proteoglycans aggrecan and decorin.Citation7,Citation8 The CF zone consists predominantly of collagen type II, with significant amounts of collagen type X and aggrecan.Citation9 Collagen type X is produced by the hypertrophic chondrocytes found in this third zone (). Both fibrocartilaginous zones stain positive for glycosaminoglycans (). Lastly, the bony region displays the cellular and biochemical composition of typical bone, with a predominance of type I collagen and a high mineral content.Citation8
Figure 1. Histological features of the fibrocartilaginous bone-tendon interface stained with (A) Safranin-O, (B) H&E, and viewed with (C) polarized light microscopy. Note the four zones of the native enthesis. Magnification 20×. B, bone; CFC, calcified fibrocartilage; UFC, uncalcified fibrocartilage; T, tendon. Reproduced with permission from reference Citation122.
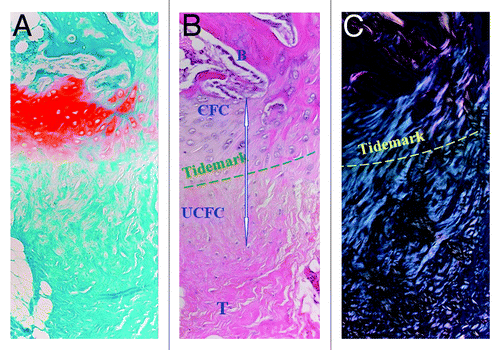
Much like the osteochondral interface of articular surfaces, a basophilic tidemark seen on histological sections separates the UF from CF and was thought to represent a mineralization front (). In support, Moffat et al.Citation10 detected mineral only within the CF and bone regions. Conversely, the application of Raman spectroscopy revealed a mineral-to-collagen ratio that increased linearly from tendon to bone, rather than abruptly, with a concomitant increase in crystalline order when moving from soft to hard tissue.Citation11 Therefore, it seems increasingly unlikely that four zones of distinct biomechanical, structural, and composition properties exist. Rather, there is a gradual transition from the compliant soft tissue (tensile modulus ~200 MPa) to rigid bone (~20 GPa). This serves to minimize stress concentrations and allow the efficient transmission of muscular forces to the skeleton.Citation8
In further support, Thomopoulos et al.Citation12 developed a two-dimensional finite element model of an insertion based upon collagen fiber orientation, as determined by polarized light microscopy. In comparing the stress concentrations in the idealized model to those of three comparison models, it was concluded that the microstructure of the insertion serves to (1) reduce stress concentrations and material mass, and (2) shield the insertion’s outward splay from higher stresses. In subsequent work, it was shown that an increasing mineral concentration coupled with decreasing collagen fiber orientation as one moves from the tendon toward bone, provides a functionally graded material composition that can explain the variation of stiffness over the length of the insertion.Citation13 Likewise, Moffat et al.Citation10 previously showed that the calcified fibrocartilage region possessed a higher Young’s modulus than the adjacent uncalcified fibrocartilage. Taken together, the transitional composition of the enthesis microstructure creates a gradient of increasing material stiffness, thereby allowing efficient joint mobilization while minimizing the risk of injury. Nevertheless, damage to the bone-tendon interface does occur, and the natural healing response fails to restore the complex anatomy of the native insertion. To recapitulate the embryonic events that give rise to the normal microstructure, thereby promoting bone-tendon regeneration, a comprehensive understanding of enthesis development is needed.
Enthesis Development
Embryonic origin of the bone-tendon interface
An understanding of both the cellular origin of interfacial tissues and the molecular mechanisms that mediate their development is in the nascent stage, due in large part to the only recent discovery of a selective marker of tendon and ligament cell-lineage. Scleraxis (Scx), a basic helix-loop-helix transcription factor, was first recognized at day 9.5 post coitum (p.c.) in the sclerotome of the somites and the mesenchymal cells in the body wall and limb buds of a mouse embryo.Citation14 Subsequent expression within mesenchymal precursors of the axial and appendicular skeleton in advance of chondrogenesis suggested a role in the developing skeleton. However, at later time-points in development, Scx expression is a highly specific marker for all the connective tissues that mediate attachment of muscle to bone.Citation15 Brent et al.Citation16 identified a Scx-positive region interposed between the sclerotome and myotome, two previously-formed somitic compartments that develop into the axial skeletal system and body wall muscles, respectively. Induction and differentiation of this interposing somitic compartment, the syndetome, is dependent on signals from the adjoining sclerotome and myotome, although the syndetomal precursors themselves are derived from the sclerotome.Citation16 Conversely, progenitor cells that ultimately constitute tendons of the limb develop independently of muscle, although subsequent tendon maturation requires reciprocal signaling between these two mesenchymal progenitors.Citation17
As tendon progenitors of both the axial and appendicular musculoskeletal system upregulate Scx early in development and maintain its expression following maturation into tenocytes, Scx is widely considered to be the most selective marker of tendon and ligament differentiation. However, despite the identification of Scx expression in cells comprising T/L, its complete functional role remains unknown. Scx heterodimerizes with bHLH protein E47 to selectively bind cis-acting elements that control collagen type I expression,Citation18 suggesting an important role in tenocyte-mediated fibrillogenesis.Citation19 However, Scx expression does not appear to be absolutely necessary for tendon formation.Citation20 Indeed, the molecular mechanisms underlying tenogenic and ligamentogenic differentiation are complex and multifactorial, surpassing the scope of this review. For a more detailed account of tendon development, the reader is referred to ref. Citation18. Nevertheless, the association of Scx expression with tendon development has proven important in elucidating the origin of interface tissues.
More specifically, Asou et al.Citation21 found that Scx expression was closely associated with, but distinct from, formation of the skeletal primordia, which expressed the known chondrogenic transcription factor Sox9. However, this study relied on whole mount in situ hybridization to determine the spatiotemporal pattern of mRNA transcript expression, thereby limiting the resolution to probe the heterotypic interactions of the primordial tendon-skeletal interface. Armed with a genetic mouse model capable of inducing a Sox9 expression reporter during development, Soeda et al.Citation22 demonstrated that at least some of the cells of tendons and ligaments originate from Sox9-expressing mesenchymal cells of the cartilage primordial, suggesting their contribution to the formation of tendon. Most recently, it was shown that a Scx+/Sox9+ progenitor pool contributes to the establishment of the interface between cartilage and T/L in the developing murine embryo.Citation23 Of particular interest, the gradual transition of Scx and Sox9 expression resembles the functional transition seen in mature entheses (). Namely, Scx-/Sox9+ mesenchymal cells were localized to the developing cartilaginous primordium, which subsequently develops into the bony skeleton by endochondral ossification. Scx+/Sox9- cells were found in the developing tendon midsubstance, while the closer the tendon was to the cartilaginous primordium, the greater the number of Scx+/Sox9+ progenitors. Furthermore, conditional inactivation of Sox9 expression in Scx+/Sox9+ progenitors results in defective formation of cartilage-T/L attachment sitesCitation23 and, more specifically, the loss of the bony eminence normally seen at insertion sites.Citation24 Therefore, the Scx+/Sox9+ progenitor pool is a multipotent mesenchymal population capable of differentiating into the tenocytes, ligamentocytes, and chondrocytes that ultimately establish the enthesis.
Figure 2. Embryonic development of the bone-tendon interface. (A) The degree of Scx and Sox9 expression determines cellular phenotype. Scx−/Sox9+ chondroprogenitors (CP) become chondrocytes of the skeletal anlagen while Scx+/Sox9− tenoprogenitors (TP) become tenocytes of the tendon midsubstance. Varying levels of Scx and Sox9 expression are seen in teno-/ligamento-/chondro-progenitors (TLCP), which give rise to cells of the bone-tendon interface. (B) Scx+/Sox9+ progenitors give rise to the primordial chondro-tendinous/ligamentous junction (CTJ/CLJ), which forms the osteo-tendinous/ligamentous junction (OTJ/OLJ) following birth. Reproduced with permission from reference Citation23.
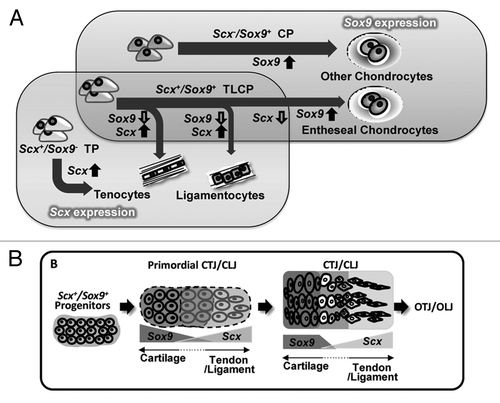
Signaling pathways active in enthesis formation
As the enthesis consists of multiple cellular phenotypes embedded in a complex extracellular matrix spanning a length less than 1 mm, the molecular mechanisms underlying its formation and maintenance are equally intricate. Much like the growth plate of bone,Citation25 the enthesis expresses Indian hedgehog (Ihh), parathyroid hormone-related peptide receptor (PTHrPR), collagen type II α1 (Col2α1), and collagen type X α1 (Col10α1).Citation9 In the growth plate, an elegant paracrine loop exists where Ihh secretion from hypertrophic chondrocytes upregulates PTHrP expression in a zone of proliferating chondrocytes, thereby inhibiting their hypertrophic differentiation and allowing continued longitudinal bone growth.Citation25 The presence of such a loop in the developing enthesis has not been established, but as discussed in Thomopoulos et al.,Citation8 there is growing evidence to support that a similar mechanism exists to guide bone ridge formation and subsequent mineralization in the fibrocartilaginous enthesis.Citation26 In addition, bone morphogenetic protein-4 (BMP-4), a member of the Transforming Growth Factor-β (TGF-β) superfamily, also plays a role in bone ridge patterning. Specifically, Scx promotes secretion of BMP-4 from nascent tenocytes, which in turn initiates formation of the bony ridge.Citation27 Inhibition of BMP-4 expression in Scx-positive cells leads to the failed formation of many bony ridges in the embryo, suggesting the pivotal role of this signaling pathway in early enthesis formation.
In addition to Ihh and its effects on PTHrP, TGF-β plays a central role in orchestrating tendon and cartilage differentiation at the developing interface. When examining the developing rat supraspinatus enthesis, Galatz et al.Citation28 could distinguish the rotator cuff as early as 13.5 d post coitum (d.p.c.). Although four insertion site zones (zone 1, tendon; zone 2, UF; zone 3, CF; zone 4, bone) were not distinct until 7 d after birth, TGF-β3 expression in zone 1 was discernible 13.5 d.p.c through 15.5 d.p.c, with TGF-β1 expression becoming upregulated at 15.5 d.p.c before diminishing after 18.5 d.p.c. In a related study, Lorda-Diez et al.Citation29 showed a differential effect of TGF-β signaling on chondrogenesis and fibrogenesis when comparing an in vitro to in vivo experimental model. Namely, TGF-β supplementation of a high density micromass culture suppressed chondrogenic markers Sox9 and Aggrecan, while upregulating fibrogenic markers Scx and Tenomodulin. Conversely, TGF-β upregulated Sox9 in vivo, thereby inducing ectopic chondrogenesis. The discrepancy between model systems may be explained by transcriptional repressors of TGF-β, including TGF-interacting factor Tgif1 and SKI-like oncogene SnoN. Regions of the developing digit expressing these transcription repressors went on to become soft connective tissues such as ligament, joint capsule, or tendon, while TGF-β regions devoid of SnoN and Tgif1 adopted a chondrogenic lineage. Furthermore, limb mesodermal cells cultured in a TGF-β-supplemented medium showed downregulation of Sox9 and Aggrecan expression when transfected with Tgif1.Citation29
Just as this orchestrated spatiotemporal expression pattern of signaling molecules results in regional differences in molecular markers that are indicative of differentiating cellular phenotypes, the expression of extracellular matrix proteins also varies by location. While the insertion site of the developing rat supraspinatus does not display four zones until 7 d following birth, the primordial tendon region stains positive for collagen type I beginning at 13.5 d.p.c.Citation28 Likewise collagen type II is expressed in the cartilaginous anlagen from 13.5 d.p.c. until postnatal day 21, when endochondral ossification replaces cartilage with bone. A fibrocartilaginous region interposed between bone and tendon is distinguishable as early as 7 d following birth, with collagen type II expression found in both zones 2 and 3. Collagen type X, indicative of chondrocyte hypertrophy, is first expressed in zone 3 at postnatal day 14, with continued expression into adulthood.Citation28 In the developing mouse patella tendon, collagen type I, the proteoglycan fibromodulin, and the glycoprotein tenomodulin (TNMD), were expressed evenly along the tendon at embryonic day 17.5 (E17.5), but TNMD expression was significantly reduced at the insertion site starting at postnatal day 7.Citation30 Conversely, glycoprotein tenascin C expression was found only at the insertion at E17.5, but increased expression in the midsubstance was noted after birth. Likewise, both biglycan and cartilage oligomeric protein were concentrated at the insertion site.Citation30
The role of mechanical loading on enthesis development
The mature enthesis, as with other musculoskeletal tissues, is responsive to mechanical stimulation.Citation7 However, the role of muscle loading in the formation of a functional bone-tendon interface has only recently been explored. As the fibrocartilaginous zones of the developing rat supraspinatus enthesis are not visible until postnatal day 7,Citation28 it was hypothesized that muscle loading following birth contributes to the formation of the graded transition seen in adult interfaces. Thomopoulos et al.Citation31 injected the left supraspinatus muscle of a rat with botulinum toxin A (BtxA) at birth, effectively inducing paralysis of the left shoulder. At day 14, evidence of transitional fibrocartilage was seen forming between the supraspinatus tendon and its humeral head insertion, but by day 21 the fibrocartilage had regressed. Instead, there was a layer of disorganized mesenchymal-like cells and hypertrophic chondrocytes. By day 56, the tendon-bone interface in the control shoulders consisted of fully developed transitional fibrocartilage organized in the classical four-zone structure. The shoulders paralyzed by BtxA injection, however, showed fibrochondrocytes embedded in a disorganized matrix lacking any indication of a zonal structure.Citation31
During normal enthesis development of the rat supraspinatus, a gradient in the mineral-to-bone ratio is detectable at the leading edge of the hard-soft tissue interface as early as postnatal day 7.Citation32 While the length of this mineralized region (~20 μm) remains constant through day 28,Citation32 the amount of mineralized bone increases over time due to endochondral ossification.Citation31 Mechanical loading is necessary for this continued maturation, as BtxA-induced paralysis results in significant reductions in bone volume, as compared with saline-injected controls.Citation31 Likewise, the BtxA-unloaded shoulders displayed reduced collagen fiber organization and crystallographic atomic order of the hydroxylapatite phase, a measure of crystallinity. These microstructural and compositional changes resulted in significantly reduced strength, modulus, and toughness in the paralyzed shoulders.Citation33 Taken together, these findings emphasize the importance of appropriate muscle loading in enthesis formation, and support the need to consider the mechanical microenvironment of the healing bone-tendon interface following injury.
Bone-Tendon and Bone-Ligament Injury and Natural Healing
Incidence of bone-tendon injury and the natural healing response
Injuries to the bone-tendon or bone-ligament interface are common and are traditionally classified as either acute ruptures or chronic degenerative changes.Citation6 However, such a strict dichotomy is unlikely to exist. While the microstructure of the degenerative enthesis (enthesopathy) has not been directly compared with that of the acutely ruptured interface, it is probable that degenerative changes precede acute tears, as is seen in the injuries of the tendon midsubstance. Kannus and JoszaCitation34 reported that nearly all (97%) of spontaneously ruptured tendons showed histopathological changes indicative of chronic tendon degeneration. Likewise, acutely ruptured tendons are significantly more degenerated than tendinopathic tendons.Citation35
Enthesopathies are defined as pathological changes in the enthesis and are frequently seen in athletic populations, in addition to those suffering from rheumatological conditions, such as rheumatoid arthritis, spondylarthropathy, calcium pyrophosphate skeletal hyperostosis (CPPD), and diffuse idiopathic skeletal hyperostosis (DISH).Citation7 Given such a broad array of etiologies, enthesopathies will not be covered in this review. Further description is provided in references Citation7–Citation8. In terms of acute damage to the bone-tendon interface, the two most common injuries involve tearing of the rotator cuff tendons of the shoulder and the cruciate ligaments of the knee. The rotator cuff is comprised of the interdigitating tendons of four muscles—subscapularis, supraspinatus, infraspinatus, and teres minor—that attach on the lateral aspect of the humeral head, serving important roles in both stabilizing and mobilizing the shoulder. Rotator cuff tears are a common cause of debilitating pain, reduced shoulder function, and weakness, affecting more than 40% of patients older than 60 y of age and resulting in 30 000 to 75 000 repairs performed annually in the United States.Citation36 Despite advances in surgical techniques and in the understanding of shoulder pathology, chronic tears fail to heal in 20–95% of cases.Citation37,Citation38 Likewise, damage to ligaments of the knee are very common, with an estimated incidence of 2/1000 people per year in the general population.Citation39 Ninety percent of these injuries involve the anterior cruciate ligament (ACL) and medial collateral ligament (MCL).Citation39 Depending on the site of injury of the MCL, conservative therapy can often be employed with satisfactory results. However, the ACL does not heal spontaneously and requires reconstructive surgery to restore knee stability.Citation40 Although the majority of ACL ruptures occur in the midsubstance, successful reconstruction is dependent upon initial fixation and subsequent osseointegration of a graft into a bone tunnel.Citation40 Traditionally, the bone-patella tendon-bone (BPTB) autograft has been the preferred autologous graft due to superior bone-to-bone healing, as opposed to autografts derived from soft tissues, most often hamstring tendons. The improved osseointegration of bone blocks in bone tunnels results in enhanced mechanical properties at early time points of healing.Citation41 However, BPTB autografts are associated with higher morbidity rates,Citation40 leading to increased use of tendon grafts. Furthermore, rotator cuff repair involves the apposition of the debrided tendon against the humeral head.Citation42 Therefore, complete restoration of the native bone-tendon interface could reduce the risk of re-rupture, while allowing earlier and more aggressive rehabilitation protocols.
While some studies have shown histological evidence of fibrocartilage formation following surgical repair of the bone-tendon interface,Citation43 the majority have failed to demonstrate restoration of the native enthesis structure. Using a flexor tendon autograft for ACL reconstruction in a dog model, Tomita et al.Citation44 found no fibrocartilage formation between the graft and the bone tunnel up to 12 wk, resulting in an ultimate strength that was 42% of the normal ACL. In a similar study, Newsham-West et al.Citation45 examined the long-term healing of the bone-tendon interface in a sheep model following surgical reattachment of the patellar tendon to the patella. At 2 y, macroscopic observation revealed a tendon that merged seamlessly with the bone tissue. However, microscopic evaluation showed residual hypercellularity, extracellular matrix disorganization, and no fibrocartilage layer. Therefore, it is generally recognized that current surgical approaches are unable to recapitulate the four zones of the native fibrocartilaginous enthesis, resulting in reduced mechanical properties and an increased risk of re-injury. Consequently, several researchers have explored the possible mechanisms to explain the failed regeneration.
Potential mechanisms to explain the failed healing response
The role of intrinsic T/L or bone cells in healing at the enthesis is currently not understood. As the fibrocartilaginous region is avascular,Citation6 endogenous progenitor cells that might reconstitute the enthesis must be derived from the surgically apposed tendon, as transdifferentiating tenocytes or tendon-derived stem cells, or from pericytes located in the granulation tissue or the subchondral bone. In seems unlikely that tendon-derived cells, found either in an autograft or the torn tendon, contribute significantly to bone-tendon healing, as shown in a recent study.Citation46 Conversely, bone marrow-derived cells may contribute to enthesis healing. In a mouse model that expresses green fluorescent protein (GFP) only in the bone marrow- and circulation-derived cells, Kida et al.Citation47 surgically repaired transected supraspinatus tendons. Drilling into the bone marrow was performed in the greater tuberosity of the right shoulder prior to repair, while the left shoulder was repaired without drilling. GFP-positive mesenchymal cells were present in the repaired rotator cuffs of both groups, with a higher concentration seen in the drilling group at 2, 4, and 8 wk. Furthermore, the ultimate load was significantly higher in the drilling group at both 4 and 8 wk.Citation47
While this study demonstrates the possibility of bone marrow-derived cells improving bone-tendon healing, the phenotype of these cells was not explored. It is unknown if these cells differentiated into resident fibrochondrocytes of the healing enthesis, or if they served as mediators of repair by paracrine factors and/or direct cell-cell contact. In vitro, Wang et al.Citation48 showed that osteoblast-fibroblast co-cultures slightly upregulated markers of chondrogenesis, including collagen type II and aggrecan. However, whether the osteoblasts or fibroblasts were principally responsible for this change in gene expression was not investigated. In a related study, a trilineage coculture system (osteoblasts–BMSC–fibroblasts) on a hybrid silk scaffold was created.Citation49 Both RT-PCR and immunohistochemistry demonstrated that the BMSCs differentiated into fibrochondrocytes, with a calcified region forming between the BMSCs and osteoblasts, but not the BMSCs and fibroblasts. Therefore, it appears that osteoblasts and fibroblasts are capable of supporting fibrocartilage differentiation of MSCs when interposed between the two. Why this does not consistently occur during the natural healing of the bone-tendon interface remains unknown.
One possible explanation is that there is an insufficient cell concentration to reconstitute the native fibrocartilage at the injury site. Given the avascular nature of the fibrocartilaginous region, this is an attractive hypothesis; it is has been the central basis for cellular therapies in bone-tendon healing, as discussed in detail below. A second, mutually compatible, explanation involves the biochemical and biomechanical microenvironment of the lesion. The synovial fluid of joints is known to inhibit tendon-to-bone healing,Citation50 ostensibly due to elevated pro-inflammatory cytokines and metalloproteases found in association with chronic tendon degeneration or acute tears. Additionally, synovial fluid contains the anti-adhesion protein lubricin, which is found on the end of ruptured intrasynovial tendons.Citation51 Regardless of the identity of the inhibitory factors, the healing of the bone-tendon interface is worse when exposed to an intra-articular environment.Citation52 Consequently, surgeons have developed repair strategies that minimize permeation of synovial fluid into the healing bone-tendon interface.Citation53 While these surgical techniques increase initial fixation strength, improve contact areas, and decrease gap formation at the healing enthesis, there is insufficient clinical evidence at this time to definitively confirm superior structural healing or functional outcomes in patients.Citation42
Mechanical loading of the post-operative bone-tendon interface is also known to affect healing. Using a rat model of a transected supraspinatus tendon, Thomopoulos et al.Citation54 examined the effect of post-operative activity levels on the biomechanical, structural, and compositional properties of the healing shoulder. Of the three activity levels—plaster-cast immobilization, normal cage activity, and daily exercise—shoulders that were immobilized possessed the best quasilinear viscoelastic properties. Likewise, the immobilized shoulders demonstrated significantly higher collagen orientation and expressed extracellular matrix genes in a pattern similar to uninjured insertions.Citation54 In contrast, complete removal of load by injecting BtxA into the supraspinatus was detrimental to rotator cuff healing, especially when combined with immobilization.Citation55 In a similar study examining the effect of early and delayed mechanical loading on tendon-to-bone healing after ACL reconstruction, it was found that delayed loading improved mechanical and biological parameters to a greater extent than immediate loading or prolonged post-operative immobilization. Therefore controlled mobilization of the healing bone-T/L interface is required to optimize the restoration of native anatomical structure and, in turn, the functional outcome.Citation56
Tissue Engineering Approaches to Bone-T/L Healing
Tissue engineering of the bone-T/L interface, like any organ system, combines cells, scaffolds, bioactive molecules, and biophysical stimulation, in an effort to restore structure and function. However, unlike certain elements of the musculoskeletal system such as the T/L midsubstance, biological interfaces present a more complex engineering challenge due the multiple cellular phenotypes and graded mechanical properties existing in highly organized, but spatially small (<1 mm), extracellular matrix. Recent efforts to fabricate graded scaffolds capable of supporting zone-appropriate cell types by incorporating biochemical componentsCitation57 or viral vectorsCitation58 have shown promise. Others have designed triphasic scaffolds, with controlled matrix topography and mechanical properties, thereby supporting cell phenotypes germane to the enthesis.Citation59,Citation60 Efforts to enhance enthesis healing by scaffolding have been extensive, with several FDA-approved products available for clinical use (see ref. Citation36 for a thorough review of scaffolds designed to improve bone-T/L healing).
Given the role of growth factorsCitation61 and metalloproteinasesCitation62 in enthesis development and healing, many investigators have used bioactive agents to augment surgical repair in animal models. BMP-2, a known regulator of osteogenesis and tenogenesis,Citation63 has been applied with variable success to multiple injury models, enhancing the mechanical propertiesCitation64 and osteoid formationCitation65 at the healing enthesis. Under a similar rationale, bone cement has also been applied to the surgically repaired interface. Gulotta et al.Citation66 augmented tibial tunnel fixation of a tendon autograft in ACL reconstruction by applying a magnesium-based bone cement. While there no was effect at 3 wk, the augmented group showed increased fibrocartilage and bone formation, as well as superior ultimate load, at 6 wk.Citation66 As a final example, several investigators have delivered TGF-β isoforms to the surgical site. TGF-β plays a significant role in interface development and healing, as discussed above. Unfortunately, exogenous application of this growth factor has shown little effect on bone-tendon healing.Citation5,Citation67 For a more comprehensive review of interface tissue engineering approaches using bioactive agents, see reference Citation5.
Cell therapy in bone-T/L interface regeneration—animal models
During surgical repair of the bone-tendon interface, the ruptured end of the tendon is debrided and the bony insertion site is decorticated, destroying any remaining fibrocartilage.Citation43 As a result, restoration on the native zonal enthesis structure requires transdifferentiation of osteoblasts or tenocytes into fibrochondrocytes, or the chondrogenesis of endogenous progenitor cells. As discussed above, it appears unlikely that the tendon contributes a substantive cell source to recapitulate the normal fibrocartilage zones. While bone marrow-derived progenitor cells can infiltrate into the healing interface,Citation47 this intrinsic response is insufficient to restore native anatomy. Instead, a fibrous enthesis often forms, with an immediate transition from soft connective tissue to stiff cortical bone. In an effort to promote fibrocartilage formation between the native bone and T/L, investigators have applied an interposing layer of cells that possess chondrogenic ability (). Four classes of cells/tissues have been explored to date—ACL-derived stem cells, chondrocytes, MSCs, and periosteum. A detailed description of the experimental design and results of these studies is provided in , and is summarized below.
Figure 3. Cell sources for augmenting bone-tendon healing. Animal studies exploring the use of MSCs to improve bone-tendon healing have derived these cells primarily from the bone marrow, although other tissues (e.g., adipose) also harbor MSCs (not shown). ACL-derived MSCs can be obtained from the ruptured ligament, while chondrocytes/cartilage plugs can be taken from non-weightbearing articular surfaces. Lastly, the periosteum is typically harvested from the anteromedial tibia, given the ease of access in the absence of overlying soft tissues.
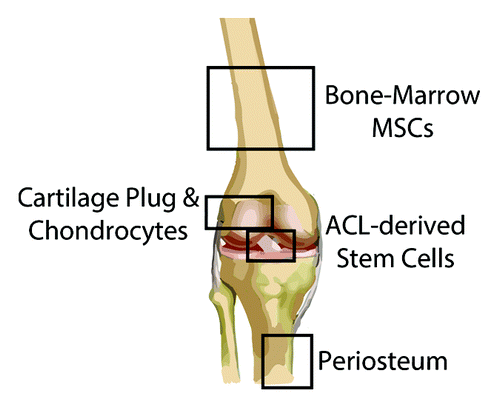
Table 1. In vivo studies of cellular therapy in bone-tendon interface healing
ACL-derived stem cells
Rupture of the ACL occurs most frequently in the ligament midsubstance and fails to spontaneously heal, necessitating ACL reconstruction. The failed healing response is traditionally attributed to the hypovascular nature of the ACL, but recent work suggests that the ACL possesses a source of multipotent stem cells that exist in the vasculature of the septum that divides the two ACL bundles.Citation68 From this vasculature, isolation of CD34- and CD146-postitive cells revealed the capacity for multipotent differentiation. In addition, these vasculature-associated stem cells were found in higher concentrations in ruptured ACLs than uninjured ligaments.Citation68 In a series of three studies, investigators hypothesized that these CD34+ stem cells could augment ACL reconstruction by improving integration of a tendon autograft into the bone tunnels.
ACL reconstructions in animal models were augmented with resected autologous ACL tissue,Citation69 CD34+ cells alone,Citation70 or monolayer sheets of CD34+ cells,Citation71 either injected intra-articularly or sutured to the bone-tunnel portion of the autograft. Across these studies, the cell-treated groups showed enhanced angiogenesis and osteogenesis in the bone tunnel, a smaller tibial tunnel cross-sectional area, and enhanced mechanical properties. Furthermore, these exogenous cells were capable of inducing angiogenic and osteogenic differentiation of host cells, as well as directly differentiating into bone and endothelial cells.Citation70,Citation71 Taken together, the above studies suggest that the ACL possesses a multipotent cell population that can improve the tendon graft integration following ACL reconstruction. However, the practicality of isolating these cells for exogenous application remains dubious, unless processing can occur intraoperatively or future technologies can augment the contribution of these cells to the intrinsic healing process.
Chondrocytes/cartilage tissue
Rather than applying a multipotent cell source capable of undergoing chondrogenesis, the interposition of mature chondrocytes between tendon and bone has shown promise as a means of restoring the transitional anatomical structure. However, the ability of differentiated chondrocytes to improve the mechanical properties of the healing interface has been limited so far. In two studies using a rabbitCitation72 and goatCitation73 model, Wong et al. resected the distal 1/3 of the patella and sutured the patella tendon to the remaining proximal 2/3 bone. Both a chondrocyte pelletCitation72 and an autologous cartilage plug derived from condylar hyaline cartilageCitation73 were placed between the tendon and bone, resulting in more fibrocartilage formation without enhancing the mechanical properties, as compared with controls.
In a rat model of Achilles insertion repair, Nourissat et al.Citation74 compared MSCs with chondrocytes in terms of their ability to restore enthesis structure and function. Both MSCs and chondrocytes produced fibrocartilage starting at day 15, as demonstrated by collagen type II expression and positive glycosaminoglycan staining. However, only the MSC-treated interfaces recapitulated the columnar structure by day 45. Additionally, both chondrocytes and MSCs restored the ultimate load to uninjured levels by day 45, with no difference between cell groups.Citation74 In a related study, cells isolated from the bone-tendon interface of the rat supraspinatus were concentrated in a gelfoam and inserted between the bone and tendon during suture repair.Citation75 The interface cell group, as compared with no cells and gelfoam alone, accelerated the healing process as demonstrated by increased cellularity, vascularity, and inflammation at week 3. By week 12, the cell-treated group showed superior collagen organization.Citation75
Mesenchymal stem cells (MSCs)
MSCs have emerged as the gold standard for cellular therapies in musculoskeletal diseases, given their ease of expansion and multipotency.Citation76 MSCs are capable of differentiating into tenocytes, chondrocytes, and osteocytes,Citation76-Citation78 thereby potentially aiding in restoration of the native structure of the healing tissues. Beyond differentiating into site-appropriate epithelial lineages, MSCs secrete bioactive molecules that provide a regenerative microenvironment for a variety of injured adult tissues.Citation79 For these reasons, several investigators have explored the effect of augmenting enthesis repair with MSCs.
Experiments investigating the utility of MSCs in augmenting bone-tendon healing were first performed in rabbit models.Citation80,Citation81 Ouyang et al.Citation81 fixed the hallicus longus tendon in a calcaneal bone tunnel with the interface augmented with bone marrow MSCs (BMSCs) in a fibrin gel, or fibrin gel alone. While the study did not include any mechanical testing, the BMSC group enhanced collagen type II staining, though only 50% of the graft-tunnel interface contained fibrocartilage. Lim et al.Citation80 and Soon et al.Citation82 reported similar findings, with the MSC group showing chondrocyte-like cells at the interface by 2 wk and a differentiated enthesis with four-zonal transition by 8 wk. Conversely, the fibrin only group contained fibrovascular scar between tendon graft and bone at every time point. The stark contrast in histological appearance between groups translated into to dramatic group differences in mechanical properties.Citation80,Citation82
These early studies provided strong evidence to support the augmentation of reconstructive surgeries involving tendon grafts fixed in bone tunnels, but the utility of cell therapy in healing the fibrocartilaginous enthesis following rotator cuff repair remained unexplored. Over the course of four studies, Gulotta and colleagues manipulated BMSCs by virus-mediated overexpression of bioactive agents that are known to affect tendon development and healing. In all cases, the rat supraspinatus tendon was transected and repaired with sutures. BMSCs aloneCitation83 or BMSCs overexpressing BMP-13,Citation84 a growth factor capable of inducing ectopic tendon formationCitation85 and improving in vivo tendon healing,Citation86 were localized to the wound site in a fibrin clot. At both 2 and 4 wk, there was no difference between groups in mechanical properties, new cartilage formation, or collagen fiber organization. In two related studies, BMSCs were infected with a viral vector designed to overexpress the tenogenic transcription factor ScleraxisCitation87 or membrane type 1 matrix metalloproteinase (MT1-MMP, also called MMP-14).Citation88 MT1-MMP is a membrane-bound matrix metalloproteinase that plays a role in embryonic development, being upregulated in areas of chondrocyte differentiation as well as in areas of tendon, perichondral bone, ligament, and joint capsule development.Citation88,Citation89 In comparison to suture repairs augmented with uninfected BMSCs both Ad-Scx MSCs (Adenoviral-transfected MSC with constitutively active Scx) and Ad-MT1-MMP MSCs increased fibrocartilage formation, and increased the mechanical properties (ultimate load, ultimate stress, stiffness) of the healing bone-tendon interface, as compared with controls.
In all the above studies, BMSCs were delivered to the wound site in a fibrin gel carrier. While fibrin clots offer sufficient form and durability to localize stem cells to wound site, they offer little mechanical support to the healing tissue.Citation90 Recent work by Butler et al.Citation91 suggests that matching the mechanical properties of a cell-seeded scaffold to that of the native tissue improves the in vivo function of the regenerating tissue. Therefore, combining BMSCs with biomimetic interfacial scaffold may work synergistically to enhance enthesis regeneration. While multiphasic scaffolds have been fabricated,Citation59,Citation92 their utility in augmenting enthesis repair has not been explored in vivo. However, Yokoya et al.Citation93 did seed BMSCs on a polyglycolic (PGA) sheet before implanting the cell-seeded scaffold into a full thickness infraspinatus defect that included the insertion site. By week 8, only the BMSC+PGA group showed fibrocartilage formation, while both the BMSC+PGA and PGA sheet alone produced positive glycosaminoglycan staining by week 16. At this later time point, the shoulders augmented with the cell-seeded scaffold possessed superior mechanical properties to those which received the PGA sheet alone, and both experimental groups drastically enhanced healing when compared with untreated defects.Citation93 Unfortunately the delivery of BMSCs alone was not explored as an additional control group.
Periosteal progenitor cells/periosteal tissue
The periosteum is a thin layer of connective tissue that lines the outer surface of bone. It is a bilayered membrane with an outer layer of aligned collagen, elastin, and fibroblasts, and an inner (cambrium) layer that consists mostly of progenitor cells that contribute to bone repair.Citation94 The periosteum has received growing interest as an autologous biomaterial for musculoskeletal tissue regeneration as it contains progenitor cells and noncollagenous proteins (including growth factors) embedded in a native extracellular matrix.Citation95 The periosteum may have particular relevance to augmentation of bone-tendon healing, as the outer, fibrous layer resembles the soft connective tissue of T/L, while the cambrium layer may provide progenitor cells for chondrogenesis and osteogenesis,Citation95 thereby restoring the four zones of native entheses.
Karaoglu et al.Citation96 compared the effects of periosteum tissue (P) against bone marrow aspirate (BMA). Relative to untreated controls, both P- and BMA-treated groups showed increased interface organization by week 6. Chondrocyte-like cells were present at 6 wk only in the BMA group, but both experimental conditions produced fibrocartilage islands by week 12. At week 6, both load-to-failure and stiffness were significantly increased in P, as compared with BMA, which was also superior to controls.Citation96 Similarly, Chen and colleagues demonstrated that periosteumCitation97,Citation98 or hydrogels containing periosteal progenitor cells with supplemented BMP-299 could drastically improve both the histological and biomechanical properties of the healing bone-tendon interface. When examining periosteum-augmented repairs at 12 wk, the interface anatomy resembled that of the native enthesis, with a clearly visible tidemark.Citation98 Since the effects of periosteum and periosteum progenitor cells with BMP-2 supplementation were investigating in independent studies, it is not possible to compare their relative efficacy.
The same can be said when comparing cell sources (e.g., MSCs vs. periosteum). As highlighted above, multiple cell sources can be used to improve fibrocartilage formation and mechanical properties of the healing bone-tendon interface. But, with the great diversity of animal models used and dependent variables measured, it is impossible to identify an ideal cell source at this time. Future work should directly compare multiple cell types within the same injury model, similar to that performed by Gulotta and colleagues.Citation83,Citation84,Citation87,Citation88 Furthermore, combining cells with growth factorsCitation99 and scaffoldsCitation93 may offer synergistic effects and should be further explored.
Cell therapy in bone-t/l interface regeneration—clinical studies
Despite the growing in vivo evidence demonstrating the benefit of cellular therapies in bone-tendon healing, the translation of these results into clinical care has been slow. To date, there have been no randomized, prospective trials investigating cell-augmented enthesis repair. However, three cases series have been performed, the results of which encourage future research.
Chen et al.Citation100 performed 372 single-bundle ACL reconstructions, all of which were augmented with periosteum harvested from the anterior tibial cortex. The median Lysholm knee scores increased from 56 points preoperatively to 95 point postoperatively. Following reconstruction, 85% of patients were able to return to moderate to strenuous activity, and 93% of patients had normal or near-normal ratings according to the International Knee Documentation Committee assessment.Citation100 In a similar study of 20 patients, a periosteal flap lateral to the rotator cuff footprint was created and subsequently interposed between the tendon and humeral head before securing with suture anchors.Citation101 At a mean follow-up of 14.4 mo, the average Constant score had increased from 51.7 points to 80.0 points. But, four patients (20%) displayed a tendon re-tear on postoperative MRI. Conversely, all patients (14/14) demonstrated tendon integrity at 12-mo follow-up in a different study in which a rotator cuff repair was augmented with autologous bone marrow mononuclear cells.Citation102 In this clinical study, the UCLA score increased from 12 ± 3.0 before surgery to 31 ± 3.2 after surgery.
Although these three clinical studies suggest the safety and feasibility of augmenting bone-tendon healing with autologous cell sources, no further conclusions can be drawn in the absence of control groups and larger patient enrollment. Moving forward, prospective, randomized trials will be required in order to establish the superiority of cell-augmented surgical repairs over the current standard of care. Furthermore, patient populations with a comparable severity of injury should be compared, as this parameter can greatly affect functional outcomes.Citation103 Standardized functional scores should also be included to enable valid comparisons between studies. To the same end, the surgical technique can also affect functional and radiological findings,Citation42 and so should be controlled as much as possible without jeopardizing patient care.
Translating Cell Therapy into Clinical Care
Regulatory, economic, and practical hurdles
As highlighted above, there is compelling evidence to support the application of cell therapies in augmenting current surgical techniques to repair bone-T/L injuries. However, only three case series involving autologous periosteum or mononuclear stromal cells have been performed in a patient population. In broader terms, less than 25% of promising biomedical discoveries yield a published randomized clinical trial, and less than 10% are established in clinical practice within 20 y.Citation104 The reasons for the stagnation of medical technologies in the pre-clinical phase are numerous, yet basic scientists and clinicians alike undoubtedly wish to see promising therapeutics make it into clinical trials. Moran et al.Citation105 have proposed several recommendations to improve bench-to-bedside translation, including changes at the level of the trainee up through governmental funding (e.g., NIH) and regulatory (i.e., FDA) institutions. Others have taken a more humble, but still ambitious approach to accelerate the implementation of regenerative medicine strategies into clinical care. “Facilitated endogenous repair,”Citation106 also referred to as “in situ guided tissue regeneration,”Citation107 is an approach to tissue engineering that avoids ex vivo culture of autologous cells and the use of manufactured scaffolds, thereby minimizing costs and the number of invasive clinical procedures. Part of this approach would involve the use of autologous cells and extracellular matrices procured, processed, and re-implanted intraoperatively. Two of the three clinical studies highlighted above could be classified under this concept.Citation100,Citation101 In addition to obviating the costs associated with establishing good manufacturing practices (GMP)-certified labs for ex vivo cell expansion and/or manipulation, many of these point-of-care approaches bypass the arduous process of receiving FDA approval. On the other hand, facilitated endogenous repair also includes the delivery of viral vectors, which would rightfully be under close regulatory scrutiny. Regardless, the philosophy of this regenerative medicine approach stands in contrast to a tissue engineering strategy involving ex vivo whole organ/tissue fabrication with subsequent in vivo implantation. By allowing the body to serve as the bioreactor, cellular therapies for musculoskeletal disease may be implemented earlier and in a larger patient population.
Limitations of current animal models
Limitations of applying discoveries in animal models to the treatment of human disease are inherent in every field of biomedical research, including orthopedic sports medicine. Even within a specific pathological condition, different animal models may be most appropriate depending upon which disease parameter is being studied. In the case of rotator cuff repair, the rat is ideal for exploring biological approaches to bone-tendon healing, given the many similarities in shoulder anatomy shared with humans, as well as its relatively inexpensive cost.Citation108 By comparison, the canine shoulder allows investigators to study clinically relevant rehabilitation modalities, including slinging, hobbling, casting, swimming, jumping, and treadmill running.Citation108 Furthermore, each animal model possesses differing intrinsic healing capabilities, limiting the extension of experimental results to particular injuries that do not heal spontaneously in humans.Citation109
Another seldom acknowledged limitation of animal models of acute T/L ruptures is that spontaneous tears in humans are almost always preceded by chronic degenerative changes.Citation34 Conversely, acute rupture and repair models involve transection of a previously healthy tendon, often in young adult animals. Degenerative changes, even with the tendon intact, are associated with upregulation of pro-inflammatory and catabolic mediators in the synovial fluid.Citation110 Therefore, it may be argued that the intra-articular milieu of spontaneously ruptured T/L in humans differs greatly from that created in animal models. Fortunately, validated animal models of tendinopathy do exist.Citation111 But to our knowledge, no one has yet studied the healing of an acute T/L rupture following a prodromal phase of degenerative changes.
Combining regenerative medicine with biophysical modalities
All musculoskeletal tissues are responsive to mechanical loading. In fact, the health of the tissue depends upon the homeostasis of anabolic and catabolic mediators, the balance of which is controlled by the stresses exerted on the system.Citation112 Furthermore, the timing and magnitude of mechanical loads placed on surgically repaired structures is carefully monitored by surgeons, while extensive research has been conducted in an effort to optimize rehabilitation protocols aimed at restoring function quickly and completely. In spite of this standard of care, mechanical loading of surgically repaired tissues in animal models is almost never controlled, except when considered as a dependent variable. Of the primary research articles reviewed above, all allowed resumption of normal cage activity immediately following surgery. While most (especially small) animal models tolerate early loading exceedingly well with low rates of re-tear, such a practice is greatly discordant with the current standard of care in almost all orthopedic surgical procedures.
Given the importance of mechanical loading in both T/L interface development and healing, future research examining the relationship between regenerative medicine approaches and physical therapeutics is greatly needed.Citation113 More specifically, there is reason to believe that providing the appropriate mechanotransducive signals to the exogenously delivered cells is needed to optimize the regenerative effect. For example, improved mechanical properties were found in a healing tendon following an injection of platelet-rich plasma (PRP), an autologous source of growth factors of known importance in wound healing.Citation114 However, the beneficial effect of PRP augmentation was only noted when mechanical loading was introduced following the acute inflammatory phase of the healing cascade (3 d). On the other hand, long-duration immobilization negated any effect of PRP.
Lastly, cellular therapies may be synergistically combined with non-invasive biophysical modalities with known benefit in promoting bone-tendon healing. Low-intensity pulsed ultrasound,Citation115 pulsed electromagnetic fields,Citation116 and extracorporeal shockwave therapy,Citation117 have been shown to independently improve histological and mechanical properties of the surgically repaired bone-tendon interface. While the mechanisms by which such novel therapies exert these effects remain largely unknown, electrical cues are known to regulate cellular orientation, division, and differentiation, as well as the rate of in vivo wound healing.Citation118,Citation119 Thus electrical stimulation may serve as a novel tool for directing the regeneration of the bone-tendon interface, although the utility of this modality is unexplored. In the meantime, there is sufficient pre-clinical data to support the implementation of autologous MSC or chondrogenic cell sources as a means of enhancing enthesis healing, even if the optimal combination of tissue engineering elements is yet to be determined.
Abbreviations: | ||
ACL | = | anterior cruciate ligament |
BMP | = | bone morphogenetic protein |
BMSC | = | bone marrow-derived mesenchymal stem cell |
BPTB | = | bone-patella tendon-bone |
BtxA | = | botulinum toxin A |
CF | = | calcified fibrocartilage |
d.p.c. | = | days post coitum |
GFP | = | green fluorescent protein |
Ihh | = | Indian hedgehog |
MCL | = | medial collateral ligament |
MSC | = | mesenchymal stem cell |
MT1-MMP | = | membrane type 1 matrix metalloproteinase |
p.c. | = | post coitum |
PTHrPR | = | Parathyroid hormone-related peptide receptor |
RT-PCR | = | real-time polymerase chain reaction |
Scx | = | Scleraxis |
SnoN | = | SKI-like oncogene |
T/L | = | Tendons and Ligaments |
TGF-β | = | transforming growth factor beta |
Tgif | = | TGF-interacting factor |
TNMD | = | Tenomodulin |
UF | = | uncalcified fibrocartilage |
Disclosure of Potential Conflicts of Interest
No potential conflicts of interest were disclosed.
Acknowledgments
This work was supported in part by Commonwealth of Pennsylvania Department of Health (SAP4100050913), NIH (T32-EB001026 and 1R01 AR062947), and the U.S. Department of Defense (W81XWH-11-2-0143).
References
- Langer R, Vacanti JP. Tissue engineering. Science 1993; 260:920 - 6; http://dx.doi.org/10.1126/science.8493529; PMID: 8493529
- Tuan RS. Regenerative medicine in 2012: the coming of age of musculoskeletal tissue engineering. Nat Rev Rheumatol 2013; 9:74 - 6; http://dx.doi.org/10.1038/nrrheum.2012.235; PMID: 23321611
- Butler DL, Juncosa N, Dressler MR. Functional efficacy of tendon repair processes. Annu Rev Biomed Eng 2004; 6:303 - 29; http://dx.doi.org/10.1146/annurev.bioeng.6.040803.140240; PMID: 15255772
- Moffat KL, Kwei ASP, Spalazzi JP, Doty SB, Levine WN, Lu HH. Novel nanofiber-based scaffold for rotator cuff repair and augmentation. Tissue Eng Part A 2009; 15:115 - 26; http://dx.doi.org/10.1089/ten.tea.2008.0014; PMID: 18788982
- Paxton JZ, Baar K, Grover LM. Current progress in enthesis repair: Strategies for interfacial tissue engineering. Orthopedic Muscul Sys, 2012.
- Benjamin M, Kumai T, Milz S, Boszczyk BM, Boszczyk AA, Ralphs JR. The skeletal attachment of tendons--tendon “entheses”. Comp Biochem Physiol A Mol Integr Physiol 2002; 133:931 - 45; http://dx.doi.org/10.1016/S1095-6433(02)00138-1; PMID: 12485684
- Benjamin M, Toumi H, Ralphs JR, Bydder G, Best TM, Milz S. Where tendons and ligaments meet bone: attachment sites (‘entheses’) in relation to exercise and/or mechanical load. J Anat 2006; 208:471 - 90; http://dx.doi.org/10.1111/j.1469-7580.2006.00540.x; PMID: 16637873
- Thomopoulos S, Genin GM, Galatz LM. The development and morphogenesis of the tendon-to-bone insertion - what development can teach us about healing -. J Musculoskelet Neuronal Interact 2010; 10:35 - 45; PMID: 20190378
- Thomopoulos S, Williams GR, Gimbel JA, Favata M, Soslowsky LJ. Variation of biomechanical, structural, and compositional properties along the tendon to bone insertion site. J Orthop Res 2003; 21:413 - 9; http://dx.doi.org/10.1016/S0736-0266(03)0057-3; PMID: 12706013
- Moffat KL, Sun WHS, Pena PE, Chahine NO, Doty SB, Ateshian GA, Hung CT, Lu HH. Characterization of the structure-function relationship at the ligament-to-bone interface. Proc Natl Acad Sci U S A 2008; 105:7947 - 52; http://dx.doi.org/10.1073/pnas.0712150105; PMID: 18541916
- Wopenka B, Kent A, Pasteris JD, Yoon Y, Thomopoulos S. The tendon-to-bone transition of the rotator cuff: a preliminary Raman spectroscopic study documenting the gradual mineralization across the insertion in rat tissue samples. Appl Spectrosc 2008; 62:1285 - 94; http://dx.doi.org/10.1366/000370208786822179; PMID: 19094386
- Thomopoulos S, Marquez JP, Weinberger B, Birman V, Genin GM. Collagen fiber orientation at the tendon to bone insertion and its influence on stress concentrations. J Biomech 2006; 39:1842 - 51; http://dx.doi.org/10.1016/j.jbiomech.2005.05.021; PMID: 16024026
- Genin GM, Kent A, Birman V, Wopenka B, Pasteris JD, Marquez PJ, Thomopoulos S. Functional grading of mineral and collagen in the attachment of tendon to bone. Biophys J 2009; 97:976 - 85; http://dx.doi.org/10.1016/j.bpj.2009.05.043; PMID: 19686644
- Cserjesi P, Brown D, Ligon KL, Lyons GE, Copeland NG, Gilbert DJ, Jenkins NA, Olson EN. Scleraxis: a basic helix-loop-helix protein that prefigures skeletal formation during mouse embryogenesis. Development 1995; 121:1099 - 110; PMID: 7743923
- Schweitzer R, Chyung JH, Murtaugh LC, Brent AE, Rosen V, Olson EN, Lassar A, Tabin CJ. Analysis of the tendon cell fate using Scleraxis, a specific marker for tendons and ligaments. Development 2001; 128:3855 - 66; PMID: 11585810
- Brent AE, Schweitzer R, Tabin CJ. A somitic compartment of tendon progenitors. Cell 2003; 113:235 - 48; http://dx.doi.org/10.1016/S0092-8674(03)00268-X; PMID: 12705871
- Liu CF, Aschbacher-Smith L, Barthelery NJ, Dyment N, Butler D, Wylie C. What we should know before using tissue engineering techniques to repair injured tendons: a developmental biology perspective. Tissue Eng Part B Rev 2011; 17:165 - 76; http://dx.doi.org/10.1089/ten.teb.2010.0662; PMID: 21314435
- Carlberg AL, Tuan RS, Hall DJ. Regulation of scleraxis function by interaction with the bHLH protein E47. Mol Cell Biol Res Commun 2000; 3:82 - 6; http://dx.doi.org/10.1006/mcbr.2000.0195; PMID: 10775504
- Léjard V, Brideau G, Blais F, Salingcarnboriboon R, Wagner G, Roehrl MHA, Noda M, Duprez D, Houillier P, Rossert J. Scleraxis and NFATc regulate the expression of the pro-alpha1(I) collagen gene in tendon fibroblasts. J Biol Chem 2007; 282:17665 - 75; http://dx.doi.org/10.1074/jbc.M610113200; PMID: 17430895
- Murchison ND, Price BA, Conner DA, Keene DR, Olson EN, Tabin CJ, Schweitzer R. Regulation of tendon differentiation by scleraxis distinguishes force-transmitting tendons from muscle-anchoring tendons. Development 2007; 134:2697 - 708; http://dx.doi.org/10.1242/dev.001933; PMID: 17567668
- Asou Y, Nifuji A, Tsuji K, Shinomiya K, Olson EN, Koopman P, Noda M. Coordinated expression of scleraxis and Sox9 genes during embryonic development of tendons and cartilage. J Orthop Res 2002; 20:827 - 33; http://dx.doi.org/10.1016/S0736-0266(01)00169-3; PMID: 12168674
- Soeda T, Deng JM, de Crombrugghe B, Behringer RR, Nakamura T, Akiyama H. Sox9-expressing precursors are the cellular origin of the cruciate ligament of the knee joint and the limb tendons. Genesis 2010; 48:635 - 44; http://dx.doi.org/10.1002/dvg.20667; PMID: 20806356
- Sugimoto Y, Takimoto A, Akiyama H, Kist R, Scherer G, Nakamura T, Hiraki Y, Shukunami C. Scx+/Sox9+ progenitors contribute to the establishment of the junction between cartilage and tendon/ligament. Development 2013; 140:2280 - 8; http://dx.doi.org/10.1242/dev.096354; PMID: 23615282
- Blitz E, Sharir A, Akiyama H, Zelzer E. Tendon-bone attachment unit is formed modularly by a distinct pool of Scx- and Sox9-positive progenitors. Development 2013; 140:2680 - 90; http://dx.doi.org/10.1242/dev.093906; PMID: 23720048
- Kronenberg HM. Developmental regulation of the growth plate. Nature 2003; 423:332 - 6; http://dx.doi.org/10.1038/nature01657; PMID: 12748651
- Schweitzer R, Zelzer E, Volk T. Connecting muscles to tendons: tendons and musculoskeletal development in flies and vertebrates. Development 2010; 137:2807 - 17; http://dx.doi.org/10.1242/dev.047498; PMID: 20699295
- Blitz E, Viukov S, Sharir A, Shwartz Y, Galloway JL, Pryce BA, Johnson RL, Tabin CJ, Schweitzer R, Zelzer E. Bone ridge patterning during musculoskeletal assembly is mediated through SCX regulation of Bmp4 at the tendon-skeleton junction. Dev Cell 2009; 17:861 - 73; http://dx.doi.org/10.1016/j.devcel.2009.10.010; PMID: 20059955
- Galatz L, Rothermich S, VanderPloeg K, Petersen B, Sandell L, Thomopoulos S. Development of the supraspinatus tendon-to-bone insertion: localized expression of extracellular matrix and growth factor genes. J Orthop Res 2007; 25:1621 - 8; http://dx.doi.org/10.1002/jor.20441; PMID: 17600822
- Lorda-Diez CI, Montero JA, Martinez-Cue C, Garcia-Porrero JA, Hurle JM. Transforming growth factors beta coordinate cartilage and tendon differentiation in the developing limb mesenchyme. J Biol Chem 2009; 284:29988 - 96; http://dx.doi.org/10.1074/jbc.M109.014811; PMID: 19717568
- Liu CF, Aschbacher-Smith L, Barthelery NJ, Dyment N, Butler D, Wylie C. Spatial and temporal expression of molecular markers and cell signals during normal development of the mouse patellar tendon. Tissue Eng Part A 2012; 18:598 - 608; http://dx.doi.org/10.1089/ten.tea.2011.0338; PMID: 21939397
- Thomopoulos S, Kim HM, Rothermich SY, Biederstadt C, Das R, Galatz LM. Decreased muscle loading delays maturation of the tendon enthesis during postnatal development. J Orthop Res 2007; 25:1154 - 63; http://dx.doi.org/10.1002/jor.20418; PMID: 17506506
- Schwartz AG, Pasteris JD, Genin GM, Daulton TL, Thomopoulos S. Mineral distributions at the developing tendon enthesis. PLoS One 2012; 7:e48630; http://dx.doi.org/10.1371/journal.pone.0048630; PMID: 23152788
- Schwartz AG, Lipner JH, Pasteris JD, Genin GM, Thomopoulos S. Muscle loading is necessary for the formation of a functional tendon enthesis. Bone 2013; 55:44 - 51; http://dx.doi.org/10.1016/j.bone.2013.03.010; PMID: 23542869
- Kannus P, Józsa L. Histopathological changes preceding spontaneous rupture of a tendon. A controlled study of 891 patients. J Bone Joint Surg Am 1991; 73:1507 - 25; PMID: 1748700
- Tallon C, Maffulli N, Ewen SWB. Ruptured Achilles tendons are significantly more degenerated than tendinopathic tendons. Med Sci Sports Exerc 2001; 33:1983 - 90; http://dx.doi.org/10.1097/00005768-200112000-00002; PMID: 11740288
- Ricchetti ET, Aurora A, Iannotti JP, Derwin KA. Scaffold devices for rotator cuff repair. J Shoulder Elbow Surg 2012; 21:251 - 65; http://dx.doi.org/10.1016/j.jse.2011.10.003; PMID: 22244069
- Derwin KA, Badylak SF, Steinmann SP, Iannotti JP. Extracellular matrix scaffold devices for rotator cuff repair. J Shoulder Elbow Surg 2010; 19:467 - 76; http://dx.doi.org/10.1016/j.jse.2009.10.020; PMID: 20189415
- Galatz LM, Ball CM, Teefey SA, Middleton WD, Yamaguchi K. The outcome and repair integrity of completely arthroscopically repaired large and massive rotator cuff tears. J Bone Joint Surg Am 2004; 86-A:219 - 24; PMID: 14960664
- Miyasaka KC, Daniel DM, Stone ML, Hirschman P. The incidence of knee ligament injuries in the general population. Am J Knee Surg 1991; 4:3 - 8
- Woo SLY, Abramowitch SD, Kilger R, Liang R. Biomechanics of knee ligaments: injury, healing, and repair. J Biomech 2006; 39:1 - 20; http://dx.doi.org/10.1016/j.jbiomech.2004.10.025; PMID: 16271583
- Park MJ, Lee MC, Seong SC. A comparative study of the healing of tendon autograft and tendon-bone autograft using patellar tendon in rabbits. Int Orthop 2001; 25:35 - 9; http://dx.doi.org/10.1007/s002640000199; PMID: 11374265
- Dines JS, Bedi A, ElAttrache NS, Dines DM. Single-row versus double-row rotator cuff repair: techniques and outcomes. J Am Acad Orthop Surg 2010; 18:83 - 93; PMID: 20118325
- Uhthoff HK, Seki M, Backman DS, Trudel G, Himori K, Sano H. Tensile strength of the supraspinatus after reimplantation into a bony trough: an experimental study in rabbits. J Shoulder Elbow Surg 2002; 11:504 - 9; http://dx.doi.org/10.1067/mse.2002.126760; PMID: 12378172
- Tomita F, Yasuda K, Mikami S, Sakai T, Yamazaki S, Tohyama H. Comparisons of intraosseous graft healing between the doubled flexor tendon graft and the bone-patellar tendon-bone graft in anterior cruciate ligament reconstruction. Arthroscopy 2001; 17:461 - 76; http://dx.doi.org/10.1053/jars.2001.24059; PMID: 11337712
- Newsham-West R, Nicholson H, Walton M, Milburn P. Long-term morphology of a healing bone-tendon interface: a histological observation in the sheep model. J Anat 2007; 210:318 - 27; http://dx.doi.org/10.1111/j.1469-7580.2007.00699.x; PMID: 17331180
- Kinneberg KRC, Galloway MT, Butler DL, Shearn JT. The native cell population does not contribute to central-third graft healing at 6, 12, or 26 weeks in the rabbit patellar tendon. J Orthop Res 2013; 31:638 - 44; http://dx.doi.org/10.1002/jor.22261; PMID: 23138453
- Kida Y, Morihara T, Matsuda K, Kajikawa Y, Tachiiri H, Iwata Y, Sawamura K, Yoshida A, Oshima Y, Ikeda T, et al. Bone marrow-derived cells from the footprint infiltrate into the repaired rotator cuff. J Shoulder Elbow Surg 2013; 22:197 - 205; http://dx.doi.org/10.1016/j.jse.2012.02.007; PMID: 22543003
- Wang IE, Shan J, Choi R, Oh S, Kepler CK, Chen FH, Lu HH. Role of osteoblast-fibroblast interactions in the formation of the ligament-to-bone interface. J Orthop Res 2007; 25:1609 - 20; http://dx.doi.org/10.1002/jor.20475; PMID: 17676622
- He P, Ng KS, Toh SL, Goh JCH. In vitro ligament-bone interface regeneration using a trilineage coculture system on a hybrid silk scaffold. Biomacromolecules 2012; 13:2692 - 703; http://dx.doi.org/10.1021/bm300651q; PMID: 22880933
- Sun L, Zhou X, Wu B, Tian M. Inhibitory effect of synovial fluid on tendon-to-bone healing: an experimental study in rabbits. Arthroscopy 2012; 28:1297 - 305; http://dx.doi.org/10.1016/j.arthro.2012.02.017; PMID: 22607829
- Funakoshi T, Martin SD, Schmid TM, Spector M. Distribution of lubricin in the ruptured human rotator cuff and biceps tendon: a pilot study. Clin Orthop Relat Res 2010; 468:1588 - 99; http://dx.doi.org/10.1007/s11999-009-1108-z; PMID: 19798542
- Bedi A, Kawamura S, Ying L, Rodeo SA. Differences in tendon graft healing between the intra-articular and extra-articular ends of a bone tunnel. HSS J 2009; 5:51 - 7; http://dx.doi.org/10.1007/s11420-008-9096-1; PMID: 19052716
- Nassos JT, ElAttrache NS, Angel MJ, Tibone JE, Limpisvasti O, Lee TQ. A watertight construct in arthroscopic rotator cuff repair. J Shoulder Elbow Surg 2012; 21:589 - 96; http://dx.doi.org/10.1016/j.jse.2011.04.008; PMID: 21782471
- Thomopoulos S, Williams GR, Soslowsky LJ. Tendon to bone healing: differences in biomechanical, structural, and compositional properties due to a range of activity levels. J Biomech Eng 2003; 125:106 - 13; http://dx.doi.org/10.1115/1.1536660; PMID: 12661203
- Galatz LM, Charlton N, Das R, Kim HM, Havlioglu N, Thomopoulos S. Complete removal of load is detrimental to rotator cuff healing. J Shoulder Elbow Surg 2009; 18:669 - 75; http://dx.doi.org/10.1016/j.jse.2009.02.016; PMID: 19427237
- Killian ML, Cavinatto L, Galatz LM, Thomopoulos S. The role of mechanobiology in tendon healing. J Shoulder Elbow Surg 2012; 21:228 - 37; http://dx.doi.org/10.1016/j.jse.2011.11.002; PMID: 22244066
- Ramalingam M, Young MF, Thomas V, Sun L, Chow LC, Tison CK, Chatterjee K, Miles WC, Simon CG Jr.. Nanofiber scaffold gradients for interfacial tissue engineering. J Biomater Appl 2013; 27:695 - 705; http://dx.doi.org/10.1177/0885328211423783; PMID: 22286209
- Phillips JE, Burns KL, Le Doux JM, Guldberg RE, García AJ. Engineering graded tissue interfaces. Proc Natl Acad Sci U S A 2008; 105:12170 - 5; http://dx.doi.org/10.1073/pnas.0801988105; PMID: 18719120
- Spalazzi JP, Doty SB, Moffat KL, Levine WN, Lu HH. Development of controlled matrix heterogeneity on a triphasic scaffold for orthopedic interface tissue engineering. Tissue Eng 2006; 12:3497 - 508; http://dx.doi.org/10.1089/ten.2006.12.3497; PMID: 17518686
- Zhang X, Bogdanowicz D, Erisken C, Lee NM, Lu HH. Biomimetic scaffold design for functional and integrative tendon repair. J Shoulder Elbow Surg 2012; 21:266 - 77; http://dx.doi.org/10.1016/j.jse.2011.11.016; PMID: 22244070
- Oliva F, Via AG, Maffulli N. Role of growth factors in rotator cuff healing. Sports Med Arthrosc 2011; 19:218 - 26; http://dx.doi.org/10.1097/JSA.0b013e3182250c78; PMID: 21822105
- Garofalo R, Cesari E, Vinci E, Castagna A. Role of metalloproteinases in rotator cuff tear. Sports Med Arthrosc 2011; 19:207 - 12; http://dx.doi.org/10.1097/JSA.0b013e318227b07b; PMID: 21822103
- Hoffmann A, Pelled G, Turgeman G, Eberle P, Zilberman Y, Shinar H, Keinan-Adamsky K, Winkel A, Shahab S, Navon G, et al. Neotendon formation induced by manipulation of the Smad8 signalling pathway in mesenchymal stem cells. J Clin Invest 2006; 116:940 - 52; http://dx.doi.org/10.1172/JCI22689; PMID: 16585960
- Rodeo SA, Suzuki K, Deng XH, Wozney J, Warren RF. Use of recombinant human bone morphogenetic protein-2 to enhance tendon healing in a bone tunnel. Am J Sports Med 1999; 27:476 - 88; PMID: 10424218
- Thomopoulos S, Kim HM, Silva MJ, Ntouvali E, Manning CN, Potter R, Seeherman H, Gelberman RH. Effect of bone morphogenetic protein 2 on tendon-to-bone healing in a canine flexor tendon model. J Orthop Res 2012; 30:1702 - 9; http://dx.doi.org/10.1002/jor.22151; PMID: 22618762
- Gulotta LV, Kovacevic D, Ying L, Ehteshami JR, Montgomery S, Rodeo SA. Augmentation of tendon-to-bone healing with a magnesium-based bone adhesive. Am J Sports Med 2008; 36:1290 - 7; http://dx.doi.org/10.1177/0363546508314396; PMID: 18319348
- Kim HM, Galatz LM, Das R, Havlioglu N, Rothermich SY, Thomopoulos S. The role of transforming growth factor beta isoforms in tendon-to-bone healing. Connect Tissue Res 2011; 52:87 - 98; http://dx.doi.org/10.3109/03008207.2010.483026; PMID: 20615095
- Matsumoto T, Ingham SM, Mifune Y, Osawa A, Logar A, Usas A, Kuroda R, Kurosaka M, Fu FH, Huard J. Isolation and characterization of human anterior cruciate ligament-derived vascular stem cells. Stem Cells Dev 2012; 21:859 - 72; http://dx.doi.org/10.1089/scd.2010.0528; PMID: 21732814
- Matsumoto T, Kubo S, Sasaki K, Kawakami Y, Oka S, Sasaki H, Takayama K, Tei K, Matsushita T, Mifune Y, et al. Acceleration of tendon-bone healing of anterior cruciate ligament graft using autologous ruptured tissue. Am J Sports Med 2012; 40:1296 - 302; http://dx.doi.org/10.1177/0363546512439026; PMID: 22427618
- Mifune Y, Matsumoto T, Ota S, Nishimori M, Usas A, Kopf S, Kuroda R, Kurosaka M, Fu FH, Huard J. Therapeutic potential of anterior cruciate ligament-derived stem cells for anterior cruciate ligament reconstruction. Cell Transplant 2012; 21:1651 - 65; http://dx.doi.org/10.3727/096368912X647234; PMID: 22732227
- Mifune Y, Matsumoto T, Takayama K, Terada S, Sekiya N, Kuroda R, Kurosaka M, Fu FH, Huard J. Tendon graft revitalization using adult anterior cruciate ligament (ACL)-derived CD34+ cell sheets for ACL reconstruction. Biomaterials 2013; 34:5476 - 87; http://dx.doi.org/10.1016/j.biomaterials.2013.04.013; PMID: 23632324
- Wong MWN, Qin L, Tai JKO, Lee SKM, Leung KS, Chan KM. Engineered allogeneic chondrocyte pellet for reconstruction of fibrocartilage zone at bone-tendon junction--a preliminary histological observation. J Biomed Mater Res B Appl Biomater 2004; 70:362 - 7; PMID: 15264320
- Wong MWN, Qin L, Lee KM, Leung KS. Articular cartilage increases transition zone regeneration in bone-tendon junction healing. Clin Orthop Relat Res 2009; 467:1092 - 100; http://dx.doi.org/10.1007/s11999-008-0606-8; PMID: 18987921
- Nourissat G, Diop A, Maurel N, Salvat C, Dumont S, Pigenet A, Gosset M, Houard X, Berenbaum F. Mesenchymal stem cell therapy regenerates the native bone-tendon junction after surgical repair in a degenerative rat model. PLoS One 2010; 5:e12248; http://dx.doi.org/10.1371/journal.pone.0012248; PMID: 20805884
- Loeffler BJ, Scannell BP, Peindl RD, Connor P, Davis DE, Hoelscher GL, Norton HJ, Hanley EN Jr., Gruber HE. Cell-based tissue engineering augments tendon-to-bone healing in a rat supraspinatus model. J Orthop Res 2013; 31:407 - 12; http://dx.doi.org/10.1002/jor.22234; PMID: 23070709
- Tuan RS, Boland G, Tuli R. Adult mesenchymal stem cells and cell-based tissue engineering. Arthritis Res Ther 2003; 5:32 - 45; http://dx.doi.org/10.1186/ar614; PMID: 12716446
- Kuo CK, Tuan RS. Mechanoactive tenogenic differentiation of human mesenchymal stem cells. Tissue Eng Part A 2008; 14:1615 - 27; http://dx.doi.org/10.1089/ten.tea.2006.0415; PMID: 18759661
- Awad HA, Butler DL, Boivin GP, Smith FNL, Malaviya P, Huibregtse B, Caplan AI. Autologous mesenchymal stem cell-mediated repair of tendon. Tissue Eng 1999; 5:267 - 77; http://dx.doi.org/10.1089/ten.1999.5.267; PMID: 10434073
- Caplan AI. Adult mesenchymal stem cells for tissue engineering versus regenerative medicine. J Cell Physiol 2007; 213:341 - 7; http://dx.doi.org/10.1002/jcp.21200; PMID: 17620285
- Lim JK, Hui J, Li L, Thambyah A, Goh J, Lee EH. Enhancement of tendon graft osteointegration using mesenchymal stem cells in a rabbit model of anterior cruciate ligament reconstruction. Arthroscopy 2004; 20:899 - 910; PMID: 15525922
- Ouyang HW, Goh JCH, Lee EH. Use of bone marrow stromal cells for tendon graft-to-bone healing: histological and immunohistochemical studies in a rabbit model. Am J Sports Med 2004; 32:321 - 7; http://dx.doi.org/10.1177/0095399703258682; PMID: 14977654
- Soon MYH, Hassan A, Hui JHR, Goh JCH, Lee EH. An analysis of soft tissue allograft anterior cruciate ligament reconstruction in a rabbit model: a short-term study of the use of mesenchymal stem cells to enhance tendon osteointegration. Am J Sports Med 2007; 35:962 - 71; http://dx.doi.org/10.1177/0363546507300057; PMID: 17400750
- Gulotta LV, Kovacevic D, Ehteshami JR, Dagher E, Packer JD, Rodeo SA. Application of bone marrow-derived mesenchymal stem cells in a rotator cuff repair model. Am J Sports Med 2009; 37:2126 - 33; http://dx.doi.org/10.1177/0363546509339582; PMID: 19684297
- Gulotta LV, Kovacevic D, Packer JD, Ehteshami JR, Rodeo SA. Adenoviral-mediated gene transfer of human bone morphogenetic protein-13 does not improve rotator cuff healing in a rat model. Am J Sports Med 2011; 39:180 - 7; http://dx.doi.org/10.1177/0363546510379339; PMID: 20956264
- Wolfman NM, Hattersley G, Cox K, Celeste AJ, Nelson R, Yamaji N, Dube JL, DiBlasio-Smith E, Nove J, Song JJ, et al. Ectopic induction of tendon and ligament in rats by growth and differentiation factors 5, 6, and 7, members of the TGF-beta gene family. J Clin Invest 1997; 100:321 - 30; http://dx.doi.org/10.1172/JCI119537; PMID: 9218508
- Forslund C, Aspenberg P. Tendon healing stimulated by injected CDMP-2. Med Sci Sports Exerc 2001; 33:685 - 7; http://dx.doi.org/10.1097/00005768-200105000-00001; PMID: 11323533
- Gulotta LV, Kovacevic D, Packer JD, Deng XH, Rodeo SA. Bone marrow-derived mesenchymal stem cells transduced with scleraxis improve rotator cuff healing in a rat model. Am J Sports Med 2011; 39:1282 - 9; http://dx.doi.org/10.1177/0363546510395485; PMID: 21335341
- Gulotta LV, Kovacevic D, Montgomery S, Ehteshami JR, Packer JD, Rodeo SA. Stem cells genetically modified with the developmental gene MT1-MMP improve regeneration of the supraspinatus tendon-to-bone insertion site. Am J Sports Med 2010; 38:1429 - 37; http://dx.doi.org/10.1177/0363546510361235; PMID: 20400753
- Sekiya I, Vuoristo JT, Larson BL, Prockop DJ. In vitro cartilage formation by human adult stem cells from bone marrow stroma defines the sequence of cellular and molecular events during chondrogenesis. Proc Natl Acad Sci U S A 2002; 99:4397 - 402; http://dx.doi.org/10.1073/pnas.052716199; PMID: 11917104
- Thomopoulos S, Soslowsky LJ, Flanagan CL, Tun S, Keefer CC, Mastaw J, Carpenter JE. The effect of fibrin clot on healing rat supraspinatus tendon defects. J Shoulder Elbow Surg 2002; 11:239 - 47; http://dx.doi.org/10.1067/mse.2002.122228; PMID: 12070496
- Butler DL, Juncosa-Melvin N, Boivin GP, Galloway MT, Shearn JT, Gooch C, Awad H. Functional tissue engineering for tendon repair: A multidisciplinary strategy using mesenchymal stem cells, bioscaffolds, and mechanical stimulation. J Orthop Res 2008; 26:1 - 9; http://dx.doi.org/10.1002/jor.20456; PMID: 17676628
- Spalazzi JP, Dagher E, Doty SB, Guo XE, Rodeo SA, Lu HH. In vivo evaluation of a multiphased scaffold designed for orthopaedic interface tissue engineering and soft tissue-to-bone integration. J Biomed Mater Res A 2008; 86:1 - 12; http://dx.doi.org/10.1002/jbm.a.32073; PMID: 18442111
- Yokoya S, Mochizuki Y, Natsu K, Omae H, Nagata Y, Ochi M. Rotator cuff regeneration using a bioabsorbable material with bone marrow-derived mesenchymal stem cells in a rabbit model. Am J Sports Med 2012; 40:1259 - 68; http://dx.doi.org/10.1177/0363546512442343; PMID: 22491821
- Colnot C, Zhang X, Knothe Tate ML. Current insights on the regenerative potential of the periosteum: molecular, cellular, and endogenous engineering approaches. J Orthop Res 2012; 30:1869 - 78; http://dx.doi.org/10.1002/jor.22181; PMID: 22778049
- Li H, Jiang J, Wu Y, Chen S. Potential mechanisms of a periosteum patch as an effective and favourable approach to enhance tendon-bone healing in the human body. Int Orthop 2012; 36:665 - 9; http://dx.doi.org/10.1007/s00264-011-1346-z; PMID: 22009448
- Karaoglu S, Celik C, Korkusuz P. The effects of bone marrow or periosteum on tendon-to-bone tunnel healing in a rabbit model. Knee Surg Sports Traumatol Arthrosc 2009; 17:170 - 8; http://dx.doi.org/10.1007/s00167-008-0646-3; PMID: 18941736
- Chen CH, Chen WJ, Shih CH, Yang CY, Liu SJ, Lin PY. Enveloping the tendon graft with periosteum to enhance tendon-bone healing in a bone tunnel: A biomechanical and histologic study in rabbits. Arthroscopy 2003; 19:290 - 6; http://dx.doi.org/10.1053/jars.2003.50014; PMID: 12627154
- Chang CH, Chen CH, Su CY, Liu HT, Yu CM. Rotator cuff repair with periosteum for enhancing tendon-bone healing: a biomechanical and histological study in rabbits. Knee Surg Sports Traumatol Arthrosc 2009; 17:1447 - 53; http://dx.doi.org/10.1007/s00167-009-0809-x; PMID: 19440695
- Chen CH, Chang CH, Wang KC, Su CI, Liu HT, Yu CM, Wong CB, Wang IC, Whu SW, Liu HW. Enhancement of rotator cuff tendon-bone healing with injectable periosteum progenitor cells-BMP-2 hydrogel in vivo. Knee Surg Sports Traumatol Arthrosc 2011; 19:1597 - 607; http://dx.doi.org/10.1007/s00167-010-1373-0; PMID: 21327764
- Chen C-H, Chang C-H, Su C-I, Wang K-C, Liu H-T, Yu C-M, Wong CB, Wang IC. Arthroscopic single-bundle anterior cruciate ligament reconstruction with periosteum-enveloping hamstring tendon graft: clinical outcome at 2 to 7 years. Arthroscopy 2010; 26:907 - 17; http://dx.doi.org/10.1016/j.arthro.2009.11.011; PMID: 20620790
- Scheibel M, Brown A, Woertler K, Imhoff AB. Preliminary results after rotator cuff reconstruction augmented with an autologous periosteal flap. Knee Surg Sports Traumatol Arthrosc 2007; 15:305 - 14; http://dx.doi.org/10.1007/s00167-006-0173-z; PMID: 16927071
- Ellera Gomes JL, da Silva RC, Silla LMR, Abreu MR, Pellanda R. Conventional rotator cuff repair complemented by the aid of mononuclear autologous stem cells. Knee Surg Sports Traumatol Arthrosc 2012; 20:373 - 7; http://dx.doi.org/10.1007/s00167-011-1607-9; PMID: 21773831
- Gladstone JN, Bishop JY, Lo IKY, Flatow EL. Fatty infiltration and atrophy of the rotator cuff do not improve after rotator cuff repair and correlate with poor functional outcome. Am J Sports Med 2007; 35:719 - 28; http://dx.doi.org/10.1177/0363546506297539; PMID: 17337727
- Contopoulos-Ioannidis DG, Ntzani E, Ioannidis JPA. Translation of highly promising basic science research into clinical applications. Am J Med 2003; 114:477 - 84; http://dx.doi.org/10.1016/S0002-9343(03)00013-5; PMID: 12731504
- Moran CJ, Barry FP, Maher SA, Shannon FJ, Rodeo SA. Advancing regenerative surgery in orthopaedic sports medicine: the critical role of the surgeon. Am J Sports Med 2012; 40:934 - 44; http://dx.doi.org/10.1177/0363546511426677; PMID: 22085730
- Evans CH, Palmer GD, Pascher A, Porter R, Kwong FN, Gouze E, Gouze JN, Liu F, Steinert A, Betz O, et al. Facilitated endogenous repair: making tissue engineering simple, practical, and economical. Tissue Eng 2007; 13:1987 - 93; http://dx.doi.org/10.1089/ten.2006.0302; PMID: 17518747
- Jakob F, Ebert R, Rudert M, Nöth U, Walles H, Docheva D, Schieker M, Meinel L, Groll J. In situ guided tissue regeneration in musculoskeletal diseases and aging : Implementing pathology into tailored tissue engineering strategies. Cell Tissue Res 2012; 347:725 - 35; http://dx.doi.org/10.1007/s00441-011-1237-z; PMID: 22011785
- Derwin KA, Baker AR, Iannotti JP, McCarron JA. Preclinical models for translating regenerative medicine therapies for rotator cuff repair. Tissue Eng Part B Rev 2010; 16:21 - 30; http://dx.doi.org/10.1089/ten.teb.2009.0209; PMID: 19663651
- Longo UG, Forriol F, Campi S, Maffulli N, Denaro V. Animal models for translational research on shoulder pathologies: from bench to bedside. Sports Med Arthrosc 2011; 19:184 - 93; http://dx.doi.org/10.1097/JSA.0b013e318205470e; PMID: 21822100
- Voloshin I, Gelinas J, Maloney MD, O’Keefe RJ, Bigliani LU, Blaine TA. Proinflammatory cytokines and metalloproteases are expressed in the subacromial bursa in patients with rotator cuff disease. Arthroscopy 2005; 21:e1 - , e9; http://dx.doi.org/10.1016/j.arthro.2005.05.017; PMID: 16171632
- Lui PPY, Maffulli N, Rolf C, Smith RKW. What are the validated animal models for tendinopathy?. Scand J Med Sci Sports 2011; 21:3 - 17; http://dx.doi.org/10.1111/j.1600-0838.2010.01164.x; PMID: 20673247
- Wang JHC. Mechanobiology of tendon. J Biomech 2006; 39:1563 - 82; http://dx.doi.org/10.1016/j.jbiomech.2005.05.011; PMID: 16000201
- Ambrosio F, Wolf SL, Delitto A, Fitzgerald GK, Badylak SF, Boninger ML, Russell AJ. The emerging relationship between regenerative medicine and physical therapeutics. Phys Ther 2010; 90:1807 - 14; http://dx.doi.org/10.2522/ptj.20100030; PMID: 21030663
- Virchenko O, Aspenberg P. How can one platelet injection after tendon injury lead to a stronger tendon after 4 weeks? Interplay between early regeneration and mechanical stimulation. Acta Orthop 2006; 77:806 - 12; http://dx.doi.org/10.1080/17453670610013033; PMID: 17068715
- Lovric V, Ledger M, Goldberg J, Harper W, Bertollo N, Pelletier MH, Oliver RA, Yu Y, Walsh WR. The effects of low-intensity pulsed ultrasound on tendon-bone healing in a transosseous-equivalent sheep rotator cuff model. Knee Surg Sports Traumatol Arthrosc 2013; 21:466 - 75; http://dx.doi.org/10.1007/s00167-012-1972-z; PMID: 22466014
- Strauch B, Patel MK, Rosen DJ, Mahadevia S, Brindzei N, Pilla AA. Pulsed magnetic field therapy increases tensile strength in a rat Achilles’ tendon repair model. J Hand Surg Am 2006; 31:1131 - 5; http://dx.doi.org/10.1016/j.jhsa.2006.03.024; PMID: 16945715
- Chow DHK, Suen PK, Fu LH, Cheung WH, Leung KS, Wong MWN, Qin L. Extracorporeal shockwave therapy for treatment of delayed tendon-bone insertion healing in a rabbit model: a dose-response study. Am J Sports Med 2012; 40:2862 - 71; http://dx.doi.org/10.1177/0363546512461596; PMID: 23075803
- Song B, Zhao M, Forrester JV, McCaig CD. Electrical cues regulate the orientation and frequency of cell division and the rate of wound healing in vivo. Proc Natl Acad Sci U S A 2002; 99:13577 - 82; http://dx.doi.org/10.1073/pnas.202235299; PMID: 12368473
- Tsai MT, Li WJ, Tuan RS, Chang WH. Modulation of osteogenesis in human mesenchymal stem cells by specific pulsed electromagnetic field stimulation. J Orthop Res 2009; 27:1169 - 74; http://dx.doi.org/10.1002/jor.20862; PMID: 19274753
- Ju YJ, Muneta T, Yoshimura H, Koga H, Sekiya I. Synovial mesenchymal stem cells accelerate early remodeling of tendon-bone healing. Cell Tissue Res 2008; 332:469 - 78; http://dx.doi.org/10.1007/s00441-008-0610-z; PMID: 18418628
- Shahab-Osterloh S, Witte F, Hoffmann A, Winkel A, Laggies S, Neumann B, Seiffart V, Lindenmaier W, Gruber AD, Ringe J, et al. Mesenchymal stem cell-dependent formation of heterotopic tendon-bone insertions (osteotendinous junctions). Stem Cells 2010; 28:1590 - 601; http://dx.doi.org/10.1002/stem.487; PMID: 20882636
- Lui PPY, Zhang P, Chan KM, Qin L. Biology and augmentation of tendon-bone insertion repair. J Orthop Surg Res 2010; 5 14; http://dx.doi.org/10.1186/1749-799X-5-59; PMID: 20727196