Abstract
The primary cilium has emerged as a hotbed of sensory and developmental signaling, serving as a privileged domain to concentrate the functions of a wide number of channels, receptors and downstream signal transducers. This realization has provided important insight into the pathophysiological mechanisms underlying the ciliopathies, an ever expanding spectrum of multi-symptomatic disorders affecting the development and maintenance of multiple tissues and organs. One emerging research focus is the subcompartmentalised nature of the organelle, consisting of discrete structural and functional subdomains such as the periciliary membrane/basal body compartment, the transition zone, the Inv compartment and the distal segment/ciliary tip region. Numerous ciliopathy, transport-related and signaling molecules localize at these compartments, indicating specific roles at these subciliary sites. Here, by focusing predominantly on research from the genetically tractable nematode C. elegans, we review ciliary subcompartments in terms of their structure, function, composition, biogenesis and relationship to human disease.
Overview of Ciliary Compartmentalisation, Transport and Disease
Over the past 15 years more than 30 distinct human diseases, embracing at least 80 genes, have been associated with defects in cilia, which are motile or non-motile microtubule-based extensions protruding from most eukaryotic cell surfaces.Citation1,Citation2 These disorders, termed ciliopathies, display a wide range of overlapping pathologies including respiratory dysfunction (e.g., primary ciliary dyskinesia), cystic kidneys (e.g., polycystic kidney disease, nephronophthisis), organ laterality defects (e.g., situs inversus), retinal degeneration (e.g., retinitis pigmentosa), bone abnormalities (e.g., Meckel-Gruber syndrome), obesity (e.g., Bardet-Biedl syndrome) and central and peripheral nervous system defects (e.g., Joubert syndrome).Citation2 Collectively, these pleiotropic phenotypes underscore the functional importance of the cilium in a wide range of cellular processes that includes cell and fluid movement, phototransduction, chemosensation, and mechanosensation, as well as developmental signaling involving sonic hedgehog and possibly Wnt, TGF-β, PDGFα, and Notch ligands.Citation3-Citation9
Conducting these functions are a diverse range of receptors, channels, signaling molecules, transport machineries and ciliopathy proteins that are enriched or almost exclusively found in cilia. While many of these proteins localize along the entire axoneme, others are asymmetrically distributed at distinct ciliary subdomains such as the transition zone and Inv (inversin) compartments, indicating specific roles and disease mechanisms for these subregions.Citation10,Citation11 Protein transport and restriction to cilia is driven by various polarized ciliary transport regulators, as well as by cytosolic and membrane diffusion barriers at the ciliary base that restrict access to the organelle and preserve its composition.Citation10,Citation12-Citation18 One key pathway is intraflagellar transport (IFT), which operates bi-directionally along ciliary microtubules to deliver and distribute “cargo” proteins required for cilium structure and function.Citation19,Citation20 The IFT machinery consists of anterograde (kinesin-2) and retrograde (cytoplasmic dynein) motors, as well as an associated IFT particle comprised of two subcomplexes, A and B. Ciliary protein transport also heavily relies on secretory and endocytic membrane regulators such as coat adaptors, small GTPases and the exocyst complex, all of which facilitate vesicle-derived ciliary membrane (and associated protein) delivery and retrieval.Citation21-Citation33 Multiple studies also report that passage of proteins into cilia employs cilium-localized nucleocytoplasmic transport regulators (karyopherins) and nuclear pore complex proteins (nucleoporins),Citation17,Citation34-Citation37 although there is some uncertainty about the latter based on recent conflicting evidence.Citation38 For delivery of ciliary proteins, one scenario is vesicle trafficking via secretory pathways to the periciliary membrane, followed by IFT-facilitated entry into cilia. However, this model is overly simplistic because some membrane proteins enter cilia independent of IFT.Citation39 Furthermore, soluble proteins below a certain size can enter the cilium via simple diffusion, indicating IFT may traffic only relatively large proteins (>40–100 kDa).Citation17,Citation38 Thus, protein trafficking and sorting to cilia depends on multiple pathways, with no universal mechanism, including the lack of universal ciliary protein sorting motifs.
In this review, we focus on the various subciliary domains, namely the periciliary membrane/basal body, transition zone, Inv, and distal segment/tip compartments. Predominantly, we focus on research from the Caenorhabditis elegans nematode that has led to a greater understanding of subcompartment composition, biogenesis, function, transport, and relationship to human disease.
Subcompartments of Cilia
All eukaryotic cilia can be subdivided into distinct subcompartments, each with unique ultrastructure and protein compositions (). The most proximal subcompartment is the mother centriole-derived basal body (BB) and surrounding periciliary membrane, which invaginates in some mammalian cells and Trypanosomes to form a ciliary pocket.Citation27 The BB centriole, normally consisting of 9 triplet microtubules, nucleates ciliary axoneme extension and is plasma membrane-docked via distal appendages, which are also called transition fibers (not to be confused with the Y-links of the adjacent transition zone subcompartment; see below).Citation40 These fibers anchor the mother centriole during early ciliogenesis and may form part of a ciliary pore, with septins and possibly nuclear pore complex proteins, regulating protein and membrane transport across the ciliary base.Citation10,Citation16,Citation17,Citation40,Citation41 Indeed, in green algae, distal appendages organize the “ciliary bracelet”, a membrane specialization defined by intramembrane particle arrays and absence of certain sterols, which could act as a barrier to lateral membrane transport.Citation42,Citation43 Distal appendages also represent a probable site of docking, assembly, and turnaround of IFT trains.Citation44 Additional accessory features of the BB are microtubule-associated subdistal appendages, basal feet and ciliary rootlets, all of which are thought to anchor, stabilize and position the centriole and associated axoneme.Citation45,Citation46 The periciliary membrane enveloping the BB also serves as a docking site for vesicles carrying cilium-destined cargos, and a zone of endocytosis and membrane/receptor retrieval.Citation18,Citation24,Citation27,Citation33
Figure 1. Structural basis of canonical ciliary subcompartments. (A) Left; representation of a canonical C. elegans amphid channel sensory neuronal cilium. Ciliary microtubules (MT) extend from a fully degenerated basal body at the ciliary base, with microtubules flaring proximally into the periciliary membrane compartment (PCMC). The PCMC is a swelling of the distal dendrite tip, bounded at its proximal side by a belt-like adherens junction between the sensory neuron and the enveloping sheath support cell (not shown). An ~0.8 μm long ciliary transition zone emerges from the ciliary base, consisting of nine closely tethered doublet microtubules (via an internal apical ring (AR); see cross section), with each doublet connected to the ciliary membrane via Y-links. Also present are inner singlet microtubules (gray) extending to varying degrees along the axoneme. The TZ is followed by an ~4 μm long middle segment consisting of 9 doublet microtubules, after which the B-tubule of each doublet terminates to establish the nine singlet A-tubule arrangement of the ~3 μm long distal segment. Right; representation of a canonical human primary cilium, showing the basal body (and associated distal (DA) and subdistal (sDA) appendages), transition zone and main axonemal compartments. Example also shows the emergence of the cilium from a ciliary pocket, which is an invagination of the periciliary membrane observed for some ciliary subtypes. (B) Schematic of the amphid sensillum—the largest sensory organ in C. elegans—in the nematode nose region. Ten ciliary axonemes occupy the environmentally exposed channel (only 3 shown for illustration purposes), created by ciliary axonemes punching through the surrounding sheath (glial) cell. Belt-like adherens junctions (AJ) at the base of each periciliary membrane compartment (PCMC) seal the channel.
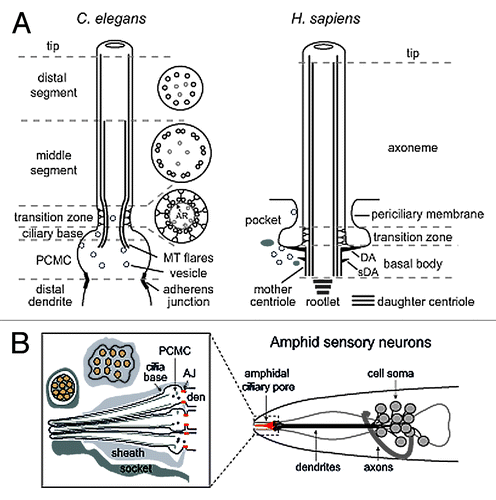
Emanating from the BB is the transition zone (TZ) compartment, which forms the first 0.5–1.0 μm of the microtubule doublet-containing ciliary axoneme.Citation10,Citation47 The TZ is distinguished ultrastructurally by characteristic three dimensional Y-link structures of unknown composition that connect each of the nine doublets with the ciliary membrane (). Multiple studies implicate the TZ as a ciliary gate, imparting physical and molecular diffusion barriers to cytosolic and membrane protein transport across the ciliary base, thereby serving to separate the cilium from the rest of the cell.Citation12-Citation15,Citation48 Indeed, like the BB distal appendages in algae, Y-links in protists and mammals organize a TZ membrane specialization termed the “ciliary necklace”, consisting of concentric rings of intramembane particles.Citation49 Consistent with the TZ being a distinct ciliary subcompartment, numerous proteins implicated in Nephronophthisis, Meckel-Gruber syndrome and Joubert Syndrome specifically localize within this domain and regulate gating, perhaps as structural subunits of the Y-links.Citation12-Citation15,Citation48
The ciliary axoneme distal to the TZ frequently displays distinct proximo-distal patterning at the level of ultrastructure and protein localization, indicative of subcompartmentalisation and regionalised sensory and signaling functions. For example, variations in microtubule (MT) arrangement define proximal (doublet MTs) and distal (singlet MTs) ciliary segments in nematodes, mating green algae, and various vertebrate cell types (olfactory, pancreatic, renal).Citation50-Citation54 Furthermore, mammalian ciliopathy proteins such as Inversin/NPHP2, NPHP3, NPHP9, and ARL13B (in some cell types) localize specifically to a proximal ciliary region (excluding the TZ) termed the Inv compartment.Citation11,Citation48,Citation55 Various proteins are also concentrated in distal ciliary regions and at the ciliary tip, including frog olfactory CNG channels and sonic hedgehog signaling proteins such as Gli1/2/3 and Sufu.Citation56-Citation58
C. elegans as a Model for Investigating Cilium Biology and Compartmentalisation
In the C. elegans adult hermaphrodite of 959 somatic cells, non-motile primary cilia extend from the distal dendrite tips of 60 sensory neurons (). Most cilia are part of environmentally exposed pores (sensillae) in the cuticle, serving chemo-, thermo-, and osmolarity- sensing functions.Citation50 Importantly, many genes associated with cilium biogenesis, transport and disease are conserved in C. elegans. Also, worms possess an extensive experimental toolbox for cilium research such as the availability of mutant alleles, behavioral assays for cilium function, transport assays for IFT and vesicular trafficking, and fluorescence assays for determining precise protein localizations at the subciliary level. Thus, despite lacking most organs associated with mammalian cilia function and disease, C. elegans has emerged as a leading metazoan model for investigating basic underpinning cilium biology and pathomechanisms at the cellular level.
Worm research has been instrumental in understanding the molecular basis of IFT, its regulation, and ciliary subtype variation.Citation19,Citation59 Furthermore, C. elegans research has provided mechanistic insight into ciliopathy protein function such as the role of BBS proteins and Joubert Syndrome-associated ARL-13/ARL13B in IFT regulation, and ciliary gating functions for MKS and NPHP at the TZ.Citation15,Citation60-Citation64 Various membrane trafficking regulators have also been linked to C. elegans cilium formation and protein transport, including clathrin adaptor (AP1, AP2) subunits, various small GTPases (RAB-5, RAB-8), OSTA-1 (organic solute transporter α-like protein), and SQL-1/GMAP210 (golgi microtubule associated protein).Citation24,Citation25,Citation65-Citation67 Finally, nematode forward genetics, transcriptomics and bioinformatics approaches have identified new conserved ciliary proteins, including IFT components DYF-1/IFT70, DYF-2/IFT144, DYF-11/IFT54, DYF-13/TTC26, and IFTA-1/IFT121.Citation63,Citation68-Citation72
C. elegans cilia possess heterogeneous morphologies ranging from rod-shaped to organelles that are forked, branched or with membrane expansions.Citation73,Citation74 Most research has focused on the bilateral amphid (head) and phasmid (tail) olfactory sensillae, consisting of 12 and 2 ciliated cells, respectively. In these pores, axonemes are typically rod-shaped, possessing the canonical subcompartments described above, with some notable distinctionsCitation50,Citation73,Citation74 (). First, the ciliary base lacks a basal body centriole and associated accessory structures.Citation74 Until recently, it was thought that distal appendages are present at the ciliary base; however, a stunning new electron tomography study of 50 3-D reconstructed nematode cilia now shows that the “appendage-type” electron densities are likely to be a flaring out of the ciliary doublet microtubules.Citation73 Thus, it would appear that once the mother centriole has docked to the plasma membrane during cilium initiation, all centriolar and accessory structure is lost. Second, the ciliary base sits at the apex of an ~0.5 μm2 asymmetric swelling of the dendrite tip, termed the periciliary membrane compartment (PCMC).Citation24 As will be discussed below, the PCMC is enriched for various ciliary proteins, and serves as a site of homeostatic regulation of ciliary membrane trafficking. Third, amphid and phasmid channel cilia possess bipartite microtubule arrangements that define a proximal or ‘middle segment’ consisting of doublet MTs, and a “distal segment” comprised of singlet MTs due to termination of each doublet B-tubule at the mid-point of the axoneme.Citation74 These subcompartmental distinctions, while perhaps unusual, are not specific to C. elegans. For example, mature rat sperm flagella lack a basal body centriole and ciliary A-tubule extensions are found in vertebrate cells.Citation51,Citation75,Citation76 Also, as will be discussed, the middle segment is analagous to the mammalian Inv compartment. Below follows a detailed description of the nematode ciliary subcompartments, with a focus on their composition, functions, and relationship to disease.
C. elegans Ciliary Base and Periciliary Membrane Compartments
Despite lacking an anchoring mother centriole and associated appendages,Citation73,Citation74 the nematode ciliary base appears to retain the functionality of a basal body. In the first instance, counterparts of many vertebrate basal body-associated proteins are also found at the nematode ciliary base, beneath the transition zone. These include IFT particle proteins, the BBSome, the ciliary rootlet-associated rootletin protein, FBF1/DYF-19 and centriole-associated HYLS-1 (hydrolethalus syndrome 1) ().Citation15,Citation19,Citation29,Citation60,Citation77-Citation79 In C. elegans, BBSome proteins control anterograde kinesin-2 motor associations and IFT turnaround, and DYF-19 regulates ciliary entry of assembled IFT machinery.Citation64,Citation79 Similar to its mammalian counterpart, C. elegans striated rootletin stabilizes ciliary axonemes.Citation78,Citation80 Also, non-filamentous rootletin stabilizes nematode cilia lacking rootlets, perhaps by regulating vesicular trafficking in the PCMC.Citation78 Thus in worms, a centriole and associated appendages, including distal appendages, are not required for stabilizing ciliary axonemes and maintaining a transport hub at the ciliary base. Instead, these functions may be facilitated by the proximal ends of the nematode ciliary microtubules, which extend proximally into the PCMC.Citation73
Figure 2.C. elegans proteins with distinct roles in specific subciliary compartments. (A) Proteins known to be enriched at the periciliary membrane compartment (PCMC) and ciliary base region. These include multiple proteins involved in secretion (orange) and endocytosis (yellow), as well as intraflagellar transport (IFT; green). Other enriched proteins are shown in red. The periciliary membrane is thought to be compartmentalised from the ciliary membrane via a lateral diffusion barrier at the transition zone. Although unproven, the belt-like adherens junction may serve to compartmentalise the PCMC from more proximal dendritic membrane. OSTA-1; organic solute transporter anion-1, DYN-1; dynamin, AP2; clathrin adaptor 2 complex, CHC; clathrin heavy chain, STAM-1; endosomal signal transducing adaptor molecule, HGRS-1; endosomal hepatocyte growth factor-regulated tyrosine kinase substrate, BBS; Bardet-Biedl syndrome complex (BBSome), A; IFT subcomplex A, B; IFT subcomplex B, HYLS-1; Hydrolethalus syndrome protein 1, RPI-2; retinitis pigmentosa gene 2, PC1/2; Polycystin 1/2, Tram; translocating chain-associated membrane protein (translocon), GPCRs; G-protein coupled receptors, OA-; organic anion (B) At least 9 proteins linked to ciliopathies are specifically localized in the transition zone (TZ). These are MKS-1/BBS13, MKS-3/TMEM67, MKS-5/RPGRIP1L, MKS-6/Cc2d2a, MKSR-1/B9D1, MKSR-2/B9D2, JBTS-14/TMEM-237, NPHP-1/NPHP1 and NPHP-4/NPHP4. Genetic analyses in C. elegans indicate these proteins form distinct, but functionally associated, modules (‘MKS’ and ‘NPHP’), possibly linked via MKS-5. Together, these modules establish TZ connections with the ciliary membrane by regulating the assembly of Y-links. Also, these modules establish the transition zone as a ciliary gate, regulating lateral diffusion of membrane proteins into and out of the cilium (e.g., as shown for RPI-2). MS; middle segment, PCMC; periciliary membrane compartment. (C) Middle segment (MS) localization of palmitoylated ARL-13/ARL13B, NPHP-2/Inversin and various cyclic gated nucleotide (CNG) channels. Also shown is an anterograde IFT assembly, which in amphid channel ciliary middle segments is powered by the coordinated (and redundant) activities of two kinesin-2 motors, heterotrimeric kinesin-II (kin-II) and homodimeric OSM-3/KIF17. Lateral diffusion barriers flanking the compartment prevent ARL-13 from diffusing out of the compartment into the transition zone (TZ) and distal segment (DS) compartments. ARL-13 can also associate with IFT trains as potential cargo. (D) Assembly of distal segments (DS) via OSM-3 powered anterograde IFT. At tip of middle segment (MS), kinesin-II turns around and is trafficked back to the ciliary base via IFT-dynein (cDHC1b) powered retrograde IFT, leaving OSM-3 to drive anterograde transport of the IFT particle (A and B) and associated cargo to the ciliary tip. At tip, OSM-3 becomes retrograde IFT cargo.
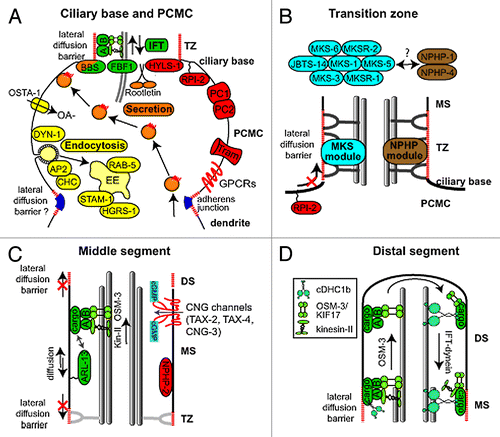
As outlined above, the degenerated nematode ciliary base is typically surrounded by a morphologically distinct patch of periciliary membrane, or PCMC, demarked proximally by a circumferential adherens (belt) junction between the ciliated neuron and supporting glial cell. We consider this swelling of the distal dendrite tip region an adjunct ciliary subcompartment because of its specific enrichment for multiple cilia-related sensory signaling and transport proteins. For example, ciliary GPCRs such as ODR-10 (odorant reception), SRBC-66 (dauer signaling), SRG-64, and SRG-36 display prominent localizations at the periciliary membrane relative to all other neuronal plasma membranes.Citation24,Citation66 Although the functional significance of these periciliary pools is not understood, they may serve to provide the cilium with rapid access to receptor molecules upon sensory stimulation. In this context, the PCMC would act as a transit station or surplus reservoir for molecules yet to cross into the cilium.
Additional PCMC enriched proteins (but mostly excluded from cilia) include established and proposed regulators of membrane trafficking. For example, early endosomal proteins STAM-1 (endosomal signal transducing adaptor molecule) and HGRS-1 (endosomal hepatocyte growth factor-regulated tyrosine kinase substrate; Hrs) localize at the PCMC and regulate the ciliary localization of LOV-1 (polycystin-1 ortholog) by preventing its accumulation in the PCMC.Citation67 In a recent report, the Sengupta lab described a novel endocytic role for PCMC-localized OSTA-1 (organic solute transporter anion), which genetically interacts with AP-2 clathrin adaptors, regulates RAB-5 vesicle flux, and facilitates the ciliary localization of various GPCRs.Citation66 Also, we and others reported PCMC localizations for RAB-5, DPY-23 (mu2 subunit of AP-2 clathrin adaptor), and DYN-1 (dynamin) and roles for these endocytic regulators in controlling ciliary and PCMC membrane volume in a RAB-8 and BBS-8 dependent manner, as well as the ciliary targeting of sensory signaling and IFT molecules.Citation24,Citation67 Indeed, this study allowed us to propose that C. elegans PCMC/ciliary membrane homeostasis is regulated by endocytosis-driven membrane retrieval, counterbalanced by BBS-8 and RAB-8-mediated membrane delivery.Citation24 In agreement with this notion, an exciting recent study from the Barr lab demonstrated that biologically active extracellular vesicles that regulate mating behavior are shed from the periciliary membrane, and that this process is dependent on IFT.Citation81 Similar findings have been made in Chlamydomonas, where bioactive ectosomes regulating daughter cell liberation following mitosis are shed from the flagellum, although in this instance vesicles derive from the tip rather than the base.Citation82 Together, the above findings implicate the PCMC as an active zone of endo- and exo-cytosis, similar to what has been described for the ciliary and flagellar pockets of protists and mammalian cells.Citation27,Citation33,Citation83
Other notable C. elegans proteins enriched at the PCMC, and specifically the periciliary membrane, include membrane-associated RPI-2 (ortholog of retinitis pigmentosa gene 2; RP2) and the glycoprotein TRAM-1 (ortholog of translocating chain-associated membrane protein; TRAM).Citation15,Citation21,Citation69 In Trypanosomes and mammalian cells, RP2 also localizes to the ciliary base where it is reported to link secretory trafficking between the Golgi and the cilium by facilitating docking of Golgi-derived vesicles to the ciliary base.Citation84,Citation85 The apparent periciliary membrane localization of the Sec61 ER translocon component TRAM-1 is particularly intriguing, and could suggest the presence of dendritic ER in the PCMC, perhaps in close apposition with the plasma membrane as ER-plasma membrane contact points.Citation86-Citation88 However, conventional chemical fixation techniques have not as yet revealed ER ultrastructure in the nematode PCMC and thus it remains a distinct possibility that the TRAM-1 PCMC signals reflect an atypical translocon functioning at the nematode periciliary membrane.
How various transmembrane (e.g., GPCRs) and membrane-associated (e.g., RPI-2) proteins are enriched at the periciliary membrane remains poorly understood. One possibility is anchoring to the underlying cytoskeleton via retention domains. For example, mammalian podocalyxin is excluded from a ciliary membrane domain by interacting with cortical actin via a PDZ motif.Citation89 Another non-mutually exclusive mechanism involves flanking diffusion barriers preventing lateral diffusion out of a periciliary membrane patch. Distally, these barriers likely involve septin rings and transition zone proteins (see next section). Proximally, at least in the case of C. elegans sensory neurons, adherens junctions may prevent diffusion of PCMC proteins into the dendritic compartment ().
C. elegans Transition Zone Compartment
Extending from the cilium base is a ~0.8 μm long transition zone (TZ).Citation74 Narrower than the rest of the axoneme, the TZ forms a constricted ring of doublet microtubules, with reduced inter-doublet spacings. Tethered to the inner face of the outer doublet microtubules is a membranous (apical) ring which draws toward it varying numbers of inner singlet microtubules that elongate distally into the axoneme. Characteristic Y-links extend from the junction between the A and B tubules and connect with the TZ membrane. Although vesicles are thought to be largely excluded from cilia, a recent report in C. elegans clearly shows that the vesicles are found in ciliary transition zones.Citation73 With the possible exception of the Y-links (discussed below), little is known regarding the functional relevance of most TZ ultrastructural features.
A primary reason for the recent interest in the TZ is that multiple ciliopathy proteins are specifically localized within this compartment, with some of the first observations made in nematodes. In C. elegans, at least 9 ciliopathy proteins are found at the TZ, including NPHP-1, NPHP-4, MKS-1, MKSR-1/B9D1, MKSR-2/B9D2, MKS-3/TMEM67, MKS-5/RPGRIP1L, MKS-6/Cc2d2a, and JBTS-14/TMEM237 ().Citation15,Citation90 Functional analyses using single mutant alleles revealed no obvious roles for most of these proteins in cilium structure and TZ integrity, although subtle cilia subtype-specific ultrastructural and IFT defects are observed in nphp-4 mutants, and mks-5 mutants possess a reduced dye-filling phenotype indicative of a cilium structure defect.Citation15,Citation91-Citation93 However, double mutant worms possessing loss of function alleles in an MKS/MKSR/JBTS-14 gene together with an NPHP gene, but not two genes from the same group, display relatively severe phenotypes, including truncated and misshapen cilia, misplaced axonemes, and chemosensory defects.Citation15,Citation90,Citation92 Furthermore, TZ morphogenesis and placement is severely impacted; TZs lack most or all Y-link connectors and are frequently de-anchored from the plasma membrane, extending instead from ectopic proximal positions within the distal dendrite.Citation15,Citation90 Also, in phasmid neurons, MKS/NPHP double mutants possess short dendrites, leading to the suggestion that during neuronal differentiation, the membrane-spanning TZ anchors the tip of the nascent dendrite to supporting cells or the extracellular matrix.Citation15,Citation92 This is because in C. elegans, dendrite elongation occurs via a trailing model, where an anchored dendrite is laid down behind a migrating cell soma.Citation94 Thus, in C. elegans, MKS and NPHP genes act in a functionally redundant manner to establish physical connections between microtubules, membranes and the ECM, required for ciliary docking and dendrite elongation.
The synthetic genetic associations observed in C. elegans implicate a modular organization of MKS, MKSR, and NPHP proteins, with NPHP-1/4 comprising an ‘NPHP’ module, and MKS-1/3/6 and MKSR-1/2 proteins comprising an ‘MKS’ module.Citation15 Consistent with this idea, NPHP-4 is required for TZ localization of NPHP-1, and MKS/MKSR proteins display interdependent TZ localizations; also, proteins of one module are not required for the TZ localization of proteins from the other module, indicating distinct pathways for assembly at the TZ.Citation15,Citation92,Citation95 The one exception is MKS-5, required for the TZ assembly of all examined NPHP and MKS/MKSR proteins; thus, MKS-5 is proposed to be a central component in docking MKS and NPHP modules at the TZ, perhaps as a linker between the two modules.Citation15
MKS and NPHP module genes also facilitate a bidirectional membrane diffusion barrier at the TZ that prevents leakage of periciliary membrane proteins (RPI-2; TRAM-1) into cilia, and leakage of ciliary membrane-associated ARL-13 out of cilia.Citation15,Citation48 These phenotypes were observed in single MKS and NPHP gene mutants, indicating barrier defects despite grossly normal TZ ultrastructure.Citation15 Interestingly, using quantitative imaging and an in vivo FRAP assay to assess flux kinetics across the TZ, we found that ARL-13 leakage out of cilia is enhanced in double mutants lacking Y-links, compared with the corresponding single mutants.Citation48 Thus, similar to what is found for the structural integrity of the TZ, MKS and NPHP genes function in a non-redundant and synthetic fashion to establish a TZ membrane diffusion barrier. Interestingly, TZ formation and barrier functions generally do not require IFT genes,Citation15 although the TZ barrier to ARL-13 diffusion partially depends on the putative IFT-B component, DYF-13/TTC26.Citation48 These nematode data are consistent with mammalian and Chlamydomonas studies showing that TZ-localized proteins comprise a diffusion barrier that controls ciliary and flagellar membrane protein composition.Citation12-Citation14
Biochemical interaction data from mammalian cells also implicate TZ components as part of distinct MKS/JBTS and NPHP modules. The MKS/JBTS module comprises at least 12 TZ-localized components that form a dense protein interaction network.Citation96 This module includes essentially all the proteins genetically associated with the C. elegans MKS module, as well as tectonic (TCTN1/2/3), TMEM (TMEM17/216/231), and AHI1 proteins. The NPHP module includes a TZ-localized subcomplex of three interconnected proteins, NPHP1, NPHP4, and NPHP8/MKS5.Citation97 Consistent with a distinct functional nature, there are few biochemical associations between NPHP1/4/8 and components of the MKS/JBTS module.Citation96 Interestingly, the mammalian NPHP module is also thought to include two additional subcomplexes, NPHP2/3/9 and ATXN10/NPHP5/NPHP6, which localize predominantly to the Inv and basal body compartments, respectively.Citation55,Citation97 Thus, these subcomplexes expand NPHP module functions beyond the TZ into neighboring ciliary compartments.
Although it is not known how NPHP/MKS/JBTS modules facilitate the ciliogenic and membrane diffusion barrier functions of the TZ, one model is regulation of TZ membrane properties such as lipid composition, fluidity and steric restriction. It has also been suggested that TZ modules are structural components of the microtubule-anchored Y-links which may directly organize biochemical and biophysical features of the TZ membrane.Citation96 As structural subunits, NPHP proteins could anchor Y-links to the axoneme via their reported ability to interact with microtubules, whereas the C2/B9 lipid binding domains of MKS-1/2/5/6 and MKSR-1/2, together with various TMEM proteins, could tether Y-links to the TZ membrane.Citation96 Furthermore, the coiled-coiled domains of CEP290/NPHP6, MKS-6/Cc2d2a, and MKS5 could form the Y-link stalk.Citation96 Consistent with these data, Chlamydomonas CEP290 localizes at the Y-links,Citation13 and efforts are on-going using immunogold labeling and super-resolution imaging to reveal the precise ultrastructural localizations of other TZ proteins. Also, the further dissection of the TZ membrane and protein environment, its fluidity and diffusive properties is being made possible by strategies employing lipid reporters and FRAP assays, and at least in Chlamydomonas, it seems TZ membranes can be isolated and biochemically analyzed.
Middle Segment/Inv Compartment
Extending from the TZ of most amphid and phasmid cilia is the middle segment compartment, defined ultrastructurally by a canonical arrangement of nine outer doublet microtubules.Citation74 At the distal end of middle segments, the B-tubule of each doublet terminates, thus signaling the transition to the microtubule singlet (A-tubule)-containing distal segment, discussed in the next section. Most amphid/phasmid ciliary middle segments are ~4 μm long, although that of the amphid winged cilium (AWB), which lies adjacent to the amphid channel, is shorter at ~2 μm.Citation98 Notably, 38 of the 60 ciliated neurons lack a middle/distal segment distinction; instead, doublet microtubules extend to the ciliary tips, either in canonical (ring of 9 doublets) or atypical arrangements.Citation50 In amphid and phasmid neurons, two kinesin-2 motors, canonical heterotrimeric kinesin-II and an accessory OSM-3-kinesin (KIF17 ortholog), cooperate to drive middle segment anterograde IFT and function in a fully redundant manner to build this subdomain.Citation99
The middle segment appears analogous to the vertebrate Inv compartment, initially described in mouse renal epithelial cells based on specific localization of the NPHP2/INV ciliopathy protein to a proximal ciliary segment, excluding the TZ.Citation11 Since then, several other ciliopathy-associated Inv compartment proteins have been identified including NPHP3, NPHP9/NEK8, ANKS6, and ARL13B,Citation48,Citation55,Citation100 and some of these proteins (Arl13b, NPHP2) dynamically exchange at the compartment’s membrane.Citation11,Citation101 Consistent with the middle segment being an Inv-like compartment, C. elegans NPHP2 and ARL13B orthologs also localize specifically at the middle segment and nematode ARL13B (ARL-13) exchanges at the membrane ().Citation48,Citation61,Citation62,Citation102 Middle segment restriction is also observed for various cyclic nucleotide-gated (CNG) cation channel subunits TAX-2, TAX-4, and CNG-3.Citation103,Citation104
The middle segment displays cilium subtype and developmental stage heterogeneity. For example, in AWB cilia, ARL-13, TAX-4, and CNG-3 localize to middle and distal segments, although middle segment enrichment is still observed.Citation61,Citation104 Similarly, in mouse cells, despite proximal ciliary restriction of Arl13b in tracheal and oviduct cilia,Citation48 this protein decorates the entire ciliary axoneme of other cell types.Citation14,Citation105,Citation106 Similar observations have been made for mammalian Inversin/NPHP2.Citation97 Furthermore, the middle segment is developmentally regulated because in C. elegans phasmid neurons, ARL-13 localization extends to the tips of newly formed cilia, before quickly restricting to a proximal subdomain as the worm ages.Citation48
How proteins are targeted to and restricted at the Inv compartment remains unclear. One obvious targeting mechanism is IFT, which in C. elegans prevents accumulation of ARL-13 and TAX-2/4 at the periciliary membrane.Citation48,Citation104 Consistent with an active transport model, ARL-13 can undergo IFT-like movement in developing cilia and human ARL13B associates with IFT complex B via IFT46/74 interactions.Citation48 However, because IFT operates along the entire cilium length, it remains unclear how IFT delivers proteins to the middle segment. One possible solution is that middle segment proteins dock with the kinesin-II motor, which operates only to the middle segment tip before turning around. In support of this notion, TAX-2/4 targeting to the middle segment of AWB cilia depends on kinesin-II, but not OSM-3-kinesin.Citation104 However, this is not a universal mechanism because middle segment targeting of TAX-2 in ASK cilia is independent of kinesin-II and OSM-3-kinesin.Citation104 Thus, either the two motors are functionally redundant or TAX-2 targeting to ASK cilia employs another as yet unidentified IFT motor, or is IFT independent.
Targeting and restriction of proteins to the middle segment also involves membrane diffusion barriers that block lateral diffusion out of the compartment. As outlined above, MKS and NPHP proteins regulate a TZ barrier to ARL-13 diffusion and may represent a general strategy for proximal gating of the middle segment domain.Citation48 However, little is known about presumed barriers at the distal side, which lack all defining ultrastructural features of the TZ. Like the TZ, a distal barrier may possess distinct lipid compositions or steric properties that prevent middle segment protein associations. Interestingly, in Trypanosomes, detergent-resistant membrane (DRM) patches are enriched in the distal flagellum vs. the proximal/mid flagellum,Citation107 thus potentially allowing the distal ciliary association or exclusion of particular membrane proteins.
Aside from establishing flanking barriers, distinct lipid compositions in the Inv compartment itself may retain proteins with lipid-anchoring post-translational acylation modifications such as myristoylation and palmitoylation. Such modifications are already known to facilitate ciliary targeting of mammalian NPHP3, a Trypanosome calcium-binding protein, and C. elegans and mammalian Arl13b/ARL-13.Citation61,Citation62,Citation108,Citation109 In the case of mammalian NPHP3, its ciliary localization depends on a GTPase activation cycle involving ARL3 and its cognate activator, RP2, which regulates release of myristoylated NPHP3 from an inhibitory interaction with UNC-119, thus allowing association with the compartment’s membrane.Citation110 This mechanism probably also involves NPHP2, which was shown to act as an intraciliary molecular anchor to stabilize the Inv compartment localization of NPHP3 (and NEK8).Citation55 Such stabilizations are likely to involve interactions with the cytoskeleton and indeed an early report showed enrichment of actin in the proximal region of quail oviduct cilia.Citation111 Finally, it is interesting to note that N-terminal palmitoylation of C. elegans ARL-13, and possibly mammalian Arl13b, prevents nuclear targeting, which suggests this reversible modification regulates shuttling between the nucleus and the Inv domain. Indeed, mammalian Arl13b possesses a candidate nuclear targeting sequence and there is evidence of nuclear Arl13b signals.Citation101 Functional connections between the Inv domain and the nucleus are also suggested by localization of NPHP9/Nek8 and Inversin to both compartments.Citation112-Citation114
The sequestration of multiple ciliopathy proteins and channels/receptors within the Inv domain implicates important ciliogenic, sensory, signaling and transport-related roles for this compartment. For example, C. elegans ARL-13 regulates cilium length and morphology, chemo- and osmosensation, middle segment association of OSM-3 and kinesin-II motors, and the ciliary levels of PKD-2 and the TRPV channel, OSM-9.Citation61,Citation62 Similarly, mammalian Arl13b controls the distribution and exchange dynamics of Shh proteins and regulates neuronal migration and radial glial scaffold formation.Citation101,Citation105,Citation115,Citation116 In C. elegans, NPHP-2 regulates the ciliary localization (AWB) of at least one sensory signaling molecule, TAX-2, and genetically interacts with TZ-localized MKS and NPHP components to control cilium formation, TZ placement and dendrite length, thus establishing functional relationships between middle segment and TZ compartments.Citation102,Citation104 Similar domain interactions have been described in mammals, with NPHP2 biochemically interacting with TZ-localized proteins B9D2, RPGRIP1L, and NPHP4, as well as the MKS1/MKS6/TECT2 submodule.Citation97,Citation117,Citation118 Indeed, a number of important signaling and cellular functions have been described of mammalian Inv compartment proteins, with NPHP2 linked to planar cell polarity (PCP) signaling and NPHP9 to the DNA damage response.Citation119-Citation122 However, it seems unlikely that all such functions are solely orchestrated from within the Inv compartment because as outlined above, Inv proteins localize along the entire ciliary axoneme of certain cells types.Citation97 Also, in mitotic cells, Inv domain proteins localize at the centrosome (NPHP9, NPHP2), spindle poles (NPHP2), and the mid-body (NPHP2).Citation113,Citation114,Citation123
Distal Segment Compartment
Extending from the middle segment of amphid and phasmid channel cilia is a ~3 μm long distal segment, comprised of 9 outer singlet MTs (A-tubules) due to B-tubule termination at the middle segment tip. In the branched AWB cilium, the distal segment is longer (~5 μm) and contains relatively few singlet A-tubules, and terminates with a membrane expansion (fans).Citation50 Microtubule singlet-containing distal segments are also found at the flagellar tips of mating green algae, and in various vertebrate olfactory, pancreatic, and renal cell cilia.Citation51-Citation54 Thus, distal segments may be a common feature of many eukaryotic cilia.
Unlike middle segment assembly, which employs two coordinated and functionally redundant kinesin-2 motors (kinesin-II, OSM-3), only OSM-3 kinesin enters the distal segment to drive anterograde IFT ().Citation99 In osm-3 mutants, cilia terminate at the middle segment tip and these worms are osmo- and chemosensory defective, highlighting the functional importance of the distal segment compartment.Citation74 Additional nematode genes required for distal segment assembly include various IFT genes (dyf-1/IFT70, dyf-6/IFT46, dyf-13/TTC26, ift-74, ift-81), a MAP kinase (dyf-5), α and β tubulin isoforms (tba-5, tbb-4) and the AP-1mu1 clathrin adaptor subunit (unc-101).Citation63,Citation124-Citation126 Many of these genes regulate OSM-3 functions: dyf-1, dyf-6, ift-74, and ift-81 activate OSM-3 motor activities, and dyf-5 regulates OSM-3 speeds; also, IFT-74 biochemically associates with OSM-3.Citation63,Citation124,Citation125 In contrast, TBB-4 and distal segment-enriched TBA-5 proteins do not regulate OSM-3 motor speeds but instead stabilize distal segment singlet microtubules.Citation125
Despite a dedicated assembly pathway, the sensory and signaling proteins specifically functioning in the nematode distal segment remain mostly unknown. Indeed, unlike the other ciliary subcompartments, to our knowledge very few, if any, proteins have been found to be enriched in the nematode ciliary distal segments. However, in other organisms, distal cilium/tip enrichment has been observed for a number of proteins. In rats, odour-detecting CNG channels preferably cluster within the microtubule singlet-containing distal segments of olfactory neuronal cilia.Citation58 Also, the Xenopus laevis calponin homology and microtubule-associated protein (CLAMP) of unknown function is enriched in the distal cilium of multi-ciliated epidermal cells, although it remains unclear if this axonemal region is comprised of doublet or singlet microtubules.Citation127 Numerous proteins associate with the ciliary tip region, a site of microtubule assembly/disassembly, IFT turnaround and signaling.Citation128 These include the mammalian microtubule stabilizing and length control protein EB3,Citation129 Chlamydomonas EB1,Citation130 mammalian sonic hedgehog signaling mediators GLI2, GLI3, and SUFU,Citation56,Citation131 and a mechanosensory Drosophila TRPN channel, NOMPC.Citation132 Very few studies have addressed how proteins are targeted to and restricted at distal ciliary regions, although in Xenopus, the planar cell polarity effector protein Fuz regulates proximo-distal localization patterning of MAP7 (microtubule-associated protein 7) and CLAMP, which are enriched in proximal and distal ciliary regions, respectively.Citation133
One important aspect of the distal ciliary axoneme, and in particular the ciliary tip, is that it is an incorporation site for structural subunits of the axoneme (e.g., tubulin, radial spokes) and is where IFT motor-particle assemblies are remodelled and cargo-unloaded as they switch from anterograde to retrograde transport phases.Citation134-Citation136 Although little is known about how these events are regulated, multiple distal segment/tip localized proteins are likely involved. For example, in Chlamydomonas, tip turnaround of IFT employs an IFT172 mediated interaction with tip-localized EB1.Citation137 In C. elegans, IFT particle turnaround at the ciliary base and tip is dependent on the BBSome at these locations, via a mechanism involving DYF-2/IFT144-mediated interactions.Citation64
Finally, the ciliary tip is a site of membrane shedding. In Chlamydomonas, biologically active ectosomes are released from the flagellar tip,Citation82 and in photoreceptors, spent membranous disks are shed from the outer segment tip for engulfment and degradation by neighboring retinal pigment epithelial cells.Citation138 Such findings suggest that the tip may serve as a local region of ciliary membrane remodeling and turnover, as well as a site for release of biologically active vesicles involved in extracellular processes, which could possibly include cell-cell communication.
Concluding Remarks
The specific localization of multiple ciliopathy proteins and signaling molecules to distinct regions of the cilium has ignited efforts to investigate the subcompartmentalised nature of the organelle. Using multiple model systems including C. elegans, our understanding of subcompartment composition and related transport is advancing. For example, the ciliopathy protein-enriched transition zone appears to regulate the cilium’s signaling capacity by serving as a ciliary gate to regulate transport into and out of the organelle. However, many questions remain. First, what is the functional basis of asymmetric ciliopathy and signaling protein distributions along the ciliary axoneme (e.g., Inv domain vs. distal cilium), and why do some subcompartments display cilium subtype as well as developmental stage heterogeneity? Presumably, such distributions allow the formation of signaling complexes at distinct regions of the ciliary membrane in a cilium subtype and age-dependent manner, but the reasons for this are unclear. Second, what are the mechanisms that separate one compartment from another: how are lateral membrane diffusion barriers defined and what are the anchoring and transport mechanisms that restrict proteins to a particular compartment? Third, what are the functional relationships between ciliopathy proteins and modules that localize in distinct compartments? We know that these relationships exist from biochemical and genetics experiments, but the signaling output from such interactions and their relationship to the spectrum of ciliopathy phenotypes remains poorly understood. Fourthly, as a final thought, it is clear that the subcompartmentalised nature of the cilium provides a new paradigm for understanding transport and signaling mechanisms operating at highly regionalised sites of an organelle; thus, the resultant findings should provide broader insight into the more general question of cellular compartmentalisation and the organization of signaling activity at distinct patches of plasma membrane.
Disclosure of Potential Conflicts of Interest
No potential conflicts of interest were disclosed.
Acknowledgments
Science Foundation Ireland Principal Investigator award (11/PI/1037) and the European Community's Seventh Framework Programme FP7/2009 under grant agreement no: 241955 (SYSCILIA) to O.E.B.
References
- van Dam TJ, Wheway G, Slaats GG, Huynen MA, Giles RH, SYSCILIA Study Group. The SYSCILIA gold standard (SCGSv1) of known ciliary components and its applications within a systems biology consortium. Cilia 2013; 2:7; http://dx.doi.org/10.1186/2046-2530-2-7; PMID: 23725226
- Waters AM, Beales PL. Ciliopathies: an expanding disease spectrum. Pediatr Nephrol 2011; 26:1039 - 56; http://dx.doi.org/10.1007/s00467-010-1731-7; PMID: 21210154
- Goetz SC, Anderson KV. The primary cilium: a signalling centre during vertebrate development. Nat Rev Genet 2010; 11:331 - 44; http://dx.doi.org/10.1038/nrg2774; PMID: 20395968
- Goggolidou P. Wnt and planar cell polarity signaling in cystic renal disease. Organogenesis 2013; 10:10; PMID: 24162855
- Christensen ST, Clement CA, Satir P, Pedersen LB. Primary cilia and coordination of receptor tyrosine kinase (RTK) signalling. J Pathol 2012; 226:172 - 84; http://dx.doi.org/10.1002/path.3004; PMID: 21956154
- Seeger-Nukpezah T, Golemis EA. The extracellular matrix and ciliary signaling. Curr Opin Cell Biol 2012; 24:652 - 61; http://dx.doi.org/10.1016/j.ceb.2012.06.002; PMID: 22819513
- Ezratty EJ, Stokes N, Chai S, Shah AS, Williams SE, Fuchs E. A role for the primary cilium in Notch signaling and epidermal differentiation during skin development. Cell 2011; 145:1129 - 41; http://dx.doi.org/10.1016/j.cell.2011.05.030; PMID: 21703454
- Bielas SL, Silhavy JL, Brancati F, Kisseleva MV, Al-Gazali L, Sztriha L, Bayoumi RA, Zaki MS, Abdel-Aleem A, Rosti RO, et al. Mutations in INPP5E, encoding inositol polyphosphate-5-phosphatase E, link phosphatidyl inositol signaling to the ciliopathies. Nat Genet 2009; 41:1032 - 6; http://dx.doi.org/10.1038/ng.423; PMID: 19668216
- Schneider L, Clement CA, Teilmann SC, Pazour GJ, Hoffmann EK, Satir P, Christensen ST. PDGFRalphaalpha signaling is regulated through the primary cilium in fibroblasts. Curr Biol 2005; 15:1861 - 6; http://dx.doi.org/10.1016/j.cub.2005.09.012; PMID: 16243034
- Reiter JF, Blacque OE, Leroux MR. The base of the cilium: roles for transition fibres and the transition zone in ciliary formation, maintenance and compartmentalization. EMBO Rep 2012; 13:608 - 18; http://dx.doi.org/10.1038/embor.2012.73; PMID: 22653444
- Shiba D, Yamaoka Y, Hagiwara H, Takamatsu T, Hamada H, Yokoyama T. Localization of Inv in a distinctive intraciliary compartment requires the C-terminal ninein-homolog-containing region. J Cell Sci 2009; 122:44 - 54; http://dx.doi.org/10.1242/jcs.037408; PMID: 19050042
- Chih B, Liu P, Chinn Y, Chalouni C, Komuves LG, Hass PE, Sandoval W, Peterson AS. A ciliopathy complex at the transition zone protects the cilia as a privileged membrane domain. Nat Cell Biol 2012; 14:61 - 72; http://dx.doi.org/10.1038/ncb2410; PMID: 22179047
- Craige B, Tsao CC, Diener DR, Hou Y, Lechtreck KF, Rosenbaum JL, Witman GB. CEP290 tethers flagellar transition zone microtubules to the membrane and regulates flagellar protein content. J Cell Biol 2010; 190:927 - 40; http://dx.doi.org/10.1083/jcb.201006105; PMID: 20819941
- Garcia-Gonzalo FR, Corbit KC, Sirerol-Piquer MS, Ramaswami G, Otto EA, Noriega TR, Seol AD, Robinson JF, Bennett CL, Josifova DJ, et al. A transition zone complex regulates mammalian ciliogenesis and ciliary membrane composition. Nat Genet 2011; 43:776 - 84; http://dx.doi.org/10.1038/ng.891; PMID: 21725307
- Williams CL, Li C, Kida K, Inglis PN, Mohan S, Semenec L, Bialas NJ, Stupay RM, Chen N, Blacque OE, et al. MKS and NPHP modules cooperate to establish basal body/transition zone membrane associations and ciliary gate function during ciliogenesis. J Cell Biol 2011; 192:1023 - 41; http://dx.doi.org/10.1083/jcb.201012116; PMID: 21422230
- Hu Q, Milenkovic L, Jin H, Scott MP, Nachury MV, Spiliotis ET, Nelson WJ. A septin diffusion barrier at the base of the primary cilium maintains ciliary membrane protein distribution. Science 2010; 329:436 - 9; http://dx.doi.org/10.1126/science.1191054; PMID: 20558667
- Kee HL, Dishinger JF, Blasius TL, Liu CJ, Margolis B, Verhey KJ. A size-exclusion permeability barrier and nucleoporins characterize a ciliary pore complex that regulates transport into cilia. Nat Cell Biol 2012; 14:431 - 7; http://dx.doi.org/10.1038/ncb2450; PMID: 22388888
- Hsiao Y-C, Tuz K, Ferland RJ. Trafficking in and to the primary cilium. Cilia 2012; 1:4; http://dx.doi.org/10.1186/2046-2530-1-4; PMID: 23351793
- Blacque OE, Cevik S, Kaplan OI. Intraflagellar transport: from molecular characterisation to mechanism. Front Biosci 2008; 13:2633 - 52; http://dx.doi.org/10.2741/2871; PMID: 17981739
- Ishikawa H, Marshall WF. Ciliogenesis: building the cell’s antenna. Nat Rev Mol Cell Biol 2011; 12:222 - 34; http://dx.doi.org/10.1038/nrm3085; PMID: 21427764
- Bae YK, Qin H, Knobel KM, Hu J, Rosenbaum JL, Barr MM. General and cell-type specific mechanisms target TRPP2/PKD-2 to cilia. Development 2006; 133:3859 - 70; http://dx.doi.org/10.1242/dev.02555; PMID: 16943275
- Deretic D, Huber LA, Ransom N, Mancini M, Simons K, Papermaster DS. rab8 in retinal photoreceptors may participate in rhodopsin transport and in rod outer segment disk morphogenesis. J Cell Sci 1995; 108:215 - 24; PMID: 7738098
- Dwyer ND, Adler CE, Crump JG, L’Etoile ND, Bargmann CI. Polarized dendritic transport and the AP-1 mu1 clathrin adaptor UNC-101 localize odorant receptors to olfactory cilia. Neuron 2001; 31:277 - 87; http://dx.doi.org/10.1016/S0896-6273(01)00361-0; PMID: 11502258
- Kaplan OI, Doroquez DB, Cevik S, Bowie RV, Clarke L, Sanders AA, Kida K, Rappoport JZ, Sengupta P, Blacque OE. Endocytosis genes facilitate protein and membrane transport in C. elegans sensory cilia. Curr Biol 2012; 22:451 - 60; http://dx.doi.org/10.1016/j.cub.2012.01.060; PMID: 22342749
- Kaplan OI, Molla-Herman A, Cevik S, Ghossoub R, Kida K, Kimura Y, Jenkins P, Martens JR, Setou M, Benmerah A, et al. The AP-1 clathrin adaptor facilitates cilium formation and functions with RAB-8 in C. elegans ciliary membrane transport. J Cell Sci 2010; 123:3966 - 77; http://dx.doi.org/10.1242/jcs.073908; PMID: 20980383
- Mazelova J, Astuto-Gribble L, Inoue H, Tam BM, Schonteich E, Prekeris R, Moritz OL, Randazzo PA, Deretic D. Ciliary targeting motif VxPx directs assembly of a trafficking module through Arf4. EMBO J 2009; 28:183 - 92; http://dx.doi.org/10.1038/emboj.2008.267; PMID: 19153612
- Molla-Herman A, Ghossoub R, Blisnick T, Meunier A, Serres C, Silbermann F, Emmerson C, Romeo K, Bourdoncle P, Schmitt A, et al. The ciliary pocket: an endocytic membrane domain at the base of primary and motile cilia. J Cell Sci 2010; 123:1785 - 95; http://dx.doi.org/10.1242/jcs.059519; PMID: 20427320
- Moritz OL, Tam BM, Hurd LL, Peränen J, Deretic D, Papermaster DS. Mutant rab8 Impairs docking and fusion of rhodopsin-bearing post-Golgi membranes and causes cell death of transgenic Xenopus rods. Mol Biol Cell 2001; 12:2341 - 51; http://dx.doi.org/10.1091/mbc.12.8.2341; PMID: 11514620
- Nachury MV, Loktev AV, Zhang Q, Westlake CJ, Peränen J, Merdes A, Slusarski DC, Scheller RH, Bazan JF, Sheffield VC, et al. A core complex of BBS proteins cooperates with the GTPase Rab8 to promote ciliary membrane biogenesis. Cell 2007; 129:1201 - 13; http://dx.doi.org/10.1016/j.cell.2007.03.053; PMID: 17574030
- Nachury MV, Seeley ES, Jin H. Trafficking to the ciliary membrane: how to get across the periciliary diffusion barrier?. Annu Rev Cell Dev Biol 2010; 26:59 - 87; http://dx.doi.org/10.1146/annurev.cellbio.042308.113337; PMID: 19575670
- Westlake CJ, Baye LM, Nachury MV, Wright KJ, Ervin KE, Phu L, Chalouni C, Beck JS, Kirkpatrick DS, Slusarski DC, et al. Primary cilia membrane assembly is initiated by Rab11 and transport protein particle II (TRAPPII) complex-dependent trafficking of Rabin8 to the centrosome. Proc Natl Acad Sci U S A 2011; 108:2759 - 64; http://dx.doi.org/10.1073/pnas.1018823108; PMID: 21273506
- Zuo X, Guo W, Lipschutz JH. The exocyst protein Sec10 is necessary for primary ciliogenesis and cystogenesis in vitro. Mol Biol Cell 2009; 20:2522 - 9; http://dx.doi.org/10.1091/mbc.E08-07-0772; PMID: 19297529
- Clement CA, Ajbro KD, Koefoed K, Vestergaard ML, Veland IR, Henriques de Jesus MP, Pedersen LB, Benmerah A, Andersen CY, Larsen LA, et al. TGF-β signaling is associated with endocytosis at the pocket region of the primary cilium. Cell Rep 2013; 3:1806 - 14; http://dx.doi.org/10.1016/j.celrep.2013.05.020; PMID: 23746451
- Dishinger JF, Kee HL, Jenkins PM, Fan S, Hurd TW, Hammond JW, Truong YN, Margolis B, Martens JR, Verhey KJ. Ciliary entry of the kinesin-2 motor KIF17 is regulated by importin-beta2 and RanGTP. Nat Cell Biol 2010; 12:703 - 10; http://dx.doi.org/10.1038/ncb2073; PMID: 20526328
- Fan S, Fogg V, Wang Q, Chen XW, Liu CJ, Margolis B. A novel Crumbs3 isoform regulates cell division and ciliogenesis via importin beta interactions. J Cell Biol 2007; 178:387 - 98; http://dx.doi.org/10.1083/jcb.200609096; PMID: 17646395
- Fan S, Whiteman EL, Hurd TW, McIntyre JC, Dishinger JF, Liu CJ, Martens JR, Verhey KJ, Sajjan U, Margolis B. Induction of Ran GTP drives ciliogenesis. Mol Biol Cell 2011; 22:4539 - 48; http://dx.doi.org/10.1091/mbc.E11-03-0267; PMID: 21998203
- Hurd TW, Fan S, Margolis BL. Localization of retinitis pigmentosa 2 to cilia is regulated by Importin beta2. J Cell Sci 2011; 124:718 - 26; http://dx.doi.org/10.1242/jcs.070839; PMID: 21285245
- Breslow DK, Koslover EF, Seydel F, Spakowitz AJ, Nachury MV. An in vitro assay for entry into cilia reveals unique properties of the soluble diffusion barrier. J Cell Biol 2013; 203:129 - 47; http://dx.doi.org/10.1083/jcb.201212024; PMID: 24100294
- Belzile O, Hernandez-Lara CI, Wang Q, Snell WJ. Regulated membrane protein entry into flagella is facilitated by cytoplasmic microtubules and does not require IFT. Curr Biol 2013; 23:1460 - 5; http://dx.doi.org/10.1016/j.cub.2013.06.025; PMID: 23891117
- Kim S, Dynlacht BD. Assembling a primary cilium. Curr Opin Cell Biol 2013; 25:506 - 11; http://dx.doi.org/10.1016/j.ceb.2013.04.011; PMID: 23747070
- Rosenbaum JL, Witman GB. Intraflagellar transport. Nat Rev Mol Cell Biol 2002; 3:813 - 25; http://dx.doi.org/10.1038/nrm952; PMID: 12415299
- Melkonian M. The functional analysis of the flagellar apparatus in green algae. Symp Soc Exp Biol 1982; 35:589 - 606; PMID: 6764051
- Weiss RL, Goodenough DA, Goodenough UW. Membrane particle arrays associated with the basal body and with contractile vacuole secretion in Chlamydomonas. J Cell Biol 1977; 72:133 - 43; http://dx.doi.org/10.1083/jcb.72.1.133; PMID: 830652
- Deane JA, Cole DG, Seeley ES, Diener DR, Rosenbaum JL. Localization of intraflagellar transport protein IFT52 identifies basal body transitional fibers as the docking site for IFT particles. Curr Biol 2001; 11:1586 - 90; http://dx.doi.org/10.1016/S0960-9822(01)00484-5; PMID: 11676918
- Bornens M. Centrosome composition and microtubule anchoring mechanisms. Curr Opin Cell Biol 2002; 14:25 - 34; http://dx.doi.org/10.1016/S0955-0674(01)00290-3; PMID: 11792541
- Kobayashi T, Dynlacht BD. Regulating the transition from centriole to basal body. J Cell Biol 2011; 193:435 - 44; http://dx.doi.org/10.1083/jcb.201101005; PMID: 21536747
- Czarnecki PG, Shah JV. The ciliary transition zone: from morphology and molecules to medicine. Trends Cell Biol 2012; 22:201 - 10; http://dx.doi.org/10.1016/j.tcb.2012.02.001; PMID: 22401885
- Cevik S, Sanders AA, Van Wijk E, Boldt K, Clarke L, van Reeuwijk J, Hori Y, Horn N, Hetterschijt L, Wdowicz A, et al. Active transport and diffusion barriers restrict Joubert Syndrome-associated ARL13B/ARL-13 to an Inv-like ciliary membrane subdomain. PLoS Genet 2013; 9:e1003977; http://dx.doi.org/10.1371/journal.pgen.1003977; PMID: 24339792
- Gilula NB, Satir P. The ciliary necklace. A ciliary membrane specialization. J Cell Biol 1972; 53:494 - 509; http://dx.doi.org/10.1083/jcb.53.2.494; PMID: 4554367
- Inglis PN, Ou G, Leroux MR, Scholey JM. The sensory cilia of C. elegans (March 8, 2007). WormBook, ed The C elegans Research Community, WormBook, doi/101895/wormbook11231 2007.
- Reese TS. Olfactory Cilia in the Frog. J Cell Biol 1965; 25:209 - 30; http://dx.doi.org/10.1083/jcb.25.2.209; PMID: 19866665
- Hidaka K, Ashizawa N, Endoh H, Watanabe M, Fukumoto S. Fine structure of the cilia in the pancreatic duct of WBN/Kob rat. Int J Pancreatol 1995; 18:207 - 13; PMID: 8708391
- Mesland DA, Hoffman JL, Caligor E, Goodenough UW. Flagellar tip activation stimulated by membrane adhesions in Chlamydomonas gametes. J Cell Biol 1980; 84:599 - 617; http://dx.doi.org/10.1083/jcb.84.3.599; PMID: 7358792
- Webber WA, Lee J. Fine structure of mammalian renal cilia. Anat Rec 1975; 182:339 - 43; http://dx.doi.org/10.1002/ar.1091820307; PMID: 1155803
- Shiba D, Manning DK, Koga H, Beier DR, Yokoyama T. Inv acts as a molecular anchor for Nphp3 and Nek8 in the proximal segment of primary cilia. Cytoskeleton (Hoboken) 2010; 67:112 - 9; PMID: 20169535
- Haycraft CJ, Banizs B, Aydin-Son Y, Zhang Q, Michaud EJ, Yoder BK. Gli2 and Gli3 localize to cilia and require the intraflagellar transport protein polaris for processing and function. PLoS Genet 2005; 1:e53; http://dx.doi.org/10.1371/journal.pgen.0010053; PMID: 16254602
- Flannery RJ, French DA, Kleene SJ. Clustering of cyclic-nucleotide-gated channels in olfactory cilia. Biophys J 2006; 91:179 - 88; http://dx.doi.org/10.1529/biophysj.105.079046; PMID: 16603488
- Matsuzaki O, Bakin RE, Cai X, Menco BP, Ronnett GV. Localization of the olfactory cyclic nucleotide-gated channel subunit 1 in normal, embryonic and regenerating olfactory epithelium. Neuroscience 1999; 94:131 - 40; http://dx.doi.org/10.1016/S0306-4522(99)00228-6; PMID: 10613503
- Broekhuis JR, Leong WY, Jansen G. Regulation of cilium length and intraflagellar transport. Int Rev Cell Mol Biol 2013; 303:101 - 38; http://dx.doi.org/10.1016/B978-0-12-407697-6.00003-9; PMID: 23445809
- Blacque OE, Reardon MJ, Li C, McCarthy J, Mahjoub MR, Ansley SJ, Badano JL, Mah AK, Beales PL, Davidson WS, et al. Loss of C. elegans BBS-7 and BBS-8 protein function results in cilia defects and compromised intraflagellar transport. Genes Dev 2004; 18:1630 - 42; http://dx.doi.org/10.1101/gad.1194004; PMID: 15231740
- Cevik S, Hori Y, Kaplan OI, Kida K, Toivenon T, Foley-Fisher C, Cottell D, Katada T, Kontani K, Blacque OE. Joubert syndrome Arl13b functions at ciliary membranes and stabilizes protein transport in Caenorhabditis elegans. J Cell Biol 2010; 188:953 - 69; http://dx.doi.org/10.1083/jcb.200908133; PMID: 20231383
- Li Y, Wei Q, Zhang Y, Ling K, Hu J. The small GTPases ARL-13 and ARL-3 coordinate intraflagellar transport and ciliogenesis. J Cell Biol 2010; 189:1039 - 51; http://dx.doi.org/10.1083/jcb.200912001; PMID: 20530210
- Ou G, Blacque OE, Snow JJ, Leroux MR, Scholey JM. Functional coordination of intraflagellar transport motors. Nature 2005; 436:583 - 7; http://dx.doi.org/10.1038/nature03818; PMID: 16049494
- Wei Q, Zhang Y, Li Y, Zhang Q, Ling K, Hu J. The BBSome controls IFT assembly and turnaround in cilia. Nat Cell Biol 2012; 14:950 - 7; http://dx.doi.org/10.1038/ncb2560; PMID: 22922713
- Broekhuis JR, Rademakers S, Burghoorn J, Jansen G. SQL-1, homologue of the Golgi protein GMAP210, modulates intraflagellar transport in C. elegans. J Cell Sci 2013; 126:1785 - 95; http://dx.doi.org/10.1242/jcs.116640; PMID: 23444385
- Olivier-Mason A, Wojtyniak M, Bowie RV, Nechipurenko IV, Blacque OE, Sengupta P. Transmembrane protein OSTA-1 shapes sensory cilia morphology via regulation of intracellular membrane trafficking in C. elegans. Development 2013; 140:1560 - 72; http://dx.doi.org/10.1242/dev.086249; PMID: 23482491
- Hu J, Wittekind SG, Barr MM. STAM and Hrs down-regulate ciliary TRP receptors. Mol Biol Cell 2007; 18:3277 - 89; http://dx.doi.org/10.1091/mbc.E07-03-0239; PMID: 17581863
- Blacque OE, Li C, Inglis PN, Esmail MA, Ou G, Mah AK, Baillie DL, Scholey JM, Leroux MR. The WD repeat-containing protein IFTA-1 is required for retrograde intraflagellar transport. Mol Biol Cell 2006; 17:5053 - 62; http://dx.doi.org/10.1091/mbc.E06-06-0571; PMID: 17021254
- Blacque OE, Perens EA, Boroevich KA, Inglis PN, Li C, Warner A, Khattra J, Holt RA, Ou G, Mah AK, et al. Functional genomics of the cilium, a sensory organelle. Curr Biol 2005; 15:935 - 41; http://dx.doi.org/10.1016/j.cub.2005.04.059; PMID: 15916950
- Efimenko E, Blacque OE, Ou G, Haycraft CJ, Yoder BK, Scholey JM, Leroux MR, Swoboda P. Caenorhabditis elegans DYF-2, an orthologue of human WDR19, is a component of the intraflagellar transport machinery in sensory cilia. Mol Biol Cell 2006; 17:4801 - 11; http://dx.doi.org/10.1091/mbc.E06-04-0260; PMID: 16957054
- Bacaj T, Lu Y, Shaham S. The conserved proteins CHE-12 and DYF-11 are required for sensory cilium function in Caenorhabditis elegans. Genetics 2008; 178:989 - 1002; http://dx.doi.org/10.1534/genetics.107.082453; PMID: 18245347
- Li C, Inglis PN, Leitch CC, Efimenko E, Zaghloul NA, Mok CA, Davis EE, Bialas NJ, Healey MP, Héon E, et al. An essential role for DYF-11/MIP-T3 in assembling functional intraflagellar transport complexes. PLoS Genet 2008; 4:e1000044; http://dx.doi.org/10.1371/journal.pgen.1000044; PMID: 18369462
- Doroquez DB, Berciu C, Anderson JR, Sengupta P, Nicastro D. A high-resolution morphological and ultrastructural map of anterior sensory cilia and glia in Caenorhabditis elegans. Elife 2014; 3:e01948; http://dx.doi.org/10.7554/eLife.01948; PMID: 24668170
- Perkins LA, Hedgecock EM, Thomson JN, Culotti JG. Mutant sensory cilia in the nematode Caenorhabditis elegans. Dev Biol 1986; 117:456 - 87; http://dx.doi.org/10.1016/0012-1606(86)90314-3; PMID: 2428682
- Manandhar G, Simerly C, Salisbury JL, Schatten G. Centriole and centrin degeneration during mouse spermiogenesis. Cell Motil Cytoskeleton 1999; 43:137 - 44; http://dx.doi.org/10.1002/(SICI)1097-0169(1999)43:2<137::AID-CM5>3.0.CO;2-7; PMID: 10379838
- Manandhar G, Simerly C, Schatten G. Highly degenerated distal centrioles in rhesus and human spermatozoa. Hum Reprod 2000; 15:256 - 63; http://dx.doi.org/10.1093/humrep/15.2.256; PMID: 10655294
- Dammermann A, Pemble H, Mitchell BJ, McLeod I, Yates JR 3rd, Kintner C, Desai AB, Oegema K. The hydrolethalus syndrome protein HYLS-1 links core centriole structure to cilia formation. Genes Dev 2009; 23:2046 - 59; http://dx.doi.org/10.1101/gad.1810409; PMID: 19656802
- Mohan S, Timbers TA, Kennedy J, Blacque OE, Leroux MR. Striated rootlet and nonfilamentous forms of rootletin maintain ciliary function. Curr Biol 2013; 23:2016 - 22; http://dx.doi.org/10.1016/j.cub.2013.08.033; PMID: 24094853
- Wei Q, Xu Q, Zhang Y, Li Y, Zhang Q, Hu Z, Harris PC, Torres VE, Ling K, Hu J. Transition fibre protein FBF1 is required for the ciliary entry of assembled intraflagellar transport complexes. Nat Commun 2013; 4:2750; http://dx.doi.org/10.1038/ncomms3750; PMID: 24231678
- Yang J, Gao J, Adamian M, Wen XH, Pawlyk B, Zhang L, Sanderson MJ, Zuo J, Makino CL, Li T. The ciliary rootlet maintains long-term stability of sensory cilia. Mol Cell Biol 2005; 25:4129 - 37; http://dx.doi.org/10.1128/MCB.25.10.4129-4137.2005; PMID: 15870283
- Wang J, Silva M, Haas LA, Morsci NS, Nguyen KC, Hall DH, Barr MM. C. elegans Ciliated Sensory Neurons Release Extracellular Vesicles that Function in Animal Communication. Curr Biol 2014; 24:519 - 25; http://dx.doi.org/10.1016/j.cub.2014.01.002; PMID: 24530063
- Wood CR, Huang K, Diener DR, Rosenbaum JL. The cilium secretes bioactive ectosomes. Curr Biol 2013; 23:906 - 11; http://dx.doi.org/10.1016/j.cub.2013.04.019; PMID: 23623554
- Allen CL, Goulding D, Field MC. Clathrin-mediated endocytosis is essential in Trypanosoma brucei. EMBO J 2003; 22:4991 - 5002; http://dx.doi.org/10.1093/emboj/cdg481; PMID: 14517238
- Evans RJ, Schwarz N, Nagel-Wolfrum K, Wolfrum U, Hardcastle AJ, Cheetham ME. The retinitis pigmentosa protein RP2 links pericentriolar vesicle transport between the Golgi and the primary cilium. Hum Mol Genet 2010; 19:1358 - 67; http://dx.doi.org/10.1093/hmg/ddq012; PMID: 20106869
- Stephan A, Vaughan S, Shaw MK, Gull K, McKean PG. An essential quality control mechanism at the eukaryotic basal body prior to intraflagellar transport. Traffic 2007; 8:1323 - 30; http://dx.doi.org/10.1111/j.1600-0854.2007.00611.x; PMID: 17645436
- Stefan CJ, Manford AG, Emr SD. ER-PM connections: sites of information transfer and inter-organelle communication. Curr Opin Cell Biol 2013; 25:434 - 42; http://dx.doi.org/10.1016/j.ceb.2013.02.020; PMID: 23522446
- Lebiedzinska M, Szabadkai G, Jones AW, Duszynski J, Wieckowski MR. Interactions between the endoplasmic reticulum, mitochondria, plasma membrane and other subcellular organelles. Int J Biochem Cell Biol 2009; 41:1805 - 16; http://dx.doi.org/10.1016/j.biocel.2009.02.017; PMID: 19703651
- Ramírez OA, Couve A. The endoplasmic reticulum and protein trafficking in dendrites and axons. Trends Cell Biol 2011; 21:219 - 27; http://dx.doi.org/10.1016/j.tcb.2010.12.003; PMID: 21215635
- Francis SS, Sfakianos J, Lo B, Mellman I. A hierarchy of signals regulates entry of membrane proteins into the ciliary membrane domain in epithelial cells. J Cell Biol 2011; 193:219 - 33; http://dx.doi.org/10.1083/jcb.201009001; PMID: 21444686
- Huang L, Szymanska K, Jensen VL, Janecke AR, Innes AM, Davis EE, Frosk P, Li C, Willer JR, Chodirker BN, et al. TMEM237 is mutated in individuals with a Joubert syndrome related disorder and expands the role of the TMEM family at the ciliary transition zone. Am J Hum Genet 2011; 89:713 - 30; http://dx.doi.org/10.1016/j.ajhg.2011.11.005; PMID: 22152675
- Bialas NJ, Inglis PN, Li C, Robinson JF, Parker JD, Healey MP, Davis EE, Inglis CD, Toivonen T, Cottell DC, et al. Functional interactions between the ciliopathy-associated Meckel syndrome 1 (MKS1) protein and two novel MKS1-related (MKSR) proteins. J Cell Sci 2009; 122:611 - 24; http://dx.doi.org/10.1242/jcs.028621; PMID: 19208769
- Williams CL, Winkelbauer ME, Schafer JC, Michaud EJ, Yoder BK. Functional redundancy of the B9 proteins and nephrocystins in Caenorhabditis elegans ciliogenesis. Mol Biol Cell 2008; 19:2154 - 68; http://dx.doi.org/10.1091/mbc.E07-10-1070; PMID: 18337471
- Jauregui AR, Nguyen KC, Hall DH, Barr MM. The Caenorhabditis elegans nephrocystins act as global modifiers of cilium structure. J Cell Biol 2008; 180:973 - 88; http://dx.doi.org/10.1083/jcb.200707090; PMID: 18316409
- Heiman MG, Shaham S. DEX-1 and DYF-7 establish sensory dendrite length by anchoring dendritic tips during cell migration. Cell 2009; 137:344 - 55; http://dx.doi.org/10.1016/j.cell.2009.01.057; PMID: 19344940
- Williams CL, Masyukova SV, Yoder BK. Normal ciliogenesis requires synergy between the cystic kidney disease genes MKS-3 and NPHP-4. J Am Soc Nephrol 2010; 21:782 - 93; http://dx.doi.org/10.1681/ASN.2009060597; PMID: 20150540
- Garcia-Gonzalo FR, Reiter JF. Scoring a backstage pass: mechanisms of ciliogenesis and ciliary access. J Cell Biol 2012; 197:697 - 709; http://dx.doi.org/10.1083/jcb.201111146; PMID: 22689651
- Sang L, Miller JJ, Corbit KC, Giles RH, Brauer MJ, Otto EA, Baye LM, Wen X, Scales SJ, Kwong M, et al. Mapping the NPHP-JBTS-MKS protein network reveals ciliopathy disease genes and pathways. Cell 2011; 145:513 - 28; http://dx.doi.org/10.1016/j.cell.2011.04.019; PMID: 21565611
- Mukhopadhyay S, Lu Y, Qin H, Lanjuin A, Shaham S, Sengupta P. Distinct IFT mechanisms contribute to the generation of ciliary structural diversity in C. elegans. EMBO J 2007; 26:2966 - 80; http://dx.doi.org/10.1038/sj.emboj.7601717; PMID: 17510633
- Snow JJ, Ou G, Gunnarson AL, Walker MR, Zhou HM, Brust-Mascher I, Scholey JM. Two anterograde intraflagellar transport motors cooperate to build sensory cilia on C. elegans neurons. Nat Cell Biol 2004; 6:1109 - 13; http://dx.doi.org/10.1038/ncb1186; PMID: 15489852
- Hoff S, Halbritter J, Epting D, Frank V, Nguyen TM, van Reeuwijk J, Boehlke C, Schell C, Yasunaga T, Helmstädter M, et al. ANKS6 is a central component of a nephronophthisis module linking NEK8 to INVS and NPHP3. Nat Genet 2013; 45:951 - 6; http://dx.doi.org/10.1038/ng.2681; PMID: 23793029
- Larkins CE, Aviles GD, East MP, Kahn RA, Caspary T. Arl13b regulates ciliogenesis and the dynamic localization of Shh signaling proteins. Mol Biol Cell 2011; 22:4694 - 703; http://dx.doi.org/10.1091/mbc.E10-12-0994; PMID: 21976698
- Warburton-Pitt SR, Jauregui AR, Li C, Wang J, Leroux MR, Barr MM. Ciliogenesis in Caenorhabditis elegans requires genetic interactions between ciliary middle segment localized NPHP-2 (inversin) and transition zone-associated proteins. J Cell Sci 2012; 125:2592 - 603; http://dx.doi.org/10.1242/jcs.095539; PMID: 22393243
- Mukhopadhyay S, Lu Y, Shaham S, Sengupta P. Sensory signaling-dependent remodeling of olfactory cilia architecture in C. elegans. Dev Cell 2008; 14:762 - 74; http://dx.doi.org/10.1016/j.devcel.2008.03.002; PMID: 18477458
- Wojtyniak M, Brear AG, O’Halloran DM, Sengupta P. Cell- and subunit-specific mechanisms of CNG channel ciliary trafficking and localization in C. elegans. J Cell Sci 2013; 126:4381 - 95; http://dx.doi.org/10.1242/jcs.127274; PMID: 23886944
- Caspary T, Larkins CE, Anderson KV. The graded response to Sonic Hedgehog depends on cilia architecture. Dev Cell 2007; 12:767 - 78; http://dx.doi.org/10.1016/j.devcel.2007.03.004; PMID: 17488627
- Duldulao NA, Lee S, Sun Z. Cilia localization is essential for in vivo functions of the Joubert syndrome protein Arl13b/Scorpion. Development 2009; 136:4033 - 42; http://dx.doi.org/10.1242/dev.036350; PMID: 19906870
- Tyler KM, Fridberg A, Toriello KM, Olson CL, Cieslak JA, Hazlett TL, Engman DM. Flagellar membrane localization via association with lipid rafts. J Cell Sci 2009; 122:859 - 66; http://dx.doi.org/10.1242/jcs.037721; PMID: 19240119
- Nakata K, Shiba D, Kobayashi D, Yokoyama T. Targeting of Nphp3 to the primary cilia is controlled by an N-terminal myristoylation site and coiled-coil domains. Cytoskeleton (Hoboken) 2012; 69:221 - 34; http://dx.doi.org/10.1002/cm.21014; PMID: 22328406
- Emmer BT, Souther C, Toriello KM, Olson CL, Epting CL, Engman DM. Identification of a palmitoyl acyltransferase required for protein sorting to the flagellar membrane. J Cell Sci 2009; 122:867 - 74; http://dx.doi.org/10.1242/jcs.041764; PMID: 19240115
- Wright KJ, Baye LM, Olivier-Mason A, Mukhopadhyay S, Sang L, Kwong M, Wang W, Pretorius PR, Sheffield VC, Sengupta P, et al. An ARL3-UNC119-RP2 GTPase cycle targets myristoylated NPHP3 to the primary cilium. Genes Dev 2011; 25:2347 - 60; http://dx.doi.org/10.1101/gad.173443.111; PMID: 22085962
- Chailley B, Bork K, Gounon P, Sandoz D. Immunological detection of actin in isolated cilia from quail oviduct. Biol Cell 1986; 58:43 - 52; http://dx.doi.org/10.1111/j.1768-322X.1986.tb00487.x; PMID: 2952200
- Habbig S, Bartram MP, Sägmüller JG, Griessmann A, Franke M, Müller RU, Schwarz R, Hoehne M, Bergmann C, Tessmer C, et al. The ciliopathy disease protein NPHP9 promotes nuclear delivery and activation of the oncogenic transcriptional regulator TAZ. Hum Mol Genet 2012; 21:5528 - 38; http://dx.doi.org/10.1093/hmg/dds408; PMID: 23026745
- Morgan D, Eley L, Sayer J, Strachan T, Yates LM, Craighead AS, Goodship JA. Expression analyses and interaction with the anaphase promoting complex protein Apc2 suggest a role for inversin in primary cilia and involvement in the cell cycle. Hum Mol Genet 2002; 11:3345 - 50; http://dx.doi.org/10.1093/hmg/11.26.3345; PMID: 12471060
- Zalli D, Bayliss R, Fry AM. The Nek8 protein kinase, mutated in the human cystic kidney disease nephronophthisis, is both activated and degraded during ciliogenesis. Hum Mol Genet 2012; 21:1155 - 71; http://dx.doi.org/10.1093/hmg/ddr544; PMID: 22106379
- Higginbotham H, Eom TY, Mariani LE, Bachleda A, Hirt J, Gukassyan V, Cusack CL, Lai C, Caspary T, Anton ES. Arl13b in primary cilia regulates the migration and placement of interneurons in the developing cerebral cortex. Dev Cell 2012; 23:925 - 38; http://dx.doi.org/10.1016/j.devcel.2012.09.019; PMID: 23153492
- Higginbotham H, Guo J, Yokota Y, Umberger NL, Su CY, Li J, Verma N, Hirt J, Ghukasyan V, Caspary T, et al. Arl13b-regulated cilia activities are essential for polarized radial glial scaffold formation. Nat Neurosci 2013; 16:1000 - 7; http://dx.doi.org/10.1038/nn.3451; PMID: 23817546
- Mahuzier A, Gaudé HM, Grampa V, Anselme I, Silbermann F, Leroux-Berger M, Delacour D, Ezan J, Montcouquiol M, Saunier S, et al. Dishevelled stabilization by the ciliopathy protein Rpgrip1l is essential for planar cell polarity. J Cell Biol 2012; 198:927 - 40; http://dx.doi.org/10.1083/jcb.201111009; PMID: 22927466
- Zhao C, Malicki J. Nephrocystins and MKS proteins interact with IFT particle and facilitate transport of selected ciliary cargos. EMBO J 2011; 30:2532 - 44; http://dx.doi.org/10.1038/emboj.2011.165; PMID: 21602787
- Simons M, Gloy J, Ganner A, Bullerkotte A, Bashkurov M, Krönig C, Schermer B, Benzing T, Cabello OA, Jenny A, et al. Inversin, the gene product mutated in nephronophthisis type II, functions as a molecular switch between Wnt signaling pathways. Nat Genet 2005; 37:537 - 43; http://dx.doi.org/10.1038/ng1552; PMID: 15852005
- Lienkamp S, Ganner A, Boehlke C, Schmidt T, Arnold SJ, Schäfer T, Romaker D, Schuler J, Hoff S, Powelske C, et al. Inversin relays Frizzled-8 signals to promote proximal pronephros development. Proc Natl Acad Sci U S A 2010; 107:20388 - 93; http://dx.doi.org/10.1073/pnas.1013070107; PMID: 21059920
- Veland IR, Montjean R, Eley L, Pedersen LB, Schwab A, Goodship J, Kristiansen K, Pedersen SF, Saunier S, Christensen ST. Inversin/Nephrocystin-2 is required for fibroblast polarity and directional cell migration. PLoS One 2013; 8:e60193; http://dx.doi.org/10.1371/journal.pone.0060193; PMID: 23593172
- Choi HJ, Lin JR, Vannier JB, Slaats GG, Kile AC, Paulsen RD, Manning DK, Beier DR, Giles RH, Boulton SJ, et al. NEK8 links the ATR-regulated replication stress response and S phase CDK activity to renal ciliopathies. Mol Cell 2013; 51:423 - 39; http://dx.doi.org/10.1016/j.molcel.2013.08.006; PMID: 23973373
- Trapp ML, Galtseva A, Manning DK, Beier DR, Rosenblum ND, Quarmby LM. Defects in ciliary localization of Nek8 is associated with cystogenesis. Pediatr Nephrol 2008; 23:377 - 87; http://dx.doi.org/10.1007/s00467-007-0692-y; PMID: 18189147
- Burghoorn J, Dekkers MP, Rademakers S, de Jong T, Willemsen R, Jansen G. Mutation of the MAP kinase DYF-5 affects docking and undocking of kinesin-2 motors and reduces their speed in the cilia of Caenorhabditis elegans. Proc Natl Acad Sci U S A 2007; 104:7157 - 62; http://dx.doi.org/10.1073/pnas.0606974104; PMID: 17420466
- Hao L, Thein M, Brust-Mascher I, Civelekoglu-Scholey G, Lu Y, Acar S, Prevo B, Shaham S, Scholey JM. Intraflagellar transport delivers tubulin isotypes to sensory cilium middle and distal segments. Nat Cell Biol 2011; 13:790 - 8; http://dx.doi.org/10.1038/ncb2268; PMID: 21642982
- Ou G, Koga M, Blacque OE, Murayama T, Ohshima Y, Schafer JC, Li C, Yoder BK, Leroux MR, Scholey JM. Sensory ciliogenesis in Caenorhabditis elegans: assignment of IFT components into distinct modules based on transport and phenotypic profiles. Mol Biol Cell 2007; 18:1554 - 69; http://dx.doi.org/10.1091/mbc.E06-09-0805; PMID: 17314406
- Gray RS, Abitua PB, Wlodarczyk BJ, Szabo-Rogers HL, Blanchard O, Lee I, Weiss GS, Liu KJ, Marcotte EM, Wallingford JB, et al. The planar cell polarity effector Fuz is essential for targeted membrane trafficking, ciliogenesis and mouse embryonic development. Nat Cell Biol 2009; 11:1225 - 32; http://dx.doi.org/10.1038/ncb1966; PMID: 19767740
- Pedersen LB, Rosenbaum JL. Intraflagellar transport (IFT) role in ciliary assembly, resorption and signalling. Curr Top Dev Biol 2008; 85:23 - 61; http://dx.doi.org/10.1016/S0070-2153(08)00802-8; PMID: 19147001
- Schrøder JM, Larsen J, Komarova Y, Akhmanova A, Thorsteinsson RI, Grigoriev I, Manguso R, Christensen ST, Pedersen SF, Geimer S, et al. EB1 and EB3 promote cilia biogenesis by several centrosome-related mechanisms. J Cell Sci 2011; 124:2539 - 51; http://dx.doi.org/10.1242/jcs.085852; PMID: 21768326
- Pedersen LB, Geimer S, Sloboda RD, Rosenbaum JL. The Microtubule plus end-tracking protein EB1 is localized to the flagellar tip and basal bodies in Chlamydomonas reinhardtii. Curr Biol 2003; 13:1969 - 74; http://dx.doi.org/10.1016/j.cub.2003.10.058; PMID: 14614822
- Briscoe J, Thérond PP. The mechanisms of Hedgehog signalling and its roles in development and disease. Nat Rev Mol Cell Biol 2013; 14:416 - 29; http://dx.doi.org/10.1038/nrm3598; PMID: 23719536
- Lee J, Moon S, Cha Y, Chung YD. Drosophila TRPN(=NOMPC) channel localizes to the distal end of mechanosensory cilia. PLoS One 2010; 5:e11012; http://dx.doi.org/10.1371/journal.pone.0011012; PMID: 20543979
- Brooks ER, Wallingford JB. Control of vertebrate intraflagellar transport by the planar cell polarity effector Fuz. J Cell Biol 2012; 198:37 - 45; http://dx.doi.org/10.1083/jcb.201204072; PMID: 22778277
- Pedersen LB, Geimer S, Rosenbaum JL. Dissecting the molecular mechanisms of intraflagellar transport in chlamydomonas. Curr Biol 2006; 16:450 - 9; http://dx.doi.org/10.1016/j.cub.2006.02.020; PMID: 16527740
- Johnson KA, Rosenbaum JL. Polarity of flagellar assembly in Chlamydomonas. J Cell Biol 1992; 119:1605 - 11; http://dx.doi.org/10.1083/jcb.119.6.1605; PMID: 1281816
- Wren KN, Craft JM, Tritschler D, Schauer A, Patel DK, Smith EF, Porter ME, Kner P, Lechtreck KF. A differential cargo-loading model of ciliary length regulation by IFT. Curr Biol 2013; 23:2463 - 71; http://dx.doi.org/10.1016/j.cub.2013.10.044; PMID: 24316207
- Pedersen LB, Miller MS, Geimer S, Leitch JM, Rosenbaum JL, Cole DG. Chlamydomonas IFT172 is encoded by FLA11, interacts with CrEB1, and regulates IFT at the flagellar tip. Curr Biol 2005; 15:262 - 6; http://dx.doi.org/10.1016/j.cub.2005.01.037; PMID: 15694311
- Young RW. The renewal of rod and cone outer segments in the rhesus monkey. J Cell Biol 1971; 49:303 - 18; http://dx.doi.org/10.1083/jcb.49.2.303; PMID: 19866760