Abstract
The primary cilium compartmentalizes a tiny fraction of the cell surface and volume, yet many proteins are highly enriched in this area and so efficient mechanisms are necessary to concentrate them in the ciliary compartment. Here we review mechanisms that are thought to deliver protein cargo to the base of cilia and are likely to interact with ciliary gating mechanisms. Given the immense variety of ciliary cytosolic and transmembrane proteins, it is almost certain that multiple, albeit frequently interconnected, pathways mediate this process. It is also clear that none of these pathways is fully understood at the present time. Mechanisms that are discussed below facilitate ciliary localization of structural and signaling molecules, which include receptors, G-proteins, ion channels, and enzymes. These mechanisms form a basis for every aspect of cilia function in early embryonic patterning, organ morphogenesis, sensory perception and elsewhere.
In an idealized round cell, with a radius of 10 µm, the membrane of a primary cilium (typically 2 µm long) occupies approximately 1/1000 of the total cell surface. Similarly, the cytoplasmic volume of the cilium (the cilioplasm) is a very small fraction of the cell’s cytoplasm. Yet many proteins are highly enriched in this area. This is accomplished by a combination of transport and barrier mechanisms that operate at cilia base. Transport into cilia involves two phases: first ciliary proteins are transported through the cytoplasm to the cilium base, and then, in the second phase, they are translocated inside the ciliary shaft by a mechanism known as intraflagellar transport (IFT) ().Citation1-Citation4 The transition from cytoplasmic to intraflageller transport occurs at cilia base, which is also the area that features selective barrier mechanisms that restrict the free movement of proteins into and out of the cilium.
Figure 1. The ciliary transition zone and related transport pathways. (A) The cilium is a hair like protrusion that extends from the cell surface. It consists of the centriole (basal body) that is located in the cytoplasm, the axoneme, which forms as an extension of centriolar microtubules, and the membrane that encases the axoneme. Several structures associate with the base of the cilium: the ciliary pocket, an invagination found at the junction of the plasma membrane and cilium membrane; the septin ring, a protein scaffold that associates with the cilium periphery; transition fibers, which are appendages that connect the distal centriole to the cell membrane; and the transition zone (TZ), which is a specialized and structurally distinct region found between the centriole and the cilium proper. (B) Key proteins that mediate and regulate transport into the ciliary compartment. Proteins destined for cilia are delivered via several routes. Transmembrane proteins are delivered from the Golgi apparatus in vesicles, which travel through the cytoplasm toward the cilium and fuse with the ciliary or periciliary membrane. Several protein complexes and pathways have been identified to function in this process (boxes). The transmembrane Smoothened (smo) protein is an unusual case because it is first deposited in the plasma membrane, from where it translocates into the cilium upon the activation of the hedgehog signaling cascade. Diffusion is likely to be the driving force that translocates cytosol-soluble proteins toward the cilium base. A subset of lipidated proteins is also solubilized in the cytoplasm by UNC119. Despite their hydrophobicity, these proteins are likely to behave similarly to cytoplasmic proteins. Inside the ciliary shaft, proteins are translocated via a mechanism known as intraflagellar transport (IFT).
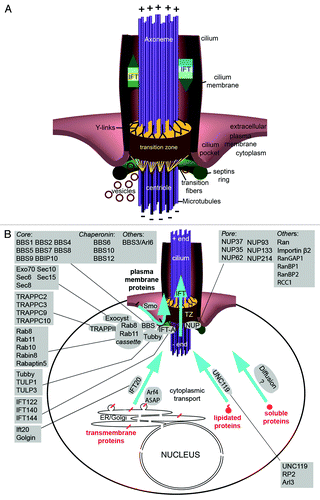
For transmembrane proteins, transport through the cytoplasm into the cilium involves a mechanism that concentrates ciliary proteins in a specific class of exocytotic vesicles, another mechanism that translocates these vesicles toward the cilium and, finally, machinery that mediates vesicle fusion at the base of the cilium (see examples in refs. Citation5–Citation8). This final step must be spatially coordinated with a diffusion barrier(s) at the cilium base. A variation of this transport pathway has been also described and involves rapid lateral translocation of a protein, which is initially deposited in the plasma membrane, into the ciliary membrane.Citation9
In the case of cytosolic proteins, translocation into the ciliary compartment does not require vesicle formation and fusion. Similar to transmembrane proteins, however, cytoplasmic proteins must cross a size-selective diffusion barrier at the ciliary base.Citation10 The nature of this barrier appears to be very different from that which restricts the movement of transmembrane proteins. Inside the ciliary compartment, both transmembrane and cytosolic proteins appear to be actively transported, which implies that they interact with ciliary motors, such as kinesins and dyneins, and with adaptor complexes of these motors, such as the IFT particle.Citation4,Citation11,Citation12
Although some similarities exist between transport in the cytoplasm and intraflagellar transport, in general these two mechanisms are considerably different. This is particularly obvious for ciliary transmembrane proteins, which are embedded in vesicle membranes during cytoplasmic transport. In contrast to this, the same proteins translocate in the plane of the lipid bilayer of the ciliary membrane during intraflagellar transport in the cilium.Citation13,Citation14 Transport in the cytoplasm and intraflagellar transport also rely on different adaptor proteins. Intraflagellar transport requires the so-called IFT particle—a protein complex of over 20 proteins. In the absence of IFT particle components, intraflagellar transport does not occur and cilia do not form.Citation15-Citation18 In contrast, IFT proteins play only a limited role in cytoplasmic transport. Although exceptions exist and are discussed below, the majority of IFT proteins do not seem to be required to transport ciliary proteins in the cytoplasm.
An additional important difference between transport in the cytoplasm and intraflagellar transport of ciliary proteins is that these two transport modes appear to rely on different motors. The plus ends of intraflagellar microtubules are directed toward cilia tips (). Consistent with this, the anterograde intraflagellar transport that translocates proteins along the ciliary axoneme is driven by microtubule-dependent, plus end-directed motors (recently reviewed in ref. Citation19). These motors are homodimeric and heterotrimeric kinesin 2 family members, which also function in some other transport processes in the cell. The directionality of cytoplasmic microtubules in many cells is, however, opposite to that observed in cilia: their minus ends point toward the apical surface of the cell, which is where the ciliary centriole is located.Citation20-Citation22 This makes it improbable that kinesin 2 family motors move vesicles all the way from the Golgi apparatus to the ciliary centriole. As a consequence, to travel from the Golgi apparatus to ciliary tips, transmembrane proteins have to switch their motors. This motor exchange most likely occurs at the ciliary base.
The movement of ciliary proteins is restricted by diffusion barriers at the cilium base. These barriers are selectively permeable to both transmembrane and cytoplasmic proteins and regulate trafficking in both directions to keep some proteins outside and others inside the cilium.Citation23-Citation25 These characteristics suggest that barriers at the cilia base or transport mechanisms that move proteins across these barriers employ intricate recognition mechanisms to assure proper selectivity. Failure of barrier function has damaging consequences, which include cell death.Citation26-Citation28
Below, we review transport mechanisms that are thought to deliver protein cargo to the base of cilia and are likely to interact with ciliary gating mechanisms. Given the immense variety of ciliary cytosolic and transmembrane proteins, it is almost certain that multiple pathways mediate this process. We focus on the ciliary base and comment on transport in the cytoplasm and intraflagellar transport only to the extent to which these mechanisms are related to protein translocation across diffusion barriers at the interface of the ciliary compartment and the cytoplasm. Mechanisms that are discussed below facilitate ciliary localization of structural and signaling molecules, which include receptors, G-proteins, ion channels, and enzymes. The ciliary localization of these proteins is essential for cilia function in many aspects of development and physiology, such as organ formation, growth, morphogenesis, and function.
The Cilium Base is a Transport Hub
The base of the cilium is located in the cytoplasm, near the cell surface, and functions as a hub for protein transport into and out of the cilium. It consists of a centriole and membrane-associated structures: the septin ring, transition fibers, and the transition zone. A specialized membrane area, called the ciliary pocket, is found at the base of some cilia.Citation29,Citation30 The centriole’s main role is to provide a template for the extension of the ciliary axoneme and to give rise to transition fibers, which are anchored to the distal end of the centriole (). The membrane-associated structures are thought to be involved in regulating ciliary transport. The septin ring and transition fibers appear to concentrate and/or confine ciliary membrane proteins and components of ciliary transport machinery to the base of the cilium. In this area, intraflagellar transport (IFT) proteins appear to bind cargo proteins and, subsequently, transport them across the transition zone into the cilium. The transition zone is thus thought of as the gate into the cilium.
Septin Ring
Septins are a family of self-polymerizing GTPases. They are implicated in a variety of cellular processes and generally form membrane barriers. In the yeast bud neck, for example, septins separate the mother cell and the budding daughter cell into distinct membrane compartments reviewed in reference Citation31. In yeast, septin polymers appear to accomplish this by binding directly to the plasma membrane.Citation31 A septin ring is also observed at the base of cilia in IMCD3 cells and in airway epithelia.Citation23,Citation32 In addition, septins are observed in the annulus found at the base of the mammalian sperm flagellum.Citation33,Citation34 The annulus is thought to be homologous to the ring centriole found at the base of the insect sperm ciliumCitation35 and it is, therefore, likely that Septin association with cilia is conserved between vertebrates and invertebrates.
The physical connection between the septin ring and other structures at the base of the cilium, such as the transition zone, transition fibers and the ciliary pocket, is not well understood. Septin 2 forms a ring of 500 nm in diameter, which is consistent with ciliary pocket dimensions.Citation36 It is therefore tempting to speculate that the septin ring is associated with the cytoplasmic face of the ciliary pocket. However, Septin 2 localization in IMCD3 cells suggests that it is positioned between the axoneme and centriole appendage proteins Cep164 and Odf2.Citation23 More detailed ultrastructural studies will help to determine precisely where the septin ring is found.
In tissue culture, Septin 2 is required to localize transition zone proteins B9D1, CC2D2A, and TMEM231, which suggests that septins function upstream in the assembly of at least some transition zone structures.Citation24 Nevertheless, Septins are currently not known to interact directly with transition zone proteins. They are, however, essential for creating a diffusion barrier between the ciliary membrane and the plasma membrane.Citation23,Citation37 Evidence for this is provided by photo-bleaching experiments, which demonstrate that partial depletion of SEPT2 in IMCD3 cells results in an abnormally short cilium that displays an increase in the diffusional mobility of several proteins from the plasma membrane to the cilium.Citation23 In addition, in SEPT2-depleted cells, ciliary membrane proteins were also found in the plasma membrane surrounding the cilium.Citation23 These observations indicate that Septins limit protein movement between the plasma membrane and the ciliary membrane.
Transition Fibers and Distal Appendages of Centrioles
Transition fibers are fibrous links that extend from the distal sides of the mother centriole (the older of the two centrioles found in a centrosome, characterized by the presence of appendages at its distal terminus) to the plasma membrane (), and feature 9-fold symmetry that mirrors the symmetry of the centriole. They have been proposed to form a meshwork that carries out two important functions. First, it provides a large capacity-docking site for IFT proteins.Citation38,Citation39 Second, vesicles and large protein complexes may be blocked from entering the cilium by the transition fiber barrier. As a consequence, vesicles that carry ciliary membrane proteins may have to fuse with the plasma or ciliary membrane (the boundary between the two is not clearly defined) at the periphery of the transition fibers.Citation40
One model hypothesizes that transition fibers and Septins function together to delimit a “landing strip” around the base of the cilium, an area that is isolated by a diffusion barrier from the rest of the plasma membrane.Citation23,Citation41 In this area, cytoplasmic vesicles that carry membrane proteins destined for the cilium fuse to the target membrane. Once membrane cargo proteins “land” in this area, they interact with IFT transport machinery to cross the transition zone.
The precise composition of transition fibers is not clear. They do, however, appear to include ODF2/Cenexin and, therefore, may be related in some way to distal appendages of the mother centriole.Citation42 ODF2 and other distal appendage proteins, such as Cep164 and Cep83, function in centriole docking.Citation43-Citation45 Docking is a process that results in the attachment of the mother centriole to the plasma membrane and is essential for cilia formation.Citation46 The presence of ODF2/Cenexin in transition fibers suggests that they may be assembled as an extension of distal appendages and anchor the centriole to the plasma membrane after the initial docking event.
It was suggested some time ago, and confirmed by recent studies, that IFT proteins accumulate near transition fibers.Citation38,Citation39 In support of the idea that distal appendages and transition fibers are related, a distal appendage protein C2cd3 is critical for both centriole docking and the recruitment of the intraflagellar transport proteins Ift88 and Ift52 to the mother centriole.Citation47 Furthermore, a distal centriole protein, CCDC41, appears to act in concert with IFT20, to support vesicle-centriole association.Citation48 These functional similarities between distal appendages and transition fibers suggest that distal appendages differentiate into transition fibers during ciliogenesis.
The Transition Zone
The transition zone is found just distal to the ciliary centriole (i.e., basal body) and is the most proximal part of the membrane-covered cilium (). The structural hallmark of the transition zone is the presence of attachments, seen on electron micrographs, between the axoneme and the ciliary membrane, referred to as “Y links.”Citation49,Citation50 The location and structural features of the transition zone led to the idea that it provides a gating mechanism that controls transport into the cilioplasm and the ciliary membrane.Citation24,Citation25,Citation51,Citation52 Support for this is provided by several observations. First, biochemical analysis of isolated Chlamydomonas flagella revealed that mutations in Cep290, a transition zone protein, result in abnormal flagellar protein content.Citation51 Second, the trans-membrane TRAM proteins, which normally localize to the dendrite plasma membrane and are excluded from the cilium, are found inside the cilium in certain C. elegans transition zone mutants.Citation25 Third, knockdown of multiple transition zone proteins in primary hippocampal neurons by RNAi reduces the amount of somatostatin receptor (SSTR3) in the cilium and, likewise, transition zone proteins are required to restrict non-ciliary-membrane proteins from cilia in IMCD3 cells.Citation24 Finally, in the mouse, the transition zone protein, Tectonic, is required to localize the membrane-associated and trans-membrane proteins Arl13b, AC3, Smoothened, and Pkd2 to cilia.Citation52 These gating functions of transition zone proteins allow the cilium to function as a distinct subcellular compartment that executes numerous signaling functions with high efficiency and without interference with metabolic and enzymatic processes in the cytoplasm.
To date, multiple transition zone proteins have been identified (). The domain content of these proteins, such as the presence of coiled-coil regions, is suggestive of a structural role. Transition zone proteins also feature transmembrane domains or membrane association motifs, such as B9/C2, which suggests that they may attach the multi-component ciliary gate complexes to the membrane.Citation25,Citation53
Figure 2. Transition zone protein modules. (A–C) Transition zone proteins classified into MKS (Meckel-Gruber Syndrome) module components (A), NPHP (Nephronophthisis) module constituents (B) and others that are not currently categorized (C). Some of the proteins, when mutated, lead to other syndromic diseases such as Joubert syndrome (JBTS). (D) The transition zone protein Cep290 is conserved in both of the main branches of eukaryotic evolution.Citation51,Citation55,Citation199
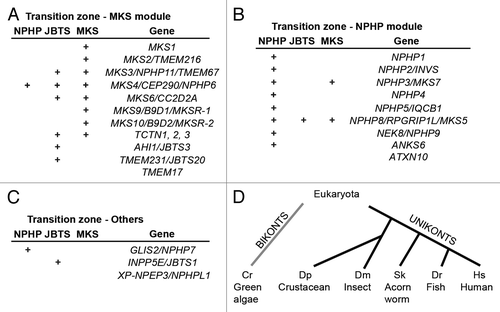
A major structural role for transition zone proteins is to form Y-links. Studies in tissue culture, mouse, and Chlamydomonas have shown that Cep290 contributes to Y-link formation.Citation51,Citation54 This is a large coil-coiled domain protein, which theoretically could span the space between the axoneme and ciliary membrane.Citation52 This would be the case if the Cep290 C-terminal microtubule binding domain associated with axonemal microtubules and its N-terminus with the membrane.Citation55 In addition, Cep290 contains many coil-coiled regions and is predicted to assume an elongated conformation.Citation51 Overall, Cep290 may act as a large scaffold to organize the transition zone and assist in the formation of the Y-links. Similar to Cep290, mutations in several Nephrocystins and Meckel-Gruber syndrome proteins affect Y-link formation.Citation25 As discussed below, these proteins form functionally redundant modules.
Given the diversity of ciliary proteins and changes of ciliary content that occur under different physiological conditions, one would expect that transport across the transition zone is regulated by proteins with enzymatic activity. Surprisingly however, only one group of transition zone proteins, NIMA-related kinases, or NEKs, displays enzymatic activity. This group includes NEK8 in mammals and Fa2p in Chlamydomonas. In mammals, complete loss of NEK8 disrupts the localization of polycystin-1 and polycystin-2, as well as the function of multiple signaling pathways.Citation56,Citation57 In contrast, the Chlamydomonas NIMA family-related kinase, Fa2p, localizes to the transition zone and is implicated in microtubule severing during deflagellation.Citation58,Citation59 The Fa2p protein may, therefore, have a role that is distinct from the regulation of ciliary transport. It is noteworthy that in tissue culture cells Nek2 plays a role in cilium disassembly. This kinase is found, however, in the distal portion of the centriole and is not known to be a transition zone component.Citation60 More recently, the cell cycle-regulated Polo-like kinase (Plk1) was found to localize to the transition zone and bind the transition zone protein NPHP1.Citation61 Also in this case, Plk1 function is implicated in cilium disassembly. Given the paucity of transition zone proteins that display enzymatic activity and have the potential to regulate ciliary transport, regulatory roles are likely to be performed by other groups of proteins. Small GTPases are good candidates for such regulators and Ran, Rabs, and Arls are implicated in ciliary transport.Citation62-Citation65
Mutations in transition zone proteins result in a broad spectrum of diseases, collectively known as ciliopathies. Remarkably, mutations in a single transition zone protein can cause a wide range of disease symptoms of varying severity. For example, mutations in CEP290 produce phenotypes ranging from relatively mild disorders, such as Retinitis PigmentosaCitation66,Citation67 and Leber’s Congenital Amaurosis,Citation68 to progressively more severe diseases, which include Nephronophthisis/Senior-Loken syndrome,Citation69,Citation70 Joubert syndrome (JBTS),Citation68,Citation71 and Meckel-Gruber syndrome (MKS).Citation72 Why a particular mutation in a transition zone protein results in one phenotype but not another remains unclear. One possibility is that distinct transition zone proteins, or domains of a single protein, interact with different sets of ciliary cargo polypeptides. Since ciliary cargo molecules are very diverse and differ considerably in different tissues, the identity of the mutant protein or the mutant domain within a polypeptide will dramatically affect the phenotypic outcome.
Modularity of transition zone components
Systematic genetic analyses of transition zone proteins, performed in C. elegans, mammalian cells and the mouse, suggest that they are organized into at least two groups, referred to as the NPHP and MKS modules ().Citation24,Citation25,Citation52 Defects in human NPHP and MKS module proteins cause mainly Nephronophthisis (NPHP) and Meckel-Gruber Syndrome (MKS), respectively. The MKS phenotype is more severe (perinatal lethality), compared with the NPHP phenotype (kidney disease and retinal degeneration), which suggests that the MKS module may play a more fundamental role. For example, MKS module proteins may have an important structural role in the transition zones of all cilia, whereas NPHP module proteins may function in transition zones of selected, specialized cilia. With few exceptions, which are discussed below, functional differences in transition zones of cilia in different tissues have not yet been characterized.
In C. elegans, where cilia are found only in sensory neurons, the NPHP and MKS modules are largely redundant and strong ciliary phenotypes are observed when mutations in genes belonging to both modules are combined.Citation73 For example, in a dye-filling assay, which is commonly used to evaluate cilia differentiation in this model organism, mks-6 or nphp-4 mutations alone display mild phenotypes, whereas the phenotypes of mks-6; nphp-4 double mutants are much more severe.Citation25 In contrast, only mild dye-filling is observed in mks-6 mutants combined with other MKS mutants, such as mks-1 and mks-3.Citation25
In agreement with genetic studies that indicate the presence of functional modules, biochemical analyses of transition zone proteins reveal that they form complexes. The Mks1, Mks6, and Tectonic proteins form a complex that is important for neural tube closure.Citation74 Likewise, all three B9-domain proteins, B9D1, B9D2, and MKS1, which functionally belong to the MKS module, co-immunoprecipitate with any epitope-tagged B9 protein.Citation75 In C. elegans, the localization of any one of the B9 proteins is disrupted by mutations in the other two.Citation76 Within the NPHP module, it was proposed that NPHP1, NPHP4, and NPHP8 bind each other at the transition zone.Citation74 Finally, NPHP proteins NPHP2/Inversin, Nphp3, Nek8/NPHP9, and ANKS6 also form a complex, which defines the so-called Inversin compartment found at the base of the cilium, above the transition zone.Citation77-Citation79 The significance of the modular structure of the transition zone is not clear. Perhaps it is related to multiple, evolutionarily conserved functions mediated by this part of the cilium. It will be interesting to examine the evolution of these modules and determine whether redundancy results in module elimination or, alternatively, adaptation to new functions in certain phyla.
Evolutionary considerations
Transition zone proteins are conserved in the eukaryotic tree of life, which indicates that they are ancient components of the cilium and likely play important roles in its biology. For example, the transition zone protein Cep290 is found in both main branches of eukaryotic evolution: the Unikonts (eukaryotes whose ancestors had a single cilium), and Bikonts (eukaryotes whose ancestors had two cilia) ().Citation80-Citation82 To date, only two transition zone proteins (Cep290 and a NIMA-related kinase Fa2p) have been studied in Bikonts. The aforementioned study of Cep290 in Chlamydomonas suggests that it has a conserved role in regulating ciliary compartmentalization.Citation51 In contrast, the analysis of Chlamydomonas Fa2p points to a role in microtubule severing during deflagellation and it is not clear whether Fa2p function is conserved.Citation58
Little is known about the role of other transition zone components in unicellular organisms and to what extent transition zone proteins have changed their function during evolution. For example, in Chlamydomonas, Cep290 is found only in the transition zone,Citation51 whereas mammalian Cep290 is also present in centrosomal and centriolar satellites.Citation83,Citation84 According to one hypothesis, the original role of centrioles is to form cilia and centriole involvement in centrosome and cell division is a later metazoan innovation.Citation85 Therefore, it is possible that Cep290 localization outside of the ciliary transition zone is a more recent evolutionary adaptation.
Specialized transition zones
Although ciliary transition zones are structurally very similar in diverse cells and tissues of organisms as different as Chlamydomonas, C. elegans, and Homo sapiens, in some cases transition zones appear to develop adaptations to specific tasks. Two examples of this have been characterized in sensory neurons and sperm cells. Transition zones in sensory neurons are longer compared with those in most cells and are referred to as “connecting cilia” () (reviewed in ref. Citation86). The exceptional length of these transition zones is presumably a structural requirement that reflects the unusual morphology of cilia in sensory cells. Another special case has been described in sperm cells, where the centriole is attached to the nucleus, the proximal end of the axoneme localizes to the cytoplasm and only the distal part of the axoneme appears to be confined in a separate membrane compartment () (reviewed in ref. Citation87). This unique cilium configuration appears to satisfy two special needs. The first is to produce a hydrodynamically streamlined shape that supports effective sperm swimming. The second is to position mitochondria, which provide an energy source, close to the axoneme, presumably, to facilitate flagellar motility. Since mitochondria are too bulky to enter the cilium, it appears that sperm cells place the proximal end of the axoneme in the proximity of mitochondria in the cytoplasm.
Figure 3. Specialized transition zones. (A) Vertebrate photoreceptors and Drosophila sensory neurons share similar cellular architecture and an atypical transition zone, known as the connecting cilium. The outer segments of vertebrate photoreceptors measure 20–25 um in rods and 10–15 um in cones of most species.Citation200-Citation202 Outer segments of fly sensory neurons are approximately 5–50 um long.Citation203,Citation204 Modified from Avidor-Reiss et al., 2004. (B and C) Illustration of compartmentalized (B) and cytoplasmic (C) ciliogenesis, featuring transition zone and ring centriole/annulus, respectively.
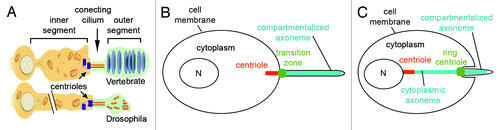
Sensory neurons
The so-called “connecting cilium” is found in insect sensory neurons and in vertebrate photoreceptors.Citation50,Citation88 In contrast to the transition zone of most cilia, the connecting cilium is formed between two bulky compartments, referred to as the “inner” and “outer” segment, which display much greater width and volume, compared with the transition zone itself (). This contrasts with the majority of cells in which the transition zone is as narrow as the rest of the cilium (). The high volume of the outer segment and the remarkably active protein traffic in connecting cilia is likely to impose unusual structural requirements, such as increased mechanical strength. This may explain why connecting cilia are longer than a typical transition zone: approximately 1μm in vertebrate photoreceptorsCitation89 and 0.6 μm in Drosophila mechanosensory neurons while typical transition zones in non-sensory cells are 0.2 μm long. This length difference is reflected in transition zone ultrastructure, which features 2-7 rows of Y links in most cilia and 30–40 rows in photoreceptors see in ref. Citation49.
Sperm Cells
Cilia in most cells assemble and maintain their axoneme within a membrane compartment that projects from the surface of the cell. In these cilia, the transition zone forms at the base of the membrane projection, close to the distal end of a centriole, which is located next to the cell’s plasma membrane.Citation40,Citation54,Citation90 In contrast, in cytoplasmic cilia, found in mammalian and Drosophila sperm cells, as well as in the microgametes of the malarial parasite Plasmodium, the axoneme localizes, at least partially, in the cytoplasm and the centriole is not associated with a membrane-enclosed axoneme ().Citation81,Citation91-Citation93
In the sperm cells of most animals, the centriole is anchored to the nuclear membrane and only the tip of the axoneme is located inside a cap-like membranous structure that is morphologically similar to a typical cell surface ciliary compartment.Citation94,Citation95 Candidate Drosophila transition zone proteins, such as Uncoordinated (thought to be homologous to OFD1), Chibby, and Dilatory (CEP131), localize to the ciliary cap base in sperm cells.Citation96-Citation98 These observations suggest that the base of the membrane-bound compartment in sperm axoneme is analogous to the transition zone in typical cilia.
Transition Zone Interactions with Ciliary Transport Mechanisms
How proteins targeted to the cilium are transported across the transition zone remains unclear. Several scenarios are possible and these are not necessarily mutually exclusive. One idea is that cytosolic proteins are first targeted to a site near the centriole or transition fibers.Citation38,Citation39 These proteins bind, sometimes as complexes, to IFT machinery and become its cargo.Citation99 For example, radial spokes, which are large axonemal complexes that consist of 22 polypeptides, are partially assembled in the cytoplasm before being transported to the flagellar tip by anterograde IFT.Citation99 In this model, the IFT machinery, which is licensed to cross the transition zone, delivers the cargo into the cilioplasm, and the capacity of transition zone proteins to interact with the IFT machinery is essential for ciliary entry.Citation100 Specificity is determined by the ability of the cargo to interact with IFT proteins. The key element of this model is thus the molecular basis of interactions between IFT proteins and the ciliary cargo molecules that are being transported into the cilium. In some cases, cargo-IFT interactions are direct,Citation4 while other cargo proteins may require the involvement of additional cargo-specific adapters.Citation101
Complexes of IFT proteins are very large. In Chlamydomonas, anterograde IFT trains fill the gap between the axoneme and the ciliary membrane, and are 700 nm long.Citation13,Citation14 They are unlikely to cross the transition zone without hindrance due to the presence of structures such as transition fibers and Y-links. Therefore, IFT proteins may have unique characteristics that allow them to interact with the transition zone. Indeed, at least some transition zone proteins interact with IFT particle components.Citation101 This may facilitate the transport of IFT associated cargo proteins into the cilium. The nature of interactions between transition zone proteins and IFT particles remains largely unknown. One can imagine, however, that IFT proteins induce conformational changes in the transition zone, which then open a path for IFT trains to go through.
In some circumstances, the regulated entry of cargo into cilia can occur independently from IFT. In Chlamydomonas, the flagellar adhesion-induced 65-kDa-membrane protein, SAG1-C65, can translocate into the cilium under the restrictive conditions of the temperature-sensitive kinesin-2 mutant, fla10, which blocks IFT.Citation102 This IFT-independent ciliary entry requires cytoplasmic microtubules but it is not clear whether it occurs due to active transport or a temporary relaxation of the ciliary diffusion barrier.
Active transport is not the only manner in which proteins can enter the cilium. There is evidence that small proteins can diffuse into the ciliary shaft, which may be limited mainly by steric hindrance that is imposed by the narrow distance between the axoneme and ciliary membrane. In an in vitro assay, using cells in which the plasma membrane was made permeable but the ciliary membrane remained intact, it was found that proteins >9 nm in diameter (100 kD) are restricted from entering cilia.Citation103 Similarly, a newly developed methodology, which relies on intact cells and has been termed “chemically inducible diffusion trap at cilia,” has provided estimates that proteins up to 7.9 nm in diameter can diffuse into the cilium.Citation104 Consistent with the idea that the ciliary base acts as a gate, mathematical modeling in this study suggested that a molecular sieve acts as a passive barrier at the cilium base. Another study, which relied on fluorescently labeled dextrans and recombinant proteins of various sizes, found that 40K and 70K dextrans and the 67K bovine serum albumin were significantly restricted from entering the cilium, while smaller dextran molecules and recombinant proteins could freely enter the cilioplasm.Citation10 In photoreceptor sensory cilia, limits on the speed of diffusion appear to be imposed by the size of the protein relative to the highly constrained spaces between outer segment disc membranes.Citation105 This study compared the entry of GFP monomers, dimers and trimers into the frog outer segment and concluded that ciliary entry may be controlled by a steric volume exclusion mechanism that does not require a diffusion barrier.
Estimates of barrier permeability vary, which may be due to the different shapes of tested cargo molecules (globular vs. rod-like) or disparate physical parameters of the transition zone in different cells. The photoreceptor cilium appears to be more permeable, compared with cilia of RPE cells.Citation10,Citation105,Citation106 This is somewhat counterintuitive as the photoreceptor transition zone is far longer, compared with that in most other cell types.Citation49 The length of the transition zone in photoreceptors may, however, reflect its mechanical role in supporting the bulky outer segment or the need to prevent retrograde diffusion of opsin, which is highly concentrated in the cilium of these cells. Despite being exceptionally long, the photoreceptor transition zone may need to be permeable to cytosolic proteins in order to accommodate machinery that supports massive anterograde opsin transport, which, at least in some species, is estimated to occur at 1000 molecules/s.Citation107
Another potential ciliary gating mechanism has emerged from electron microscopic analysis of Tetrahymena flagella.Citation108 This study described a structure at the ciliary base, termed the “ciliary partitioning system” (CPS), which appears to block the ciliary entry of cytoplasmic proteins. It features nine openings, one near each of the axoneme doublet microtubules, that could allow cargo-loaded IFT particles to enter the cilium. It is not clear, however, whether the findings of this analysis can be extrapolated to cilia in other organisms.
Ciliary Targeting Sequences
How are the proteins involved in cilia formation and function identified as cargo by ciliary transport machinery? Studies of cilia proteins have revealed specialized signal sequences, known as ciliary targeting sequences (CTS), which direct them to the ciliary compartment. In transmembrane proteins, CTSs are found on the cytoplasmic side of the membrane, both in intracellular vesicles and in the ciliary shaft. The CTSs most often localize to the C-termini of proteins, less frequently to N-termini and in some cases to intracellular loops of muti-pass transmembrane proteins (). The cilia targeting sequences are quite diverse, which suggests that they interact with different pieces of the transport machinery. This is not unexpected as multiple pathways are likely to mediate protein targeting to cilia.
The most common molecular feature of ciliary targeting sequences is the VxP motif, characterized only in transmembrane and membrane-associated proteins so far, which include G protein-coupled receptors (GPCRs), polycystins, CNG channels, and retinol dehydrogenase (). In experimental conditions, the VxP motif is frequently necessary for ciliary targeting and its mutations abolish ciliary localization (references in ). Human mutations in the VxP motif of rod opsin cause rapid rod photoreceptor degeneration (reviewed in ref. Citation109), most likely as the result of ectopic opsin accumulation in the cell body. However, not all C-terminal VxP motifs in ciliary proteins are essential. Mutation of the VxP (VRP) residues in the C-terminus of INPP5E, a ciliary inositol polyphosphate phosphatase, does not impact the ciliary localization of INPP5E.Citation110 Apart from opsin, the role of VxP in GPCRs has not been tested.
The opsin VxP motif is necessary but not sufficient for ciliary targeting. The C-terminal sequence of 44 amino acids in the opsin protein (hereafter opsinC44), which contains the VxP motif, is, however, sufficient to target exogenous proteins, such as GFP, to photoreceptor cilia.Citation111 The targeting function of opsinC44 requires that it is associated with a membrane. In the wild-type opsinC44, this requirement is fulfilled by the palmitoylation of two sequential cysteine residues ().Citation111 Deletion of these cysteines abrogates ciliary targeting. Surprisingly, this effect is more severe than that caused by a loss of the C-terminal VxP motif from a similar construct, and a myristoylated GFP is targeted more efficiently to cilia than GFP fused to non-palmitoylated opsinC44.Citation111 These observations highlight the peculiar importance of membrane association for ciliary targeting in photoreceptors.
Further analysis of photoreceptors has revealed that transmembrane proteins are generally targeted to photoreceptor cilia unless they contain signals that direct them elsewhere.Citation112 This finding is, however, more than likely a peculiarity of vertebrate photoreceptors as it has not been observed in other cells.Citation112 The unusual behavior of photoreceptors is believed to result from the exceptionally active transport of membrane material from the cell body into the ciliary compartment, necessitated by the continuous shedding of membrane in the distal region of photoreceptor cilia at the rate of approximately 10% per day.Citation113,Citation114 It is this unusual feature that results in the photoreceptor cilium being a default destination for the trafficking of transmembrane proteins. In other cells, membrane association of proteins appears to be neither necessary nor sufficient for ciliary targeting.
In addition to the VxP sequence and palmitoylation sites, opsinC44 contains the FR motif that was originally identified in a nematode olfactory GPCR, ODR-10.Citation115 This juxtamembrane motif consists of a hydrophobic amino acid followed by a basic amino acid and is also found in cytoplasmic tails of other GPCRs.Citation116 The importance of this motif has not been tested in opsin but its ablation eliminates ciliary targeting of ODR-10 and Smoothened.Citation115,Citation116
The VxP motif is present in many GPCRs ( in ref. Citation117). In contrast to opsin, however, it is not positioned at the very C-terminus of the protein sequence and its importance has not been experimentally tested. Many GPCRs feature an additional targeting sequence in the 3rd intracellular loop (hereafter I3-CTS) (). For example, a somatostatin receptor, SSTR3, contains the AxxxQ motif in this region.Citation117 This motif is absent in five other SSTR receptors that are expressed in the rat CNS but do not localize to cilia.Citation117
The importance of the I3-CTS has been demonstrated by domain swap experiments. Although the wild type SSTR5 receptor does not localize to cilia, a chimeric version of SSTR5, which contains the 3rd intracellular loop from SSTR3, does. Mutating the AxxxQ motifs in the SSTR3 I3-CTS abolishes the ciliary localization of this chimeric SSTR5/3.Citation117 Similar experiments have been performed for HTR6 and also demonstrated the importance of the AxxxQ motif in the 3rd intracellular loop.Citation117 Not all I3-CTS motifs of GPCRs are, however, related to each other. The neuropeptide Y receptor, NPY2R, contains two I3-CTS motifs that show no similarity to those in SSTRs.Citation118
In contrast to opsin, another abundant membrane protein of photoreceptor cilia, peripherin, does not feature the VxP motif.Citation119 Peripherin CTS is unrelated to that found in opsin. This dissimilarity of opsin and peripherin CTS is consistent with genetic studies. Mutations that affect opsin transport do not affect peripherin.Citation101,Citation120-Citation122 Based on these observations, it has been suggested that these two transmembrane proteins are translocated into the same ciliary compartment by different pathways.Citation119
The CTSs of cytoplasmic proteins are less well characterized. The targeting sequences characterized thus far in Gli and Kif17 are unrelated to one another (). The CTS of the Kif17 kinesin is a stretch of basic amino acids that is related to nuclear localization signals (see below). Whether similar signals function in other cilia-targeted cytosol-soluble proteins remains to be determined.
CTS-interacting Proteins
Several CTSs are well conserved in orthologous proteins throughout vertebrate evolution, at the level of the primary sequence.Citation110,Citation123 This suggests that they mediate interactions with the components of transport machinery. Indeed, binding partners have been described for CTSs in GPCRs and a ciliary phosphoinositide phosphatase, INPP5E.
To determine which proteins bind opsinC44 and target opsin to the photoreceptor cilium, several groups have used proteomic approaches. This included cross-linking and yeast two-hybrid experiments that used opsin C-terminal sequences as the bait.Citation7,Citation124,Citation125 Molecular analyses of proteins identified via these approaches suggest that they function sequentially at several stages of opsin transport. It is believed that opsin transport from the Golgi to the cilium is initiated by a small GTP-ase, Arf4, which binds the opsin CTS and appears to mediate the budding of opsin-carrier vesicles from the trans-Golgi network.Citation124 It has been recently proposed that Arf4 enhances the binding of an Arf GAP, ASAP1, to the FR motif in opsinC44. This, in turn, initiates the assembly of the Rab11/Rabin8/Rab8 complex in the cytoplasm and is thought to trigger opsin translocation to cilia recently reviewed in references Citation3,Citation5,Citation126.
Once formed at the Golgi, opsin carrier vesicles are translocated to the base of the cilium.Citation127,Citation128 Based on results of a yeast two-hybrid screen, which revealed that the opsin C-terminus interacts directly with cytoplasmic dynein, it has been suggested that microtubule-dependent transport accounts for opsin translocation through the cytoplasm.Citation7 Support for this idea is also provided by observations that cytoplasmic Dynein-1 heavy chain (DYNC1H1) co-purifies with IFT complex A proteins. As discussed below, in addition to their role in ciliary IFT, complex A proteins are thought to function at the base of cilia to facilitate the transport of GPCRs.Citation6 Evidence has also been presented, however, for the transport of opsin by microtubule-independent mechanisms.Citation129 The contribution of microtubule-dependent motors to the transport of GPCRs in the cytoplasm requires further investigation.
In contrast to opsin, many GPCRs require I3-CTS for their ciliary localization. The function of the I3-CTS appears to be mediated by BBS proteins. Evidence for this has been provided by the observations that mouse knockouts of BBS2 and BBS4 abolish the ciliary localization of SSTR3 and MCHR1.Citation130 Similarly, interference with BBS3 and BBS4 in tissue culture disrupts ciliary targeting of a chimeric transmembrane receptor that contains SSTR3 I3-CTS.Citation8 In protein interaction tests, I3-CTS of SSTR3 pulls down all BBSome components tested, while the I3-CTS of the dopamine receptor 1 appears to interact directly with BBS5.Citation8,Citation131 A likely function of I3-CTS is thus to integrate GPCRs in membrane coats formed by BBS proteins.Citation8
Finally, in INPP5E, CTS is also found at the C-terminus and mediates direct interactions with a small GTP-ase, Arl13b. Mutations in the sequence of this CTS abolish Arl13b binding.Citation110 The studies of ciliary targeting sequences have been highly informative and are likely to continue to generate valuable insights. The binding partners of most CTSs remain unknown.
The Rab8/Rab11 Cassette: A Central Component of the Ciliary Transport Hub?
A number of recent studies have revealed the importance of a cassette of two small GTPases, Rab8, and Rab11, in cilia-directed traffic (). The first indication that Rab8 may play a central role in ciliary transport came from the observation that the GDP-locked variant of Rab8 causes a striking accumulation of vesicles around the ciliary centriole when overexpressed in photoreceptor cells.Citation132 Later studies revealed that Rab8 localizes to the ciliary membrane and is a major component of a mechanism that drives cilia formation in general.Citation133,Citation134 The overexpression of GTP-locked mutant Rab8 results in cilia elongation and uncoupling between cilia length and the extent of the acetylated region on the ciliary axoneme.Citation133,Citation135,Citation136 These observations suggest that Rab8 acts as a potent activator of vesicle fusion to membranes at the cilia base.
Further analysis demonstrated that Rab8 is a downstream component of a pathway that involves Rabin8 and Rab11. The Rabin8 protein functions as a Rab8 guanine nucleotide exchange factor (GEF) and is found at the cilia base.Citation133,Citation135,Citation136 In agreement with its role in stimulating Rab8 activity and cilia formation, Rabin8 presence at the ciliary centriole is transient and correlates with ciliogenesis.Citation136 The Rab11 protein localizes Rabin8 to vesicles at the cilia base and in the GTP-bound state stimulates its GEF activity.Citation135 All three proteins are required for normal ciliogenesis in tissue culture conditions.Citation133,Citation135-Citation137
Several additional studies showed that the Rab11/Rabin8/Rab8 pathway is functionally related to vesicle trafficking by the exocyst and TRAPP vesicle tethering complexes, and BBS protein membrane coats.Citation133,Citation136,Citation137 In addition, Rab8 and Rabin8 bind centriolar appendage proteins, ODF2 and Cep164, respectively, which indicates that they may function in vesicle docking in the basal body area.Citation45,Citation138 In agreement with this, CEP290 is also necessary for Rab8 localization to the ciliary membrane.Citation84 Finally, Rab8 binds a Rab effector protein, Rabaptin5, which in turn appears to interact with Elipsa/IFT54, an IFT particle component.Citation134 These observations place the Rab8/Rabin8/Rab11 pathway at the center of several molecular events that are likely to facilitate vesicle delivery to the cilia base: vesicle coat formation, recognition of the vesicle target site, tethering at the target site and, potentially, interaction with transport machinery that will carry vesicle cargo further into the ciliary shaft.
The picture of Rab8/Rabin8/Rab11 function is still incomplete however. Although several cell culture studies suggest that Rab8 is a key regulator of ciliogenesis,Citation133,Citation136 a double knockout of both Rab8 genes (two are found in the mouse genome) does not affect cilia in the mouse.Citation139 A knockdown of a related gene, Rab10, in the Rab8a−/−;Rab8b−/− double mutant background does, however, cause cilia defects.Citation139 This result reveals an unexpected level of redundancy in ciliary Rab function, an area that requires further investigation. Likewise, as discussed below, factors that function upstream of Rab11, its GEFs in particular, remain to be identified.
The exocyst
The exocyst is a vesicle tethering complex that is closely related to the Rab8/Rab11 ciliogenic pathway (). In both yeast and mammals, the exocyst consists of eight proteins that mediate exocytosis.Citation140 Intriguing similarities exist between ciliary GTPases and Rabs that regulate yeast exocyst function. Yeast Rabs, Ypt31 and Ypt32, interact with an effector, Sec2p, which, in turn, functions as a GEF for Sec4p.Citation141 It has been proposed that Ypt31/32, Sec2p, and Sec4p are functionally homologous to Rab11, Rabin8, and Rab8, respectively,Citation142 and thus related Rab cascades may function in ciliogenesis and in the budding of yeast cells.
The Rab8/Sec4p protein, in the GTP-bound state, interacts with an exocyst constituent, Sec15, both in yeast and in higher eukaryotes. This led to the conclusion that the exocyst complex functions as a Rab effector and mediates Rab8/Sec4p function.Citation143,Citation144 Is this also the case in ciliogenesis? Indeed, the exocyst does seem to play some role in cilia formation as several of its components, such as Sec 6, 8, and 15, localize to ciliaCitation145,Citation146 and the knockdown of some others, Sec10 and Sec15 in particular, inhibits cilia formation in tissue culture conditions.Citation146,Citation147 The role of human Sec15 in ciliogenesis is further supported by observations that it binds Rabin8, an interaction that is enhanced by the phosphorylation of Rabin8 by NRD2, a kinase known to function in cilia.Citation137,Citation146 Based on knockdown results, exocyst function is not confined to a specialized subset of ciliary membrane cargo proteins, but rather appears to have a more general role in cilia formation. By analogy to yeast exocyst function, it is likely to act downstream in the Rab11/Rab8 ciliogenic pathway, possibly facilitating vesicle fusion with periciliary membranes.
TRAPPII complex in ciliary trafficking
Another Rab8/Rab11-related mechanism in the delivery of cilia-bound vesicles appears to involve TRAPPII, a vesicle tethering complex that consists of 10 cytoplasmic proteins and is best known for its role in trafficking to Golgi membranes (reviewed in ref. Citation148) (). Evidence for its relatedness to the Rab8/Rab11 pathway is provided by TAP-tag experiments showing that all TRAPPII complex constituents co-purify with Rabin8.Citation136 Moreover, RNAi depletion of three TRAPPII complex components, TRAPPC3, TRAPPC9, and TRAPPC10, leads to a loss of Rabin8 localization from the pericentrosomal area and impaired ciliogenesis.Citation136 These experiments strongly suggest that TRAPP proteins contribute to cilia formation.
The idea that the TRAPPII complex functions in the Rab8/Rab11 pathway has been recently strengthened by the observation that TRAPPC10 contains a longin domain, which is a protein interaction module frequently found in Rab GEFs.Citation149 This has led to the proposition that a dimerization of longin domains from TRAPPC10 and TRAPPC2 provides a surface for Rab11 binding.Citation149 As TRAPP complexes are known to display GEF activity toward Rab GTPases, including Ypt31/32 (putative yeast homologs of Rab11), ample precedent exists for a potential role of the TRAPPII complex as a Rab11 GEF.Citation150,Citation151 No other candidate Rab11 GEF has been identified thus far and so the mechanism of Rab11 activation remains unknown.Citation149 The TRAPPII complex could function upstream of the Rab11/Rab8 pathway by activating Rab11, and thereby trigger a series of events that eventually result in vesicle fusion. This idea appears less convincing, however, in the light of observations that only a subset of TRAPPII complex components display knockdown phenotypes that affect ciliogenesis.Citation136
BBS proteins as membrane coats
A major contributor to ciliary GPCR transport appears to be a complex of proteins encoded by genes originally identified as defective in a human ciliopathy, the Bardet-Biedl Syndrome (BBS) ().Citation152 Quite remarkably, seven of these proteins co-purify in tandem-affinity purification (TAP) experiments. This led to the realization that they form a complex, which was named the BBSome.Citation133 As the number of BBS genes exceeds the number of BBS proteins that co-purify in TAP assays, some BBS proteins may be less tightly associated or form separate complexes. Indeed, three chaperonin-related BBS proteins, BBS6, BBS10, and BBS12, are thought to form another complex that mediates BBSome assembly.Citation153
Genetic studies have shown that BBS complex components are required for the ciliary localization of opsin, somatostatin receptor 3 (SSTR3), melanin concentrating hormone receptor (MCHR1), neuropeptide Y receptor (NPYR2) and potentially other GPCRs.Citation118,Citation122,Citation130,Citation154 These observations are complemented by intriguing cell biological and protein-protein interaction data showing that, at least in vitro, BBS proteins form coats on the surface of lipid vesicles.Citation8 Formation of BBS membrane coats requires a non-core BBS protein, Arl6. This is a small GTP-ase, also known at BBS3, which interacts with membrane lipids via an N-terminal amphipathic helix.Citation8,Citation155 The idea that BBS proteins form vesicle coats also finds support from bioinformatic analyses, which suggest a similarity between ciliary transport proteins and vesicle coat components that function in ER to Golgi transport (COPI, COPII) or the internalization of surface proteins (Clathrin coated pits).Citation8,Citation81,Citation156,Citation157
By analogy to COPI, COPII and Clathrin coated pits, one function of BBS membrane coat complexes could be to trap GPCRs in vesicles and direct their translocation into the cilium. As discussed above, experimental support for this idea comes from genetic and biochemical analyses of interactions between GPCR I3-CTS sequences and BBS proteins.Citation8,Citation130,Citation131 These experiments provide strong evidence that BBS proteins participate in the ciliary targeting of GPCRs. The exact mechanism of how this occurs remains, however, unclear. Some insight into this process has come from observations that the absence of BBS proteins does not affect cell surface targeting of cilia-bound cargo but rather shifts its localization from the ciliary membrane to plasma membrane.Citation8 Nevertheless, the existing data does not allow one to conclusively discriminate between several models. In one scenario, BBS coats could assemble on membranes of intracellular vesicles in a CTS-dependent-manner to target vesicles to the ciliary compartment. However, direct evidence for the presence of such coated vesicles is missing, as BBS proteins have not been detected on intracellular membranes thus far.Citation8 Alternatively, BBS proteins could assemble membrane-associated complexes, referred to as planar coats, on the cell surface outside cilia, bind GPCRs and then translocate them across the diffusion barrier into the ciliary compartment. This model finds support in the realization that IFT trains, which contain BBS proteins, can be thought of as planar coats.Citation157-Citation159 Yet another possibility is that BBS coated vesicles transport ciliary proteins from the cell surface to the periciliary endosome, which serves as a relay station on the way to cilia.Citation160 Clearly, further experimentation is required to resolve this matter.
It is noteworthy that a link between BBS proteins and the Rab8/Rab11 cassette has been established, although its functional importance is not entirely clear. This link is provided by observations that BBS1 binds Rabin8, an interaction enhanced by Rab11.Citation133,Citation135 The BBSome-Rab8/Rab11 interactions could thus integrate ciliary transport of selected cargos, such as GPCRs, into a general delivery mechanism for ciliary membrane components, mediated by the Rab11-Rabin8-Rab8 pathway.
Curiously, BBS1, 2, and 4 also bind Exo70, which is one of the two exocyst proteins that interact directly with the cell membrane by binding negatively charged phosphoinositides and so are likely to play a role in the recognition of the target membrane.Citation161,Citation162 This is reminiscent of BBS3 interactions with membrane phosphoinositides, which were proposed to mediate the formation of BBS vesicle coats.Citation8 Importantly, Exo70 appears to provide positional information in polarized exocytosis (reviewed in refs. Citation163 and Citation164). This suggests a speculative hypothesis that perhaps a complex of BBS proteins and exocyst components defines the site of cargo delivery during cilia formation.
Tubby and IFT Proteins at the Gate to the Ciliary Compartment
Another group of genes with a role in trafficking to the cilium is the Tubby family, which consists of Tubby and several Tubby-like (TULP) genes (reviewed in ref. Citation165) (). These proteins are not directly related to the Rab8/Rab11 pathway, but nonetheless display functional similarities to BBS proteins. Similar to BBS mutations, loss of Tubby family genes does not cause pronounced structural cilia defects but impairs ciliary localization of selected GPCRs, which include opsins, SSTR3, MCHR1, Gpr161 and NPY.Citation118,Citation120,Citation166,Citation167 Mutations in the human TULP1 gene cause retinal degeneration, which is consistent with a role in opsin trafficking.Citation168 The Tubby family proteins share a conserved C-terminal region that folds into a 12 stranded β-barrel and interacts with phosphoinositides.Citation169 N-terminal regions, on the other hand, are variable and thought to play diverse roles. The N-terminus of Tubby-like 3 (TULP3), for example, binds IFT complex A polypeptides.Citation6 Based on the TULP3 requirement for GPCR transport and its potential involvement in dual binding interactions with membrane phosphoinositides and IFT polypeptides, TULP3 has been proposed to link periciliary vesicles (i.e., vesicles that accumulate at the cilia base and are likely to transport GPCRs) with cytoplasmic IFT A protein complexes. This could bring GPCR-carrying vesicles into the proximity of transition fibers, the transition zone and other ciliary gating mechanisms.
Many questions regarding the function of Tubby family genes remain to be answered. For example, it is not clear why their role is selective for only some GPCRs and Smoothened is not affected by the loss of TULP3.Citation6 Given their interactions with phosphoinositides, Tubby proteins could contribute to vesicle coat formation. This idea is supported by genetic data from vertebrates and nematodes alike, which suggests that Tubby-family and BBS genes play related roles.Citation118,Citation170 One indication of this relatedness is that both BBS and Tubby-family gene mutants share obesity as a phenotype.Citation154,Citation171-Citation173 This phenotype correlates with the role of TULP3 and BBS18/BBIP10 in the ciliary targeting of a Neuropeptide Y receptor, NPY2R.Citation118 Molecular underpinnings of this functional relatedness remain obscure.
IFT complex A function at the cilia base
A set of experiments has suggested that IFT complex A functions in concert with Tubby proteins. First, TAP-tag co-purification analysis revealed that TULP3 binds IFT complex A components.Citation6 Furthermore, functional studies showed that depletion of three IFT complex A proteins, Ift122, Ift140 and Ift144, does not cause ciliogenesis defects but decreases the ciliary content of TULP3.Citation6,Citation166 Finally, both Tubby and IFT complex A proteins are necessary for the ciliary entry of GPCRs.Citation6 In agreement with this, we (Avidor-Reiss lab) also observed that, in fly oseg1/ift122 mutants, the CNG channel is no longer transported into the cilium and accumulates at its base (). Taken together, these observations indicate that the role of IFT complex A in ciliary compartment-directed transport extends to a fairly broad spectrum of cargos.
Figure 4. Oseg1/ IFT122 function in ciliogenesis. An IFT Complex A subunit, Oseg1/ IFT122, is essential for CNG channel transport into the sensory cilium. (A) In control Drosophila larvae, the sensory neurons that innervate olfactory organs (autofluorescent in orange) express CNG-Channel-GFP in both the inner (IS) and outer segment (OS). (B) In contrast to that, in oseg1/ift122 mutant larvae, the CNG-Chanel-GFP is only found in the inner segment. (C) This is a specific defect in CNG transport as Tubulin-GFP is found in both the inner and the outer segment of oseg1 mutants. Avidor-Reiss Lab, unpublished results.
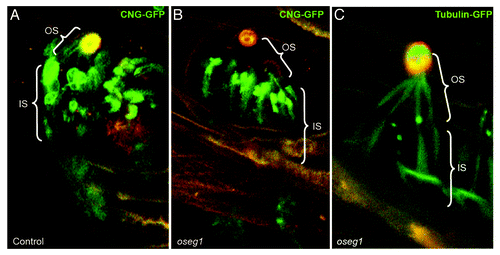
In agreement with the idea that the function of IFT complex A proteins extends beyond intraflagellar transport, IFT complex A and Tubby mutants are phenotypically similar to one another but differ from mutants in the intraflagellar dynein, DYNC2H1 (reviewed in refs. Citation6 and Citation174). These results led to the idea that three IFT complex A “core” proteins function at the base of the cilium to facilitate ciliary access of certain proteins.Citation6 The molecular basis of this ciliary access mechanism remains unknown. It could enhance the fusion of cytoplasmic vesicles. Alternatively, IFT complex A-vesicle interactions at the cilia base could initiate the loading of vesicle cargo, GPCRs and CNG channels, for example, onto the axonemal IFT particle trains, which also include IFT complex A proteins.
Some information has also become available on the mechanisms that drive the translocation of IFT complex A proteins toward the cilium. Proteomic experiments revealed that Ift140 physically interacts with cytoplasmic dynein, DYNC1H1, which led to the idea that this motor may transport complex A proteins to the cilia base.Citation6 Another player in the cytoplasmic transport of IFT proteins may be a planar cell polarity effector gene, fuzzy. Interference with fuzzy function causes loss of Ift43 from the basal body area.Citation175 Whether fuzzy is also required for the targeting of other IFT complex A proteins remains to be investigated. The Fuzzy protein does not seem to function in transporting the complex B component, IFT20.Citation175 As a side note, Fuzzy appears to play multiple roles in ciliogenesis because it has also been recently reported to recruit Rab8-positive vesicles to the basal body.Citation176 Although data from several studies have yet to be assembled into a coherent model, evidence is accumulating that IFT complex A proteins function in the ciliary centriole area to mediate transport into the ciliary compartment.
IFT20 in Transport from Cytoplasm to Cilia
In addition to IFT complex A proteins, a complex B component, Ift20, also appears to facilitate cilia-directed trafficking in the cytoplasm. This has been suggested by the observation that a mild knockdown of Ift20 does not affect ciliary length but does decrease the ciliary content of Pkd2, a ciliary transmembrane protein.Citation177 The Ift20 protein has also been found to localize to the Golgi apparatus, which led to suggestions that it may play a role in Golgi to cilium transport. This function does not appear to extend to all IFT complex B constituents because Ift88 knockdown does not produce a similar effect.Citation177
Consistent with the above experiments, two Golgi-associated proteins, Golgin and CCDC41, have been shown to be functionally related to Ift20. When Golgin is mutated in mouse cells, it causes loss of Ift20 from the Golgi apparatus, cilia shortening and a partial reduction of ciliary Pkd2 expression.Citation178 These observations suggest that Golgin and Ift20 function in the same pathway, to localize Pkd2 to cilia. The second protein, CCDC41, is necessary for Ift20 localization to the ciliary centriole but not to the Golgi apparatus.Citation48 The role of CCDC41 in Pkd2 targeting has not been investigated.
Lateral Transport into the Cilium from the Plasma Membrane
Although most models of ciliary targeting mechanisms invoke vesicle delivery from cytoplasmic pools located at the cilia base, an important alternative involves translocation of transmembrane proteins from the plasma membrane that surrounds the cilium. This mechanism has been best characterized in the case of Smoothened (Smo), a hedgehog cascade component that rapidly translocates to the cilium following hedgehog pathway stimulation recently reviewed in reference Citation179. Sequential labeling of SNAP-tagged Smo with fluorescent and non-fluorescent SNAP substrates made it possible to distinguish membrane and intracellular pools of Smo and led to the conclusion that Smo rapidly translocates into the ciliary membrane from the surrounding plasmalemma.Citation9 The contribution of Smo from the plasma membrane is pronounced during the first hour after hedgehog pathway stimulation. Beyond this, the intracellular pools become the principal source of ciliary Smo. Transport from the plasma membrane to the cilium has been also reported in Chlamydomonas following earlier studies of agglutinins.Citation180 Little is known, however, about its importance in other contexts. It is possible, but remains to be evaluated, that other transmembrane receptors, such as GPCRs, are also transported to cilia from the plasma membrane.
Transport of Cytosolic Proteins
The majority of structural and motility-related ciliary proteins, such as tubulin, radial spoke components and dynein arms, are soluble in the cell’s cytoplasm and thus do not require vesicle transport for their delivery to the ciliary compartment.Citation4,Citation99,Citation181 Whether an active transport mechanism is involved is currently unclear. As discussed above, the transport of cytoplasm-soluble proteins into the cilium appears to be restricted by mechanisms that limit diffusion. The nature of diffusion barriers that limit the movement of cytoplasmic proteins appears to be very different from these that restrict transmembrane proteins.
Is the base of the cilium similar to the nuclear pore?
It has been noted that some ciliary proteins, such as the Kif17 kinesin, which is the best studied in this regard, feature sequences related to nuclear localization signals ().Citation63 This observation led to the intriguing idea that the base of the cilium, also referred to as the ciliary pore, functions in a similar manner to the nuclear pore. In the context of the cell nucleus, nuclear import signals of cargo polypeptides interact with Importins, which are proteins that mediate nuclear import (reviewed in ref. Citation182). Importins bind cargo polypeptides in the cytoplasm and translocate them through the nuclear pore into the nucleus. Inside the nucleus, a Ras superfamily GTPase, Ran, binds Importins to induce a conformational change that leads to cargo release. The function of Ran in the nucleus is potentiated by a GEF, RCC1, which contributes to the high intranuclear concentration of Ran-GTP. In the cytoplasm, Ran is inactivated by interactions with GAP proteins, RanGAP1, RanBP1, and RanBP2, which results in a low level of GTP-bound Ran.Citation182
Several studies have provided evidence that many components of the nuclear import system function in ciliary targeting. First, deletion of nuclear localization signal-related sequences from Kif17 impairs its transport into the ciliary compartment.Citation63 Second, consistent with the nuclear import model and the cilioplasm behaving like the nucleoplasm, overexpression of GTP-locked Ran in the cytoplasm also impairs ciliary localization of Kif17, presumably as a result of premature Importin-cargo dissociation prior to ciliary import. In agreement with this, Kif17 binds Importin β2, an interaction that is also dependent on the presence of CLS and is disrupted by GTP-locked Ran.Citation63 Another study has also revealed that RanBP1 (a protein that stimulates Ran GAP) localizes to the ciliary centriole, and the cilium itself, and its knockdown promotes ciliogenesis.Citation183 Although this observation can be interpreted in several ways, it also supports the idea that nuclear pore-related pathways function at the cilia base. Finally, nuclear pore components, nucleoporins, including NUP37, NUP35, NUP62, NUP93, NUP133, and NUP214, are found in cilia, where they co-localize with a transition zone protein, CEP290.Citation10 This raises an intriguing possibility that nuclear pore proteins are integrated into the structure of the transition zone.
Kif17 is not the only protein that requires Importin for its ciliary localization. An Arl3 GAP, RP2, also binds Importin β2 and its ciliary localization is impaired in Importin-deficient cells.Citation183 Importin β2 knockdown appears, however, to have a limited impact on cilia size and morphology, which suggests that this pathway regulates only a subset of cargos.Citation183 It is not clear whether the transport of major ciliary structural elements, such as tubulin, radial spokes or dynein arms, also requires nuclear pore-related transport machinery. If so, it most likely relies on alternative Importins. As the ciliary proteome contains numerous cytosol-soluble proteins, one has to wonder what fraction of these polypeptides relies on a system related to nuclear import for their ciliary localization.
Delivery of lipidated proteins from cytoplasm to cilia
A unique transport mechanism exists for a group of lipidated proteins that are targeted to cilia. Despite hydrophobic characteristics of their lipid tails, these proteins are thought to be solubilized in the cytoplasm by binding to another polypeptide. Lipid-modifications are fairly common in ciliary proteinsCitation110,Citation184 and lipid attachment sites are frequently associated with CTSs (). A specialized mechanism exists that renders at least some of N-myristoylated ciliary proteins soluble in the cytoplasm. These proteins are thus likely to be subject to transport barrier mechanisms that are typical of cytoplasmic, but not transmembrane, polypeptides.
The key player in the transport of N-myristoylated ciliary proteins is UNC119, a protein that is structurally related to Guanine Nucleotide Dissociation Inhibitors (GDIs), which maintain prenylated small GTPases in a soluble form (reviewed recently in ref. Citation185). The UNC119 proteins have been shown to bind a Nephrocystin, NPHP3, and Gα subunits of several heterotrimeric ciliary G-proteins.Citation186,Citation187 It has been proposed that UNC119 interactions with NPHP3 and a ciliary Transducin solubilize these polypeptides in the cytoplasm by providing a hydrophobic environment for their acyl tails. The UNC119-solublized Transducin is thought to translocate into the photoreceptor cilium via diffusion.Citation186 Whether diffusion also drives the transport of UNC119 complexes into cilia of other cells is unclear. In the cilioplasm of RPE cells, UNC119b interacts with GTP-bound Arl3, a small GTPase, which is thought to dissociate UNC119b from NPHP3 and release NHPH3 into the ciliary membrane.Citation187 Following this, the Arl3-UNC119 complex is, in turn, dissociated by an interaction with RP2, which functions as an Arl3 GAP.Citation187
Does UNC119 target N-myristoylated proteins to cilia or act solely as a solubilizing factor? The N-terminal myristoylation motif of NPHP3 is necessary for its ciliary localization.Citation188 This sequence is, however, insufficient for ciliary targeting, which indicates that additional signals in the NPHP3 polypeptide contribute to ciliary localization.Citation188 An additional layer of complexity in the ciliary targeting of NPHP3 has been revealed by observations that an N-terminal coiled-coil domain of NPHP3, which does not include the myristoylation site, is both necessary and sufficient for targeting to the ciliary centriole. This observation led to the idea that the ciliary targeting of NPHP3 is a two-step process. The first step is driven by the N-terminal CTS but does not require myristoylation, which is required only in the second step.Citation188
UNC119 myristoyl-binding mutant does not localize to cilia, which suggests that UNC119 ciliary localization requires interaction with NPHP3.Citation187 The most plausible interpretation of these results is that UNC119 does not provide a CTS for ciliary targeting and serves as a solubilization factor that facilitates the transport of myristoylated proteins across the transition zone. In photoreceptor cells, it may also function as a solubilization factor during cytoplasmic transport of the ciliary Transducin.Citation186 Finally, NPHP3 transport seems to proceed independently from the ciliary pore Ran/Importin system.Citation188 Thus, lipidated proteins appear to follow their own unique traffic rules during cilia-directed transport.
Protein-protein interaction tests have revealed that UNC119 binds the N-termini of many proteins, which include Src-type tyrosine kinases and small Arf-like GTPases (reviewed in ref. Citation184). This suggests that the role of UNC119 in cilia-directed trafficking may extend beyond the transport of NPHP3 and G-proteins that has been characterized so far. Genetic analysis of this issue in vertebrates has so far been confounded by potential functional redundancy of UNC119 genes (two are found in vertebrate genomes).Citation186 A role for UNC119 in the transport of diverse cargos is also suggested by the observation that it regulates Lck kinase localization during immunological synapse formation, a process related to ciliogenesis.Citation189,Citation190
In contrast to the myristoylated proteins discussed above, the CaaX prenylation motif found in a ciliary phosphatidylinositol phosphatase, INPP5E, is not necessary for ciliary targeting.Citation110 The CTS of INPP5E is well characterized and does not include the CaaX motif. It mediates direct interactions with a small GTPase, Arl13b, which localizes to the ciliary membrane.Citation110 Surprisingly, Arl13b binding to the CTS of INPP5E releases its interaction with PDE6D, a prenyl-binding protein. It is not clear how the lipid tail of INPP5E is shielded during cytoplasmic transport. Perhaps as yet unidentified factors interact with lipid tails of prenylated proteins during their transport to cilia.
Some Closing Remarks
The targeting of proteins to cilia is of fundamental importance for the formation and function of these fascinating cell surface structures. Given the diversity of signaling events that are mediated by cilia and the corresponding variety of signal transduction components that localize to the cilioplasm and the ciliary membrane, it is not surprising that the transport pathways that deliver ciliary proteins are also diverse. Separate pathways appear to handle the transport of transmembrane, membrane-associated and cytoplasmic proteins and, even within each of the above three categories, the transport of structurally disparate proteins is likely to involve somewhat different mechanisms. Although this has not been investigated in sufficient detail thus far, ciliary transport mechanisms are likely to modulate ciliary signal transduction by regulating, for example, interactions between transport machinery and essential signaling proteins, such as GPCRs.
Ciliary transport pathways have to be closely integrated with the function of diffusion barriers that operate at the boundary of the ciliary compartment. Such barriers affect the movement of both transmembrane and cytoplasmic proteins, although transport of the latter appears to be less stringently controlled. The diversity of ciliary transport pathways and their cargos is most likely paralleled by similarly diverse ciliary gating mechanisms. It is usually assumed that transition zones are largely the same in all cilia. This view is most likely incorrect, as cilia of many tissues are likely to feature specialized gating mechanisms to accommodate similarly specialized ciliary proteins.
The transport of even fairly generic ciliary protein complexes poses some difficult questions as it remains unclear how large protein conglomerates, the IFT particle for example, navigate through gating mechanisms at cilia base. Such large complexes may induce conformational changes in transition zone components. Visualizing such changes is technically difficult but perhaps can be achieved with the help of recent advances in microscopy.Citation191
Efforts to purposely manipulate ciliary transport to achieve practical benefits have not been undertaken to any significant degree so far. Along with an increased understanding of ciliary transport pathways, it will become possible to manipulate cilia function by designing artificial ciliary cargo molecules. Such cargo could be used to modify ciliary microtubules and thereby affect cilia stability or to change cilia responsiveness to ligands by engineering ciliary receptors. Engineered cilia could be used to manipulate cell proliferation or to direct cell migration. Cells with engineered cilia could be used for therapeutic purposes to stimulate tissue repair in many organs, such as lungs, oviducts, brain and the spinal canal. Such goals are relatively far off at this time but may become accessible in the long run. In the shorter term, many questions regarding the function of transport mechanisms and ciliary diffusion barriers remain to be solved. We need to address these questions before we wish cilia-directed cargo proteins bon voyage.
Disclosure of Potential Conflicts of Interest
No potential conflicts of interest were disclosed.
Acknowledgments
The authors are thankful to Prof Elizabeth Smythe for insightful comments on earlier versions of this work and to Emily Lillian Fishman for drawing . J.M. is supported, in part, by an R01 award R01EY018176 from the National Eye Institute, NIH, USA. T.A.R. is supported, in part, by award R01GM098394 from the National Institute of General Medical Sciences and NSF award 1121176.
References
- Emmer BT, Maric D, Engman DM. Molecular mechanisms of protein and lipid targeting to ciliary membranes. J Cell Sci 2010; 123:529 - 36; http://dx.doi.org/10.1242/jcs.062968; PMID: 20145001
- Sung C-H, Leroux MR. The roles of evolutionarily conserved functional modules in cilia-related trafficking. Nat Cell Biol 2013; 15:1387 - 97; http://dx.doi.org/10.1038/ncb2888; PMID: 24296415
- Wang J, Deretic D. Molecular complexes that direct rhodopsin transport to primary cilia. Prog Retin Eye Res 2014; 38:1 - 19; http://dx.doi.org/10.1016/j.preteyeres.2013.08.004; PMID: 24135424
- Bhogaraju S, Cajanek L, Fort C, Blisnick T, Weber K, Taschner M, Mizuno N, Lamla S, Bastin P, Nigg EA, et al. Molecular basis of tubulin transport within the cilium by IFT74 and IFT81. Science 2013; 341:1009 - 12; http://dx.doi.org/10.1126/science.1240985; PMID: 23990561
- Wang J, Morita Y, Mazelova J, Deretic D. The Arf GAP ASAP1 provides a platform to regulate Arf4- and Rab11-Rab8-mediated ciliary receptor targeting. EMBO J 2012; 31:4057 - 71; http://dx.doi.org/10.1038/emboj.2012.253; PMID: 22983554
- Mukhopadhyay S, Wen X, Chih B, Nelson CD, Lane WS, Scales SJ, Jackson PK. TULP3 bridges the IFT-A complex and membrane phosphoinositides to promote trafficking of G protein-coupled receptors into primary cilia. Genes Dev 2010; 24:2180 - 93; http://dx.doi.org/10.1101/gad.1966210; PMID: 20889716
- Tai AW, Chuang JZ, Bode C, Wolfrum U, Sung CH. Rhodopsin’s carboxy-terminal cytoplasmic tail acts as a membrane receptor for cytoplasmic dynein by binding to the dynein light chain Tctex-1. Cell 1999; 97:877 - 87; http://dx.doi.org/10.1016/S0092-8674(00)80800-4; PMID: 10399916
- Jin H, White SR, Shida T, Schulz S, Aguiar M, Gygi SP, Bazan JF, Nachury MV. The conserved Bardet-Biedl syndrome proteins assemble a coat that traffics membrane proteins to cilia. Cell 2010; 141:1208 - 19; http://dx.doi.org/10.1016/j.cell.2010.05.015; PMID: 20603001
- Milenkovic L, Scott MP, Rohatgi R. Lateral transport of Smoothened from the plasma membrane to the membrane of the cilium. J Cell Biol 2009; 187:365 - 74; http://dx.doi.org/10.1083/jcb.200907126; PMID: 19948480
- Kee HL, Dishinger JF, Blasius TL, Liu CJ, Margolis B, Verhey KJ. A size-exclusion permeability barrier and nucleoporins characterize a ciliary pore complex that regulates transport into cilia. Nat Cell Biol 2012; 14:431 - 7; http://dx.doi.org/10.1038/ncb2450; PMID: 22388888
- Hao L, Thein M, Brust-Mascher I, Civelekoglu-Scholey G, Lu Y, Acar S, Prevo B, Shaham S, Scholey JM. Intraflagellar transport delivers tubulin isotypes to sensory cilium middle and distal segments. Nat Cell Biol 2011; 13:790 - 8; http://dx.doi.org/10.1038/ncb2268; PMID: 21642982
- Qin H, Burnette DT, Bae YK, Forscher P, Barr MM, Rosenbaum JL. Intraflagellar transport is required for the vectorial movement of TRPV channels in the ciliary membrane. Curr Biol 2005; 15:1695 - 9; http://dx.doi.org/10.1016/j.cub.2005.08.047; PMID: 16169494
- Kozminski KG, Beech PL, Rosenbaum JL. The Chlamydomonas kinesin-like protein FLA10 is involved in motility associated with the flagellar membrane. J Cell Biol 1995; 131:1517 - 27; http://dx.doi.org/10.1083/jcb.131.6.1517; PMID: 8522608
- Pigino G, Geimer S, Lanzavecchia S, Paccagnini E, Cantele F, Diener DR, Rosenbaum JL, Lupetti P. Electron-tomographic analysis of intraflagellar transport particle trains in situ. J Cell Biol 2009; 187:135 - 48; http://dx.doi.org/10.1083/jcb.200905103; PMID: 19805633
- Tsujikawa M, Malicki J. Intraflagellar transport genes are essential for differentiation and survival of vertebrate sensory neurons. Neuron 2004; 42:703 - 16; http://dx.doi.org/10.1016/S0896-6273(04)00268-5; PMID: 15182712
- Murcia NS, Richards WG, Yoder BK, Mucenski ML, Dunlap JR, Woychik RP. The Oak Ridge Polycystic Kidney (orpk) disease gene is required for left-right axis determination. Development 2000; 127:2347 - 55; PMID: 10804177
- Qin H, Rosenbaum JL, Barr MM. An autosomal recessive polycystic kidney disease gene homolog is involved in intraflagellar transport in C. elegans ciliated sensory neurons. Curr Biol 2001; 11:457 - 61; http://dx.doi.org/10.1016/S0960-9822(01)00122-1; PMID: 11301258
- Brazelton WJ, Amundsen CD, Silflow CD, Lefebvre PA. The bld1 mutation identifies the Chlamydomonas osm-6 homolog as a gene required for flagellar assembly. Curr Biol 2001; 11:1591 - 4; http://dx.doi.org/10.1016/S0960-9822(01)00485-7; PMID: 11676919
- Scholey JM. Kinesin-2: a family of heterotrimeric and homodimeric motors with diverse intracellular transport functions. Annu Rev Cell Dev Biol 2013; 29:443 - 69; http://dx.doi.org/10.1146/annurev-cellbio-101512-122335; PMID: 23750925
- Mogensen MM, Malik A, Piel M, Bouckson-Castaing V, Bornens M. Microtubule minus-end anchorage at centrosomal and non-centrosomal sites: the role of ninein. J Cell Sci 2000; 113:3013 - 23; PMID: 10934040
- Bergen LG, Kuriyama R, Borisy GG. Polarity of microtubules nucleated by centrosomes and chromosomes of Chinese hamster ovary cells in vitro. J Cell Biol 1980; 84:151 - 9; http://dx.doi.org/10.1083/jcb.84.1.151; PMID: 7350167
- Troutt LL, Burnside B. Microtubule polarity and distribution in teleost photoreceptors. J Neurosci 1988; 8:2371 - 80; PMID: 3249231
- Hu Q, Milenkovic L, Jin H, Scott MP, Nachury MV, Spiliotis ET, Nelson WJ. A septin diffusion barrier at the base of the primary cilium maintains ciliary membrane protein distribution. Science 2010; 329:436 - 9; http://dx.doi.org/10.1126/science.1191054; PMID: 20558667
- Chih B, Liu P, Chinn Y, Chalouni C, Komuves LG, Hass PE, Sandoval W, Peterson AS. A ciliopathy complex at the transition zone protects the cilia as a privileged membrane domain. Nat Cell Biol 2012; 14:61 - 72; http://dx.doi.org/10.1038/ncb2410; PMID: 22179047
- Williams CL, Li C, Kida K, Inglis PN, Mohan S, Semenec L, Bialas NJ, Stupay RM, Chen N, Blacque OE, et al. MKS and NPHP modules cooperate to establish basal body/transition zone membrane associations and ciliary gate function during ciliogenesis. J Cell Biol 2011; 192:1023 - 41; http://dx.doi.org/10.1083/jcb.201012116; PMID: 21422230
- Chang B, Khanna H, Hawes N, Jimeno D, He S, Lillo C, Parapuram SK, Cheng H, Scott A, Hurd RE, et al. In-frame deletion in a novel centrosomal/ciliary protein CEP290/NPHP6 perturbs its interaction with RPGR and results in early-onset retinal degeneration in the rd16 mouse. Hum Mol Genet 2006; 15:1847 - 57; http://dx.doi.org/10.1093/hmg/ddl107; PMID: 16632484
- Won J, Marín de Evsikova C, Smith RS, Hicks WL, Edwards MM, Longo-Guess C, Li T, Naggert JK, Nishina PM. NPHP4 is necessary for normal photoreceptor ribbon synapse maintenance and outer segment formation, and for sperm development. Hum Mol Genet 2011; 20:482 - 96; http://dx.doi.org/10.1093/hmg/ddq494; PMID: 21078623
- Tiwari S, Hudson S, Gattone VH 2nd, Miller C, Chernoff EAG, Belecky-Adams TL. Meckelin 3 is necessary for photoreceptor outer segment development in rat Meckel syndrome. PLoS One 2013; 8:e59306; http://dx.doi.org/10.1371/journal.pone.0059306; PMID: 23516626
- Molla-Herman A, Ghossoub R, Blisnick T, Meunier A, Serres C, Silbermann F, Emmerson C, Romeo K, Bourdoncle P, Schmitt A, et al. The ciliary pocket: an endocytic membrane domain at the base of primary and motile cilia. J Cell Sci 2010; 123:1785 - 95; http://dx.doi.org/10.1242/jcs.059519; PMID: 20427320
- Sorokin S. Centrioles and the formation of rudimentary cilia by fibroblasts and smooth muscle cells. J Cell Biol 1962; 15:363 - 77; http://dx.doi.org/10.1083/jcb.15.2.363; PMID: 13978319
- Mostowy S, Cossart P. Septins: the fourth component of the cytoskeleton. Nat Rev Mol Cell Biol 2012; 13:183 - 94; PMID: 22314400
- Fliegauf M, Kahle A, Häffner K, Zieger B. Distinct localization of septin proteins to ciliary sub-compartments in airway epithelial cells. Biol Chem 2014; 395:151 - 6; http://dx.doi.org/10.1515/hsz-2013-0252; PMID: 24317785
- Ihara M, Kinoshita A, Yamada S, Tanaka H, Tanigaki A, Kitano A, Goto M, Okubo K, Nishiyama H, Ogawa O, et al. Cortical organization by the septin cytoskeleton is essential for structural and mechanical integrity of mammalian spermatozoa. Dev Cell 2005; 8:343 - 52; http://dx.doi.org/10.1016/j.devcel.2004.12.005; PMID: 15737930
- Kissel H, Georgescu MM, Larisch S, Manova K, Hunnicutt GR, Steller H. The Sept4 septin locus is required for sperm terminal differentiation in mice. Dev Cell 2005; 8:353 - 64; http://dx.doi.org/10.1016/j.devcel.2005.01.021; PMID: 15737931
- Buckland-Nicks JA, Chia FS. Spermatogenesis of a marine snail, Littorina sitkana. Cell Tissue Res 1976; 170:455 - 75; http://dx.doi.org/10.1007/BF00361704; PMID: 963725
- Benmerah A. The ciliary pocket. Curr Opin Cell Biol 2013; 25:78 - 84; http://dx.doi.org/10.1016/j.ceb.2012.10.011; PMID: 23153502
- Kim SK, Shindo A, Park TJ, Oh EC, Ghosh S, Gray RS, Lewis RA, Johnson CA, Attie-Bittach T, Katsanis N, et al. Planar cell polarity acts through septins to control collective cell movement and ciliogenesis. Science 2010; 329:1337 - 40; http://dx.doi.org/10.1126/science.1191184; PMID: 20671153
- Deane JA, Cole DG, Seeley ES, Diener DR, Rosenbaum JL. Localization of intraflagellar transport protein IFT52 identifies basal body transitional fibers as the docking site for IFT particles. Curr Biol 2001; 11:1586 - 90; http://dx.doi.org/10.1016/S0960-9822(01)00484-5; PMID: 11676918
- Wei Q, Xu Q, Zhang Y, Li Y, Zhang Q, Hu Z, Harris PC, Torres VE, Ling K, Hu J. Transition fibre protein FBF1 is required for the ciliary entry of assembled intraflagellar transport complexes. Nat Commun 2013; 4:2750; http://dx.doi.org/10.1038/ncomms3750; PMID: 24231678
- Reiter JF, Blacque OE, Leroux MR. The base of the cilium: roles for transition fibres and the transition zone in ciliary formation, maintenance and compartmentalization. EMBO Rep 2012; 13:608 - 18; http://dx.doi.org/10.1038/embor.2012.73; PMID: 22653444
- Vieira OV, Gaus K, Verkade P, Fullekrug J, Vaz WLC, Simons K. FAPP2, cilium formation, and compartmentalization of the apical membrane in polarized Madin-Darby canine kidney (MDCK) cells. Proc Natl Acad Sci U S A 2006; 103:18556 - 61; http://dx.doi.org/10.1073/pnas.0608291103; PMID: 17116893
- Tateishi K, Yamazaki Y, Nishida T, Watanabe S, Kunimoto K, Ishikawa H, Tsukita S. Two appendages homologous between basal bodies and centrioles are formed using distinct Odf2 domains. J Cell Biol 2013; 203:417 - 25; http://dx.doi.org/10.1083/jcb.201303071; PMID: 24189274
- Tanos BE, Yang HJ, Soni R, Wang WJ, Macaluso FP, Asara JM, Tsou MF. Centriole distal appendages promote membrane docking, leading to cilia initiation. Genes Dev 2013; 27:163 - 8; http://dx.doi.org/10.1101/gad.207043.112; PMID: 23348840
- Ishikawa H, Kubo A, Tsukita S, Tsukita S. Odf2-deficient mother centrioles lack distal/subdistal appendages and the ability to generate primary cilia. Nat Cell Biol 2005; 7:517 - 24; http://dx.doi.org/10.1038/ncb1251; PMID: 15852003
- Schmidt KN, Kuhns S, Neuner A, Hub B, Zentgraf H, Pereira G. Cep164 mediates vesicular docking to the mother centriole during early steps of ciliogenesis. J Cell Biol 2012; 199:1083 - 101; http://dx.doi.org/10.1083/jcb.201202126; PMID: 23253480
- Avasthi P, Marshall WF. Stages of ciliogenesis and regulation of ciliary length. Differentiation 2012; 83:S30 - 42; http://dx.doi.org/10.1016/j.diff.2011.11.015; PMID: 22178116
- Ye X, Zeng H, Ning G, Reiter JF, Liu A. C2cd3 is critical for centriolar distal appendage assembly and ciliary vesicle docking in mammals. Proc Natl Acad Sci U S A 2014; 111:2164 - 9; http://dx.doi.org/10.1073/pnas.1318737111; PMID: 24469809
- Joo K, Kim CG, Lee M-S, Moon H-Y, Lee S-H, Kim MJ, Kweon H-S, Park W-Y, Kim C-H, Gleeson JG, et al. CCDC41 is required for ciliary vesicle docking to the mother centriole. Proc Natl Acad Sci U S A 2013; 110:5987 - 92; http://dx.doi.org/10.1073/pnas.1220927110; PMID: 23530209
- Dentler WL. Microtubule-membrane interactions in cilia and flagella. Int Rev Cytol 1981; 72:1 - 47; http://dx.doi.org/10.1016/S0074-7696(08)61193-6; PMID: 7019129
- Besharse JC, Horst CJ. The photoreceptor connecting cilium A model for the transition zone. Ciliary and flagellar membranes: Springer, 1990:389-417.
- Craige B, Tsao CC, Diener DR, Hou Y, Lechtreck KF, Rosenbaum JL, Witman GB. CEP290 tethers flagellar transition zone microtubules to the membrane and regulates flagellar protein content. J Cell Biol 2010; 190:927 - 40; http://dx.doi.org/10.1083/jcb.201006105; PMID: 20819941
- Garcia-Gonzalo FR, Corbit KC, Sirerol-Piquer MS, Ramaswami G, Otto EA, Noriega TR, Seol AD, Robinson JF, Bennett CL, Josifova DJ, et al. A transition zone complex regulates mammalian ciliogenesis and ciliary membrane composition. Nat Genet 2011; 43:776 - 84; http://dx.doi.org/10.1038/ng.891; PMID: 21725307
- Nalefski EA, Wisner MA, Chen JZ, Sprang SR, Fukuda M, Mikoshiba K, Falke JJ. C2 domains from different Ca2+ signaling pathways display functional and mechanistic diversity. Biochemistry 2001; 40:3089 - 100; http://dx.doi.org/10.1021/bi001968a; PMID: 11258923
- Wang W-J, Tay HG, Soni R, Perumal GS, Goll MG, Macaluso FP, Asara JM, Amack JD, Tsou M-FB. CEP162 is an axoneme-recognition protein promoting ciliary transition zone assembly at the cilia base. Nat Cell Biol 2013; 15:591 - 601; http://dx.doi.org/10.1038/ncb2739; PMID: 23644468
- Drivas TG, Holzbaur ELF, Bennett J. Disruption of CEP290 microtubule/membrane-binding domains causes retinal degeneration. J Clin Invest 2013; 123:4525 - 39; http://dx.doi.org/10.1172/JCI69448; PMID: 24051377
- Sohara E, Luo Y, Zhang J, Manning DK, Beier DR, Zhou J. Nek8 regulates the expression and localization of polycystin-1 and polycystin-2. J Am Soc Nephrol 2008; 19:469 - 76; http://dx.doi.org/10.1681/ASN.2006090985; PMID: 18235101
- Frank V, Habbig S, Bartram MP, Eisenberger T, Veenstra-Knol HE, Decker C, Boorsma RAC, Göbel H, Nürnberg G, Griessmann A, et al. Mutations in NEK8 link multiple organ dysplasia with altered Hippo signalling and increased c-MYC expression. Hum Mol Genet 2013; 22:2177 - 85; http://dx.doi.org/10.1093/hmg/ddt070; PMID: 23418306
- Mahjoub MR, Montpetit B, Zhao L, Finst RJ, Goh B, Kim AC, Quarmby LM. The FA2 gene of Chlamydomonas encodes a NIMA family kinase with roles in cell cycle progression and microtubule severing during deflagellation. J Cell Sci 2002; 115:1759 - 68; PMID: 11950892
- Mahjoub MR, Qasim Rasi M, Quarmby LM. A NIMA-related kinase, Fa2p, localizes to a novel site in the proximal cilia of Chlamydomonas and mouse kidney cells. Mol Biol Cell 2004; 15:5172 - 86; http://dx.doi.org/10.1091/mbc.E04-07-0571; PMID: 15371535
- Spalluto C, Wilson DI, Hearn T. Nek2 localises to the distal portion of the mother centriole/basal body and is required for timely cilium disassembly at the G2/M transition. Eur J Cell Biol 2012; 91:675 - 86; http://dx.doi.org/10.1016/j.ejcb.2012.03.009; PMID: 22613497
- Seeger-Nukpezah T, Liebau MC, Höpker K, Lamkemeyer T, Benzing T, Golemis EA, Schermer B. The centrosomal kinase Plk1 localizes to the transition zone of primary cilia and induces phosphorylation of nephrocystin-1. PLoS One 2012; 7:e38838; http://dx.doi.org/10.1371/journal.pone.0038838; PMID: 22701722
- Cevik S, Hori Y, Kaplan OI, Kida K, Toivenon T, Foley-Fisher C, Cottell D, Katada T, Kontani K, Blacque OE. Joubert syndrome Arl13b functions at ciliary membranes and stabilizes protein transport in Caenorhabditis elegans. J Cell Biol 2010; 188:953 - 69; http://dx.doi.org/10.1083/jcb.200908133; PMID: 20231383
- Dishinger JF, Kee HL, Jenkins PM, Fan S, Hurd TW, Hammond JW, Truong YN-T, Margolis B, Martens JR, Verhey KJ. Ciliary entry of the kinesin-2 motor KIF17 is regulated by importin-β2 and RanGTP. Nat Cell Biol 2010; 12:703 - 10; http://dx.doi.org/10.1038/ncb2073; PMID: 20526328
- Fan Y, Esmail MA, Ansley SJ, Blacque OE, Boroevich K, Ross AJ, Moore SJ, Badano JL, May-Simera H, Compton DS, et al. Mutations in a member of the Ras superfamily of small GTP-binding proteins causes Bardet-Biedl syndrome. Nat Genet 2004; 36:989 - 93; http://dx.doi.org/10.1038/ng1414; PMID: 15314642
- Qin H. Regulation of intraflagellar transport and ciliogenesis by small G proteins. Int Rev Cell Mol Biol 2012; 293:149 - 68; http://dx.doi.org/10.1016/B978-0-12-394304-0.00010-5; PMID: 22251561
- Menotti-Raymond M, David VA, Schäffer AA, Stephens R, Wells D, Kumar-Singh R, O’Brien SJ, Narfström K. Mutation in CEP290 discovered for cat model of human retinal degeneration. J Hered 2007; 98:211 - 20; http://dx.doi.org/10.1093/jhered/esm019; PMID: 17507457
- Littink KW, Pott JW, Collin RW, Kroes HY, Verheij JB, Blokland EA, de Castro Miró M, Hoyng CB, Klaver CC, Koenekoop RK, et al. A novel nonsense mutation in CEP290 induces exon skipping and leads to a relatively mild retinal phenotype. Invest Ophthalmol Vis Sci 2010; 51:3646 - 52; http://dx.doi.org/10.1167/iovs.09-5074; PMID: 20130272
- Sayer JA, Otto EA, O’Toole JF, Nurnberg G, Kennedy MA, Becker C, Hennies HC, Helou J, Attanasio M, Fausett BV, et al. The centrosomal protein nephrocystin-6 is mutated in Joubert syndrome and activates transcription factor ATF4. Nat Genet 2006; 38:674 - 81; http://dx.doi.org/10.1038/ng1786; PMID: 16682973
- Vallespin E, Lopez-Martinez MA, Cantalapiedra D, Riveiro-Alvarez R, Aguirre-Lamban J, Avila-Fernandez A, Villaverde C, Trujillo-Tiebas MJ, Ayuso C. Frequency of CEP290 c.2991_1655A>G mutation in 175 Spanish families affected with Leber congenital amaurosis and early-onset retinitis pigmentosa. Mol Vis 2007; 13:2160 - 2; PMID: 18079693
- den Hollander AI, Koenekoop RK, Yzer S, Lopez I, Arends ML, Voesenek KE, Zonneveld MN, Strom TM, Meitinger T, Brunner HG, et al. Mutations in the CEP290 (NPHP6) gene are a frequent cause of Leber congenital amaurosis. Am J Hum Genet 2006; 79:556 - 61; http://dx.doi.org/10.1086/507318; PMID: 16909394
- Valente EM, Silhavy JL, Brancati F, Barrano G, Krishnaswami SR, Castori M, Lancaster MA, Boltshauser E, Boccone L, Al-Gazali L, et al, International Joubert Syndrome Related Disorders Study Group. Mutations in CEP290, which encodes a centrosomal protein, cause pleiotropic forms of Joubert syndrome. Nat Genet 2006; 38:623 - 5; http://dx.doi.org/10.1038/ng1805; PMID: 16682970
- Frank V, den Hollander AI, Brüchle NO, Zonneveld MN, Nürnberg G, Becker C, Du Bois G, Kendziorra H, Roosing S, Senderek J, et al. Mutations of the CEP290 gene encoding a centrosomal protein cause Meckel-Gruber syndrome. Hum Mutat 2008; 29:45 - 52; http://dx.doi.org/10.1002/humu.20614; PMID: 17705300
- Williams CL, Winkelbauer ME, Schafer JC, Michaud EJ, Yoder BK. Functional redundancy of the B9 proteins and nephrocystins in Caenorhabditis elegans ciliogenesis. Mol Biol Cell 2008; 19:2154 - 68; http://dx.doi.org/10.1091/mbc.E07-10-1070; PMID: 18337471
- Sang L, Miller JJ, Corbit KC, Giles RH, Brauer MJ, Otto EA, Baye LM, Wen X, Scales SJ, Kwong M, et al. Mapping the NPHP-JBTS-MKS protein network reveals ciliopathy disease genes and pathways. Cell 2011; 145:513 - 28; http://dx.doi.org/10.1016/j.cell.2011.04.019; PMID: 21565611
- Bialas NJ, Inglis PN, Li C, Robinson JF, Parker JD, Healey MP, Davis EE, Inglis CD, Toivonen T, Cottell DC, et al. Functional interactions between the ciliopathy-associated Meckel syndrome 1 (MKS1) protein and two novel MKS1-related (MKSR) proteins. J Cell Sci 2009; 122:611 - 24; http://dx.doi.org/10.1242/jcs.028621; PMID: 19208769
- Dowdle WE, Robinson JF, Kneist A, Sirerol-Piquer MS, Frints SG, Corbit KC, Zaghloul NA, van Lijnschoten G, Mulders L, Verver DE, et al. Disruption of a ciliary B9 protein complex causes Meckel syndrome. Am J Hum Genet 2011; 89:94 - 110; http://dx.doi.org/10.1016/j.ajhg.2011.06.003; PMID: 21763481
- Shiba D, Manning DK, Koga H, Beier DR, Yokoyama T. Inv acts as a molecular anchor for Nphp3 and Nek8 in the proximal segment of primary cilia. Cytoskeleton (Hoboken) 2010; 67:112 - 9; PMID: 20169535
- Shiba D, Yamaoka Y, Hagiwara H, Takamatsu T, Hamada H, Yokoyama T. Localization of Inv in a distinctive intraciliary compartment requires the C-terminal ninein-homolog-containing region. J Cell Sci 2009; 122:44 - 54; http://dx.doi.org/10.1242/jcs.037408; PMID: 19050042
- Hoff S, Halbritter J, Epting D, Frank V, Nguyen TM, van Reeuwijk J, Boehlke C, Schell C, Yasunaga T, Helmstädter M, et al. ANKS6 is a central component of a nephronophthisis module linking NEK8 to INVS and NPHP3. Nat Genet 2013; 45:951 - 6; http://dx.doi.org/10.1038/ng.2681; PMID: 23793029
- Li JB, Gerdes JM, Haycraft CJ, Fan Y, Teslovich TM, May-Simera H, Li H, Blacque OE, Li L, Leitch CC, et al. Comparative genomics identifies a flagellar and basal body proteome that includes the BBS5 human disease gene. Cell 2004; 117:541 - 52; http://dx.doi.org/10.1016/S0092-8674(04)00450-7; PMID: 15137946
- Avidor-Reiss T, Maer AM, Koundakjian E, Polyanovsky A, Keil T, Subramaniam S, Zuker CS. Decoding cilia function: defining specialized genes required for compartmentalized cilia biogenesis. Cell 2004; 117:527 - 39; http://dx.doi.org/10.1016/S0092-8674(04)00412-X; PMID: 15137945
- Cheng YZ, Eley L, Hynes AM, Overman LM, Simms RJ, Barker A, Dawe HR, Lindsay S, Sayer JA. Investigating embryonic expression patterns and evolution of AHI1 and CEP290 genes, implicated in Joubert syndrome. PLoS One 2012; 7:e44975; http://dx.doi.org/10.1371/journal.pone.0044975; PMID: 23028714
- Stowe TR, Wilkinson CJ, Iqbal A, Stearns T. The centriolar satellite proteins Cep72 and Cep290 interact and are required for recruitment of BBS proteins to the cilium. Mol Biol Cell 2012; 23:3322 - 35; http://dx.doi.org/10.1091/mbc.E12-02-0134; PMID: 22767577
- Kim J, Krishnaswami SR, Gleeson JG. CEP290 interacts with the centriolar satellite component PCM-1 and is required for Rab8 localization to the primary cilium. Hum Mol Genet 2008; 17:3796 - 805; http://dx.doi.org/10.1093/hmg/ddn277; PMID: 18772192
- Bornens M, Azimzadeh J. Origin and evolution of the centrosome. Adv Exp Med Biol 2007; 607:119 - 29; http://dx.doi.org/10.1007/978-0-387-74021-8_10; PMID: 17977464
- Silverman MA, Leroux MR. Intraflagellar transport and the generation of dynamic, structurally and functionally diverse cilia. Trends Cell Biol 2009; 19:306 - 16; http://dx.doi.org/10.1016/j.tcb.2009.04.002; PMID: 19560357
- Toure A, Rode B, Hunnicutt GR, Escalier D, Gacon G. Septins at the annulus of mammalian sperm. Biol Chem 2011; 392:799 - 803; http://dx.doi.org/10.1515/BC.2011.074; PMID: 21740329
- Keil TA. Functional morphology of insect mechanoreceptors. Microsc Res Tech 1997; 39:506 - 31; http://dx.doi.org/10.1002/(SICI)1097-0029(19971215)39:6<506::AID-JEMT5>3.0.CO;2-B; PMID: 9438251
- Liu X, Udovichenko IP, Brown SD, Steel KP, Williams DS. Myosin VIIa participates in opsin transport through the photoreceptor cilium. J Neurosci 1999; 19:6267 - 74; PMID: 10414956
- Szymanska K, Johnson CA. The transition zone: an essential functional compartment of cilia. Cilia 2012; 1:10; http://dx.doi.org/10.1186/2046-2530-1-10; PMID: 23352055
- Tates AD. Cytodifferentiation during Spermatogenesis in Drosophila melanogaster: An Electron Microscope Study. Rijksuniversiteit de Leiden. Leiden, Netherlands, 1971.
- Sinden RE, Canning EU, Spain B. Gametogenesis and fertilization in Plasmodium yoelii nigeriensis: a transmission electron microscope study. Proc R Soc Lond B Biol Sci 1976; 193:55 - 76; http://dx.doi.org/10.1098/rspb.1976.0031; PMID: 4810
- Fawcett DW, Eddy EM, Phillips DM. Observations on the fine structure and relationships of the chromatoid body in mammalian spermatogenesis. Biol Reprod 1970; 2:129 - 53; http://dx.doi.org/10.1095/biolreprod2.1.129; PMID: 4106274
- Tokuyasu KT. Dynamics of spermiogenesis in Drosophila melanogaster. V. Head-tail alignment. J Ultrastruct Res 1975; 50:117 - 29; http://dx.doi.org/10.1016/S0022-5320(75)90013-1; PMID: 803563
- Phillips DM. Insect sperm: their structure and morphogenesis. J Cell Biol 1970; 44:243 - 77; http://dx.doi.org/10.1083/jcb.44.2.243; PMID: 4903810
- Ma L, Jarman AP. Dilatory is a Drosophila protein related to AZI1 (CEP131) that is located at the ciliary base and required for cilium formation. J Cell Sci 2011; 124:2622 - 30; http://dx.doi.org/10.1242/jcs.084798; PMID: 21750193
- Enjolras C, Thomas J, Chhin B, Cortier E, Duteyrat JL, Soulavie F, Kernan MJ, Laurençon A, Durand B. Drosophila chibby is required for basal body formation and ciliogenesis but not for Wg signaling. J Cell Biol 2012; 197:313 - 25; http://dx.doi.org/10.1083/jcb.201109148; PMID: 22508513
- Baker JD, Adhikarakunnathu S, Kernan MJ. Mechanosensory-defective, male-sterile unc mutants identify a novel basal body protein required for ciliogenesis in Drosophila. Development 2004; 131:3411 - 22; http://dx.doi.org/10.1242/dev.01229; PMID: 15226257
- Qin H, Diener DR, Geimer S, Cole DG, Rosenbaum JL. Intraflagellar transport (IFT) cargo: IFT transports flagellar precursors to the tip and turnover products to the cell body. J Cell Biol 2004; 164:255 - 66; http://dx.doi.org/10.1083/jcb.200308132; PMID: 14718520
- Cevik S, Sanders AA, Van Wijk E, Boldt K, Clarke L, van Reeuwijk J, Hori Y, Horn N, Hetterschijt L, Wdowicz A, et al. Active transport and diffusion barriers restrict Joubert Syndrome-associated ARL13B/ARL-13 to an Inv-like ciliary membrane subdomain. PLoS Genet 2013; 9:e1003977; http://dx.doi.org/10.1371/journal.pgen.1003977; PMID: 24339792
- Zhao C, Malicki J. Nephrocystins and MKS proteins interact with IFT particle and facilitate transport of selected ciliary cargos. EMBO J 2011; 30:2532 - 44; http://dx.doi.org/10.1038/emboj.2011.165; PMID: 21602787
- Belzile O, Hernandez-Lara CI, Wang Q, Snell WJ. Regulated membrane protein entry into flagella is facilitated by cytoplasmic microtubules and does not require IFT. Curr Biol 2013; 23:1460 - 5; http://dx.doi.org/10.1016/j.cub.2013.06.025; PMID: 23891117
- Breslow DK, Koslover EF, Seydel F, Spakowitz AJ, Nachury MV. An in vitro assay for entry into cilia reveals unique properties of the soluble diffusion barrier. J Cell Biol 2013; 203:129 - 47; http://dx.doi.org/10.1083/jcb.201212024; PMID: 24100294
- Lin YC, Niewiadomski P, Lin B, Nakamura H, Phua SC, Jiao J, Levchenko A, Inoue T, Rohatgi R, Inoue T. Chemically inducible diffusion trap at cilia reveals molecular sieve-like barrier. Nat Chem Biol 2013; 9:437 - 43; http://dx.doi.org/10.1038/nchembio.1252; PMID: 23666116
- Najafi M, Calvert PD. Transport and localization of signaling proteins in ciliated cells. Vision Res 2012; 75:11 - 8; http://dx.doi.org/10.1016/j.visres.2012.08.006; PMID: 22922002
- Kee HL, Verhey KJ. Molecular connections between nuclear and ciliary import processes. Cilia 2013; 2:11; http://dx.doi.org/10.1186/2046-2530-2-11; PMID: 23985042
- Malicki J, Besharse JC. Kinesin-2 family motors in the unusual photoreceptor cilium. Vision Res 2012; 75:33 - 6; http://dx.doi.org/10.1016/j.visres.2012.10.008; PMID: 23123805
- Ounjai P, Kim KD, Liu H, Dong M, Tauscher AN, Witkowska HE, Downing KH. Architectural insights into a ciliary partition. Curr Biol 2013; 23:339 - 44; http://dx.doi.org/10.1016/j.cub.2013.01.029; PMID: 23375896
- Rakoczy EP, Kiel C, McKeone R, Stricher F, Serrano L. Analysis of Disease-Linked Rhodopsin Mutations Based on Structure, Function, and Protein Stability Calculations. J Mol Biol 2011; 405:584 - 606; PMID: 21094163
- Humbert MC, Weihbrecht K, Searby CC, Li Y, Pope RM, Sheffield VC, Seo S. ARL13B, PDE6D, and CEP164 form a functional network for INPP5E ciliary targeting. Proc Natl Acad Sci U S A 2012; 109:19691 - 6; http://dx.doi.org/10.1073/pnas.1210916109; PMID: 23150559
- Tam BM, Moritz OL, Hurd LB, Papermaster DS. Identification of an outer segment targeting signal in the COOH terminus of rhodopsin using transgenic Xenopus laevis. J Cell Biol 2000; 151:1369 - 80; http://dx.doi.org/10.1083/jcb.151.7.1369; PMID: 11134067
- Baker SA, Haeri M, Yoo P, Gospe SM 3rd, Skiba NP, Knox BE, Arshavsky VY. The outer segment serves as a default destination for the trafficking of membrane proteins in photoreceptors. J Cell Biol 2008; 183:485 - 98; http://dx.doi.org/10.1083/jcb.200806009; PMID: 18981232
- Young RW. The renewal of photoreceptor cell outer segments. J Cell Biol 1967; 33:61 - 72; http://dx.doi.org/10.1083/jcb.33.1.61; PMID: 6033942
- Young RW. Shedding of discs from rod outer segments in the rhesus monkey. J Ultrastruct Res 1971; 34:190 - 203; http://dx.doi.org/10.1016/S0022-5320(71)90014-1; PMID: 4992906
- Dwyer ND, Adler CE, Crump JG, L’Etoile ND, Bargmann CI. Polarized dendritic transport and the AP-1 mu1 clathrin adaptor UNC-101 localize odorant receptors to olfactory cilia. Neuron 2001; 31:277 - 87; http://dx.doi.org/10.1016/S0896-6273(01)00361-0; PMID: 11502258
- Corbit KC, Aanstad P, Singla V, Norman AR, Stainier DY, Reiter JF. Vertebrate Smoothened functions at the primary cilium. Nature 2005; 437:1018 - 21; http://dx.doi.org/10.1038/nature04117; PMID: 16136078
- Berbari NF, Johnson AD, Lewis JS, Askwith CC, Mykytyn K. Identification of ciliary localization sequences within the third intracellular loop of G protein-coupled receptors. Mol Biol Cell 2008; 19:1540 - 7; http://dx.doi.org/10.1091/mbc.E07-09-0942; PMID: 18256283
- Loktev AV, Jackson PK. Neuropeptide Y family receptors traffic via the Bardet-Biedl syndrome pathway to signal in neuronal primary cilia. Cell Rep 2013; 5:1316 - 29; http://dx.doi.org/10.1016/j.celrep.2013.11.011; PMID: 24316073
- Tam BM, Moritz OL, Papermaster DS. The C terminus of peripherin/rds participates in rod outer segment targeting and alignment of disk incisures. Mol Biol Cell 2004; 15:2027 - 37; http://dx.doi.org/10.1091/mbc.E03-09-0650; PMID: 14767063
- Hagstrom SA, Adamian M, Scimeca M, Pawlyk BS, Yue G, Li T. A role for the Tubby-like protein 1 in rhodopsin transport. Invest Ophthalmol Vis Sci 2001; 42:1955 - 62; PMID: 11481257
- Zhao Y, Hong DH, Pawlyk B, Yue G, Adamian M, Grynberg M, Godzik A, Li T. The retinitis pigmentosa GTPase regulator (RPGR)- interacting protein: subserving RPGR function and participating in disk morphogenesis. Proc Natl Acad Sci U S A 2003; 100:3965 - 70; http://dx.doi.org/10.1073/pnas.0637349100; PMID: 12651948
- Abd-El-Barr MM, Sykoudis K, Andrabi S, Eichers ER, Pennesi ME, Tan PL, Wilson JH, Katsanis N, Lupski JR, Wu SM. Impaired photoreceptor protein transport and synaptic transmission in a mouse model of Bardet-Biedl syndrome. Vision Res 2007; 47:3394 - 407; http://dx.doi.org/10.1016/j.visres.2007.09.016; PMID: 18022666
- Geng L, Okuhara D, Yu Z, Tian X, Cai Y, Shibazaki S, Somlo S. Polycystin-2 traffics to cilia independently of polycystin-1 by using an N-terminal RVxP motif. J Cell Sci 2006; 119:1383 - 95; http://dx.doi.org/10.1242/jcs.02818; PMID: 16537653
- Deretic D, Williams AH, Ransom N, Morel V, Hargrave PA, Arendt A. Rhodopsin C terminus, the site of mutations causing retinal disease, regulates trafficking by binding to ADP-ribosylation factor 4 (ARF4). Proc Natl Acad Sci U S A 2005; 102:3301 - 6; http://dx.doi.org/10.1073/pnas.0500095102; PMID: 15728366
- Chuang JZ, Zhao Y, Sung CH. SARA-regulated vesicular targeting underlies formation of the light-sensing organelle in mammalian rods. Cell 2007; 130:535 - 47; http://dx.doi.org/10.1016/j.cell.2007.06.030; PMID: 17693260
- Mazelova J, Astuto-Gribble L, Inoue H, Tam BM, Schonteich E, Prekeris R, Moritz OL, Randazzo PA, Deretic D. Ciliary targeting motif VxPx directs assembly of a trafficking module through Arf4. EMBO J 2009; 28:183 - 92; http://dx.doi.org/10.1038/emboj.2008.267; PMID: 19153612
- Besharse JC, Pfenninger KH. Membrane assembly in retinal photoreceptors I. Freeze-fracture analysis of cytoplasmic vesicles in relationship to disc assembly. J Cell Biol 1980; 87:451 - 63; http://dx.doi.org/10.1083/jcb.87.2.451; PMID: 7430251
- Papermaster DS, Schneider BG, DeFoe D, Besharse JC. Biosynthesis and vectorial transport of opsin on vesicles in retinal rod photoreceptors. J Histochem Cytochem 1986; 34:5 - 16; http://dx.doi.org/10.1177/34.1.2934469; PMID: 2934469
- Vaughan DK, Fisher SK, Bernstein SA, Hale IL, Linberg KA, Matsumoto B. Evidence that microtubules do not mediate opsin vesicle transport in photoreceptors. J Cell Biol 1989; 109:3053 - 62; http://dx.doi.org/10.1083/jcb.109.6.3053; PMID: 2687292
- Berbari NF, Lewis JS, Bishop GA, Askwith CC, Mykytyn K. Bardet-Biedl syndrome proteins are required for the localization of G protein-coupled receptors to primary cilia. Proc Natl Acad Sci U S A 2008; 105:4242 - 6; http://dx.doi.org/10.1073/pnas.0711027105; PMID: 18334641
- Domire JS, Green JA, Lee KG, Johnson AD, Askwith CC, Mykytyn K. Dopamine receptor 1 localizes to neuronal cilia in a dynamic process that requires the Bardet-Biedl syndrome proteins. Cell Mol Life Sci 2011; 68:2951 - 60; http://dx.doi.org/10.1007/s00018-010-0603-4; PMID: 21152952
- Moritz OL, Tam BM, Hurd LL, Peränen J, Deretic D, Papermaster DS. Mutant rab8 Impairs docking and fusion of rhodopsin-bearing post-Golgi membranes and causes cell death of transgenic Xenopus rods. Mol Biol Cell 2001; 12:2341 - 51; http://dx.doi.org/10.1091/mbc.12.8.2341; PMID: 11514620
- Nachury MV, Loktev AV, Zhang Q, Westlake CJ, Peränen J, Merdes A, Slusarski DC, Scheller RH, Bazan JF, Sheffield VC, et al. A core complex of BBS proteins cooperates with the GTPase Rab8 to promote ciliary membrane biogenesis. Cell 2007; 129:1201 - 13; http://dx.doi.org/10.1016/j.cell.2007.03.053; PMID: 17574030
- Omori Y, Zhao C, Saras A, Mukhopadhyay S, Kim W, Furukawa T, Sengupta P, Veraksa A, Malicki J. Elipsa is an early determinant of ciliogenesis that links the IFT particle to membrane-associated small GTPase Rab8. Nat Cell Biol 2008; 10:437 - 44; http://dx.doi.org/10.1038/ncb1706; PMID: 18364699
- Knödler A, Feng S, Zhang J, Zhang X, Das A, Peränen J, Guo W. Coordination of Rab8 and Rab11 in primary ciliogenesis. Proc Natl Acad Sci U S A 2010; 107:6346 - 51; http://dx.doi.org/10.1073/pnas.1002401107; PMID: 20308558
- Westlake CJ, Baye LM, Nachury MV, Wright KJ, Ervin KE, Phu L, Chalouni C, Beck JS, Kirkpatrick DS, Slusarski DC, et al. Primary cilia membrane assembly is initiated by Rab11 and transport protein particle II (TRAPPII) complex-dependent trafficking of Rabin8 to the centrosome. Proc Natl Acad Sci U S A 2011; 108:2759 - 64; http://dx.doi.org/10.1073/pnas.1018823108; PMID: 21273506
- Chiba S, Amagai Y, Homma Y, Fukuda M, Mizuno K. NDR2-mediated Rabin8 phosphorylation is crucial for ciliogenesis by switching binding specificity from phosphatidylserine to Sec15. EMBO J 2013; 32:874 - 85; http://dx.doi.org/10.1038/emboj.2013.32; PMID: 23435566
- Yoshimura S, Egerer J, Fuchs E, Haas AK, Barr FA. Functional dissection of Rab GTPases involved in primary cilium formation. J Cell Biol 2007; 178:363 - 9; http://dx.doi.org/10.1083/jcb.200703047; PMID: 17646400
- Sato T, Iwano T, Kunii M, Matsuda S, Mizuguchi R, Jung Y, Hagiwara H, Yoshihara Y, Yuzaki M, Harada R, et al. Rab8a and Rab8b are essential for several apical transport pathways but insufficient for ciliogenesis. J Cell Sci 2014; 127:422 - 31; http://dx.doi.org/10.1242/jcs.136903; PMID: 24213529
- Munson M, Novick P. The exocyst defrocked, a framework of rods revealed. . 2006; 13:577 - 81
- Ortiz D, Medkova M, Walch-Solimena C, Novick P. Ypt32 recruits the Sec4p guanine nucleotide exchange factor, Sec2p, to secretory vesicles; evidence for a Rab cascade in yeast. J Cell Biol 2002; 157:1005 - 15; http://dx.doi.org/10.1083/jcb.200201003; PMID: 12045183
- Das A, Guo W. Rabs and the exocyst in ciliogenesis, tubulogenesis and beyond. Trends Cell Biol 2011; 21:383 - 6; http://dx.doi.org/10.1016/j.tcb.2011.03.006; PMID: 21550243
- Guo W, Roth D, Walch-Solimena C, Novick P. The exocyst is an effector for Sec4p, targeting secretory vesicles to sites of exocytosis. EMBO J 1999; 18:1071 - 80; http://dx.doi.org/10.1093/emboj/18.4.1071; PMID: 10022848
- Wu S, Mehta SQ, Pichaud F, Bellen HJ, Quiocho FA. Sec15 interacts with Rab11 via a novel domain and affects Rab11 localization in vivo. Nat Struct Mol Biol 2005; 12:879 - 85; http://dx.doi.org/10.1038/nsmb987; PMID: 16155582
- Rogers KK, Wilson PD, Snyder RW, Zhang X, Guo W, Burrow CR, Lipschutz JH. The exocyst localizes to the primary cilium in MDCK cells. Biochem Biophys Res Commun 2004; 319:138 - 43; http://dx.doi.org/10.1016/j.bbrc.2004.04.165; PMID: 15158452
- Feng S, Knödler A, Ren J, Zhang J, Zhang X, Hong Y, Huang S, Peränen J, Guo W. A Rab8 guanine nucleotide exchange factor-effector interaction network regulates primary ciliogenesis. J Biol Chem 2012; 287:15602 - 9; http://dx.doi.org/10.1074/jbc.M111.333245; PMID: 22433857
- Zuo X, Guo W, Lipschutz JH. The exocyst protein Sec10 is necessary for primary ciliogenesis and cystogenesis in vitro. Mol Biol Cell 2009; 20:2522 - 9; http://dx.doi.org/10.1091/mbc.E08-07-0772; PMID: 19297529
- Sacher M, Kim Y-G, Lavie A, Oh B-H, Segev N. The TRAPP complex: insights into its architecture and function. Traffic 2008; 9:2032 - 42; http://dx.doi.org/10.1111/j.1600-0854.2008.00833.x; PMID: 18801063
- Levine TP, Daniels RD, Wong LH, Gatta AT, Gerondopoulos A, Barr FA. Discovery of new Longin and Roadblock domains that form platforms for small GTPases in Ragulator and TRAPP-II. Small GTPases 2013; 4:62 - 9; http://dx.doi.org/10.4161/sgtp.24262; PMID: 23511850
- Jones S, Newman C, Liu F, Segev N. The TRAPP complex is a nucleotide exchanger for Ypt1 and Ypt31/32. Mol Biol Cell 2000; 11:4403 - 11; http://dx.doi.org/10.1091/mbc.11.12.4403; PMID: 11102533
- Zou S, Liu Y, Zhang XQ, Chen Y, Ye M, Zhu X, Yang S, Lipatova Z, Liang Y, Segev N. Modular TRAPP complexes regulate intracellular protein trafficking through multiple Ypt/Rab GTPases in Saccharomyces cerevisiae. Genetics 2012; 191:451 - 60; http://dx.doi.org/10.1534/genetics.112.139378; PMID: 22426882
- Zaghloul NA, Katsanis N. Mechanistic insights into Bardet-Biedl syndrome, a model ciliopathy. J Clin Invest 2009; 119:428 - 37; http://dx.doi.org/10.1172/JCI37041; PMID: 19252258
- Seo S, Baye LM, Schulz NP, Beck JS, Zhang Q, Slusarski DC, Sheffield VC. BBS6, BBS10, and BBS12 form a complex with CCT/TRiC family chaperonins and mediate BBSome assembly. Proc Natl Acad Sci U S A 2010; 107:1488 - 93; http://dx.doi.org/10.1073/pnas.0910268107; PMID: 20080638
- Nishimura DY, Fath M, Mullins RF, Searby C, Andrews M, Davis R, Andorf JL, Mykytyn K, Swiderski RE, Yang B, et al. Bbs2-null mice have neurosensory deficits, a defect in social dominance, and retinopathy associated with mislocalization of rhodopsin. Proc Natl Acad Sci U S A 2004; 101:16588 - 93; http://dx.doi.org/10.1073/pnas.0405496101; PMID: 15539463
- Gillingham AK, Munro S. The small G proteins of the Arf family and their regulators. Annu Rev Cell Dev Biol 2007; 23:579 - 611; http://dx.doi.org/10.1146/annurev.cellbio.23.090506.123209; PMID: 17506703
- van Dam TJP, Townsend MJ, Turk M, Schlessinger A, Sali A, Field MC, Huynen MA. Evolution of modular intraflagellar transport from a coatomer-like progenitor. Proc Natl Acad Sci U S A 2013; 110:6943 - 8; http://dx.doi.org/10.1073/pnas.1221011110; PMID: 23569277
- Jékely G, Arendt D. Evolution of intraflagellar transport from coated vesicles and autogenous origin of the eukaryotic cilium. Bioessays 2006; 28:191 - 8; http://dx.doi.org/10.1002/bies.20369; PMID: 16435301
- Ou G, Blacque OE, Snow JJ, Leroux MR, Scholey JM. Functional coordination of intraflagellar transport motors. Nature 2005; 436:583 - 7; http://dx.doi.org/10.1038/nature03818; PMID: 16049494
- Wei Q, Zhang Y, Li Y, Zhang Q, Ling K, Hu J. The BBSome controls IFT assembly and turnaround in cilia. Nat Cell Biol 2012; 14:950 - 7; http://dx.doi.org/10.1038/ncb2560; PMID: 22922713
- Nachury MV, Seeley ES, Jin H. Trafficking to the ciliary membrane: how to get across the periciliary diffusion barrier?. Annu Rev Cell Dev Biol 2010; 26:59 - 87; http://dx.doi.org/10.1146/annurev.cellbio.042308.113337; PMID: 19575670
- Oeffner F, Moch C, Neundorf A, Hofmann J, Koch M, Grzeschik KH. Novel interaction partners of Bardet-Biedl syndrome proteins. Cell Motil Cytoskeleton 2008; 65:143 - 55; http://dx.doi.org/10.1002/cm.20250; PMID: 18000879
- He B, Xi F, Zhang X, Zhang J, Guo W. Exo70 interacts with phospholipids and mediates the targeting of the exocyst to the plasma membrane. EMBO J 2007; 26:4053 - 65; http://dx.doi.org/10.1038/sj.emboj.7601834; PMID: 17717527
- Sp O, Gasman S. Rho GTPases and exocytosis: What are the molecular links?. Semin Cell Dev Biol 2010; 22:27 - 32
- He B, Guo W. The exocyst complex in polarized exocytosis. Curr Opin Cell Biol 2009; 21:537 - 42; http://dx.doi.org/10.1016/j.ceb.2009.04.007; PMID: 19473826
- Mukhopadhyay S, Jackson PK. The tubby family proteins. Genome Biol 2011; 12:225; http://dx.doi.org/10.1186/gb-2011-12-6-225; PMID: 21722349
- Mukhopadhyay S, Wen X, Ratti N, Loktev A, Rangell L, Scales SJ, Jackson PK. The ciliary G-protein-coupled receptor Gpr161 negatively regulates the Sonic hedgehog pathway via cAMP signaling. Cell 2013; 152:210 - 23; http://dx.doi.org/10.1016/j.cell.2012.12.026; PMID: 23332756
- Sun X, Haley J, Bulgakov OV, Cai X, McGinnis J, Li T. Tubby is required for trafficking G protein-coupled receptors to neuronal cilia. Cilia 2012; 1:21; PMID: 23351594
- Hagstrom SA, North MA, Nishina PL, Berson EL, Dryja TP. Recessive mutations in the gene encoding the tubby-like protein TULP1 in patients with retinitis pigmentosa. Nat Genet 1998; 18:174 - 6; http://dx.doi.org/10.1038/ng0298-174; PMID: 9462750
- Santagata S, Boggon TJ, Baird CL, Gomez CA, Zhao J, Shan WS, Myszka DG, Shapiro L. G-protein signaling through tubby proteins. Science 2001; 292:2041 - 50; http://dx.doi.org/10.1126/science.1061233; PMID: 11375483
- Mak HY, Nelson LS, Basson M, Johnson CD, Ruvkun G. Polygenic control of Caenorhabditis elegans fat storage. Nat Genet 2006; 38:363 - 8; http://dx.doi.org/10.1038/ng1739; PMID: 16462744
- Stubdal H, Lynch CA, Moriarty A, Fang Q, Chickering T, Deeds JD, Fairchild-Huntress V, Charlat O, Dunmore JH, Kleyn P, et al. Targeted deletion of the tub mouse obesity gene reveals that tubby is a loss-of-function mutation. Mol Cell Biol 2000; 20:878 - 82; http://dx.doi.org/10.1128/MCB.20.3.878-882.2000; PMID: 10629044
- Ashrafi K, Chang FY, Watts JL, Fraser AG, Kamath RS, Ahringer J, Ruvkun G. Genome-wide RNAi analysis of Caenorhabditis elegans fat regulatory genes. Nature 2003; 421:268 - 72; http://dx.doi.org/10.1038/nature01279; PMID: 12529643
- Mykytyn K, Mullins RF, Andrews M, Chiang AP, Swiderski RE, Yang B, Braun T, Casavant T, Stone EM, Sheffield VC. Bardet-Biedl syndrome type 4 (BBS4)-null mice implicate Bbs4 in flagella formation but not global cilia assembly. Proc Natl Acad Sci U S A 2004; 101:8664 - 9; http://dx.doi.org/10.1073/pnas.0402354101; PMID: 15173597
- Goetz SC, Anderson KV. The primary cilium: a signalling centre during vertebrate development. Nat Rev Genet 2010; 11:331 - 44; http://dx.doi.org/10.1038/nrg2774; PMID: 20395968
- Brooks ER, Wallingford JB. Control of vertebrate intraflagellar transport by the planar cell polarity effector Fuz. J Cell Biol 2012; 198:37 - 45; http://dx.doi.org/10.1083/jcb.201204072; PMID: 22778277
- Zilber Y, Babayeva S, Seo JH, Liu JJ, Mootin S, Torban E. The PCP effector Fuzzy controls cilial assembly and signaling by recruiting Rab8 and Dishevelled to the primary cilium. Mol Biol Cell 2013; 24:555 - 65; http://dx.doi.org/10.1091/mbc.E12-06-0437; PMID: 23303251
- Follit JA, Tuft RA, Fogarty KE, Pazour GJ. The intraflagellar transport protein IFT20 is associated with the Golgi complex and is required for cilia assembly. Mol Biol Cell 2006; 17:3781 - 92; http://dx.doi.org/10.1091/mbc.E06-02-0133; PMID: 16775004
- Follit JA, San Agustin JT, Xu F, Jonassen JA, Samtani R, Lo CW, Pazour GJ. The Golgin GMAP210/TRIP11 anchors IFT20 to the Golgi complex. PLoS Genet 2008; 4:e1000315; http://dx.doi.org/10.1371/journal.pgen.1000315; PMID: 19112494
- Briscoe J, Thérond PP. The mechanisms of Hedgehog signalling and its roles in development and disease. Nature Publishing Group 2013.
- Hunnicutt GR, Kosfiszer MG, Snell WJ. Cell body and flagellar agglutinins in Chlamydomonas reinhardtii: the cell body plasma membrane is a reservoir for agglutinins whose migration to the flagella is regulated by a functional barrier. J Cell Biol 1990; 111:1605 - 16; http://dx.doi.org/10.1083/jcb.111.4.1605; PMID: 2170424
- Fowkes ME, Mitchell DR. The role of preassembled cytoplasmic complexes in assembly of flagellar dynein subunits. Mol Biol Cell 1998; 9:2337 - 47; http://dx.doi.org/10.1091/mbc.9.9.2337; PMID: 9725897
- Clarke PR, Zhang C. Spatial and temporal coordination of mitosis by Ran GTPase. Nat Rev Mol Cell Biol 2008; 9:464 - 77; http://dx.doi.org/10.1038/nrm2410; PMID: 18478030
- Hurd TW, Fan S, Margolis BL. Localization of retinitis pigmentosa 2 to cilia is regulated by Importin beta2. J Cell Sci 2011; 124:718 - 26; http://dx.doi.org/10.1242/jcs.070839; PMID: 21285245
- Constantine R, Zhang H, Gerstner CD, Frederick JM, Baehr W. Uncoordinated (UNC)119: coordinating the trafficking of myristoylated proteins. Vision Res 2012; 75:26 - 32; http://dx.doi.org/10.1016/j.visres.2012.08.012; PMID: 23000199
- Cherfils J, Zeghouf M. Regulation of small GTPases by GEFs, GAPs, and GDIs. Physiol Rev 2013; 93:269 - 309; http://dx.doi.org/10.1152/physrev.00003.2012; PMID: 23303910
- Zhang H, Constantine R, Vorobiev S, Chen Y, Seetharaman J, Huang YJ, Xiao R, Montelione GT, Gerstner CD, Davis MW, et al. UNC119 is required for G protein trafficking in sensory neurons. Nat Neurosci 2011; 14:874 - 80; http://dx.doi.org/10.1038/nn.2835; PMID: 21642972
- Wright KJ, Baye LM, Olivier-Mason A, Mukhopadhyay S, Sang L, Kwong M, Wang W, Pretorius PR, Sheffield VC, Sengupta P, et al. An ARL3-UNC119-RP2 GTPase cycle targets myristoylated NPHP3 to the primary cilium. 2011; 25:2347 - 60
- Nakata K, Shiba D, Kobayashi D, Yokoyama T. Targeting of Nphp3 to the primary cilia is controlled by an N-terminal myristoylation site and coiled-coil domains. Cytoskeleton (Hoboken) 2012; 69:221 - 34; http://dx.doi.org/10.1002/cm.21014; PMID: 22328406
- Gorska MM, Liang Q, Karim Z, Alam R. Uncoordinated 119 protein controls trafficking of Lck via the Rab11 endosome and is critical for immunological synapse formation. J Immunol 2009; 183:1675 - 84; http://dx.doi.org/10.4049/jimmunol.0900792; PMID: 19592652
- Finetti F, Baldari CT. Compartmentalization of signaling by vesicular trafficking: a shared building design for the immune synapse and the primary cilium. Immunol Rev 2013; 251:97 - 112; http://dx.doi.org/10.1111/imr.12018; PMID: 23278743
- Galbraith CG, Galbraith JA. Super-resolution microscopy at a glance. J Cell Sci 2011; 124:1607 - 11; http://dx.doi.org/10.1242/jcs.080085; PMID: 21536831
- Deretic D. A role for rhodopsin in a signal transduction cascade that regulates membrane trafficking and photoreceptor polarity. Vision Res 2006; 46:4427 - 33; http://dx.doi.org/10.1016/j.visres.2006.07.028; PMID: 17010408
- Ward HH, Brown-Glaberman U, Wang J, Morita Y, Alper SL, Bedrick EJ, Gattone VH 2nd, Deretic D, Wandinger-Ness A. A conserved signal and GTPase complex are required for the ciliary transport of polycystin-1. Mol Biol Cell 2011; 22:3289 - 305; http://dx.doi.org/10.1091/mbc.E11-01-0082; PMID: 21775626
- Jenkins PM, Hurd TW, Zhang L, McEwen DP, Brown RL, Margolis B, Verhey KJ, Martens JR. Ciliary targeting of olfactory CNG channels requires the CNGB1b subunit and the kinesin-2 motor protein, KIF17. Curr Biol 2006; 16:1211 - 6; http://dx.doi.org/10.1016/j.cub.2006.04.034; PMID: 16782012
- Follit JA, Li L, Vucica Y, Pazour GJ. The cytoplasmic tail of fibrocystin contains a ciliary targeting sequence. J Cell Biol 2010; 188:21 - 8; http://dx.doi.org/10.1083/jcb.200910096; PMID: 20048263
- Tao B, Bu S, Yang Z, Siroky B, Kappes JC, Kispert A, Guay-Woodford LM. Cystin localizes to primary cilia via membrane microdomains and a targeting motif. J Am Soc Nephrol 2009; 20:2570 - 80; http://dx.doi.org/10.1681/ASN.2009020188; PMID: 19850956
- Luo W, Marsh-Armstrong N, Rattner A, Nathans J. An outer segment localization signal at the C terminus of the photoreceptor-specific retinol dehydrogenase. J Neurosci 2004; 24:2623 - 32; http://dx.doi.org/10.1523/JNEUROSCI.5302-03.2004; PMID: 15028754
- Santos N, Reiter JF. A central region of Gli2 regulates its localization to the primary cilium and transcriptional activity. J Cell Sci 2014; 127:1500 - 10; http://dx.doi.org/10.1242/jcs.139253; PMID: 24463817
- Cheng Y-Z, Eley L, Hynes AM, Overman LM, Simms RJ, Barker A, Dawe HR, Lindsay S, Sayer JA. Investigating embryonic expression patterns and evolution of AHI1 and CEP290 genes, implicated in Joubert syndrome. PLoS One 2012; 7:e44975; http://dx.doi.org/10.1371/journal.pone.0044975; PMID: 23028714
- Branchek T, Bremiller R. The development of photoreceptors in the zebrafish, Brachydanio rerio. I. Structure. J Comp Neurol 1984; 224:107 - 15; http://dx.doi.org/10.1002/cne.902240109; PMID: 6715574
- Townes-Anderson E, Dacheux RF, Raviola E. Rod photoreceptors dissociated from the adult rabbit retina. J Neurosci 1988; 8:320 - 31; PMID: 3339415
- Walls G. The Verterbrate Eye and its Adaptive Radiation. New York: Hafner, 1942.
- Shanbhag SR, Park S-K, Pikielny CW, Steinbrecht RA. Gustatory organs of Drosophila melanogaster: fine structure and expression of the putative odorant-binding protein PBPRP2. Cell Tissue Res 2001; 304:423 - 37; http://dx.doi.org/10.1007/s004410100388; PMID: 11456419
- Keil TA. Functional morphology of insect mechanoreceptors. Microsc Res Tech 1997; 39:506 - 31; http://dx.doi.org/10.1002/(SICI)1097-0029(19971215)39:6<506::AID-JEMT5>3.0.CO;2-B; PMID: 9438251