Abstract
This commentary discusses the rationale behind our recently reported work entitled “Mimicking isovolumic contraction with combined electromechanical stimulation improves the development of engineered cardiac constructs,” introduces new data supporting our hypothesis, and discusses future applications of our bioreactor system. The ability to stimulate engineered cardiac tissue in a bioreactor system that combines both electrical and mechanical stimulation offers a unique opportunity to simulate the appropriate dynamics between stretch and contraction and model isovolumic contraction in vitro. Our previous study demonstrated that combined electromechanical stimulation that simulated the timing of isovolumic contraction in healthy tissue improved force generation via increased contractile and calcium handling protein expression and improved hypertrophic pathway activation. In new data presented here, we further demonstrate that modification of the timing between electrical and mechanical stimulation to mimic a non-physiological process negatively impacts the functionality of the engineered constructs. We close by exploring the various disease states that have altered timing between the electrical and mechanical stimulation signals as potential future directions for the use of this system.
Introduction and Motivation
Our recently published work “Mimicking isovolumic contraction with combined electromechanical stimulation improves the development of engineered cardiac constructs” describes a bioreactor system that can precisely control the timing between combined electrical and mechanical stimulation of engineered cardiac tissue constructs, allowing us to study how synchronized vs. delayed electromechanical stimulation influences the development of function in engineered cardiac tissues.Citation1 The aim of this commentary is to provide additional insights into the rationale behind the simulation of isovolumic contractions in a dual stimulation bioreactor system, introduce new data that supports our claims that the timing of combined stimulation is important, and highlight potential uses of this technology in the study of various cardiac diseases.
Individually, both mechanical and electrical stimulation have been established as standard conditioning methods for engineered cardiac constructs and have been studied extensively previously. For instance, mechanical stimulation has been used in a wide variety of bioreactor systems,Citation2-4 and has been shown previously to activate FAK and RhoA, which initiates myotube formationCitation5 and promotes extracellular matrix (ECM) formation,Citation6 improving the overall organization of the tissue and thus the contraction force.Citation2 Similarly, electrical stimulation has been used in other systems,Citation7-9 and has been shown to lead to synchronous contractions of the myocytes, by inducing alignment in the cardiomyocytesCitation10 and increasing the volume fraction and organization of sarcomeres.Citation11 Likewise, in our system, we observed that though electrical and mechanical stimulation both improve twitch forces over the static conditions, they influenced development in different ways. Mechanical stimulation had a statistically significant increase in the cell number in the constructs after 14 d in culture, while electrical stimulation appeared to have a slightly higher percentage of cardiomyocytes in the culture.
In modeling the dynamic electromechanical environment present in vitro, it is important to note that the mechanical stimulation simulates the ventricle filing with blood, while electrical stimulation mimics the induction of the ventricular contraction. While single-modality stimulation has its benefits, the environment that cells are subjected to in vivo involves both electrical and mechanical signaling, and the timing of these two events can be critical. The interaction of these two stimuli occurs during Isovolumic contraction, which is a critical period in the cardiac cycle where the left ventricle is stimulated to contract, but it is maintained in a stretched position until enough pressure is built up in the ventricle to cause the aortic valve to open.Citation12 The alteration of Isovolumic contraction time has been established as a method of evaluating cardiac functionCitation13 due to its direct relationship with myocardial contractilityCitation14 and its sensitivity to alterations in both hemodynamic activity and contractile function.Citation15 Thus, when creating engineered cardiac tissue, in vitro methods of recapitulating the specific timing of combined electromechanical stimulation could be an important factor in promoting optimal construct function.
Though electrical and mechanical stimulation have been previously combined in other bioreactor systems,Citation16-19 the effect of delayed timing between electrical and mechanical stimulation had not been studied. Mimicking the timing present in vivo causes a switch in the contractile model of the muscle from an isometric mode when the valve is closed to an isotonic shortening, once the valve opens.Citation20 Previous studies have shown that muscles, on a tissueCitation21 and cellular level,Citation22 behave differently under isometric conditions vs. isotonic shortening. Under isometric conditions, mechanical stretch shortens the intracellular calcium transients,Citation23 and maximizes the forces generated by the tissue. Under isotonic shortening, the intracellular calcium transients are prolonged.Citation24 Thus, the switch in contractile modes that would be generated during a stimulation that mimics isovolumic contraction could allow for a higher force to be maintained during the shortening period, which would increase the network output,Citation25 and could modulate the calcium dependent action potential duration in our engineered constructs,Citation20 thus maximizing the forces generated.Citation26
Our system allows for the study of changes in isovolumic contraction by changing the timing between the beginning of the mechanical stimulation and the beginning of electrical stimulation. To model the isovolumic contraction, the electrical stimulation was delayed until 10 ms before the end of the mechanical stimulation. While the ICT in the human heart is approximately 40 ms,Citation27 an ICT of 10 ms was chosen to model the ICT observed in the rat. This time was based on a derivation of the ICT from previously published data on the Myocardial Performance Index(MPI)Citation28 and literature values of ejection time and isovolumic relaxation time.Citation29,30 With the delayed condition, we observed an increase in the twitch force as compared with a simultaneous electromechanical stimulation. The increase in function was likely due to the increases in the protein expression of Troponin T, the thin-filament regulatory protein necessary for coordinated force generationCitation31 and cardiac sarcoplasmic reticulum Ca2+ ATPase (SERCA2a), the protein responsible for pumping calcium back into the sarcoplasmic reticulum during diastole.Citation32 The increase in Troponin T and SERCA2a indicates that modeling the isovolumic contraction improves calcium sensitivity and handling, which is necessary for cardiomyocyte maturation. Observed increases in Akt expression further suggested that this maturation could be via physiological hypertrophic pathway.Citation33,34
Offsetting Electrical and Mechanical Stimulation
While the data from our previous paperCitation1 indicate that the timing between electrical and mechanical stimulation can be utilized to enhance the function of engineered cardiac tissues, we only investigated variations in the timing where the two stimuli were overlapping. To further build the case on the importance of timing in a combined electromechanical bioreactor system, here we present new data investigating the effects of offsetting the electrical and mechanical stimulation so that they were completely out of phase. As shown in , two additional conditions beyond those studied in the original paper were added: an Offset (O) condition where the electrical stimulation was completely out of phase with the mechanical stimulation but the rate of mechanical stimulation was maintained (1Hz), and a Half Offset (H) condition in which the electrical and mechanical stimulation alternated at 1 Hz stimulation. The offset condition represents a condition that is physiologically irrelevant, given that electrical and mechanical stimulation does not occur in isolation in the native heart. In this case, though the alternating electrical and mechanical stimulation could potentially combat the cellular adaptation to stimuli, the electrical stimulation would cause the construct to contract against no resistance, likely reducing its effect. Thus, we hypothesized that the offset conditions would have less functionality than the delayed electromechanical stimulation.
Figure 1. (A) A diagram demonstrating the stimulation regimens implemented. The blue line represents the mechanical stretch while the red line represents the electrical stimulation. Portions reprinted with permission from reference 1.
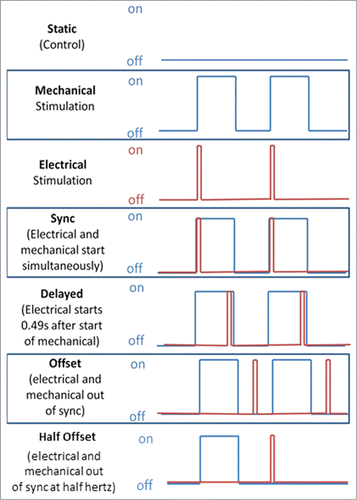
It is important to note that all experimental groups, including both the new and previously published data, were created from the same cell isolation and cultured at the same time in the bioreactor system. Twitch force results () indicate that the static condition (2.08 ± 0.77 mN) had a statistically significantly lower contraction force than all other conditions but the half offset condition (2.03 ± 1.13 mN) (P < 0.05). The offset condition exhibited a similar twitch force (3.33 ± 1.02 mN) to the delayed condition (3.29 ± 1.09 mN). The force generation time () and the force relaxation time () were statistically greater in the half offset condition than in the static, mechanical only, electrical only and synchronized conditions. The force generation and relaxation rates were also statistically less in the static and half offset conditions (, respectively). Contrary to our initial hypothesis, the offset condition maintained a similar twitch force to the delayed conditions, indicating that an additional factor could be influencing the development of the constructs and offsetting the potential negative effects of mistimed stimulation. One potential explanation was that by offsetting the electrical and mechanical stimulation in the manner that we did, we increased the effective rate of stimulation from 1 Hz to 2 Hz. Increases in the frequency of both mechanicalCitation3 and electricalCitation35 stimulation have been shown to lead to increased force development. To assess what effect this was having in our system, we added a half offset condition in which the stimulation occurred at half of the frequency of the other groups (total stimulation frequency of 1 Hz, individual stimulation was at 0.5 Hz) to determine if the offset electromechanical stimulation or the increased frequency was the cause of the observed functionality. Though twitch force testing occurred at the same rates for all groups, unlike the standard offset condition, the half offset condition demonstrated a reduced twitch force and decreased rate of force generation and relaxation, similar to the static conditions. Twitch force generation rate signifies the ability of the tissue to contract simultaneously, indicating high levels of cell-cell coupling, which allows for the quick propagation of the calcium signal and a faster rate of force generation.Citation36 Previous contraction kinetics experiments in skinned fibers, skinned myocytes, and subcellular cardiac myofibrils have also indicated that elevated Ca2+ increases both the forces generated and the rate of force generation.Citation37 Furthermore, alterations in relaxation rate are likely due to changes in the expression, function, and interactions of Ca2+ handling proteins, such as SERCA2a.Citation38,39 Our results indicate that increased stimulation rate was likely the cause of the similar levels of contractile function observed in the standard offset condition as compared with the other conditions, analogous to previously published results indicating that increased frequency of mechanical stimulation improved contractile function.Citation3 In the case of the half offset we see the true effect of a 1 Hz offset stimulation and the reduction in force is accompanied by a reduction in both generation and relaxation rates indicating that altered calcium handling may be the culprit, but further testing is needed to verify this hypothesis. Additionally, these results in sum further implicate the critical importance of the timing of combined electromechanical stimulation in vitro. In summary, our experiments have demonstrated that varying the timing between electrical and mechanical stimulation in our bioreactor system can be an important factor to consider when conditioning engineered myocardium. When optimized, dual electromechanical stimulation can be a powerful tool, but mismatched stimulation can also hinder the conditioning process.
Figure 2. Observed twitch forces for all groups. and denotes statistically significantly different than all other conditions but the half offset (O) (P < 0.05). Portions reprinted with permission from reference 1.
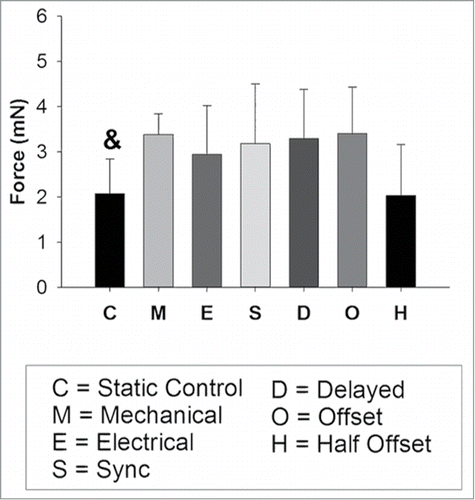
Figure 3. (A) Force generation time for all conditions. (B) Force generation rate for all conditions (mN/s). (C) Force relaxation time and (D) force relaxation rate for all conditions (mN/s). For all figures A–D, * denotes statistically different than all other conditions (P < 0.05), and denotes statistically significantly different than all other conditions but the half offset (O) (P < 0.05). # denotes statistically significantly different than all conditions but the static control (C) (P < 0.05). Portions reprinted with permission from reference 1.
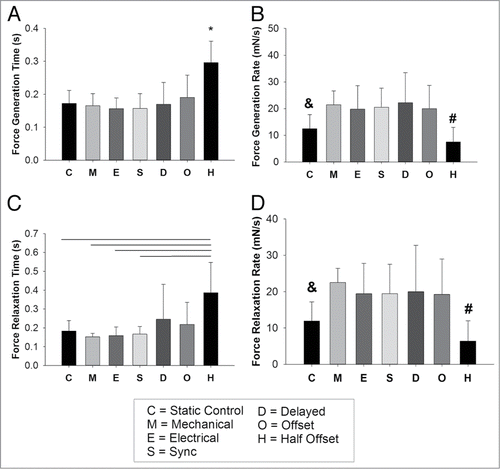
Future Directions in Modeling Development and Disease
One possible future direction of study for our system is utilizing its unique ability to vary the timings between electrical and mechanical stimulation to mimic the change of isovolumic contraction time (ICT) that occurs in normal developmentCitation40-42 or to model various myocardial diseases,Citation13,Citation43-45 specifically those that affect the ICT. For example, in fetal heart development, four different distinct stages of development occur, each with its own contraction mechanics and conduction parameters.Citation41 Contractions coupled with action potential appear by the end of the 3-somite stage.Citation42 Early cardiac development is characterized by changes in the blood flow and heart function, which are necessary to maintain the circulatory needs of the developing embryo as the heart changes morphologically and physiologically.Citation46 Previous studies have shown that the ICT appears by 6 wk of gestation to be approximately 20% of the total cardiac cycle.Citation46 ICT as a proportion of the total cardiac cycle decreases in the first trimester, even though a steady fetal heart rate is maintained, indicating a decrease in the total ICT.Citation47 Through 18–31 wk of gestation, the ICT remains constant at approximately 43 ± 14 ms.Citation48 By modeling fetal ICT over time in our system, we can more closely recapitulate the mechanical and electrical signals observed and its changes, which is likely to be beneficial in engineered cardiac tissue development, particularly when the cells utilized are immature cardiomyocytes derived from stem cell sources.
With respect to disease modeling, patients with aortic stenosis, a disease in which the aortic valve does not fully open, demonstrate a decrease in ICTCitation15 when they have preserved systolic left function, and an increase in ICT when depression of the systolic left ventricular function occurs.Citation43 A delay in the contraction occurs likely due to the prolonged effort of the left ventricle in ejecting the blood through the obstructed aortic valve,Citation49 which ultimately results in an increased afterload and slower contractions.Citation50 Aortic valve regurgitation can lead to ventricular pump failure due to a mismatch of afterload with preload.Citation51 When increases in afterload occur through reductions in the wall shortening and stroke volume, it leads to decreased performance, ultimately causing heart failure. Another potential example where the ability to alter the timing between electrical and mechanical stimulation could offer greater insight into the disease process involves patients with LV myocardial disease, where the ICT is increased significantly, from 35 ± 26 ms to 69 ± 30 ms,Citation44 likely due to decreased myocardial contractility.Citation52 In patients with heart failure, the widening of the QRS complex indicates an impaired or slowed propagation of the electrical input, which is associated with increased morbidity and mortality.Citation53 This impairment of the electrical input causes ventricular dysynchrony. Biventricular devices used in cardiac resynchronization therapy have been established as a therapeutic treatment option for patients. However, studies have indicated that optimal pacing combinations change over time and high temporal individual variability of the activation pattern exists,Citation54 indicating electrical remodeling of the affected tissue.Citation53 In these cases, a system such as ours may be able to shed light on the role that the mismatch between mechanical and electrical signaling is playing in promoting or maintaining the diseased phenotype.
The creation of a dual electromechanical stimulation bioreactor system has demonstrated the importance of modeling the isovolumic contraction in the conditioning process of engineered cardiac tissues.Citation1 The ability to vary the timing between electrical and mechanical stimulation is a powerful tool that allows us to mimic both normal developmental processes that are likely important in cardiomyocyte and myocardial tissue maturation as well as diseased tissues, such as those that exist in aortic valve stenosis and heart failure. Future studies will aim to investigate the effects of elongated and shortened isovolumic contraction time and its effects on tissue development with the goal of simulating a disease model system in vitro for studying potential treatment options.
Disclosure of Potential Conflicts of Interest
No potential conflicts of interest were disclosed.
Funding
This work was supported by the National Institutes of Health - National Heart, Lung and Blood Institute (Award # R00HL093358 to L.D.B.).
References
- Morgan KY, Black LD 3rd. Mimicking isovolumic contraction with combined electromechanical stimulation improves the development of engineered cardiac constructs. Tissue Eng Part A 2014; (Forthcoming); PMID:24410342; http://dx.doi.org/10.1089/ten.tea.2013.0355
- Zimmermann W-H, Schneiderbanger K, Schubert P, Didié M, Münzel F, Heubach JF, Kostin S, Neuhuber WL, Eschenhagen T. Tissue engineering of a differentiated cardiac muscle construct. Circ Res 2002; 90:223-30; PMID:11834716; http://dx.doi.org/10.1161/hh0202.103644
- Shimko VF, Claycomb WC. Effect of mechanical loading on three-dimensional cultures of embryonic stem cell-derived cardiomyocytes. Tissue Eng Part A 2008; 14:49-58; PMID:18333804; http://dx.doi.org/10.1089/ten.a.2007.0092
- Birla RK, Huang YC, Dennis RG. Development of a novel bioreactor for the mechanical loading of tissue-engineered heart muscle. Tissue Eng 2007; 13:2239-48; PMID:17590151; http://dx.doi.org/10.1089/ten.2006.0359
- Zhang SJ, Truskey GA, Kraus WE. Effect of cyclic stretch on beta1D-integrin expression and activation of FAK and RhoA. Am J Physiol Cell Physiol 2007; 292:C2057-69; PMID:17267546; http://dx.doi.org/10.1152/ajpcell.00493.2006
- Akhyari P, Fedak PWM, Weisel RD, Lee TY, Verma S, Mickle DA, Li RK. Mechanical stretch regimen enhances the formation of bioengineered autologous cardiac muscle grafts. Circulation 2002; 106(Suppl 1):I137-42; PMID:12354723
- Radisic M, Park H, Shing H, Consi T, Schoen FJ, Langer R, Freed LE, Vunjak-Novakovic G. Functional assembly of engineered myocardium by electrical stimulation of cardiac myocytes cultured on scaffolds. Proc Natl Acad Sci U S A 2004; 101:18129-34; PMID:15604141; http://dx.doi.org/10.1073/pnas.0407817101
- Lasher RA, Pahnke AQ, Johnson JM, Sachse FB, Hitchcock RW. Electrical stimulation directs engineered cardiac tissue to an age-matched native phenotype. J Tissue Eng 2012; 3:2041731412455354; PMID:22919458; http://dx.doi.org/10.1177/2041731412455354
- Hsiao C-W, Bai M-Y, Chang Y, Chung M-F, Lee T-Y, Wu C-T, Maiti B, Liao Z-X, Li R-K, Sung H-W. Electrical coupling of isolated cardiomyocyte clusters grown on aligned conductive nanofibrous meshes for their synchronized beating. Biomaterials 2013; 34:1063-72; PMID:23164424; http://dx.doi.org/10.1016/j.biomaterials.2012.10.065
- Wikswo JP Jr., Lin SF, Abbas RA. Virtual electrodes in cardiac tissue: a common mechanism for anodal and cathodal stimulation. Biophys J 1995; 69:2195-210; PMID:8599628; http://dx.doi.org/10.1016/S0006-3495(95)80115-3
- Huang C-C, Liao C-K, Yang M-J, Chen C-H, Hwang S-M, Hung Y-W, Chang Y, Sung H-W. A strategy for fabrication of a three-dimensional tissue construct containing uniformly distributed embryoid body-derived cells as a cardiac patch. Biomaterials 2010; 31:6218-27; PMID:20537702; http://dx.doi.org/10.1016/j.biomaterials.2010.04.067
- Fukuta H, Little WC. The cardiac cycle and the physiologic basis of left ventricular contraction, ejection, relaxation, and filling. Heart Fail Clin 2008; 4:1-11; PMID:18313620; http://dx.doi.org/10.1016/j.hfc.2007.10.004
- Weissler AM, Harris WS, Schoenfeld CD. Systolic time intervals in heart failure in man. Circulation 1968; 37:149-59; PMID:5640345; http://dx.doi.org/10.1161/01.CIR.37.2.149
- Martin CE, Shaver JA, Thompson ME, Reddy PS, Leonard JJ. Direct correlation of external systolic time intervals with internal indices of left ventricular function in man. Circulation 1971; 44:419-31; PMID:5097444; http://dx.doi.org/10.1161/01.CIR.44.3.419
- Hirschfeld S, Meyer R, Korfhagen J, Kaplan S, Liebman J. The isovolumic contraction time of the left ventricle. An echographic study. Circulation 1976; 54:751-6; PMID:975470; http://dx.doi.org/10.1161/01.CIR.54.5.751
- Kensah G, Gruh I, Viering J, Schumann H, Dahlmann J, Meyer H, Skvorc D, Bär A, Akhyari P, Heisterkamp A, et al. A novel miniaturized multimodal bioreactor for continuous in situ assessment of bioartificial cardiac tissue during stimulation and maturation. Tissue Eng Part C Methods 2011; 17:463-73; PMID:21142417; http://dx.doi.org/10.1089/ten.tec.2010.0405
- Lu L, Mende M, Yang X, Körber H-F, Schnittler HJ, Weinert S, Heubach J, Werner C, Ravens U. Design and validation of a bioreactor for simulating the cardiac niche: a system incorporating cyclic stretch, electrical stimulation, and constant perfusion. Tissue Eng Part A 2013; 19:403-14; PMID:22991978; http://dx.doi.org/10.1089/ten.tea.2012.0135
- Simmons CS, Petzold BC, Pruitt BL. Microsystems for biomimetic stimulation of cardiac cells. Lab Chip 2012; 12:3235-48; PMID:22782590; http://dx.doi.org/10.1039/c2lc40308k
- Wang B, Wang G, To F, Butler JR, Claude A, McLaughlin RM, Williams LN, de Jongh Curry AL, Liao J. Myocardial scaffold-based cardiac tissue engineering: application of coordinated mechanical and electrical stimulations. Langmuir 2013; 29:11109-17; PMID:23923967; http://dx.doi.org/10.1021/la401702w
- Calaghan SC, Belus A, White E. Do stretch-induced changes in intracellular calcium modify the electrical activity of cardiac muscle? Prog Biophys Mol Biol 2003; 82:81-95; PMID:12732270; http://dx.doi.org/10.1016/S0079-6107(03)00007-5
- Sela G, Landesberg A. The external work-pressure time integral relationships and the afterload dependence of Frank-Starling mechanism. J Mol Cell Cardiol 2009; 47:544-51; PMID:19463830; http://dx.doi.org/10.1016/j.yjmcc.2009.05.007
- Iribe G, Helmes M, Kohl P. Force-length relations in isolated intact cardiomyocytes subjected to dynamic changes in mechanical load. Am J Physiol Heart Circ Physiol 2007; 292:H1487-97; PMID:17098830; http://dx.doi.org/10.1152/ajpheart.00909.2006
- Allen DG, Kurihara S. The effects of muscle length on intracellular calcium transients in mammalian cardiac muscle. J Physiol 1982; 327:79-94; PMID:7120151
- Lab MJ, Allen DG, Orchard CH. The effects of shortening on myoplasmic calcium concentration and on the action potential in mammalian ventricular muscle. Circ Res 1984; 55:825-9; PMID:6499137; http://dx.doi.org/10.1161/01.RES.55.6.825
- James RS, Young IS, Cox VM, Goldspink DF, Altringham JD. Isometric and isotonic muscle properties as determinants of work loop power output. Pflugers Arch 1996; 432:767-74; PMID:8772125; http://dx.doi.org/10.1007/s004240050197
- Markhasin VS, Solovyova O, Katsnelson LB, Protsenko Y, Kohl P, Noble D. Mechano-electric interactions in heterogeneous myocardium: development of fundamental experimental and theoretical models. Prog Biophys Mol Biol 2003; 82:207-20; PMID:12732280; http://dx.doi.org/10.1016/S0079-6107(03)00017-8
- Karabag T, Aydin M, Dogan SM, Sayin MR, Cetiner MA. The influence of circadian variations on echocardiographic parameters in healthy people. Echocardiography 2011; 28:612-8; PMID:21676017; http://dx.doi.org/10.1111/j.1540-8175.2011.01411.x
- Tei C, Nishimura RA, Seward JB, Tajik AJ. Noninvasive Doppler-derived myocardial performance index: correlation with simultaneous measurements of cardiac catheterization measurements. J Am Soc Echocardiogr 1997; 10:169-78; PMID:9083973; http://dx.doi.org/10.1016/S0894-7317(97)70090-7
- Pfeffer MA, Pfeffer JM, Fishbein MC, Fletcher PJ, Spadaro J, Kloner RA, Braunwald E. Myocardial infarct size and ventricular function in rats. Circ Res 1979; 44:503-12; PMID:428047; http://dx.doi.org/10.1161/01.RES.44.4.503
- Hayward R, Hydock DS. Doxorubicin cardiotoxicity in the rat: an in vivo characterization. J Am Assoc Lab Anim Sci 2007; 46:20-32; PMID:17645292
- Anderson PAW, Greig A, Mark TM, Malouf NN, Oakeley AE, Ungerleider RM, Allen PD, Kay BK. Molecular basis of human cardiac troponin T isoforms expressed in the developing, adult, and failing heart. Circ Res 1995; 76:681-6; PMID:7534662; http://dx.doi.org/10.1161/01.RES.76.4.681
- Periasamy M, Huke S. SERCA pump level is a critical determinant of Ca(2+)homeostasis and cardiac contractility. J Mol Cell Cardiol 2001; 33:1053-63; PMID:11444913; http://dx.doi.org/10.1006/jmcc.2001.1366
- Kim S-J, Abdellatif M, Koul S, Crystal GJ. Chronic treatment with insulin-like growth factor I enhances myocyte contraction by upregulation of Akt-SERCA2a signaling pathway. Am J Physiol Heart Circ Physiol 2008; 295:H130-5; PMID:18456736; http://dx.doi.org/10.1152/ajpheart.00298.2008
- Chaanine AH, Hajjar RJ. AKT signalling in the failing heart. Eur J Heart Fail 2011; 13:825-9; PMID:21724622; http://dx.doi.org/10.1093/eurjhf/hfr080
- Tandon N, Marsano A, Maidhof R, Wan L, Park H, Vunjak-Novakovic G. Optimization of electrical stimulation parameters for cardiac tissue engineering. J Tissue Eng Regen Med 2011; 5:e115-25; PMID:21604379; http://dx.doi.org/10.1002/term.377
- Black LD 3rd, Meyers JD, Weinbaum JS, Shvelidze YA, Tranquillo RT. Cell-induced alignment augments twitch force in fibrin gel-based engineered myocardium via gap junction modification. Tissue Eng Part A 2009; 15:3099-108; PMID:19338433; http://dx.doi.org/10.1089/ten.tea.2008.0502
- Stehle R, Iorga B. Kinetics of cardiac sarcomeric processes and rate-limiting steps in contraction and relaxation. J Mol Cell Cardiol 2010; 48:843-50; PMID:20060002; http://dx.doi.org/10.1016/j.yjmcc.2009.12.020
- Yano M, Ikeda Y, Matsuzaki M. Altered intracellular Ca2+ handling in heart failure. J Clin Invest 2005; 115:556-64; PMID:15765137; http://dx.doi.org/10.1172/JCI24159
- Pieske B, Houser SR. [Na+]i handling in the failing human heart. Cardiovasc Res 2003; 57:874-86; PMID:12650866; http://dx.doi.org/10.1016/S0008-6363(02)00841-6
- Pervolaraki E, Anderson RA, Benson AP, Hayes-gill B, Holden AV, Moore BJR, Paley MN, Zhang H. Antenatal architecture and activity of the human heart Antenatal architecture and activity of the human heart. Interface Focus 2013
- Sedmera D. Function and form in the developing cardiovascular system. Cardiovasc Res 2011; 91:252-9; PMID:21367775; http://dx.doi.org/10.1093/cvr/cvr062
- Hirota A, Kamino K, Komuro H, Sakai T, Yada T. Early events in development of electrical activity and contraction in embryonic rat heart assessed by optical recording. J Physiol 1985; 369:209-27; PMID:4093880
- Bruch C, Schmermund A, Dagres N, Katz M, Bartel T, Erbel R. Severe aortic valve stenosis with preserved and reduced systolic left ventricular function: diagnostic usefulness of the Tei index. J Am Soc Echocardiogr 2002; 15:869-76; PMID:12221402; http://dx.doi.org/10.1067/mje.2002.120977
- Kono M, Kisanuki A, Takasaki K, Nakashiki K, Yuasa T, Kuwahara E, Mizukami N, Uemura T, Kubota K, Ueya N, et al. Left ventricular systolic function is abnormal in diastolic heart failure: re-assessment of systolic function using cardiac time interval analysis. J Cardiol 2009; 53:437-46; PMID:19477388; http://dx.doi.org/10.1016/j.jjcc.2009.02.014
- Gaasch WH, Little WC. Assessment of left ventricular diastolic function and recognition of diastolic heart failure. Circulation 2007; 116:591-3; PMID:17679627; http://dx.doi.org/10.1161/CIRCULATIONAHA.107.716647
- Leiva MC, Tolosa JE, Binotto CN, Weiner S, Huppert L, Denis AL, Huhta JC. Fetal cardiac development and hemodynamics in the first trimester. Ultrasound Obstet Gynecol 1999; 14:169-74; PMID:10550875; http://dx.doi.org/10.1046/j.1469-0705.1999.14030169.x
- Mäkikallio K, Jouppila P, Räsänen J. Human fetal cardiac function during the first trimester of pregnancy. Heart 2005; 91:334-8; PMID:15710713; http://dx.doi.org/10.1136/hrt.2003.029736
- Friedman D, Buyon J, Kim M, Glickstein JS. Fetal cardiac function assessed by Doppler myocardial performance index (Tei Index). Ultrasound Obstet Gynecol 2003; 21:33-6; PMID:12528158; http://dx.doi.org/10.1002/uog.11
- Moreno R, Zamorano J, Almería C, Pérez-González JA, Mataix L, Rodrigo JL, Herrera D, Aubele A, Pérez de Isla L, De Marco E, et al. Isovolumic contraction time by pulsed-wave Doppler tissue imaging in aortic stenosis. Eur J Echocardiogr 2003; 4:279-85; PMID:14611823; http://dx.doi.org/10.1016/S1525-2167(03)00009-X
- Dorn GW 2nd, Robbins J, Ball N, Walsh RA. Myosin heavy chain regulation and myocyte contractile depression after LV hypertrophy in aortic-banded mice. Am J Physiol 1994; 267:H400-5; PMID:8048605
- Ross J Jr. Cardiac function and myocardial contractility: a perspective. J Am Coll Cardiol 1983; 1:52-62; PMID:6826945; http://dx.doi.org/10.1016/S0735-1097(83)80010-2
- Duzenli MA, Ozdemir K, Aygul N, Soylu A, Aygul MU, Gök H. Comparison of myocardial performance index obtained either by conventional echocardiography or tissue Doppler echocardiography in healthy subjects and patients with heart failure. Heart Vessels 2009; 24:8-15; PMID:19165562; http://dx.doi.org/10.1007/s00380-008-1069-2
- Cutler MJ, Jeyaraj D, Rosenbaum DS. Cardiac electrical remodeling in health and disease. Trends Pharmacol Sci 2011; 32:174-80; PMID:21316769; http://dx.doi.org/10.1016/j.tips.2010.12.001
- Porciani MC, Dondina C, Macioce R, Demarchi G, Cappelli F, Lilli A, Pappone A, Ricciardi G, Colombo PC, Padeletti M, et al. Temporal variation in optimal atrioventricular and interventricular delay during cardiac resynchronization therapy. J Card Fail 2006; 12:715-9; PMID:17174233; http://dx.doi.org/10.1016/j.cardfail.2006.08.001