Abstract
The cerebral cortex is the center of our highest cognitive function, allowing the capacity for complex thought, language, and multiple other uniquely human characteristics. The mature cerebral cortex is organized in both the radial (inside to out) dimension into cortical layers as well as orthogonally into functional areas. In the radial dimension, cortical neurons reside in six functionally and morphologically distinct layers whose neurons differ in morphology, neurotransmitter use, and connectivity. In the orthogonal or tangential dimension, the cortex is also organized into functional areas that subserve distinct functions such as somatosensory, motor, visual, and higher association functions. Studies investigating the patterning of the cerebral cortex in this tangential dimension have revealed a complex interplay of discrete patterning centers serving to organize cell identity by the establishment of multiple opposing gradients of signaling molecules and transcription factors (Sur and Rubenstein, 2005). Relatively less is known about the molecules that pattern the cerebral cortex in the radial dimension. This review focuses on the role of the canonical Wnt/β-catenin signaling pathway in radial organization of the cerebral cortex.
Introduction
The cerebral cortex is the center of our highest cognitive function, allowing the capacity for complex thought, language and multiple other uniquely human characteristics. The mature cerebral cortex is organized in both the radial (inside to out) dimension into cortical layers as well as orthogonally into functional areas. In the radial dimension, cortical neurons reside in six functionally and morphologically distinct layers whose neurons differ in morphology, neurotransmitter use and connectivity. In the orthogonal or tangential dimension, the cortex is also organized into functional areas that subserve distinct functions such as somatosensory, motor, visual and higher association functions. Studies investigating the patterning of the cerebral cortex in this tangential dimension have revealed a complex interplay of discrete patterning centers serving to organize cell identity by the establishment of multiple opposing gradients of signaling molecules and transcription factors.Citation1 Relatively less is known about the molecules that pattern the cerebral cortex in the radial dimension. This review focuses on the role of the canonical Wnt/β-catenin signaling pathway in radial organization of the cerebral cortex.
Canonical Wnt/β-catenin Signaling
Canonical Wnt signaling is defined by the stabilization of β-catenin subsequent to Wnt stimulation. β-catenin is an integral component of the adherens junction,Citation2 that interacts with proteins of the T cell factor/lymphoid enhancer binding factor (TCF/LEF) family to transduce Wnt binding to cell-surface Frizzled receptors.Citation3 β-catenin binding to TCF/LEF converts these transcriptional repressors into activators and results in the upregulation of a variety of genes important in a wide variety of developmental events.Citation2,Citation3
Wnt binding to its receptors initiates a cascade of events that culminate in the accumulation of cytosolic β-catenin. In the absence of Wnt signaling, β-catenin is rapidly degraded. A complex of proteins that include Disheveled (Dsh) Adenomatous Polyposis Coli (APC) and Axin enable the phosphorylation of the N-terminus of β-catenin by glycogen synthase kinase 3β (GSK-3β). Phosphorylated β-catenin is then tagged with ubiquitin and degraded by the cell. Upon binding of secreted Wnt to cell surface receptors of the Frizzled family and LRP co-receptors, the degradation machinery is inhibited, allowing for the accumulation of β-catenin in the cytosol and nucleus. Nuclear β-catenin can then interact with a DNA-binding protein of the TCF/LEF family to turn on the transcription of specific genes. The normal requirement for Wnt signaling can be bypassed with N-terminally-truncated forms of β-catenin that eliminate the phosphorylation sites that target β-catenin for degradation. These truncated forms of β-catenin are stabilized constitutively in vivo yet can still function as transcription factors.Citation3
Stabilization of β-catenin results in persistent activation of signaling, which can increase expression of genes that drive proliferation such as cyclin D1Citation4,Citation5 and c-myc.Citation6 Mutations in APC have been found in most colon cancers, and inappropriate activation of β-catenin have been found in a variety of other human cancers (reviewed in ref. Citation7). Expression of stabilized β-catenin in colonic epithelial cells stimulates proliferation,Citation8 and expression in skin causes hair tumors and de novo hair follicle formation.Citation9 Genetic defects that cause β-catenin stabilization are implicated in melanoma progression.Citation10
Wnt/β-catenin Signaling in Neural Development
A series of fundamental developmental processes create the underlying neuronal scaffolding that enables the complex cognitive functions of the cerebral cortex. The production and differentiation of the enormous diversity of cells of the nervous system span a series of crucial events, from the regional specification of progenitors, division and expansion of neural precursor populations, generation of neurons, migration of young neurons to appropriate positions, elaboration of neuronal processes, and formation of specific synaptic connections. The following sections will describe the role of Wnt signaling in cerebral cortical development.
Wnts and Regional Specification of the Cortex
In neural development, Wnt/β-catenin signaling regulates a wide variety of patterning events (). In forebrain development, a growing literature suggests that patterning centers regulate development along the rostral-caudal and dorsal-ventral axes.Citation11 In the developing cerebral cortex, a caudomedial structure called the cortical hem is proposed to serve as a vital dorsal signaling center with Wnt3a, 5a, 2b, 7b and 8b expressed in the cortical hem.Citation12 In contrast, Wnt7a is not expressed in the cortical hem, but is expressed in the adjacent cortical ventricular zone. Loss of Wnt3a causes underproliferation and loss of the hippocampus,Citation13 while a Lef1-lacZ mediated inactivation of LEF/TCF signaling results in loss of the entire hippocampus.Citation14 Together, these studies support the proposal that a complex pattern of Wnt signaling controls the rostral-caudal and medial-lateral tissue patterning in the developing telencephalon.
Canonical Wnt/β-catenin signaling also regulates the overall dorsal-ventral patterning of the telencephalon, and the cerebral cortex arises in development from the dorsal telencephalon. Wnt signaling is required to generate dorsal telencephalic cells in chick explanted cultures, with Wnt signaling initially serving to block Shh ventral signals, and subsequent Wnt and FGF signals inducing later definitive dorsal telencephalic cells.Citation15 In the developing mouse brain, conditional inactivation of β-catenin in the telencephalon before the onset of neurogenesis causes ventralization, with upregulation of ventral markers Gsh2, Mash1 and Dlx2, and corresponding down-regulation of dorsal markers Emx1, Emx2 and Ngn2.Citation16 Furthermore, overexpression of activated β-catenin causes the opposite effect, and expansion of dorsal identities and repression of ventral identities.Citation16
Wnt Signaling in Radial Dimension of the Cerebral Cortex
The role of Wnt/β-catenin signaling in regulation of the radial dimension of the developing cerebral cortex may parallel its function in dorsal-ventral brain patterning. In the developing cortex, a variety of Wnt genes, Frizzled family receptors, as well as members of the Secreted Frizzled Related protein (SFRP) family of Wnt inhibitors are expressed in overlapping patterns.Citation12,Citation17 Furthermore, specific Wnts and their receptors are expressed in distinct radial compartments in the developing cortex. The cortical ventricular zone highly expresses mFz-5, mFz-8, SFRP-1Citation17 as well as Wnt7aCitation12 and β-catenin.Citation18 In contrast, Wnt7b is absent from the ventricular zone and expressed in the intermediate zone and developing cortical plate.Citation19,Citation20
A number of recent studies provide evidence that cortical ventricular zone precursors exhibit canonical Wnt/β-catenin signaling. Reporter mice in which the LacZ reporter is driven by a series of Wnt/TCF consensus binding elements and a minimal promoter show evidence that canonical Wnt/β-catenin signaling is present in a developmental gradient matching the gradient of maturation across the developing cortex.Citation21 In utero electroporation of a similar reporter construct driving the expression of a destabilized GFP suggested that signaling is largely restricted to the neural precursors within the ventricular zone proper, and that upon cellular exit from the ventricular zone, signaling is reduced.Citation22 Observations that active signaling is present in the developing cortical plate raises the possibility that Wnt signaling also plays roles in the developing the cortical plate as well as in ventricular zone progenitors.Citation21,Citation22
Wnt Signaling in Proliferation of Cortical Progenitors
The neurons of the cortical plate arise from the ventricular zone, a population of multipotent precursor cells that line the lateral ventricles of the developing telencephalon (). These neural precursors can divide in three basic ways: (1) symmetrically to generate two new precursor cells; these divisions serve to expand the precursor pool; (2) asymmetrically to generate one precursor and one postmitotic daughter neuron, and (3) symmetrically to generate two differentiated daughters; these symmetric terminal divisions are thought to deplete the precursor pool at the close of neurogenesis (Caviness et al., 1995b). It has been suggested that minor changes in the relative production of precursors and neurons could produce dramatic increases in neuronal number (Caviness et al., 1995a; Rakic, 1995).
Recent studies provide evidence that specification of neural precursor identity is complex; distinct subpopulations of precursors have been identified, with precursors residing both in the ventricular zone and in the later-developing subventricular zone.Citation23–Citation25 In addition to the simple modes of cell division described earlier (symmetric proliferative, symmetric terminal and asymmetric), a more complex two-step pattern of neuronal generation is believed to arise in cortical development as neuroepithelial precursors develop into radial glial cells, which possess neural stem cell characteristics.Citation26 Radial glia cells can divide asymmetrically in a stem-cell mode to generate one radial glial cell and one intermediate progenitor, which then divides symmetrically in the subventricular zone to generate two postmitotic neurons.Citation27 The observations that neural stem cells and radial glial cells reside in the ventricular zone, while intermediate amplifying progenitors reside outside suggest the possibility that the ventricular zone serves a distinct regulatory function in neural stem cell development.
The function of canonical Wnt/β-catenin signaling in cortical development has been investigated with a variety of different in vivo approaches. Overexpression of a stabilized β-catenin in transgenic neural precursors causes massive expansion of the cerebral cortex.Citation18 The overgrowth of the cortex in this example results from increased cell cycle re-entry of transgenic precursors and a subsequent over-production of precursors. A recent study supported the function of canonical Wnt/β-catenin signaling by a similar overexpression of a β-catenin-Lef1 fusion that served to activate canonical targets.Citation21 While these overexpression studies suggested a simple model that Wnt/β-catenin signaling served to maintain the progenitor state, loss of function studies of signaling were complicated by the dual role of β-catenin in cellular adhesion.
Specific examination of Wnt signaling in cortical development required different approaches. Focal in utero electroporation of crerecombinase in a mouse homozygous for a floxed allele of β-catenin allowed examination of β-catenin function in an otherwise intact epithelium.Citation22 Focal loss of β-catenin caused cells to prematurely exit the ventricular zone and migrate to the developing cortical plate. Further studies overexpressing specific inhibitors of Wnt/β-catenin signaling (a dominant negative TCF4 or ICAT) revealed that cell-autonomous Wnt/β-catenin signaling plays important roles in maintaining cortical precursor identity.Citation22 Loss of β-catenin or inhibition of β-catenin-mediated signaling causes premature cell cycle exit and subsequent neuronal differentiation.
Together these gain and loss of function studies suggest that one key function of canonical Wnt/β-catenin signaling in the radial development of the cerebral cortex is to maintain neural progenitor phenotype. While overactivation of signaling causes decreased cell cycle exit and decreased differentiation, reduction of signaling results in premature cell cycle exit and increased neuronal differentiation. While this model of Wnt/β-catenin function in cortical precursors is attractive, it does not address whether additional complexities of cortical development, such as the control of laminar identity, are influenced by Wnts.
Wnt Signaling in Laminar Fate Decisions
Thymidine birthdating studies have shown that the neuronal layers of the cerebral cortex are generated in an inside-first, outside-last pattern during development. This initial observation that laminar fate is related to time of cell cycle led to experiments that tested whether neural precursors followed an intrinsic developmental clock or responded to changing environmental signals during development. Transplantation experiments suggested that early precursors are transplanted during S-phase, they are able to adopt a laminar fate appropriate for the host into which they are transplanted (McConnell). In contrast, older cortical precursors underwent progressive restriction in their potential, becoming unable to produce earlier-generated cortical layers. While these experiments suggest that the microenvironment in which precursor cells undergo their terminal mitosis regulates their laminar fate choice, recent experiments tracking the ordered generation of neurons in vitro suggest that cortical precursors follow an cell-intrinsic timing mechanism.Citation28 Knockdown of the FoxG1 transcriptional repressor allowed older precursors to re-establish earlier potential to a limited degree. One clue that Wnt signaling might regulate cortical phenotypes came from observations that FoxG1 might normally serve to downregulate Wnt genes in the developing cortex.Citation29 Together, these studies suggest that neural precursors, while capable of adopting laminar phenotypes by responding to environmental signals, follow a cell-intrinsic timing mechanism that progressively restricts their fate decisions.
One of the earliest suggestions that Wnt signaling functions in laminar fate decisions was the description of a mouse mutant in the Wnt co-receptor LRP6. These mice were found to have a dramatically thinner cortical plate and a reduction in the numbers of layer 6 and layers 2–4 neurons, while the neurons in layer 5 were apparently spared.Citation30 Examination of transgenic mice overexpressing stabilized β-catenin suggested that overactivation of signaling delayed the maturation of radial glia into intermediate progenitors.Citation31 As a result of this relative delay in development, transgenic mice did not develop a distinct subventricular zone or superficial cortical layers. Overexpression of β-catenin by in utero electroporation showed reduction in the production of later-born (upper-layer) laminar types, and a relative increase in deeper layer neurons, providing further evidence that cell-autonomous Wnt/β-catenin signaling functioned in laminar fate decisions during cortical development.
Even within a particular layer of the cortex, neurons can project to distinctly different targets. For example, neurons in cortical layer 5 can project across the corpus callosum to the contralateral hemisphere or subcortically to the spinal cord. Recent studies have isolated molecular determinants that appear to identify these specific subtypes of projection neurons,Citation32 and loss of function of some of these genes disrupt the specification of certain classes of projection neurons.Citation32–Citation34 Interestingly, in the zebrafish forebrain, fezl appears to be regulated by Wnt signaling, with fezl upregulated in embryos expressing the Wnt inhibitor Dkk1 and reduced with overexpression of Wnt8b.Citation35 Whether Wnt/β-catenin signaling regulates the acquisition of specific laminar phenotypes in cortical development remains unknown.
Later Events Mediated by Wnt/β-catenin Signaling
Examination of transgenic reporter miceCitation21 and expression from electroporated reporter constructsCitation22 suggest that in addition to signaling in neural precursors, mature cortical plate neurons also exhibit canonical Wnt/β-catenin signaling. Studies of the developing cerebellum suggest that Wnt7a regulates axon and growth cone remodeling in cerebellar mossy fibers as well as early events in synaptic formation.Citation36
Studies in the spinal cord provide additional evidence that Wnts regulate axonal and dendritic remodeling. Wnt3, expressed in lateral motor neurons, causes axon branching and regulates growth cone size in specific subclasses of sensory neurons that contact the motor neurons.Citation37 These studies suggest that Wnt3 acts as a retrograde signal that regulates branching and arborization when these sensory neuron contacts their target motor neurons. Wnt7b, expressed in the mouse hippocampus and cortical plate, increases dendritic branching in cultured hippocampal neurons.Citation38
More recent studies have suggested that Wnts function to coordinate development of the pre-and postsynaptic compartment,Citation39 as well as neurotransmitter release.Citation40 Additional studies in C. elegans suggest that Wnts may spatially regulate the patterning of synaptic connections.Citation41 While the function of Wnt/β-catenin signaling in post-mitotic cerebral cortical neurons remains somewhat unclear, together these studies suggest that Wnt/β-catenin signaling is likely to have significant function in later events in establishing or stabilizing the patterns of neuronal communication of cortical neurons.
Wnt Signaling in Primary Brain Cancers
In the developing brain, inactivation of specific Wnts,Citation13,Citation42 TCF/LEF members,Citation14 or β-cateninCitation43 results in specific brain defects, and persistent activation of β-catenin is implicated in a variety of human nervous system cancers.Citation3 A subset of Torcot's syndrome cases, characterized by multiple colorectal ademonas and primary brain tumors, is characterized by mutation of the APC gene, leading to overactivation of the canonical Wnt signaling pathway.Citation44
The evidence for a role for Wnt signaling is most frequently observed in medulloblastoma, a primary brain tumor that resembles cerebellar neural progenitors. Medulloblastoma arise in the fourth ventricle and represent the most frequent (∼30%) of all pediatric brain tumors. They are characterized by primitive, poorly differentiated cells resembling neural precursors,Citation45 and histologically similar tumors (Primitive Neuro Ectodermal Tumors or PNET) can be found at other anatomic locations and may represent a similar developmental process. Activating mutations in β-catenin have been identified in sporadic medulloblastomas.Citation46,Citation47 Deletions and or mutations in APC, Axin1, and Axin2 have been observed in sporadic medulloblastomas, leading to activation of Wnt signaling.Citation48–Citation51 Interestingly, mutation in β-catenin appears to confer an improved prognosis for patients with medulloblastoma.Citation52
While the mechanisms of Wntβ-catenin in primary brain tumors remain unclear, the seemingly paradoxical improved outlook in medulloblastoma may lend insight into Wnt/β-catenin function in this disease. During the development of carcinoma (or cancers of epithelial origin), tumor cells lose many of their original epithelial features and acquire new abilities to invade the epithelial basal lamina and become migratory. This process resembles a phenomenon seen in normal development of organs called epithelial to mesenchymal transition (EMT).Citation53 Recent studies support the idea that many of the mechanisms governing normal EMT may also regulate the changes believed to occur during tumor development.Citation53 Focal inhibition of β-catenin signaling from cortical neural precursors in vivo causes premature exit from the ventricular zone and migration.Citation22 Furthermore, transgenic mice expressing a stabilized β-catenin in neural precursors develop enlarged brains as a result of delayed neural precursor differentiation, with prolonged persistence of the ventricular zone precursor phenotype.Citation18,Citation31 Together, these studies suggest the possibility that β-catenin signaling may maintain the epithelial neural precursor phenotype and inhibit EMT both in development and medulloblastoma.
Canonical Wnt/β-catenin signaling functions in a wide variety of neural developmental processes. In addition to employing its well known function in regulating cell proliferation, the developing nervous system uses Wnts in many other roles ranging from cell fate specification to axonal/dendritic and synaptic remodeling. Understanding Wnt signaling's many diverse functions during normal neural development should undoubtedly provide greater insight into the mechanisms underlying the development and progression of neural tumors.
Figures and Tables
Figure 1 Expression of Wnts, Frizzled and Wnt activity in the developing cerebral cortex. Neural precursor cells reside in the ventricular zone (VZ). Ventricular cells are polarized neuroepithelial cells oriented with their apical surfaces towards the ventricular lumen; the endfeet of ventricular zone neural precursors are joined by β-catenin-rich adherens junctions (red). Wnt7a, Fz-5 and Fz-8 are expressed in the cortical ventricular zone, while Wnt7b is expressed in the developing cortical plate. Active Wnt/β-catenin signaling is present in the ventricular zone and cortical plate (blue). Increasing evidence points to important Wnt function in proliferation of precursors, laminar fate determination of cortical neurons, axonal/dendritic remodeling, as well as synapse formation.
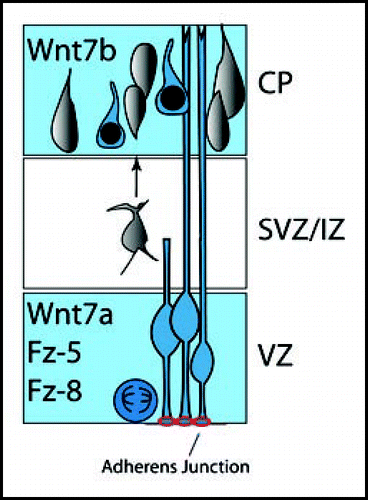
Table 1 CNS phenotype of Wnt knockouts
References
- Sur M, Rubenstein JL. Patterning and plasticity of the cerebral cortex. Science 2005; 310:805 - 810
- Cox RT, Kirkpatrick C, Leifer M. Armadillo is required for adherens junction assembly, cell polarity, and morphogenesis during Drosophila embryogenesis. J Cell Biology 1996; 134:133 - 148
- Peifer M, Polakis P. Wnt signaling in oncogenesis and embryogenesis—a look outside the nucleus. Science 2000; 287:1606 - 1609
- Shtutman M, Zhurinsky J, Simcha I, Albanese C, D'Amico M, Pestell R, Ben-Ze'ev A. The cyclin D1 gene is a target of the beta-catenin/LEF-1 pathway. Proc Natl Acad Sci USA 1999; 96:5522 - 5527
- Tetsu O, McCormick F. Beta-catenin regulates expression of cyclin D1 in colon carcinoma cells. Nature 1999; 398:422 - 426
- He TC, Sparks AB, Rago C, Hermeking H, Zawel L, da Costa LT, Morin PJ, Vogelstein B, Kinzler KW. Identification of c-MYC as a target of the APC pathway. Science 1998; 281:1509 - 1512 [see comments]
- Polakis P. Wnt signaling and cancer. Genes Dev 2000; 14:1837 - 1851
- Wong MH, Hermiston ML, Syder AJ, Gordon JI. Forced expression of the tumor suppressor adenomatosis polyposis coli protein induces disordered cell migration in the intestinal epithelium. Proc Natl Acad Sci USA 1996; 93:9588 - 9593
- Gat U, DasGupta R, Degenstein L, Fuchs E. De Novo hair follicle morphogenesis and hair tumors in mice expressing a truncated beta-catenin in skin. Cell 1998; 95:605 - 614
- Rubinfeld B, Robbins P, El-Gamil M, Albert I, Porfiri E, Polakis P. Stabilization of beta-catenin by genetic defects in melanoma cell lines. Science 1997; 275:1790 - 1792 [see comments]
- O'Leary DD, Chou SJ, Sahara S. Area patterning of the Mammalian cortex. Neuron 2007; 56:252 - 269
- Grove EA, Tole S, Limon J, Yip L, Ragsdale CW. The hem of the embryonic cerebral cortex is defined by the expression of multiple Wnt genes and is compromised in Gli3-deficient mice. Development 1998; 125:2315 - 2325
- Lee SM, Tole S, Grove E, McMahon AP. A local Wnt-3a signal is required for development of the mammalian hippocampus. Development 2000; 127:457 - 467
- Galceran J, Miyashita-Lin EM, Devaney E, Rubenstein JL, Grosschedl R. Hippocampus development and generation of dentate gyrus granule cells is regulated by LEF1. Development 2000; 127:469 - 482
- Gunhaga L, Marklund M, Sjodal M, Hsieh JC, Jessell TM, Edlund T. Specification of dorsal telencephalic character by sequential Wnt and FGF signaling. Nat Neurosci 2003; 6:701 - 707
- Backman M, Machon O, Mygland L, van den Bout CJ, Zhong W, Taketo MM, Krauss S. Effects of canonical Wnt signaling on dorso-ventral specification of the mouse telencephalon. Dev Biol 2005; 279:155 - 168
- Kim AS, Lowenstein DH, Pleasure SJ. Wnt receptors and Wnt inhibitors are expressed in gradients in the developing telencephalon. Mech Dev 2001; 103:167 - 172
- Chenn A, Walsh CA. Regulation of cerebral cortical size by control of cell cycle exit in neural precursors. Science 2002; 297:365 - 369
- Funatsu N, Inoue T, Nakamura S. Gene expression analysis of the late embryonic mouse cerebral cortex using DNA microarray: identification of several region- and layer-specific genes. Cereb Cortex 2004; 14:1031 - 1044
- Rubenstein JL, Anderson S, Shi L, Miyashita-Lin E, Bulfone A, Hevner R. Genetic control of cortical regionalization and connectivity. Cereb Cortex 1999; 9:524 - 532
- Machon O, Backman M, Machonova O, Kozmik Z, Vacik T, Andersen L, Krauss S. A dynamic gradient of Wnt signaling controls initiation of neurogenesis in the mammalian cortex and cellular specification in the hippocampus. Dev Biol 2007; 311:223 - 237
- Woodhead GJ, Mutch CA, Olson EC, Chenn A. Cell-Autonomous beta-Catenin Signaling Regulates Cortical Precursor Proliferation. J Neurosci 2006; 26:12620 - 12630
- Haubensak W, Attardo A, Denk W, Huttner WB. Neurons arise in the basal neuroepithelium of the early mammalian telencephalon: a major site of neurogenesis. Proc Natl Acad Sci USA 2004; 101:3196 - 3201
- Noctor SC, Martinez Cerdeno V, Ivic L, Kriegstein AR. Cortical neurons arise in symmetric and asymmetric division zones and migrate through specific phases. Nat Neurosci 2004; 7:136 - 144
- Miyata T, Kawaguchi A, Saito K, Kawano M, Muto T, Ogawa M. Asymmetric production of surface-dividing and non-surface-dividing cortical progenitor cells. Development 2004; 131:3133 - 3145
- Noctor SC, Flint AC, Weissman TA, Dammerman RS, Kriegstein AR. Neurons derived from radial glial cells establish radial units in neocortex. Nature 2001; 409:714 - 720
- Kriegstein A, Noctor S, Martinez Cerdeno V. Patterns of neural stem and progenitor cell division may underlie evolutionary cortical expansion. Nat Rev Neurosci 2006; 7:883 - 890
- Shen Q, Wang Y, Dimos JT, Fasano CA, Phoenix TN, Lemischka IR, Ivanova NB, Stifani S, Morrisey EE, Temple S. The timing of cortical neurogenesis is encoded within lineages of individual progenitor cells. Nat Neurosci 2006; 9:743 - 751
- Muzio L, Mallamaci A. Foxg1 confines Cajal-Retzius neuronogenesis and hippocampal morphogenesis to the dorsomedial pallium. J Neurosci 2005; 25:4435 - 4441
- Zhou CJ, Borello U, Rubenstein JL, Pleasure SJ. Neuronal production and precursor proliferation defects in the neocortex of mice with loss of function in the canonical Wnt signaling pathway. Neuroscience 2006; 142:1119 - 1131
- Wrobel CN, Mutch CA, Swaminathan S, Taketo MM, Chenn A. Persistent expression of stabilized beta-catenin delays maturation of radial glial cells into intermediate progenitors. Dev Biol 2007; 309:285 - 297
- Arlotta P, Molyneaux BJ, Chen J, Inoue J, Kominami R, Macklis JD. Neuronal subtype-specific genes that control corticospinal motor neuron development in vivo. Neuron 2005; 45:207 - 221
- Molyneaux BJ, Arlotta P, Hirata T, Hibi M, Macklis JD. Fezl is required for the birth and specification of corticospinal motor neurons. Neuron 2005; 47:817 - 831
- Chen B, Schaevitz LR, McConnell SK. Fezl regulates the differentiation and axon targeting of layer 5 subcortical projection neurons in cerebral cortex. Proc Natl Acad Sci USA 2005; 102:17184 - 17189
- Hashimoto H, Yabe T, Hirata T, Shimizu T, Bae Y, Yamanaka Y, Hirano T, Hibi M. Expression of the zinc finger gene fez-like in zebrafish forebrain. Mech Dev 2000; 97:191 - 195
- Hall AC, Lucas FR, Salinas PC. Axonal remodeling and synaptic differentiation in the cerebellum is regulated by WNT-7a signaling. Cell 2000; 100:525 - 535
- Krylova O, Herreros J, Cleverley KE, Ehler E, Henriquez JP, Hughes SM, Salinas PC. WNT-3, expressed by motoneurons, regulates terminal arborization of neurotrophin-3-responsive spinal sensory neurons. Neuron 2002; 35:1043 - 1056
- Rosso SB, Sussman D, Wynshaw-Boris A, Salinas PC. Wnt signaling through Dishevelled, Rac and JNK regulates dendritic development. Nat Neurosci 2005; 8:34 - 42
- Packard M, Koo ES, Gorczyca M, Sharpe J, Cumberledge S, Budnik V. The Drosophila Wnt, wingless, provides an essential signal for pre- and postsynaptic differentiation. Cell 2002; 111:319 - 330
- Ahmad Annuar A, Ciani L, Simeonidis I, Herreros J, Fredj NB, Rosso SB, Hall A, Brickley S, Salinas PC. Signaling across the synapse: a role for Wnt and Dishevelled in presynaptic assembly and neurotransmitter release. J Cell Biol 2006; 174:127 - 139
- Klassen MP, Shen K. Wnt signaling positions neuromuscular connectivity by inhibiting synapse formation in C. elegans. Cell 2007; 130:704 - 716
- McMahon AP, Bradley A. The Wnt-1 (int-1) proto-oncogene is required for development of a large region of the mouse brain. Cell 1990; 62:1073 - 1085
- Brault V, Moore R, Kutsch S, Ishibashi M, Rowitch DH, McMahon AP, Sommer L, Boussadia O, Kemler R. Inactivation of the beta-catenin gene by Wnt1-Cre-mediated deletion results in dramatic brain malformation and failure of craniofacial development. Development 2001; 128:1253 - 1264
- Hamilton SR, Liu B, Parsons RE, Papadopoulos N, Jen J, Powell SM, Krush AJ, Berk T, Cohen Z, Tetu B, et al. The molecular basis of Turcot's syndrome. N Engl J Med 1995; 332:839 - 847 [see comments]
- Louis DN, Ohgaki H, Wiestler OD, Cavenee WK, Burger PC, Jouvet A, Scheithauer BW, Kleihues P. The 2007 WHO classification of tumours of the central nervous system. Acta Neuropathol 2007; 114:97 - 109
- Eberhart CG, Tihan T, Burger PC. Nuclear localization and mutation of beta-catenin in medulloblastomas. J Neuropathol Exp Neurol 2000; 59:333 - 337
- Zurawel RH, Chiappa SA, Allen C, Raffel C. Sporadic medulloblastomas contain oncogenic beta-catenin mutations. Cancer Res 1998; 58:896 - 899
- Koch A, Hrychyk A, Hartmann W, Waha A, Mikeska T, Waha A, Schuller U, Sorensen N, Berthold F, Goodyer CG, Wiestler OD, Birchmeier W, Behrens J, Pietsch T. Mutations of the Wnt antagonist AXIN2 (Conductin) result in TCF-dependent transcription in medulloblastomas. Int J Cancer 2007; 121:284 - 291
- Baeza N, Masuoka J, Kleihues P, Ohgaki H. AXIN1 mutations but not deletions in cerebellar medulloblastomas. Oncogene 2003; 22:632 - 636
- Dahmen RP, Koch A, Denkhaus D, Tonn JC, Sorensen N, Berthold F, Behrens J, Birchmeier W, Wiestler OD, Pietsch T. Deletions of AXIN1, a component of the WNT/wingless pathway, in sporadic medulloblastomas. Cancer Res 2001; 61:7039 - 7043
- Huang H, Mahler Araujo BM, Sankila A, Chimelli L, Yonekawa Y, Kleihues P, Ohgaki H. APC mutations in sporadic medulloblastomas. Am J Pathol 2000; 156:433 - 437
- Ellison DW, Onilude OE, Lindsey JC, Lusher ME, Weston CL, Taylor RE, Pearson AD, Clifford SC. beta-Catenin status predicts a favorable outcome in childhood medulloblastoma: the United Kingdom Children's Cancer Study Group Brain Tumour Committee. J Clin Oncol 2005; 23:7951 - 7957
- Thiery JP. Epithelial-mesenchymal transitions in tumour progression. Nat Rev Cancer 2002; 2:442 - 454
- McMahon AP, Joyner AL, Bradley A, McMahon JA. The midbrain-hindbrain phenotype of Wnt-1-/Wnt-1- mice results from stepwise deletion of engrailed-expressing cells by 9.5 days postcoitum. Cell 1992; 69:581 - 595
- Ikeya M, Lee SM, Johnson JE, McMahon AP, Takada S. Wnt signalling required for expansion of neural crest and CNS progenitors. Nature 1997; 389:966 - 970
- Lie DC, Colamarino SA, Song HJ, Desire L, Mira H, Consiglio A, Lein ES, Jessberger S, Lansford H, Dearie AR, Gage FH. Wnt signalling regulates adult hippocampal neurogenesis. Nature 2005; 437:1370 - 1375