Abstract
Renal replacement therapy (i.e., kidney transplantation) represents the optimal treatment for end-stage renal disease (a condition which is expected to increase over the next decade or so). However, the demand for transplantable kidneys currently outpaces the availability of donor kidneys, a situation not expected to improve in the foreseeable future. An alternative route to cadaveric or living-related donors would be to engineer kidneys for allograft transplantation from cells based on concepts derived from our current understanding of normal kidney development. Although the use of cells for this purpose remains hypothetical, recent research from our laboratory has provided strong evidence that implantation of kidney-like tissue bio-engineered from the recombination of in vitro culture systems which model discrete aspects of kidney development (i.e., cell culture, isolated WD, isolated UB, and isolated MM) is possible. These recent findings are discussed here. Pathway based system biology approaches to understanding the mechanism(s) of kidney development are also discussed, particularly in the setting of this novel and seemingly powerful xeno-based tissue engineering strategy.
Introduction
Dr. Sanjay Nigam: As the population of the United States continues to age, the prevalence of chronic kidney failure is expected to rise.Citation1 For most patients, hemodialysis is the therapeutic treatment of choice, as it has been for several decades. Although the majority of patients do reasonably well on hemodialysis for years, there are several quality of life issues, as well as long term morbidity and mortality which remain as significant concerns. Although modifications to dialysis, such as the so-called bioartificial kidney, have been proposed,Citation2 kidney transplantation continues to be the best option for improved long term outcomes for some patients suffering from chronic renal failure and end stage renal disease. However, the scarcity of cadaveric and living-related donor kidneys means that the numbers of patients benefiting from such surgical procedures are still far from optimal and represents the main limitation to kidney transplantation.
Over the past several years, improvements in our understanding of normal kidney development and/or its recovery from injury,Citation2–Citation6 together with advances in the fields of developmental biology, regenerative medicine and tissue engineering have led investigators to propose designing/engineering kidney tissue-based transplantable “artificial” organs. Several of these varied approaches, which have stimulated much research and may lead to new renal replacement therapies have been reviewed elsewhere.Citation7 Although, as described above, many labs have made seminal contributions, the research presented today will focus primarily on developmental biology-based approaches recently characterized and currently employed by our group at the University of California, San Diego. This work has utilized in vitro model systems of rodent kidney development and provided a potentially novel and seemingly powerful xeno-based strategy for renal replacement therapy. Moreover, if these kidney-like tissues can be engineered from cultured cell lines, the potential clinical and/or therapeutic implications could be profound.
Although there are many excellent reviews on the subject,Citation7–Citation11 a very brief outline of kidney development is necessary to provide context for the discussion. Metanephric kidney development is initiated with the emergence of the UB from the epithelial WD. The outgrowth of the UB is induced by GDNF produced by the MM. There are also data to suggest that other soluble growth factors (probably produced by mesenchyme cells surrounding the WD) also play a role in modulating/regulating this process (or in compensating for the loss of GDNF) (Maeshima et al. 2007). Following its outgrowth, the UB penetrates the MM where it is induced to undergo several rounds of iterative branching morphogenesis eventually differentiating into the renal collecting system. During this process of branching morphogenesis within the MM, its tips induce the aggregation, condensation and MET of some of the MM cells. These newly formed epithelial cells undergo a series of morphologically recognizable stages of tubulogenesis and will ultimately form the nephron from Bowman's capsule to the proximal convoluted tubule, the loop of Henle and the distal convoluted tubule (including a portion of the segment connecting it to the collecting system). Vascularization occurs through invasion of blood vessels (angiogenesis), but vasculogenesis within the kidney also occurs.
Cell Culture Models for Study of Branching Tubulogenesis and Kidney Regeneration
We originally got into this area through our interest in epithelial morphogenesis, particularly the processes of tubulogenesis and branching morphogenesis. Our initial work focused on cell culture models using three dimensional cultures of mature polarized epithelial cells (MDCK and IMCD cells) which form branching tubular structures when cultured in an appropriate milieu of growth factors and extracellular matrix componentsCitation12–Citation15 (). Questions of the relevance of the 3D culture of these adult epithelial cells to the embryonic aspects of kidney organogenesis eventually lead to the development of additional cell culture model systems using cells derived from the mouse embryonic kidney: A UB cell branching tubulogenesis model that was stimulated by a conditioned medium from a MM-derived cell lineCitation16 () and the operational isolation of a set of cells with progenitor-like properties from the adult kidney.Citation17 These latter cells appeared to be similar or identical to the cells that incorporated BrdU in the proximal tubule of the injured kidney.Citation17 While the major interest was in their role in renal regeneration, it is noteworthy from the perspective tissue engineering and kidney repair that these cells were able to undergo growth factor-induced tubulogenesis and, when microinjected into kidney organ cultures, homed to cell populations of different embryonic derivation. Other groups have also identified similar cells.Citation18
Once again relevance to the in vivo situation from the studies of these two cell culture models for kidney development (MM cell conditioned medium-induced UB cell branching) and (adult progenitor-like cells capable of undergoing tubulogenesis and homing in the embryonic kidney), lead to the development and characterization of two in vitro model systems which allowed for examination, in isolation, of critical components of metanephric kidney development: The isolated WD budding systemCitation19,Citation20 (ureteric bud emergence and growth, ) and the isolated UB systemCitation21 (UB branching morphgenesis and collecting duct differentiation, ). These later two systems have enabled the identification of growth factors, matrix components and other gene products that are necessary for in vitro budding and branching. I will briefly describe each system below.
Isolated Wolffian Duct Culture
Recently, we have demonstrated that the WD can be isolated and cultured independent of tissues of the metanephric kidney.Citation19 When grown in the presence of the appropriate growth factors, budding of the UB can be induced from this cultured WDCitation20 (). The use of this model system has provided evidence that the layers of mesodermal/mesenchymal cells immediately adjacent to the tubular epithelial cells of the WD appear to play an important modulatory/regulatory role in UB outgrowth. Stimulatory and inhibitory growth factors for budding in this in vitro system have been identified and consistent with knockout and other previous data (reviewed in refs. Citation7–Citation11), GDNF is a potent inducer of budding, while BMP2 is inhibitory. Activin is a powerful budding inhibitor in vitro.Citation19 Moreover, we have found a FGF dependent pathway that can bypass GDNF-ret, at least in vitro.Citation22 This is brought out most clearly when activin signaling is inactivated. Whether this holds up in vivo as well remains to be seen, but it could explain why some GDNF knockouts result in rudimentary kidney formation as a result of WD budding.
Isolated Ureteric Bud Culture
About ten years ago, we described the culture of the isolated UB in the absence of cell-cell contact with the MM, a culture model which heretofore was thought not to be possibleCitation21 (). The system was based on analogy to the UB cell branching tubulogenesis system, which responded best when the cells were cultured within a matrix containing Matrigel and in the presence of a MM cell-derived (BSN cell) conditioned medium.Citation16 Robust branching of the isolated UB was obtained when it was cultured in 3D gels composed of the appropriate extracellular matrix and in the presence of BSN conditioned medium supplemented with GDNF. We have purified and/or identified a number of growth factors that stimulate UB branching morphogenesis using this in vitro system. These include pleiotropin,Citation23 several FGFsCitation24 and heregulin.Citation25 We have also identified inhibitory growth factors, including members of the TGFβ superfamily such as activin, BMPs, LIF and TGFβ.Citation26 The UB branching pattern and the shape of the tube appears to be regulated by combinations of stimulatory and inhibitory factorsCitation26 along with extracellular matrix components.Citation20,Citation27,Citation28 This has been a powerful system to help understand the process of branching through budding and promises to be useful in detailing the cell biological events essential to this process.Citation29
Propagation Strategy
Both the isolated WD culture and the isolated UB culture have potential for propagation.Citation20,Citation30 For example, the isolated WD forms multiple buds along its length, each of which can be individually microdissected and propagated by culturing them in a manner similar to the isolated UB culture (). Similarly, the UB undergoes many iterations of branching in culture, and each tip can potentially be micro-dissected and propagated. This has been done for several generations (). Thus, inherent to a successful isolated WD culture and/or isolated UB culture is the possibility of extensive propagation and multiple UB tips can be created from a single tip or UB outgrowth. These propagated UBs can be recombined with MM in a manner similar to that described for the wild type UBCitation7,Citation20,Citation21,Citation30 (). Hence, there is the potential of creating scores of renal-like recombined tissue from a single in vitro induced UB or isolated T-shaped UB. Because of the clonal nature of the process, one can conceivably transfect immunomodulatory genes or genes that enhance function (e.g., toxin transporters) into the original WD or UB, and these potentially could end up being incorporated into each of the daughter renal-like tissues.Citation20,Citation30
Tissue Engineering Strategy
It is hard to imagine a kidney-like tissue with significant function in the absence of appropriate spatial relationships of the nephrons and vasculature, including definition of corticomedullary boundaries. Simply differentiating stem cells into cells with renal markers would, at first glance, appear to be insufficient. Based on the argument that 3D spatial relationships are critical for significant renal function, and that this is inherent in the developmental program, we have been using the in vitro systems we and others have developed to try to “engineer” renal-like tissue with evidence of function and vascularization.
Essentially, it involves trying to recapitulate the majority of the developmental program for kidney organogenesis in vitro. The approach has been recently described elsewhere,Citation20 so it is only outlined here. Basically, it follows our in vitro work. Starting with the isolated WD, one can induce it to form a UB-like bud on a filter in the presence of appropriate growth factors (GDNF, FGFs). Multiple buds form, each of which can be propagated by excising the buds and culturing them in a 3D extracellular matrix gel in the presence of BSN conditioned medium and GDNF. Robust branching follows, and it is equivalent to the native isolated UB culture which starts with the T-shaped UB. The structure can be extracted from the gel and recombined with fresh isolated MM leading to the formation of apparently functional tubular nephrons (). The recombined tissue has gene expression patterns similar to the E17 or E18 kidney and is even capable of organic anion transport into the proximal tubule. This recombined structure—originally beginning with just the WD, and later, growth factors and MM—can then be transplanted under the renal capsule and shows evidence of early vascularization ().
Several points are worth emphasizing: (1) If a pluripotent WD-like tube can be constructed from cells, then appropriate soluble factors could, in the right context be used to guide the cells and structures along the pathway just described; (2) There are points of propagation at several stages; and (3) If many renal-like tissues can be generated from a single cell or set of cells, it may be possible to introduce immunomodulatory or functional genes in all daughter structures.
It is also important to note that, although we have developed a very useful MM cell line (BSN cellsCitation16), we have yet to induce it to interact with the WD or UB. Others have developed such cell lines as well, for example, the RIMM 18 cells.Citation31 However, it remains to be seen whether these cells can serve as MM-like tissue. In addition, an isolated MM culture system has been developed and studied.Citation32 Together, there seems to be the potential on the MM side for a similar strategy to what we have described on the collecting system side.
Efficient vascularization and appropriate mechanical connections are very crucial for full renal function. Our work so far has not gone beyond showing the ability of the in vitro engineered renal tissue to undergo rudimentary vascularization (). Others have, through transplantation of whole metanephric kidneys, shown that the metanephric kidney can be fully vascularized and anastomosed to the ureteral end as well and shown that the whole parcel is capable of in vivo function at the level of a GFR of around 10 ml/min in rats.Citation5,Citation33 Taken together with our own work starting with the WD just described, as well as the cell culture approach discussed below, there does not seem to be any theoretical impediment to making a kidney from one or two basic cell types.
Starting from Cells
If one could start from cells, it might even be better, both from the perspective of transfection and the perspective of issues related to tissue engineering. For one thing, one would not have to worry about finding a suitable WD, say, from pigs or another animal. About fifteen years ago, we demonstrated that the embryonic kidney could, when cocultured atop an extracellular matrix gel seeded with MDCK or IMCD cells, induce robust tubulogenesis and branching of the cellsCitation12 (). The UB cell branching tubulogenesis system described aboveCitation16 as well the homing and tubulogenic properties of the progenitor-like adult kidney cellsCitation17 also described above suggest that cells can be used to construct an epithelial tubule with a pluripotent character. Since one can, as already discussed, get a WD to form renal-like tissue with transport function and the ability to undergo early vascularization upon transplantion under the renal capsuleCitation20 and since the WD is essentially a pluripotent epithelial tube, then perhaps one can start with cells and achieve the appropriate 3D spatial relations necessary for significant renal function. The data we have is tantalizing, but much remains to be proven. Obviously, the same needs to be done on the MM end. The BSN cellsCitation16 and RIMM18 cellsCitation31 suggest the potential, but true MM-like behavior needs to be demonstrated. Of note, mouse embryonic stem cells may differentiate so as to acquire some MM markers,Citation34 but how far they go remains to be seen.
The Usefulness of In Vitro Systems to Develop a Systems Theory of Kidney Organogenesis
Robert O. Stuart, a gifted scientist and nephrologist who trained in our lab, and then, as an Assistant Professor continued to collaborate with us for many years, was the first to do a sophisticated time series analysis of global gene expression during organogenesis with microarrays ().Citation35 We did this work in the embryonic in vivo isolated rat kidney and we subsequently did a similar analysis of gene expression in the in vitro models of rat kidney development, including the isolated UB and the induced (by spinal cord) MM ().Citation36 These data, together with our in vitro and knockout data from many labs, led us to formulate something of a “systems” theory of kidney development. The theory, a preliminary one which may well be flawed, has been discussed elsewhere in detail,Citation29,Citation36–Citation38 but several features are noteworthy: (1) Kidney development proceeds in stages that are mediated largely by short and long-range growth factor interactions with heparin sulfate proteoglycans and other matrix molecules; (2) The networks for each of these stages has a unique “scale-free” architecture, leading to predictions about resiliency to perturbation of each stage. Thus, the UB branching network may be more decentralized than the WD budding network and therefore less amenable to all-or-none perturbation; (3) In the transition from budding to branching, the network becomes “autocatalytic.” This may be mediated by a combination of paracrine and autocrine pathways, some of which are redundant. Thus the UB is largely protected from mutation owing to the redundant mechanisms programmed into autocrine and paracrine processes regulating branching morphogenesis. As an example, there is much overlap between the heparin-binding growth factors (GDNF, FGFs, activin, BMPs, etc.) that stimulate and inhibit budding and branching. There appears to be more growth factor-matrix “redundancy” for UB branching than WD budding, although a GDNF bypass pathway seems likely to exist in the WD;Citation22 (4) Branching stops as a result of inhibitory factors/matrix made by the MM; these switches are presumably cued to MM and collecting duct differentiation. Certain molecules (e.g., hyaluronic acid, LIF, TGFβ, wnts, collagen 18/endostatin) may have the ability to inhibit UB branching while stimulating MM and collecting duct differentiation; and (5) the mesenchyme provides cues (secreted and/or via cell contact) for vectoriality of branching, tapering of collecting ducts and their differentiation.Citation21
Whether these “systems” concepts hold up over the long run remains to be seen. The point is that the combined use of the in vitro systems in the context of in vivo data from knockout animals is beginning to suggest a more general theory of kidney organogenesis. The nature of this organogenesis forum lecture has limited the discussion largely to work from our lab, but our work is indebted to investigators who have done important work in this field; much of this excellent work is cited in several detailed reviews.Citation7–Citation11
Conference Questions and Answers
Have you tried to incorporate the vascular component into your recombination experiments? In other words, you could put in an embryonic aorta and vascularize the nephrons that you are making using the isolated bud and blastema in 3D organ culture ()?
We have tried in the past, but the system wasn't as robust then. We are trying again.
One of the events we don't hear much about is the molecular regulation of the joining of the collecting system with the proper nephron, so I was just wondering if you had any leads or had any models to study this process.
Now that we have several recombination models, we are beginning to ask that question. A number of other labs have, over the years, identified some interesting molecules, but I don't know how those studies have progressed. Here, I have been, of course, almost exclusively been discussing work from our lab.
In the experiments with the recombination of the metanephric mesenchyme and the in vitro grown ureteric bud tree, do you get hook up of the nephron distal tubule with the collecting duct?
Morphologically, this appears to be the case.
I noticed a difference between what you designate as WD+ mesoderm versus the isolated WD (). With the former you observed only one sided branching towards the mesonephric tubules when GDNF was added, but with the latter there was branching in all directions. Is there some inhibitory activity in the ‘dirty’ preparation?
We think that the surrounding cell layers are very crucial to regulating the budding process. In general, however, they are not well characterized. One might expect that the balance of stimulatory and inhibitory factors is very important to the site and degree of budding. In vitro, it appears that budding may require cancellation of these inhibitory activities.
I have a couple of questions. First, the intermediate mesoderm is pre-specified along the anteroposterior axis and this determines the position and the number of the ureters that come out of the Wolffian duct. How about the Wolffian duct epithelium itself? Do you think it has any anteroposterior pre-specification?
We think it ought to, but in most of our experiments we don't tend to see this. Maybe we are actually removing something crucial for this specification in order to perform the type of in vitro studies we're interested in.
My second question is, you probably would like to eventually have a functional ureter in the cultured recombination system for transplantation. Do you see any kind of structures resembling the ureter in your culture system? If there is one, where does the ureteric mesenchyme come from? It usually comes from the more distal end rather than from the metanephric mesenchyme. Also, is there any kind of expression of Tbx18, for example, as a ureteric mesenchyme marker?
We have published data some time ago on development of the ureter in the six-1 knockout. In that study, the knockout ureter was able develop on its own in the absence of much, if any, mesenchyme. This was a surprise to us. However, there may have been an early induction event that was missed. We haven't looked at Tbx18.
If you look at the other side, like in the recombination system using a cut-off ureteric bud, do you see any sign of a ureter with ureteric mesenchyme being developed there?
We haven't yet looked at this carefully. It's an interesting question.
After blocking BMP2 and activin, it seems that isolated ureteric bud is more susceptible to the effect of GDNF. Do you know exactly how it works? Is it because their individual signaling pathways converge at some common point downstream? In other words, how do these positive and negative regulators of epithelial branching morphogenesis interact with each other to decide the final fate of the branching program?
If I understand, you're asking how is the whole thing integrated. That is a great question, and we're hoping over the next few years that our in vitro studies, together with the in vivo studies being performed here by Dr. Jain as well as other groups around the world, will enable one to come up with a detailed answer to your question.
Abbreviations
WD | = | wolffian duct |
UB | = | ureteric bud |
GDNF | = | glial-derived neurotrophic factor |
MM | = | metanephric mesenchyme |
MET | = | mesenchymal-to-epithelial transformation |
BSN cell | = | MM derived cell |
BrdU | = | 5-bromo-2′-deoxyuridine |
Figures and Tables
Figure 1 (A and B) Phase contrast photomicrographs of either (A) mIMCD cells or (B) MDCK cells cocultured for 48 hr with the ed11.5–12.5 embryonic murine kidney (EK) as a source of soluble growth factors. Both cell types are induced to form complex branching tubular structures in the presence of the soluble factors secreted by the embryonic kidney (Bar = 50 µm). (From ref. Citation12).
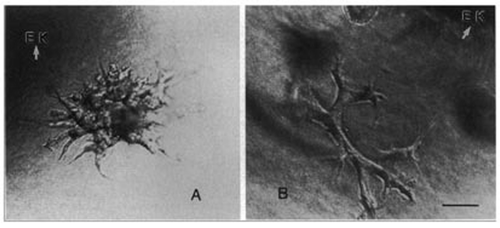
Figure 2 (A and B) Phase contrast photomicrographs of 3D cultures of either (A) mIMCD cells or (B) ureteric bud (UB) cells grown for 48 hr. The cells were grown in the presence of either 3t3 cell conditioned medium (A—source of HGF) or BSN cell conditioned medium supplemented with 0.5% FBS (Bar = 25 µm). (From ref. Citation39).
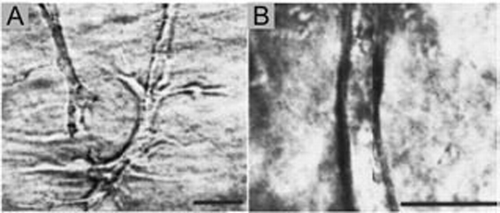
Figure 3 (A–C) Phase contrast photomicrographs of either (A) isolated whole mesonephros, including the Wolffian duct, (B) isolated WD in which the mesonephric tubules, along with most of the non-epithelial mesoderm is removed or (C) isolated WD cleared of all surrounding mesoderm before in vitro culture (“naked” epithelial tube) suspended within a 3D extracellular matrix gel. The cultures were grown for 3 days in either (A and B) DME/F12 supplemented with FBS and soluble grow factors [(A) 10 ng/ml GDNF; (B) 125 ng/ml GDNF and 250 ng/ml FGF1] or (C) BSN conditioned medium supplemented with 125 ng/ml GDNF and 250 ng/ml FGF1 ; (Scale bar: 500 µm.) (From ref. Citation20).
![Figure 3 (A–C) Phase contrast photomicrographs of either (A) isolated whole mesonephros, including the Wolffian duct, (B) isolated WD in which the mesonephric tubules, along with most of the non-epithelial mesoderm is removed or (C) isolated WD cleared of all surrounding mesoderm before in vitro culture (“naked” epithelial tube) suspended within a 3D extracellular matrix gel. The cultures were grown for 3 days in either (A and B) DME/F12 supplemented with FBS and soluble grow factors [(A) 10 ng/ml GDNF; (B) 125 ng/ml GDNF and 250 ng/ml FGF1] or (C) BSN conditioned medium supplemented with 125 ng/ml GDNF and 250 ng/ml FGF1 ; (Scale bar: 500 µm.) (From ref. Citation20).](/cms/asset/ec28b221-f111-496a-8bd5-638838cd598c/kogg_a_10906498_f0003.gif)
Figure 4 (A–E) Phase-contrast photomicrographs of UBs isolated from ed13 embryonic rat kidney and cultured for up to 5 days in BSN conditioned media supplemented with 10% FBS and soluble growth factors (125 ng/ml GDNF and 250 ng/ml FGF-1). (From ref. Citation36).

Figure 5 (A–C) Phase contrast photomicrographs demonstrating the ability of in vitro formed UBs (induced from an isolated WD) to undergo branching morphogenesis in 3D cultures. (A) Isolated WD cultured for 4 days in DME/F12 supplemented with 125 ng/ml GDNF and 250 ng/ml FGF1 to induce the emergence of supernumerary UBs. (B and C) A single in vitro formed UB (circle in A) was excised, suspended within a 3D extracellular matrix gel (1:1 Matrigel/DME-F12) and cultured in BSN conditioned medium supplemented with 10% FBS, 125 ng/ml GDNF and 250 ng/ml FGF1. (From ref. Citation20).
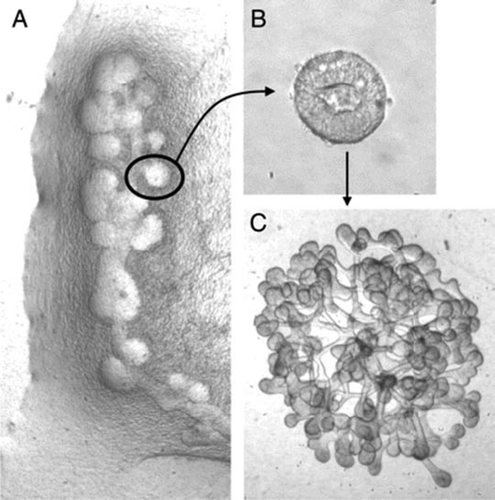
Figure 6 (A and B) Phase contrast photomicrographs demonstrating the ability to microdissect and propogate cultured isolated rat embryonic UBs. (A) UB cultured for 7 days in BSN conditioned medium (supplemented with 10% FBS, 125 ng/ml GDNF and 250 ng/ml FGF1) were dissected and subdivided into thirds and resuspended within 3D ECM gels. This subdivided UBs were cultured for an additional 8 days using the same culture conditions. (B) One of these second generation cultured was further subdivided, resuspended and cultured for an additional 8 days in BSN conditioned media (supplemented with 10% FBS, 125 ng/ml GDNF and 250 ng/ml FGF1). (C) A schematic representation of the procedure for isolated UB propagation indicates the potential for generating a large number of UBs from a single progenitor UB. (From ref. Citation30).
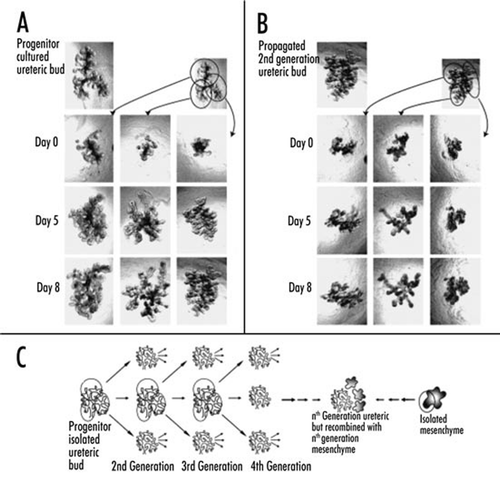
Figure 7 (A–C) Confocal fluorescent micrographs of kidney-like tissue engineered from the recombination of cultured UB (red—UB-derived tubules are stained with TRITC-conjugated D. biflorus,) and freshly isolated metanephric mesenchyme (7 days after recombination). The accumulation of 6-CF (green—a fluorescent organic anion which is transported via the basolaterally localized organic anion transporters of the proximal tubule) in the MM-derived tubules of the recombined tissue indicates that structural and functional differentiation of the mesenchyme is occurring. (B and C) The accumulation is seen only in the cells of non-UB-derived tubules (arrows) and is inhibited by probenecid- (a competitive inhibitor of organic anion transport) [Scale bars: (A and C), 500 µm; (B), 200 µm]. (From ref. Citation20).
![Figure 7 (A–C) Confocal fluorescent micrographs of kidney-like tissue engineered from the recombination of cultured UB (red—UB-derived tubules are stained with TRITC-conjugated D. biflorus,) and freshly isolated metanephric mesenchyme (7 days after recombination). The accumulation of 6-CF (green—a fluorescent organic anion which is transported via the basolaterally localized organic anion transporters of the proximal tubule) in the MM-derived tubules of the recombined tissue indicates that structural and functional differentiation of the mesenchyme is occurring. (B and C) The accumulation is seen only in the cells of non-UB-derived tubules (arrows) and is inhibited by probenecid- (a competitive inhibitor of organic anion transport) [Scale bars: (A and C), 500 µm; (B), 200 µm]. (From ref. Citation20).](/cms/asset/67099385-8f6c-4ab8-b35c-737794cedfca/kogg_a_10906498_f0007.gif)
Figure 8 Schematic of a proposed strategy for bioengineering kidneys from in vitro models of kidney development. Initially, supernumerary UBs are induced from the isolated WD. Potentially each of these buds can be isolated and propagated to form multiple branched UBs in 3D ECM gels; each of which can then be recombined with freshly isolated MM. Following a period of mutual induction (i.e., 4–7 days), the recombined tissue (which now resembles a late-stage embryonic kidney) is then implanted into a host animal where it vascularizes and forms glomeruli. The possible use of cultured cells to engineer progenitor tissues (i.e., WD, UB and/or MM-like tissue) is also indicated. (From ref. Citation20).
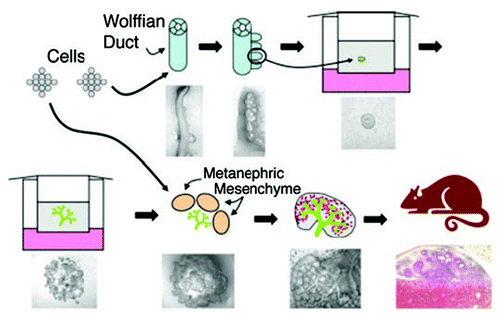
Figure 9 Clustergram [made using the hierarchical clustering algorithm, GENESPRING (Silicon Genetics)] showing the grouping of 873 genes whose expression changed significantly at some point in rat kidney development using Affymetrix cDNA microarray genechips. Genes were identified using RNA isolated from a time series of kidney development [i.e., ed13, ed15, ed17, ed19, newborn (N), 1 week postpartum (W) and adult (A) kidneys] and clustered in two dimensions according to their gene expression and experimental vectors in Euclidian space after compressing the equalized data to a target maximum value of 3. K-means clustering revealed 5 distinct groups of gene expression patterns within the clustergram (numbers at the bottom) depending on their time-frame of expression. (From ref. Citation35).
![Figure 9 Clustergram [made using the hierarchical clustering algorithm, GENESPRING (Silicon Genetics)] showing the grouping of 873 genes whose expression changed significantly at some point in rat kidney development using Affymetrix cDNA microarray genechips. Genes were identified using RNA isolated from a time series of kidney development [i.e., ed13, ed15, ed17, ed19, newborn (N), 1 week postpartum (W) and adult (A) kidneys] and clustered in two dimensions according to their gene expression and experimental vectors in Euclidian space after compressing the equalized data to a target maximum value of 3. K-means clustering revealed 5 distinct groups of gene expression patterns within the clustergram (numbers at the bottom) depending on their time-frame of expression. (From ref. Citation35).](/cms/asset/68f207b3-84c3-4d19-b16d-0a578dc354e6/kogg_a_10906498_f0009.gif)
Figure 10 Clustergram showing the grouping of differentially expressed genes from cultures of isolated UB and isolated MM together with genes from whole kidney development data. The genes were clustered in two dimensions according to their gene expression. Designations on the right side of cluster-gram refer to the tissue from which the genes were identified. Abbreviations are: K, kidney; e13, e15, e17, e19, day of embryonic gestation; nb, newborn; wk, 1 week postpartum; sc, spinal cord; no, no spinal cord. Red represents upregulated; blue represents downregulated. (From ref. Citation36).
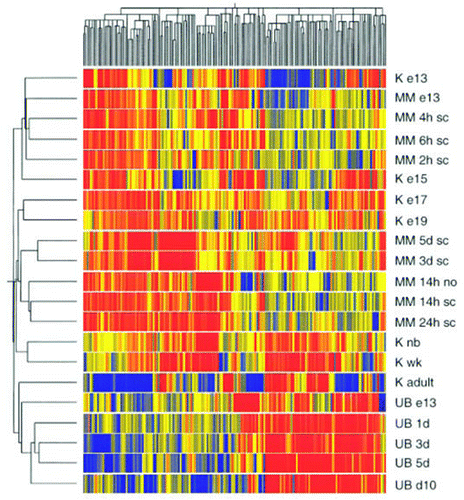
Acknowledgements
I wish to express my gratefulness to the many individuals who have worked in our lab on this topic over the years, most of whose contributions have been herein cited, but especially to Kevin Bush and Hiroyuki Sakurai. This work was supported by NIH grants DK57286 and DK65831.
Note
Edited transcripts of research conferences sponsored by Organogenesis and the Washington University George M. O'Brien Center for Kidney Disease Research (P30 DK079333) are published in Organogenesis. These conferences cover organogenesis in all multi-cellular organisms including research into tissue engineering, artificial organs and organ substitutes and are participated in by faculty at Washington University School of Medicine, St. Louis, MO.
References
- National Institute of Diabetes and Digestive and Kidney Diseases (NIDDK), NIoHN. U.S. Department of Health and Human Services (DHHS) 2005; Bethesda, MD System USRD
- Humes HD, Weitzel WF, Fissell WH. Renal cell therapy in the treatment of patients with acute and chronic renal failure. Blood Purif 2004; 22:60 - 72
- Dekel B, Burakova T, Arditti FD, Reich-Zeliger S, Milstein O, Aviel-Ronen S, Rechavi G, Friedman N, Kaminski N, Passwell JH, Reisner Y. Human and porcine early kidney precursors as a new source for transplantation. Nat Med 2003; 9:53 - 60
- Lanza RP, Chung HY, Yoo JJ, Wettstein PJ, Blackwell C, Borson N, Hofmeister E, Schuch G, Soker S, Moraes CT, West MD, Atala A. Generation of histocompatible tissues using nuclear transplantation. Nat Biotechnol 2002; 20:689 - 696
- Rogers SA, Lowell JA, Hammerman NA, Hammerman MR. Transplantation of developing metanephroi into adult rats. Kidney Int 1998; 54:27 - 37
- Steer DL, Nigam SK. Developmental approaches to kidney tissue engineering. Am J Physiol Renal Physiol 2004; 286:1 - 7
- Davies JA. Do different branching epithelia use a conserved developmental mechanism?. Bioessays 2002; 24:937 - 948
- Costantini F. Renal branching morphogenesis: concepts, questions and recent advances. Differentiation 2006; 74:402 - 421
- Dressler GR. The cellular basis of kidney development. Annu Rev Cell Dev Biol 2006; 22:509 - 529
- Shah MM, Sampogna RV, Sakurai H, Bush KT, Nigam SK. Branching morphogenesis and kidney disease. Development 2004; 131:1449 - 1462
- Vainio S, Lin Y. Coordinating early kidney development: Lessons from gene targeting. Nat Rev Genet 2002; 3:533 - 543
- Barros EJ, Santos OF, Matsumoto K, Nakamura T, Nigam SK. Differential tubulogenic and branching morphogenetic activities of growth factors: implications for epithelial tissue development. Proc Natl Acad Sci USA 1995; 92:4412 - 4416
- Cantley LG, Barros EJ, Gandhi M, Rauchman M, Nigam SK. Regulation of mitogenesis, motogenesis and tubulogenesis by hepatocyte growth factor in renal collecting duct cells. Am J Physiol 1994; 267:271 - 280
- Montesano R, Schaller G, Orci L. Induction of epithelial tubular morphogenesis in vitro by fibroblast-derived soluble factors. Cell 1991; 66:697 - 711
- Santos OF, Nigam SK. HGF-induced tubulogenesis and branching of epithelial cells is modulated by extracellular matrix and TGFbeta. Dev Biol 1993; 160:293 - 302
- Sakurai H, Barros EJ, Tsukamoto T, Barasch J, Nigam SK. An in vitro tubulogenesis system using cell lines derived from the embryonic kidney shows dependence on multiple soluble growth factors. Proc Natl Acad Sci USA 1997; 94:6279 - 6284
- Maeshima A, Sakurai H, Nigam SK. Adult kidney tubular cell population showing phenotypic plasticity, tubulogenic capacity and integration capability into developing kidney. J Am Soc Nephrol 2006; 17:188 - 198
- Gupta S, Verfaillie C, Chmielewski D, Kren S, Eidman K, Connaire J, Heremans Y, Lund T, Blackstad M, Jiang Y, Luttun A, Rosenberg ME. Isolation and characterization of kidney-derived stem cells. J Am Soc Nephrol 2006; 17:3028 - 3040
- Maeshima A, Vaughn DA, Choi Y, Nigam SK. Activin A is an endogenous inhibitor of ureteric bud outgrowth from the Wolffian duct. Dev Biol 2006; 295:473 - 485
- Rosines E, Sampogna RV, Johkura K, Vaughn DA, Choi Y, Sakurai H, Shah MM, Nigam SK. Staged in vitro reconstitution and implantation of engineered rat kidney tissue. Proc Natl Acad Sci USA 2007; 104:20938 - 20943
- Qiao J, Sakurai H, Nigam SK. Branching morphogenesis independent of mesenchymalepithelial contact in the developing kidney. Proc Natl Acad Sci USA 1999; 96:7330 - 7335
- Maeshima A, Sakurai H, Choi Y, Kitamura S, Vaughn DA, Tee JB, Nigam SK. Glial cell-derived neurotrophic factor independent ureteric bud outgrowth from the Wolffian duct. J Am Soc Nephrol 2007; 18:3147 - 3155
- Sakurai H, Bush KT, Nigam SK. Identification of pleiotrophin as a mesenchymal factor involved in ureteric bud branching morphogenesis. Development 2001; 128:3283 - 3293
- Qiao J, Bush KT, Steer DL, Stuart RO, Sakurai H, Wachsman W, Nigam SK. Multiple fibroblast growth factors support growth of the ureteric bud but have different effects on branching morphogenesis. Mech Dev 2001; 109:123 - 135
- Sakurai H, Bush KT, Nigam SK. Heregulin induces glial cell line-derived neurotrophic growth factor-independent, non-branching growth and differentiation of ureteric bud epithelia. J Biol Chem 2005; 280:42181 - 42187
- Bush KT, Sakurai H, Steer DL, Leonard MO, Sampogna RV, Meyer TN, Schwesinger C, Qiao J, Nigam SK. TGFbeta superfamily members modulate growth, branching, shaping and patterning of the ureteric bud. Dev Biol 2004; 266:285 - 298
- Kanwar YS, Wada J, Lin S, Danesh FR, Chugh SS, Yang Q, Banerjee T, Lomasney JW. Update of extracellular matrix, its receptors, and cell adhesion molecules in mammalian nephrogenesis. Am J Physiol Renal Physiol 2004; 286:202 - 215
- Steer DL, Shah MM, Bush KT, Stuart RO, Sampogna RV, Meyer TN, Schwesinger C, Bai X, Esko JD, Nigam SK. Regulation of ureteric bud branching morphogenesis by sulfated proteoglycans in the developing kidney. Dev Biol 2004; 272:310 - 327
- Meyer TN, Schwesinger C, Bush KT, Stuart RO, Rose DW, Shah MM, Vaughn DA, Steer DL, Nigam SK. Spatiotemporal regulation of morphogenetic molecules during in vitro branching of the isolated ureteric bud: toward a model of branching through budding in the developing kidney. Dev Biol 2004; 275:44 - 67
- Steer DL, Bush KT, Meyer TN, Schwesinger C, Nigam SK. A strategy for in vitro propagation of rat nephrons. Kidney Int 2002; 62:1958 - 1965
- Levashova ZB, Plisov SY, Perantoni AO. Conditionally immortalized cell line of inducible metanephric mesenchyme. Kidney Int 2003; 63:2075 - 2087
- Barasch J, Yang J, Ware CB, Taga T, Yoshida K, Erdjument-Bromage H, Tempst P, Parravicini E, Malach S, Aranoff T, Oliver JA. Mesenchymal to epithelial conversion in rat metanephros is induced by LIF. Cell 1999; 99:377 - 386
- Hammerman MR. Transplantation of embryonic kidneys. Clin Sci (Lond) 2002; 103:599 - 612
- Kim D, Dressler GR. Nephrogenic factors promote differentiation of mouse embryonic stem cells into renal epithelia. J Am Soc Nephrol 2005; 16:3527 - 3534
- Stuart RO, Bush KT, Nigam SK. Changes in global gene expression patterns during development and maturation of the rat kidney. Proc Natl Acad Sci USA 2001; 98:5649 - 5654
- Stuart RO, Bush KT, Nigam SK. Changes in gene expression patterns in the ureteric bud and metanephric mesenchyme in models of kidney development. Kidney Int 2003; 64:1997 - 2008
- Monte JC, Sakurai H, Bush KT, Nigam SK. The developmental nephrome: systems biology in the developing kidney. Curr Opin Nephrol Hypertens 2007; 16:3 - 9
- Sampogna RV, Nigam SK. Implications of gene networks for understanding resilience and vulnerability in the kidney branching program. Physiology (Bethesda) 2004; 19:339 - 347
- Sakurai H, Nigam SK. In vitro branching tubulogenesis: Implications for developmental and cystic disorders, nephron number, renal repair and nephron engineering. Kidney Int 1998; 54:14 - 26
- Bush KT, Vaughn DA, Li X, Rosenfeld MG, Rose DW, Mendoza SA, Nigam SK. Development and differentiation of the ureteric bud into the ureter in the absence of a kidney collecting system. Dev Biol 2006; 298:571 - 584