Abstract
Tissue engineering has shown promise for the development of constructs to facilitate large volume soft tissue augmentation in reconstructive and cosmetic plastic surgery. This article reviews the key progress to date in the field of adipose tissue engineering. In order to effectively design a soft tissue substitute, it is critical to understand the native tissue environment and function. As such, the basic physiology of adipose tissue is described and the process of adipogenesis is discussed. In this article, we have focused on tissue engineering using a cell-seeded scaffold approach, where engineered extracellular matrix substitutes are seeded with exogenous cells that may contribute to the regenerative response. The strengths and limitations of each of the possible cell sources for adipose tissue engineering, including adipose-derived stem cells, are detailed. We briefly highlight some of the results from the major studies to date, involving a range of synthetic and naturally derived scaffolds. While these studies have shown that adipose tissue regeneration is possible, more research is required to develop optimized constructs that will facilitate safe, predictable, and long-term augmentation in clinical applications.
Introduction
The focus of this review is on adipose engineering using a cell-seeded scaffold approach. Tissue engineering holds promise for the treatment of post-operative, congenital or post-traumatic loss of the subcutaneous fat layer, which can result in scar tissue formation deformity, and a loss of function.Citation1 Current clinical strategies for soft tissue augmentation primarily involve autologous, allogenic and alloplastic materials.Citation1 Free fat transfer yields unsatisfactory and unpredictable results, with varying degrees of graft resorption due to a lack of supporting vasculature.Citation2 Only small defects can be corrected with injected autologous fat, and even these limited applications require repeated treatments to maintain the desired volume.Citation3 Autologous tissue transfer using vascularized flaps that incorporate skin, fat and muscle, represents the gold standard treatment for large volume reconstruction.Citation4 These techniques require considerable surgical skill, but can yield far superior results to those obtained with synthetic implants. However, there are high costs in terms of donor site morbidity and deformity, as well as hospitalization and surgical time. Alloplastic and allogenic materials can be associated with immune rejection, allergic reaction, implant migration or resorption, and a failure to integrate into the host tissues.Citation1,Citation5
To successfully engineer a soft tissue substitute, it is critical to understand the basic physiology of adipose tissue and the process of adipogenesis. As such, we begin with a brief description of the primary functions and cellular composition of fat, with an emphasis on the adipose-derived stem cell population. Following this, we outline some of the key events and mediators involved in adipogenesis. By understanding the cellular processes involved in fat formation, it is possible to more accurately characterize the cellular response within an engineered scaffold, facilitating the optimization of the construct properties to maximize regeneration. The results from some of the main adipose tissue engineering studies involving cells in scaffolds are highlighted, and a perspective for the future is provided.
Adipose Tissue
Adipose tissue is a dynamic and multi-functional tissue that is ubiquitous throughout the human body.Citation6 Fat functions as a specialized organ that maintains the energy balance through controlled storage and release. Adipocytes store energy in the form of triglycerides, and accumulate or mobilize triacylglycerol in response to the body's energy requirements.Citation7,Citation8 Adipose tissue is highly plastic and can adapt to facilitate greater storage through the hypertrophic expansion of terminally-differentiated mature adipocytes, as well as the hyperplastic growth and differentiation of precursor cells present in the stroma.Citation9,Citation10
In addition to energy regulation, fat functions in maintaining normal body contours, providing mechanical protection, serving as a storage depot for cholesterol and steroid hormones, and is an important site of estrogen biosynthesis.Citation11–Citation14 Adipose tissue is also the source of multiple autocrine, paracrine and endocrine factors, many of which are involved in energy regulation and the modulation of insulin sensitivity,Citation15 including leptin,Citation16 tumor necrosis factor-α (TNFα),Citation17 plasminogen activator inhibitor-1 (PAI-1)Citation18 and adiponectin.Citation19 However, the complement of secreted factors can induce a range of responses within multiple systems, including the reproductive, immunological and vascular systems.Citation20
Cellular Composition of Adipose Tissue
Adipogenesis, the differentiation of precursor cells into mature, terminally-differentiated adipocytes, occurs throughout the human life cycle and is believed to be the primary cause of increases in body fat.Citation21 Adipose tissue contains multipotent mesenchymal stem cells (MSC), which can differentiate in culture along the adipogenic, chondrogenic, myogenic, osteogenic and putative neurogenic lineages.Citation22–Citation26 The term “adipose-derived stem cell” (ASC) is used to refer to the collective population of MSC and more-committed adipose progenitors that are found within the stroma of adipose tissue. When induced to differentiate, ASC accumulate intracellular lipid, increasing in diameter up to 20 times, and ultimately forming the characteristic morphology of mature white adipocytes.Citation7 Adipose tissue also contains fibroblasts, histiocytes and mast cells, as well as cells associated with the vascular and neural systems.Citation13,Citation27
Adipose-Derived Stem Cell Markers
ASC display a very similar immunophenotype to MSC isolated from bone marrow and skeletal muscle.Citation28,Citation29 Various studies have yielded differences in the detected expression patterns of some markers, potentially due to cell culture variations and/or donor variability.Citation30 A low percentage of ASC are positive for the multi-lineage marker STRO-1,Citation31 and, at early passage, a subset express stem cell markers such as CD90 (Thy-1), CD34, ATP-binding cassette subfamily G member 2 (ABCG2), and aldehyde dehydrogenase (ALDH).Citation30 Preadipocyte factor-1 (Pref-1), a transmembrane protein that is believed to have a role in regulating differentiation, is an additional marker of the undifferentiated cells.Citation32–Citation35 Endothelial markers can be detected in samples of freshly isolated stromal cells and early passage ASC. These markers include vascular endothelial growth factor receptor (Flk-1), CD31, CD144 and von Willebrand factor.Citation30 Some additional proteins expressed by the ASC are highlighted in .
Adipogenic Factors and Differentiation
There appears to be an inverse relationship between ASC proliferation and adipogenic differentiation.Citation36 In vitro differentiation is associated with growth arrest and can be induced in serum-free medium supplemented with cytokines that target adipogenic pathways.Citation37 Serum contains multiple factors, such as TNFα, growth hormone (GH) and epidermal growth factor (EGF), that inhibit lipogenesis.Citation38,Citation39 The differentiation of human ASC is strongly inhibited in the presence of serum, potentially due to its strong mitogenic effect, which may promote cellular decommitment.Citation40
The most common components in adipogenic medium formulations include insulin, triiodothyronine (T3), and glucocorticoids.Citation41,Citation42 Biotin and pantothenate are added to facilitate lipogenesis from glucose.Citation37 Isobutylmethylxanthine (IBMX), a phosphodiesterase inhibitor that has a protective effect on cAMP, has been included in some formulations with positive results.Citation42 Thiazolidinediones (TZD) including troglitazone and rosiglitazone, which are peroxisome proliferator-activated receptor-γ (PPARγ) ligands, have been shown to potently stimulate in vitro adipogenesis.Citation43–Citation45 PPARγ has been identified as a master regulator of adipogenic differentiation.Citation46 In general, the supplemented factors must be present at physiological concentrations or higher to have an effect.Citation12
Transcriptional Control of Adipocyte Differentiation
The differentiation of human ASC involves a complex series of changes in the cellular gene and protein expression patterns.Citation1,Citation47 In vitro differentiation is characterized by growth arrest, the induction and expression of multiple adipogenic genes (), and ultimately, triglyceride accumulation.Citation48 Three groups of transcription factors have been identified as key regulatorsCitation1 of adipocyte differentiation: (1) PPARγ,Citation49 (2) the CCAAT/enhancer binding proteins (C/EBP)Citation50 and (3) adipocyte determination- and differentiation-dependent factor-1/sterol regulatory element-binding protein-1 (ADD-1/SREBP-1).Citation51 highlights some of the interactions of these key factors. Detailed reviews of the transcriptional cascades can be found in the literature.Citation52–Citation55
Adipose Tissue Engineering
To date, a number of different constructs have been investigated for adipose tissue-engineering applications. The general strategy involves seeding an extracellular matrix (ECM) substitute with cells capable of contributing to the regenerative response. These studies have shown the promise of soft tissue regeneration. Further research is required to understand the optimal characteristics to facilitate stable fat formation.
Cellular Component
Most tissue-engineering strategies involve preadipocyte cell lines or primary adipogenic stem cells. These approaches circumvent the limitations of mature adipocytes, which are large, fragile, and highly prone to ischemic cell death.Citation56 Further, terminally-differentiated adipocytes do not proliferate in their mature form, thereby limiting their regenerative potential.Citation9,Citation10
Cell lines.
The most investigated adipogenic cell lines are the 3T3-L1, 3T3-F442a and Ob17 murine cell lines.Citation57,Citation58 Clonally derived cell lines are homogeneous, well defined, and can be cultured in vitro for extended time periods. Cell lines circumvent many of the problems associated with primary cells including tissue availability and donor variability.Citation21,Citation59 However, the primary cell response may be more representative of the true in vivo cellular behavior.Citation12 There are significant differences in the hormonal requirements for differentiation between cell lines and primary cells.Citation60,Citation61 In general, cell lines differentiate much more readily than primary cells, and can spontaneously convert into adipocytes in the presence of serum if growth arrest is maintained through confluence.Citation62
Mesenchymal stem cells.
Bone marrow (BM) is a source of MSC capable of adipogenic differentiation.Citation63 However, BM procurement can be painful and the cell yield is often extremely low, with only a very small percentage (0.001–0.01%) of the cells having multilineage potential.Citation64,Citation65 The culture conditions must be carefully regulated, and serum selection and growth factor supplementation are commonly required to facilitate expansion.Citation64 The differentiation capacity tends to decrease with increasing passage and time in culture.Citation66
As discussed, adipose tissue is another possible MSC source for tissue engineering. Most patients are able to donate sufficient fat for cell isolation without any adverse effects.Citation5,Citation56,Citation67 Using centrifugal separation and filtration techniques, up to 1 × 108 viable ASC can be isolated from processed tissues in the range of 100–500 mL.Citation68 ASC are more resistant to mechanical damage and ischemia than mature adipocytes.Citation1,Citation69 During lipogenesis, differentiating ASC synthesize new ECM and reorganize the matrix into the mature form found in fat.Citation70 Hence, ASC may remodel tissue-engineered constructs to more closely resemble native adipose tissue ECM. ASC also secrete bioactive factors when differentiating that stimulate endothelial cell growth and motility in vitro and angiogenesis in vivo.Citation71–Citation73 In immunosuppressed rodents, injected ASC have been shown to hone to sites of injury, engraft and contribute to regeneration.Citation63,Citation65,Citation74
It is important to note that cell isolation techniques and culture methods dramatically impact ASC proliferation and differentiation. While the cells can be expanded in culture for extended periods of time, they will senesce.Citation75 The differentiation capacity also declines with increasing passage and freezing.Citation36,Citation40 Differences in differentiation have been noted depending on the cell donor and the anatomic site of harvest.Citation76 Cells harvested from younger donors generally have a greater potential to proliferate and differentiate than those harvested from older donors.Citation41,Citation42
Engineered Extracellular Matrices
A tissue-engineered adipose substitute should facilitate complete and predictable volume restoration.Citation1 As mentioned, a common strategy is to incorporate a 3D scaffold that defines and maintains the desired tissue volume. The constructs must be easy to handle in the clinic and be readily modifiable to suit the individual needs of each patient.Citation77 These ECM substitutes should facilitate normal cellular organization and behavior, promote regeneration, and restore functionality.Citation1 Materials should be selected that are capable of controlled, non-toxic degradation, as they are replaced by healthy host tissues. Some of the results to date for a variety of investigated scaffolds are reviewed below.
Synthetic scaffolds.
Poly(lactic-co-glycolic) acid scaffolds. Patrick et al.Citation78 designed poly(lactic-co-glycolic) acid (PLGA) scaffolds seeded with primary rat adipose precursor cells, harvested from epididymal fat pads. When implanted into the dorsa of rats, differentiated adipocytes could be detected within the constructs by 5 weeks. Longer term in vivo studies showed that the adipose tissue completely resorbed by 5 months, likely due to scaffold degradation.Citation77
PLGA-polyethylene glycol (PLGA-PEG) microspheres were studied as drug delivery vehicles for insulin, insulin-like growth factor-1 (IGF-1), and basic fibroblast growth factor (bFGF) by Shenaq and Yuskel.Citation79 In a subcutaneous rat model, incorporating the growth factors improved autologous free fat graft weight and volume, with the best results observed for either insulin or IGF-1 alone or in combination. A PLGA (75:25) foam was also assessed in vivo in combination with IGF-1 and insulin, with fibroelastic tissue formation at the implantation site at 12 weeks.
In another study evaluating the impact of bFGF, Neubauer et al.Citation80 investigated rat BM MSC on PLGA scaffolds in vitro with or without the addition of bFGF. The investigation, which included measurements of GPDH activity and PPARγ and Glut4 gene expression, indicated that bFGF had a positive effect on adipogenesis.
Polyglycolic acid scaffolds. The cellular response of 3T3-L1 cells induced to differentiate in vitro on polyglycolic acid (PGA) scaffolds was investigated by Fischbach et al.Citation81 Within the scaffolds, the cells readily accumulated lipid and assumed a mature, unilocular morphology by 35 days in culture. Interestingly, leptin secretion and laminin expression were augmented in comparison to traditional two-dimensional culture. When the constructs were subcutaneously implanted in nude mice, histological results showed the formation of mature fat pads surrounded by a fibrous capsule at 35 days.
Polytetrafluoroethylene scaffolds. The differentiation of human ASC on non-degradable polytetrafluoroethylene (PTFE) surgical mesh coated with collagen, albumin or fibronectin was examined by Kral and Crandall.Citation67 While all of the coatings improved cell adhesion compared to the uncoated control, the highest seeding efficiency was observed with the fibronectin coating. Differentiation was induced, and some lipid accumulation was reported using SEM at 2 weeks after the induction of differentiation.
Poly(ethylene glycol)-diacrylate hydrogels. Alhadlaq et al.Citation82 reported on the encapsulation of human BM MSC within poly(ethylene glycol)-diacrylate (PEGDA) hydrogels. Differentiation was assessed at 4 weeks after seeding both in vitro and in vivo (subcutaneous athymic mouse model). In vivo, the scaffolds were shown to maintain their architecture and immunohistochemical staining indicated that the encapsulated cells expressed PPARγ. A later study confirmed that the cell-seeded PEGDA scaffolds facilitated adipose tissue formation and had excellent volume retention properties after 4 weeks in vivo.Citation83 More specifically, the scaffolds showed 100% volume retention as compared to 35–65% volume retention for collagen gels within the same system.
Polyethylene terephthalate scaffolds. The in vitro response of 3T3-L1 cells seeded on non-degradable polyethylene terephthalate (PET) scaffolds was characterized by Kang et al.Citation84 Oil red O staining showed high levels of intracellular lipid accumulation, with most of the cells having a mature morphology by 14 days after the induction of differentiation. RT-PCR analysis showed that the cells expressed multiple adipogenic markers including PPARγ, leptin, aP2 and Glut4. Similar to the results with the PGA scaffolds, the cells were shown to secrete higher levels of leptin in the three-dimensional scaffolds as compared to two-dimensional controls.
Naturally derived scaffolds.
Matrigel™. Matrigel™ is a commercially available product that is derived from the reconstituted basement membrane of mouse sarcoma. This gelatinous material contains an undefined mixture of proteins, including collagen type IV, laminin and a variety of growth factors. Matrigel™ has been shown to support cell growth and has been used extensively to study cellular responses within a 3D environment. However, its clinical application is prohibited by its murine and tumorigenic origins.Citation85
Kawaguchi et al.Citation86 demonstrated de novo adipose tissue formation when Matrigel™ was subcutaneously injected with bFGF in nude mice. The material induced a strong neovascularization response within 1 week, followed by the recruitment and differentiation of endogenous precursor cells. The fat pad was reported to have expanded for the first 3 weeks after implantation and retained its volume for the entire 10-week study. Matrigel™ and bFGF also supported in vivo adipogenesis in combination with seeded 3T3-F442A cells.
Matrigel™ scaffolds containing bFGF were also investigated in vivo within a specialized silicone housing that isolated a pedicled blood supply by Walton et al.Citation87 The authors noted significant adipogenesis and the maintenance of volume when both Matrigel™ and bFGF were implanted. Fibrovascular tissue growth and the formation of a fibrous capsule were also noted. A similar study was reported by Vashi et al.Citation85 Collagen was used in place of Matrigel™ and FGF-2 was encapsulated in microspheres to facilitate long-term delivery. Histological results indicated that the collagen combined with FGF-2 microspheres could facilitate adipose tissue formation, although a fibrous capsule could be observed surrounding the implant.
Collagen scaffolds. Collagen scaffolds have been extensively studied as cell adhesive biomaterials for a variety of tissue engineering applications. Collagen has advantages in terms of availability, cost, biodegradability, and relative biocompatibility.Citation88,Citation89
Von Heimburg et al. seeded collagen scaffolds with human ASC and subcutaneously implanted them in nude mice for 3 and 8 weeks.Citation90 The implants were generally coated in a thin layer of adipose tissue that contained new blood vessels. Under histological analysis, adipose tissue formation and neovascularization of the outer layers of the construct were confirmed. A fibrous capsule was reported and there was a significant decrease in graft thickness and structural collapse with time.
Gelatin microspheres containing bFGF were combined with human ASC and collagen sponges by Kimura et al. and the constructs were implanted in nude mice.Citation91 Adipose tissue developed in the constructs within 6 weeks. All three components were required to induce the response, and the extent of adipose tissue formation was dependent on the number of seeded cells and the bFGF concentration. It was hypothesized that the bFGF may have contributed to adipogenesis by promoting angiogenesis.
Gentleman et al.Citation92 investigated collagen gels embedded with short collagen fibres to make the constructs more resistant to cellular contraction. The gels were seeded with 3T3-L1 cells. At 21 days after the induction of differentiation, the cells were shown to have accumulated intracellular lipid.
Glutaraldehyde cross-linked collagen-chitosan hydrogels for soft tissue augmentation were developed by Wu et al.Citation93 The scaffolds were seeded with primary rat adipose precursors isolated from the epididymal fat pad. In vitro, the cells could be visualized on the scaffolds and were viable over a 10-day period. In a subcutaneous rat model, Oil Red O positive cells were present in the scaffold at 7 and 14 days.
HYAFF® scaffolds. A group in the Department of Plastic and Reconstructive Surgery at the Aachen University of Technology has extensively studied biodegradable HYAFF® scaffolds for adipose tissue engineering applications. HYAFF® is a semi-synthetic material that is a benzyl ester of hyaluronan (HA). In 2001, they investigated HYAFF® sponges, HYAFF® nonwoven carriers and freeze-dried collagen scaffolds, seeded with human ASC in a subcutaneous athymic mouse model.Citation94 The results indicated that the strongest differentiation response was observed within the pores of the HYAFF® sponges. Histology showed the presence of clusters of differentiating cells within the sponges at 3 weeks. In contrast, a greater number of undifferentiated ASC were observed in the nonwoven carriers. In 2003, they investigated cellular adhesion of human ASC on HYAFF® scaffolds with varying degrees of esterification (i.e., hydrophobicity) and pore size.Citation95 The results indicated that the greatest adhesion was observed with the scaffolds with 100% esterification and a mean pore size of 400 µm. Differentiation was observed when the scaffolds were maintained in adipogenic culture. In 2005, the group reported on a new 400 µm pore HYAFF® sponge with a different architecture.Citation96 In addition, they investigated the effect of coating the scaffolds with HA to make the constructs more hydrophilic and allow greater infiltration of the aqueous cell suspension. The scaffolds were seeded with human ASC and implanted for 3, 8 and 12 weeks in a subcutaneous athymic mouse model. The scaffolds showed improved cellular penetration and neovascularization as compared to the previously studied HYAFF® sponges. However, histological analysis indicated that there was only minimal adipose tissue formation within the constructs.
Alginate scaffolds. Marler et al.Citation97 investigated injectable alginate scaffolds seeded with syngeneic fibroblasts in an inbred rat subcutaneous model. A modified form of alginate was developed that incorporated the cell-adhesive peptide arginine, glycine and aspartic acid (RGD). A greater loss of volume was reported in the unseeded RGD-modified scaffolds (25% volume retention at 8 weeks) as compared to the unmodified controls (58% volume retention at 8 weeks). The addition of the fibroblasts improved the volume retention in both gels. In general, a fibrous capsule could be observed surrounding the implanted material. The RGD-modified material facilitated fibrovascular ingrowth from the surrounding tissues, which resulted in the fragmentation of the gel. Adipose tissue formation was not reported within any of the constructs.
Fibrin scaffolds. Injectable fibrin matrices containing human ASC and bFGF were investigated by Cho et al. in an athymic mouse model.Citation98 The gel was injected within an implanted PGA fibre mesh dome, reinforced with poly(lactic acid) (PLA). This supporting structure preserved the original volume of the implant for a 6-week period. Oil Red O staining indicated that there was adipose tissue formation within the fibrin gel when the dome was used.
Borges et al.Citation99 investigated the angiogenic potential of human ASC in fibrin glue in a chorioallantoic membrane model. The study indicated that the ASC were required to facilitate vascularization. Interestingly, the addition of vascular endothelial growth factor (VEGF) and bFGF to the medium did not influence the extent of vascularization.
Decellularized matrices. Our adipose tissue engineering research has focused on the investigation of naturally derived placental decellular matrix (PDM) and cross-linked hyaluronan (XLHA) scaffolds for soft tissue augmentation. Our initial work involved the development of a protocol to decellularize an intact, large segment of the human placenta.Citation100 Following the finalization of the decellularization protocol, the in vitro cellular response of primary human ASC to several different PDM and XLHA scaffolds was studied.Citation101,Citation102 Cellular organization, viability, proliferation, and glucose consumption were assessed during the proliferative phase prior to the induction of differentiation. The ASC differentiation response was characterized in terms of GPDH enzyme activity, intracellular lipid accumulation, and adipogenic gene expression using end-point (LPL, PPARγ, aP2, Glut4, leptin, GPDH) and real-time RT-PCR (PPARγ, GPDH). The cellular response was impacted by the scaffold environment and cell donor source. The cell-adhesive PDM scaffolds promoted ASC attachment, spreading and proliferation. In contrast, while all of the scaffolds could support the adipogenic differentiation of the ASC to some extent, differentiation was augmented when the cells were encapsulated in the non-cell-adhesive XLHA gels.
The PDM and PDM with XLHA scaffolds, seeded with primary human ASC, were also investigated in a subcutaneous athymic mouse model.Citation103 The in vivo response at 3 and 8 weeks was characterized using histological and immunohistochemical staining. The relative number of adipocytes within each implant was quantified. Undifferentiated ASC were localized using immunostaining for human vimentin. Unilocular and multilocular adipocytes were identified by Oil Red O staining and implant vascularization was assessed by staining for murine CD31. The 3D volume of the PDM and PDM with XLHA implants was qualitatively maintained over the course of the study. Mature adipocytes were detected within both types of scaffolds, generally in greater numbers at the 8-week time point. There were extensive numbers of adipocytes within a number of the implants, with the developing tissues closely resembling normal adipose tissue. Incorporating the XLHA appeared to have a positive effect on angiogenesis and adipogenesis.
Vascularization
To facilitate large volume soft tissue regeneration, it is important to recognize that adipogenesis and angiogenesis are highly interconnected processes.Citation104–Citation106 Following implantation, tissue-engineered adipose substitutes must rely on either revascularization or inosculation to become incorporated into the host vasculature.Citation1 Revascularization is limited by the slow kinetics of nutrient and waste diffusion. This process may be insufficient to support cellular survival in large or dense matrices.Citation56 In such devices, it may be necessary to create a capillary network within the adipose substitute and subsequently fuse this engineered system with the host vasculature.Citation107
Summary and Future Outlook
Adipose tissue engineering studies to date have shown that regeneration is possible using a cell-seeded scaffold approach. As discussed, many different types of scaffolds have been studied both in vitro and in vivo. Every scaffold has associated strengths and limitations, and the ideal environment for stable fat formation over the long-term remains unresolved.
While early research focused on qualitative histological assessments, the application of cellular and molecular biology techniques is required to develop a more thorough understanding of the cellular behavior. Techniques such as end-point and real-time RT-PCR, flow cytometry, Western blotting and DNA and cytokine arrays, should be used to characterize the gene and protein expression patterns of cells seeded within the scaffold environments. By using these approaches, it may be possible to develop a better understanding of the scaffold properties that promote adipogenesis, thereby facilitating construct optimization.
Human ASC may represent an ideal cellular population for a range of tissue-engineering applications, including adipose tissue regeneration. As discussed, the investigation of primary human cells may provide more relevant data to facilitate the ultimate goal of clinical application. The ASC population includes multipotent mesenchymal stem cells capable of differentiation along multiple lineages.Citation108,Citation109 To date, most adipose tissue engineering studies involving cell-seeded scaffolds have focused solely on probing adipogenic markers. However, to understand the impact of the various scaffold environments on the cellular behavior, the key signal transduction pathways involved in the commitment process should be probed. MSC express factors from each of the lineages, which cross-regulate one another and maintain the undifferentiated state.Citation54 During differentiation, there is an increase in the key transcription factors from one specific lineage, combined with a repression of the controlling factors for the other lineages. It is recognized that cellular interactions with scaffolds can influence signal transduction.Citation110 As such, it is likely that there are scaffold properties that would favor adipogenic differentiation of seeded MSC.
Our research has suggested a possible role for cell adhesion and shape in the modulation of adipogenic differentiation.Citation101,Citation102 Primary adipocytes maintain their mature, unilocular phenotype when cultured in suspension. When promoted to adhere to a surface, the adipocytes spread and de-differentiate.Citation111 Spiegelman and Ginty showed that adipogenic differentiation in the 3T3-F422A cell line was inhibited when strong cellular adhesion to fibronectin matrices was promoted, while disrupting the actin cytoskeleton and culturing the cells in a rounded state could restore the differentiation capacity.Citation112 Cell shape was also shown to modulate human MSC differentiation through the RhoA-ROCK signalling pathway, with adipogenic differentiation favored in MSC that were cultured with a rounded phenotype. In contrast, osteogenic differentiation was observed in culture conditions that promoted cellular attachment and spreading.Citation113 These results could have implications on the success of cell-seeded scaffolds for adipose tissue engineering.
Overall, adipose tissue engineering is an exciting and emerging field. By building on the work conducted to date, the ultimate goal of predictable, long-term soft tissue regeneration for volume augmentation may be possible in the future.
Abbreviations
α2Col6 | = | alpha 2 chain of collagen type VI |
ABCG2 | = | ATP-binding cassette subfamily G member 2 |
ADD-1/SREBP-1 | = | adipocyte determination- and differentiation-dependent factor-1/sterol regulatory element-binding protein-1 |
ALDH | = | aldehyde dehydrogenase |
aP2 | = | fatty acid binding protein |
ASC | = | adipose-derived stem cells |
bFGF | = | basic fibroblast growth factor |
BM | = | bone marrow |
ECM | = | extracellular matrix |
EGF | = | epidermal growth factor |
C/EBP | = | CCAAT/enhancer binding proteins |
FAS | = | fatty acid synthase |
FAT | = | fatty acid translocase |
GH | = | growth hormone |
Glut4 | = | glucose transporter-4 |
GPDH | = | glycerol-3-phosphate dehydrogenase |
HA | = | hyaluronan |
HSL | = | hormone sensitive lipase |
IBMX | = | isobutylmethylxanthine |
IGF-1 | = | insulin-like growth factor-1 |
LPL | = | lipoprotein lipase |
MSC | = | mesenchymal stem cells |
PAI-1 | = | plasminogen activator inhibitor-1 |
PDM | = | placental decellular matrix |
PEG | = | polyethylene glycol |
PEGDA | = | polyethylene glycol diacrylate |
PGA | = | polyglycolic acid |
PLA | = | poly(lactic acid) |
PLGA | = | poly(lactic-co-glycolic) |
PPARγ | = | peroxisome proliferator-activated receptor-γ |
PPRE | = | PPAR response elements |
PTFE | = | polytetrafluoroethylene |
RXR | = | retinoid X receptor |
T3 | = | triiodothyronine |
TNFα | = | tumour necrosis factor-α |
TZD | = | thiazolidinedione |
vWF | = | von willebrand factor |
VEGF | = | vascular endothelial cell growth factor |
XLHA | = | cross-linked hyaluronan |
Figures and Tables
Figure 1 Overview of the differentiation of human ASC into mature adipocytes. The detectable markers associated with the various stages of differentiation are highlighted (LPL = lipoprotein lipase; α2Col6 = alpha 2 chain of collagen type VI; FAT = fatty acid translocase; FAS = fatty acid synthase; aP2 = fatty acid binding protein; Glut4 = glucose transporter-4; GPDH = glycerol-3-phosphate dehydrogenase; HSL = hormone sensitive lipase).
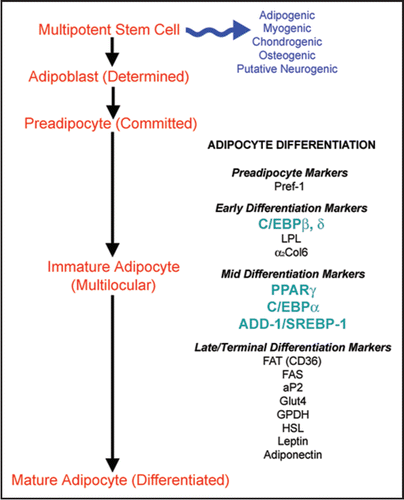
Figure 2 Transcriptional events in adipogenic differentiation. The diagram highlights the critical role of multiple transcription factors in adipogenesis. Arrows generally indicate a stimulatory interaction (with the exception of TNFα and leptin). After ligand binding with either endogenous ligands or TZD, PPARγ binds as a heterodimer with retinoid X receptor (RXR) to PPAR response elements (PPRE) in the transcriptional regulatory regions of the target genes. Insulin R., Insulin Receptor.
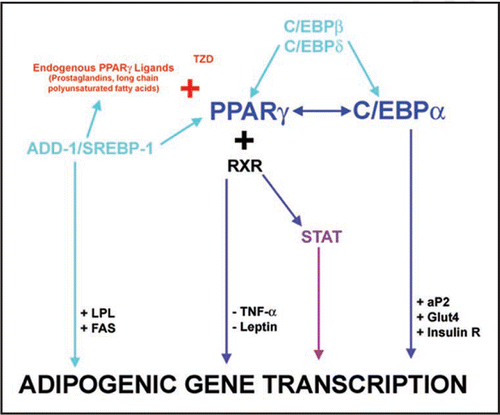
Table 1 ASC protein expressionCitation28,Citation47,Citation114
References
- Katz AJ, Llull R, Hedrick MH, Futrell JW. Emerging approaches to the tissue engineering of fat. Clin Plast Surg 1999; 26:587 - 603
- Peer LA. The neglected free fat graft. Plast Reconstr Surg 1956; 18:233 - 250
- Huss FR, Kratz G. Adipose tissue processed for lipoinjection shows increased cellular survival in vitro when tissue engineering principles are applied. Scand J Plast Reconstr Surg Hand Surg 2002; 36:166 - 171
- Beahm EK, Walton RL, Patrick CW Jr. Progress in adipose tissue construct development. Clin Plast Surg 2003; 30:547 - 558
- Patrick CW Jr. Tissue engineering strategies for adipose tissue repair. Anat Rec 2001; 263:361 - 366
- Klein J, Permana PA, Owecki M, Chaldakov GN, Bohm M, Hausman G, Lapiere CM, Atanassova P, Sowinski J, Fasshauer M, Hausman DB, Maquoi E, Tonchev AB, Peneva VN, Vlachanov KP, Fiore M, Aloe L, Slominski A, Reardon CL, Ryan TJ, Pond CM, Ryan TJ. What are subcutaneous adipocytes really good for?. Exp Dermatol 2007; 16:45 - 70
- Butterwith SC. Molecular events in adipocyte development. Pharmacol Ther 1994; 61:399 - 411
- Klaus S. Overview: Biological Significance of Fat and Adipose Tissues. Adipose Tissues 2001; Georgetown, Texas Landes Bioscience 1 - 10
- Prins JB, O'Rahilly S. Regulation of adipose cell number in man. Clin Sci (Lond) 1997; 92:3 - 11
- Sorisky A. From preadipocyte to adipocyte: differentiation-directed signals of insulin from the cell surface to the nucleus. Crit Rev Clin Lab Sci 1999; 36:1 - 34
- Pond CM. Morphological aspects and the ecological and mechanical consequences of fat deposition in wild vertebrates. Annu Rev Ecol Syst 1978; 9:519 - 570
- Ailhaud G. Development of White Adipose Tissue and Adipocyte Differentiation. Adipose Tissues 2001; Georgetown, Texas Landes Bioscience 27 - 35
- Pond CM. Physiological specialisation of adipose tissue. Prog Lipid Res 1999; 38:225 - 248
- Evans CT, Corbin CJ, Saunders CT, Merrill JC, Simpson ER, Mendelson CR. Regulation of estrogen biosynthesis in human adipose stromal cells. Effects of dibutyryl cyclic AMP, epidermal growth factor, and phorbol esters on the synthesis of aromatase cytochrome P-450. J Biol Chem 1987; 262:6914 - 6920
- Trayhurn P, Hoggard N, Rayner DV. White Adipose Tissue as a Secretory and Endocrine Organ: Leptin and Other Secreted Proteins. Adipose Tissues 2001; Georgetown, Texas Landes Bioscience 158 - 182
- Bornstein SR, Abu-Asab M, Glasow A, Path G, Hauner H, Tsokos M, Chrousos GP, Scherbaum WA. Immunohistochemical and ultrastructural localization of leptin and leptin receptor in human white adipose tissue and differentiating human adipose cells in primary culture. Diabetes 2000; 49:532 - 538
- Hotamisligil GS, Arner P, Caro JF, Atkinson RL, Spiegelman BM. Increased adipose tissue expression of tumor necrosis factor-alpha in human obesity and insulin resistance. J Clin Invest 1995; 95:2409 - 2415
- Crandall DL, Quinet EM, Morgan GA, Busler DE, McHendry-Rinde B, Kral JG. Synthesis and secretion of plasminogen activator inhibitor-1 by human preadipocytes. J Clin Endocrinol Metab 1999; 84:3222 - 3227
- Berg AH, Combs TP, Scherer PE. ACRP30/adiponectin: an adipokine regulating glucose and lipid metabolism. Trends Endocrinol Metab 2002; 13:84 - 89
- Schaffler A, Muller-Ladner U, Scholmerich J, Buchler C. Role of adipose tissue as an inflammatory organ in human diseases. Endocr Rev 2006; 27:449 - 467
- Smas CM, Sul HS. Control of adipocyte differentiation. Biochem J 1995; 309:697 - 710
- Zuk PA, Zhu M, Mizuno H, Huang J, Futrell JW, Katz AJ, et al. Multilineage cells from human adipose tissue: implications for cell-based therapies. Tissue Eng 2001; 7:211 - 228
- Gaustad KG, Boquest AC, Anderson BE, Gerdes AM, Collas P. Differentiation of human adipose tissue stem cells using extracts of rat cardiomyocytes. Biochem Biophys Res Commun 2004; 314:420 - 427
- Mizuno H, Zuk PA, Zhu M, Lorenz HP, Benhaim P, Hedrick MH. Myogenic differentiation by human processed lipoaspirate cells. Plast Reconstr Surg 2002; 109:199 - 209
- Betre H, Ong SR, Guilak F, Chilkoti A, Fermor B, Setton LA. Chondrocytic differentiation of human adipose-derived adult stem cells in elastin-like polypeptide. Biomaterials 2006; 27:91 - 99
- Safford KM, Rice HE. Stem cell therapy for neurologic disorders: therapeutic potential of adipose-derived stem cells. Curr Drug Targets 2005; 6:57 - 62
- Cinit S. Morphology of the Adipose Organ. Adipose Tissues 2001; Georgetown, Texas Landes Bioscience 11 - 26
- Gimble J, Guilak F. Adipose-derived adult stem cells: isolation, characterization and differentiation potential. Cytotherapy 2003; 5:362 - 369
- Aust L, Devlin B, Foster SJ, Halvorsen YD, Hicok K, du Laney T, Sen A, Willingmyre GD, Gimble JM. Yield of human adipose-derived adult stem cells from liposuction aspirates. Cytotherapy 2004; 6:7 - 14
- Mitchell JB, McIntosh K, Zvonic S, Garrett S, Floyd ZE, Kloster A, Di Halvorsen Y, Storms RW, Goh B, Kilroy G, Wu X, Gimble JM. Immunophenotype of human adipose-derived cells: temporal changes in stromal-associated and stem cell-associated markers. Stem Cells 2006; 24:376 - 385
- De Ugarte DA, Alfonso Z, Zuk PA, Elbarbary A, Zhu M, Ashjian P, Benhaim P, Hedrick MH, Fraser JK. Differential expression of stem cell mobilization-associated molecules on multi-lineage cells from adipose tissue and bone marrow. Immunol Lett 2003; 89:267 - 270
- Sul HS, Smas CM, Wang D, Chen L. Regulation of fat synthesis and adipose differentiation. Prog Nucleic Acid Res Mol Biol 1998; 60:317 - 345
- Smas CM, Sul HS. Pref-1, a protein containing EGF-like repeats, inhibits adipocyte differentiation. Cell 1993; 73:725 - 734
- Smas CM, Sul HS. Characterization of Pref-1 and its inhibitory role in adipocyte differentiation. Int J Obes Relat Metab Disord 1996; 20:65 - 72
- Sul HS, Smas C, Mei B, Zhou L. Function of pref-1 as an inhibitor of adipocyte differentiation. Int J Obes Relat Metab Disord 2000; 24:15 - 19
- Entenmann G, Hauner H. Relationship between replication and differentiation in cultured human adipocyte precursor cells. Am J Physiol 1996; 270:1011 - 1016
- Hauner H, Skurk T, Wabitsch M. Cultures of human adipose precursor cells. Methods Mol Biol 2001; 155:239 - 247
- Hauner H, Rohrig K, Petruschke T. Effects of epidermal growth factor (EGF), platelet-derived growth factor (PDGF) and fibroblast growth factor (FGF) on human adipocyte development and function. Eur J Clin Invest 1995; 25:90 - 96
- Serrero G, Lepak N. Endocrine and paracrine negative regulators of adipose differentiation. Int J Obes Relat Metab Disord 1996; 20:58 - 64
- Hemmrich K, von Heimburg D, Cierpka K, Haydarlioglu S, Pallua N. Optimization of the differentiation of human preadipocytes in vitro. Differentiation 2005; 73:28 - 35
- Hauner H, Schmid P, Pfeiffer EF. Glucocorticoids and insulin promote the differentiation of human adipocyte precursor cells into fat cells. J Clin Endocrinol Metab 1987; 64:832 - 835
- Hauner H, Entenmann G, Wabitsch M, Gaillard D, Ailhaud G, Negrel R, Pfeiffer EF. Promoting effect of glucocorticoids on the differentiation of human adipocyte precursor cells cultured in a chemically defined medium. J Clin Invest 1989; 84:1663 - 1670
- Halvorsen YD, Bond A, Sen A, Franklin DM, Lea-Currie YR, Sujkowski D, Ellis PN, Wilkison WO, Gimble JM. Thiazolidinediones and glucocorticoids synergistically induce differentiation of human adipose tissue stromal cells: biochemical, cellular and molecular analysis. Metabolism 2001; 50:407 - 413
- Patel NG, Holder JC, Smith SA, Kumar S, Eggo MC. Differential regulation of lipogenesis and leptin production by independent signaling pathways and rosiglitazone during human adipocyte differentiation. Diabetes 2003; 52:43 - 50
- Rodriguez AM, Elabd C, Delteil F, Astier J, Vernochet C, Saint-Marc P, Guesnet J, Guezennec A, Amri EZ, Dani C, Ailhaud G. Adipocyte differentiation of multipotent cells established from human adipose tissue. Biochem Biophys Res Commun 2004; 315:255 - 263
- Kliewer SA. Ntambi JM. “Structure and Function of the Peroxisome Proliferator-Activated Receptors”. Adipocyte Biology and Hormone Signalling 2000; Amsterdam IOS Press 211 - 223
- DeLany JP, Floyd ZE, Zvonic S, Smith A, Gravois A, Reiners E, Wu X, Kilroy G, Lefevre M, Gimble JM. Proteomic analysis of primary cultures of human adipose-derived stem cells: modulation by Adipogenesis. Mol Cell Proteomics 2005; 4:731 - 740
- Gregoire FM, Smas CM, Sul HS. Understanding adipocyte differentiation. Physiol Rev 1998; 78:783 - 809
- Hamm JK, el Jack AK, Pilch PF, Farmer SR. Role of PPARgamma in regulating adipocyte differentiation and insulin-responsive glucose uptake. Ann N Y Acad Sci 1999; 892:134 - 145
- Farmer SR. Regulation of PPARgamma activity during adipogenesis. Int J Obes (Lond) 2005; 29:13 - 16
- Kim JB, Wright HM, Wright M, Spiegelman BM. ADD1/SREBP1 activates PPARgamma through the production of endogenous ligand. Proc Natl Acad Sci USA 1998; 95:4333 - 4337
- Gregoire FM. Adipocyte differentiation: from fibroblast to endocrine cell. Exp Biol Med (Maywood) 2001; 226:997 - 1002
- Lazar MA. Becoming fat. Genes Dev 2002; 16:1 - 5
- Rosen ED, MacDougald OA. Adipocyte differentiation from the inside out. Nat Rev Mol Cell Biol 2006; 7:885 - 896
- Morrison RF, Farmer SR. Hormonal signaling and transcriptional control of adipocyte differentiation. J Nutr 2000; 130:3116 - 3121
- Patrick CW Jr. Adipose tissue engineering: the future of breast and soft tissue reconstruction following tumor resection. Semin Surg Oncol 2000; 19:302 - 311
- Green H, Kehinde O. Spontaneous heritable changes leading to increased adipose conversion in 3T3 cells. Cell 1976; 7:105 - 113
- Green H, Meuth M. An established pre-adipose cell line and its differentiation in culture. Cell 1974; 3:127 - 133
- Cornelius P, MacDougald OA, Lane MD. Regulation of adipocyte development. Annu Rev Nutr 1994; 14:99 - 129
- Adachi H, Kurachi H, Homma H, Adachi K, Imai T, Morishige K, Matsuzawa Y, Miyake A. Epidermal growth factor promotes adipogenesis of 3T3-L1 cell in vitro. Endocrinology 1994; 135:1824 - 1830
- Morikawa M, Nixon T, Green H. Growth hormone and the adipose conversion of 3T3 cells. Cell 1982; 29:783 - 789
- Kuri-Harcuch W, Green H. Adipose conversion of 3T3 cells depends on a serum factor. Proc Natl Acad Sci USA 1978; 75:6107 - 6109
- Ogawa R. The importance of adipose-derived stem cells and vascularized tissue regeneration in the field of tissue transplantation. Current Stem Cell Research and Therapy 2006; 1:13 - 20
- De Ugarte DA, Morizono K, Elbarbary A, Alfonso Z, Zuk PA, Zhu M, Dragoo JL, Ashjian P, Thomas B, Benhaim P, Chen I, Fraser J, Hedrick MH. Comparison of multi-lineage cells from human adipose tissue and bone marrow. Cells Tissues Organs 2003; 174:101 - 109
- Barry FP, Murphy JM. Mesenchymal stem cells: clinical applications and biological characterization. Int J Biochem Cell Biol 2004; 36:568 - 584
- Ringe J, Kaps C, Burmester GR, Sittinger M. Stem cells for regenerative medicine: advances in the engineering of tissues and organs. Naturwissenschaften 2002; 89:338 - 351
- Kral JG, Crandall DL. Development of a human adipocyte synthetic polymer scaffold. Plast Reconstr Surg 1999; 104:1732 - 1738
- De Ugarte DA, Ashjian PH, Elbarbary A, Hedrick MH. Future of fat as raw material for tissue regeneration. Ann Plast Surg 2003; 50:215 - 219
- von Heimburg D, Hemmrich K, Zachariah S, Staiger H, Pallua N. Oxygen consumption in undifferentiated versus differentiated adipogenic mesenchymal precursor cells. Respir Physiol Neurobiol 2005; 146:107 - 116
- Nakajima I, Yamaguchi T, Ozutsumi K, Aso H. Adipose tissue extracellular matrix: newly organized by adipocytes during differentiation. Differentiation 1998; 63:193 - 200
- Dobson DE, Kambe A, Block E, Dion T, Lu H, Castellot JJ Jr, Spiegelman BM. 1-Butyrylglycerol: a novel angiogenesis factor secreted by differentiating adipocytes. Cell 1990; 61:223 - 230
- Castellot JJ Jr, Karnovsky MJ, Spiegelman BM. Differentiation-dependent stimulation of neovascularization and endothelial cell chemotaxis by 3T3 adipocytes. Proc Natl Acad Sci USA 1982; 79:5597 - 5601
- Castellot JJ Jr, Karnovsky MJ, Spiegelman BM. Potent stimulation of vascular endothelial cell growth by differentiated 3T3 adipocytes. Proc Natl Acad Sci USA 1980; 77:6007 - 6011
- Meyerrose TE, De Ugarte DA, Hofling AA, Herrbrich PE, Cordonnier TD, Shultz LD, Eagon JC, Wirthlin L, Sands MS, Hedrick MA, Nolta JA. In vivo distribution of human adipose-derived mesenchymal stem cells in novel xenotransplantation models. Stem Cells 2007; 25:220 - 227
- Ryden M, Dicker A, Gotherstrom C, Astrom G, Tammik C, Arner P, Le Blanc K. Functional characterization of human mesenchymal stem cell-derived adipocytes. Biochem Biophys Res Commun 2003; 311:391 - 397
- Tchkonia T, Tchoukalova YD, Giorgadze N, Pirtskhalava T, Karagiannides I, Forse RA, Koo A, Stevenson M, Chinnappan D, Cartwright A, Jensen MD, Kirkland JL. Abundance of two human preadipocyte subtypes with distinct capacities for replication, adipogenesis and apoptosis varies among fat depots. Am J Physiol Endocrinol Metab 2005; 288:267 - 277
- Patrick CW. Breast tissue engineering. Annu Rev Biomed Eng 2004; 6:109 - 130
- Patrick CW Jr, Chauvin PB, Hobley J, Reece GP. Preadipocyte seeded PLGA scaffolds for adipose tissue engineering. Tissue Eng 1999; 5:139 - 151
- Shenaq SM, Yuksel E. New research in breast reconstruction: adipose tissue engineering. Clin Plast Surg 2002; 29:111 - 125
- Neubauer M, Hacker M, Bauer-Kreisel P, Weiser B, Fischbach C, Schulz MB, Goepferich A, Blunk T. Adipose tissue engineering based on mesenchymal stem cells and basic fibroblast growth factor in vitro. Tissue Eng 2005; 11:1840 - 1851
- Fischbach C, Spruss T, Weiser B, Neubauer M, Becker C, Hacker M, Gopferich A, Blunk T. Generation of mature fat pads in vitro and in vivo utilizing 3-D long-term culture of 3T3-L1 preadipocytes. Exp Cell Res 2004; 300:54 - 64
- Alhadlaq A, Tang M, Mao JJ. Engineered adipose tissue from human mesenchymal stem cells maintains predefined shape and dimension: implications in soft tissue augmentation and reconstruction. Tissue Eng 2005; 11:556 - 566
- Stosich MS, Mao JJ. Adipose tissue engineering from human adult stem cells: clinical implications in plastic and reconstructive surgery. Plast Reconstr Surg 2007; 119:71 - 83
- Kang X, Xie Y, Kniss DA. Adipose tissue model using three-dimensional cultivation of preadipocytes seeded onto fibrous polymer scaffolds. Tissue Eng 2005; 11:458 - 468
- Vashi AV, Abberton KM, Thomas GP, Morrison WA, O'Connor AJ, Cooper-White JJ, Thompson EW. Adipose Tissue Engineering Based on the Controlled Release of Fibroblast Growth Factor-2 in a Collagen Matrix. Tissue Eng 2006;
- Kawaguchi N, Toriyama K, Nicodemou-Lena E, Inou K, Torii S, Kitagawa Y. De novo adipogenesis in mice at the site of injection of basement membrane and basic fibroblast growth factor. Proc Natl Acad Sci USA 1998; 95:1062 - 1066
- Walton RL, Beahm EK, Wu L. De novo adipose formation in a vascularized engineered construct. Microsurgery 2004; 24:378 - 384
- Nimni ME, Cheung D, Strates B, Kodama M, Sheikh K. Chemically modified collagen: a natural biomaterial for tissue replacement. J Biomed Mater Res 1987; 21:741 - 771
- Kim BS, Baez CE, Atala A. Biomaterials for tissue engineering. World J Urol 2000; 18:2 - 9
- von Heimburg D, Zachariah S, Heschel I, Kuhling H, Schoof H, Hafemann B, Pallua N. Human preadipocytes seeded on freeze-dried collagen scaffolds investigated in vitro and in vivo. Biomaterials 2001; 22:429 - 438
- Kimura Y, Ozeki M, Inamoto T, Tabata Y. Adipose tissue engineering based on human preadipocytes combined with gelatin microspheres containing basic fibroblast growth factor. Biomaterials 2003; 24:2513 - 2521
- Gentleman E, Nauman EA, Livesay GA, Dee KC. Collagen composite biomaterials resist contraction while allowing development of adipocytic soft tissue in vitro. Tissue Eng 2006; 12:1639 - 1649
- Wu X, Black L, Santacana-Laffitte G, Patrick CW Jr. Preparation and assessment of glutaraldehyde-crosslinked collagen-chitosan hydrogels for adipose tissue engineering. J Biomed Mater Res A 2007; 81:59 - 65
- von Heimburg D, Zachariah S, Low A, Pallua N. Influence of different biodegradable carriers on the in vivo behavior of human adipose precursor cells. Plast Reconstr Surg 2001; 108:411 - 420
- Halbleib M, Skurk T, de Luca C, von Heimburg D, Hauner H. Tissue engineering of white adipose tissue using hyaluronic acid-based scaffolds. I: in vitro differentiation of human adipocyte precursor cells on scaffolds. Biomaterials 2003; 24:3125 - 3132
- Hemmrich K, von Heimburg D, Rendchen R, Di Bartolo C, Milella E, Pallua N. Implantation of preadipocyte-loaded hyaluronic acid-based scaffolds into nude mice to evaluate potential for soft tissue engineering. Biomaterials 2005;
- Marler JJ, Guha A, Rowley J, Koka R, Mooney D, Upton J, Vacanti JP. Soft-tissue augmentation with injectable alginate and syngeneic fibroblasts. Plast Reconstr Surg 2000; 105:2049 - 2058
- Cho SW, Kim SS, Rhie JW, Cho HM, Choi CY, Kim BS. Engineering of volume-stable adipose tissues. Biomaterials 2005; 26:3577 - 3585
- Borges J, Torio-Padron N, Momeni A, Mueller MC, Tegtmeier FT, Stark BG. Adipose precursor cells (preadipocytes) induce formation of new vessels in fibrin glue on the newly developed cylinder chorioallantoic membrane model (CAM). Minim Invasive Ther Allied Technol 2006; 15:246 - 252
- Flynn L, Semple JL, Woodhouse KA. Decellularized placental matrices for adipose tissue engineering. J Biomed Mater Res A 2006; 79:359 - 369
- Flynn L, Prestwich GD, Semple JL, Woodhouse KA. Adipose tissue engineering with naturally derived scaffolds and adipose-derived stem cells. Biomaterials 2007; 28:3834 - 3842
- Flynn L, Prestwich GD, Semple JL, Woodhouse KA. Proliferation and differentiation of adipose-derived stem cells on naturally derived scaffolds. Biomaterials 2008; 29:1862 - 1871
- Flynn L, Prestwich GD, Semple JL, Woodhouse KA. Adipose tissue engineering in vivo with adipose-derived stem cells on naturally derived scaffolds. J Biomed Mater Res A 2008; in press
- Hausman GJ, Richardson RL. Adipose tissue angiogenesis. J Anim Sci 2004; 82:925 - 934
- Fukumura D, Ushiyama A, Duda DG, Xu L, Tam J, Krishna V, Chatterjee K, Garkavtsev I, Jain RK. Paracrine regulation of angiogenesis and adipocyte differentiation during in vivo adipogenesis. Circ Res 2003; 93:88 - 97
- Lau DC, Schillabeer G, Li ZH, Wong KL, Varzaneh FE, Tough SC. Paracrine interactions in adipose tissue development and growth. Int J Obes Relat Metab Disord 1996; 20:16 - 25
- Frerich B, Lindemann N, Kurtz-Hoffmann J, Oertel K. In vitro model of a vascular stroma for the engineering of vascularized tissues. Int J Oral Maxillofac Surg 2001; 30:414 - 420
- Zuk PA, Zhu M, Ashjian P, De Ugarte DA, Huang JI, Mizuno H, Alfonso ZC, Fraser JK, Benhaim P, Hedrick MH. Human adipose tissue is a source of multipotent stem cells. Mol Biol Cell 2002; 13:4279 - 4295
- Caplan AI. Mesenchymal stem cells. J Orthop Res 1991; 9:641 - 650
- Gumbiner BM. Cell adhesion: the molecular basis of tissue architecture and morphogenesis. Cell 1996; 84:345 - 357
- Tholpady SS, Aojanepong C, Llull R, Jeong JH, Mason AC, Futrell JW, Ogle RC, Katz AJ. The cellular plasticity of human adipocytes. Ann Plast Surg 2005; 54:651 - 656
- Spiegelman BM, Ginty CA. Fibronectin modulation of cell shape and lipogenic gene expression in 3T3-adipocytes. Cell 1983; 35:657 - 666
- McBeath R, Pirone DM, Nelson CM, Bhadriraju K, Chen CS. Cell shape, cytoskeletal tension, and RhoA regulate stem cell lineage commitment. Dev Cell 2004; 6:483 - 495
- Zvonic S, Lefevre M, Kilroy G, Floyd ZE, DeLany JP, Kheterpal I, Gravois A, Dow R, White A, Wu X, Gimble JM. Secretome of primary cultures of human adipose-derived stem cells: modulation of serpins by adipogenesis. Mol Cell Proteomics 2007; 6:18 - 28