Abstract
The transcriptional cascade governing adipogenesis has been thoroughly examined throughout the years. Transcription factors PPARγ and C/EBPα are universally recognized as the master regulators of adipocyte differentiation and together they direct the establishment of the gene expression pattern of mature adipose cells. However, this familiar landscape has been considerably broadened in recent years by the identification of novel factors that participate in the regulation of adipogenesis, either favoring or inhibiting it, through their effects on chromatin. Epigenetic signals and chromatin-modifying proteins contribute to adipogenesis and, through regulation of the phenotypic maintenance of the mature adipocytes, to the control of metabolism. In this review we intend to summarize the recently described epigenetic events that participate in adipogenesis and their connections with the main factors that constitute the classical transcriptional cascade.
Introduction
The concept of epigenetics currently refers to the study of cellular mechanisms that control somatically, and sometimes transgenerationally, inheritable gene expression states that are established in the absence of a change in the DNA sequence itself.Citation1 Epigenetic mechanisms comprise but are not limited to an array of molecular modifications to both DNA and its associated proteins that control the “transcriptional state” of the cells.Citation2 Consequently, epigenetic processes play a crucial role in the establishment and maintenance of cell identity by selectively activating or repressing transcription of subsets of tissue-specific genes.
Adipogenesis is one of the most studied and best characterized differentiation processes. The increased prevalence of obesity in present day society, and the current view of the adipose tissue as one of the most critical regulators of energy homeostasis, metabolism and immunity have warranted a sustained interest in the study of the mechanisms controlling its formation and regulation.Citation3 The transcriptional cascade governing adipogenesis has been extensively studied for many years and is reviewed elsewhere, Citation4,Citation5 but the underlying role of epigenetic processes in the development of fat cells has only begun to attract attention.
The molecular mechanisms that mediate epigenetic regulation are principally DNA methylation, chromatin remodeling, the post-translational modifications of the histones, the control of the higher-level organization of chromatin within the nucleus and the regulation by non-codings RNAs (reviewed in ref. Citation2). DNA methylation, which takes place at the carbon-5 position of cytosine in CpG dinucleotides, is usually associated with gene silencing and is the only known modification that targets DNA itself.Citation6 But the most studied epigenetic mechanism to date is the covalent modification of the histone tails.Citation7 Several transcriptional coregulators have the capacity to enzymatically modify histones by acetylation, methylation, phosphorylation, sumoylation or ubiquitylationCitation8,Citation9 and numerous reports have shown a clear link between the pattern of histone modifications in the chromatin of a given gene and its transcriptional state. Thus, histone lysine acetylation is usually related to gene activationCitation10,Citation11 whereas lysine methylation results in different outcomes, depending on the modified residue.Citation9 Global analysis of histone lysine methylation by means of chromatin immunopecipitation assays coupled to microarray hybridization or direct sequencing have shown that methylation of lysine 4 of histone 3 (H3K4) usually correlates with gene activationCitation12–Citation14 whereas methylation of H3K9 or H3K27 associate with transcriptional silencing.Citation15,Citation16 Adding a layer of complexity, a number of histone-modifying enzymes also target nonhistone proteins, such as sequence-specific transcription factors or RNA polymerase II, thus broadening their range of influence upon gene activity.Citation8
In this review we intend to summarize the role that different epigenetic signals and chromatin-modifying proteins play in adipogenesis by their interactions with the classical transcriptional cascade regulating the differentiation of adipose cells.
The Adipose Tissue
There are in mammals two types of adipose tissue (reviewed in refs. Citation17 and Citation18). The white adipose tissue (WAT) is constituted by highly specialized cells that store high quantities of lipids and respond to environmental cues by releasing a wide variety of bioactive molecules known as adipocytokines that participate in the regulation of insulin sensitivity, energy balance, vascular haemostasis or inflammation.Citation19 White adipocytes are found in stereotypical depots throughout the body, displaying distinct molecular and physiological properties depending on their location.Citation20,Citation21 Brown adipose tissue (BAT), on the other hand, is predominantly localized in the interescapular region in both humans and rodents. The distinctive feature of brown adipocytes is their high mitochondrial content and expression of uncoupling protein 1 (UCP1), that make these cells opt for energy burning instead of storage since they can metabolize fatty acids and generate heat.Citation22,Citation23 Thus, brown adipocytes play a crucial role in the regulation of nonshivering thermogenesis, essential in newborn mammals, but until recently considered only of marginal significance in adults. However, several recent works have reported the existence of defined regions of functionally active BAT in adult humans.Citation24,Citation25 Interestingly, the amount of BAT inversely correlates with body-mass index, especially in older people, suggesting a role for brown fat in the protection against obesity.Citation26 These findings have emphasized the necessity to better understand the subtle balance in the regulation of white and brown adipose tissue development and identify which are the environmental cues and molecular basis upon which each cell chooses its fate.
The adipose tissue, together with muscle and bone, is considered to emerge from the mesenchymal stem cells (MSCs) derived from the mesodermal layer of the embryo. However, lineage-tracking studies have shown that at least a subset of adipocytes originates from MSCs derived from the neuroectoderm.Citation27 Comprehensive lineage-tracking studies are required to clarify this question, but a mixed origin of the different fat deposits would explain their distinctive molecular and biological features.Citation18,Citation21
An extracellular cue recruits MSCs to commit to a particular lineage (). This step results in the conversion of the pluripotent stem cells into preadipocytes, which can not be distinguished morphologically from their precursors but have lost the capacity to differentiate into other cell types. This is the first phase of adipocyte differentiation itself and is known as determination. In the second phase, known as terminal differentiation, the preadipocytes acquire all the features of mature adipocytes including the necessary machinery for lipid biosynthesis and transport, and the secretion of adipocyte-specific proteins such as leptin or adiponectin.
The murine 3T3-L1 or 3T3-F442A cell lines have been extensively used to study the differentiation of committed preadipocytes into adipocytes, because they are thought to recapitulate faithfully the main steps of the in vivo differentiation process.Citation28,Citation29 To study the earlier steps of the process, that is, the commitment of pluripotent precursors into preadipocytes, other cell types can be used such as totipotent embryonic stem cells (ESCs) that can give rise to any cell type, or multipotent MSCs that can develop into fat, muscle, cartilage or bone.Citation30,Citation31 By using these tools, the main transcription factors involved in the differentiation of adipocytes have been and are still being identified. However, in recent years this well-known scenario has been considerably widened by the identification of novel factors that participate in the regulation of adipogenesis, either favoring or inhibiting it, through their effects on chromatin ().
Commitment of Pluripotent Precursors into Preadipocytes
Chromatin dynamics in pluripotent cells.
The chromatin of pluripotent cells possesses a number of features of its own. Pluripotent cells display highly dynamic and heterogeneous chromatin, with a high level of decondensation and diffuse binding of heterochromatin protein HP1.Citation32,Citation33 Interestingly, these features were observed in the pluripotent MSC line 10T1/2, which can give rise to preadipocytes, myoblasts and chondroblast, but not in the still undifferentiated but already committed C2C12 myoblasts, Citation32 probably reflecting the genetic flexibility of pluripotent cells and how it gets restricted as differentiation proceeds and the number of cell fate choices diminishes. In order to maintain chromatin plasticity, pluripotent cells express a wide range of chromatin regulators, and their loss usually results in aberrant differentiation. Thus, ESCs lacking the BAF250a subunit of the SWI/SNF chromatin remodeling complex are unable to differentiate into adipocytes or cardiomyocytes, but are still capable of differentiating into neurons.Citation34 These data suggest that the role of particular chromatin regulators in differentiation is lineagedependent, probably reflecting the subset of promoters that they regulate.
In accordance with this highly dynamic state of their chromatin, pluripotent cells are enriched in epigenetic signals associated with gene activity, such as histone acetylation or H3K4 methylation, that can be found even in silenced genes.Citation35 Most strikingly, the histones of developmentally important genes display a bivalent epigenetic mark in ESCs.Citation36 The promoters of genes that regulate cell fate decisions, including several markers of adipogenesisCitation37,Citation38 present a large region of the silencing mark H3K27me3 and smaller regions of the activation-related mark H3K4me3.Citation35–Citation37 This bivalent signal ensures that developmentally important genes remain silent but poised for transcription. H3K27 methylation is mediated by the Polycomb group (PcG) proteins, a family of transcriptional repressors that play a crucial role in the maintenance of pluripotency by repressing differentiation-related genes.Citation37,Citation39 In the absence of the H3K27 methyltransferase Eed or the H3K4 methylation regulator PTIP, resulting in alteration of these histone signals, developmental genes are expressed aberrantly and spontaneous differentiation occurs.Citation35,Citation40 On the other hand, induction of adipogenic differentiation results in H3K27 demethylation specifically occurring in adipogenic promoters, thus establishing the definitive activated state of this subset of genes by erasing this silencing mark.Citation38
Developmentally important genes are also marked by DNA methylation in their promoter regions, a complementary mechanism to ensure gene repression.Citation39,Citation41 In fact, most of the silenced genes presenting the H3K27me3/H3K4me3 bivalent histone mark display either PcG binding or DNA methylation as an additional silencing mechanism.Citation39,Citation42 Once differentiation is induced, lineage-specific genes are DNA demethylated whereas pluripotency genes are methylated correlating with their silencing.Citation42 Several lines of evidence support the fact that DNA demethylation plays a significant role in the cell fate decision of MSCs, at least in part by inducing expression of genes responsible for lineage commitment. Thus, treatment of the pluripotent cell line 10T1/2 with the methyltransferase inhibitor 5-azacytidine results in random DNA demethylation and cell differentiation. Interestingly, adipogenesis is associated in this model with DNA demethylation of the bmp4 gene.Citation43 The secreted factor BMP4 favors commitment of MSCs to the adipogenic lineage, and its increased expression correlates with the capacity of these cells to undergo adipogenesis.Citation44 Similarly, under 5-azacytidine treatment, commitment of 10T1/2 cells to the myogenic lineage correlates with DNA demethylation of the promoter region of the myogenic transcription factor myod gene.Citation45 In accordance with all these data, the promoters of adipogenic genes such as pparg2 or leptin are unmethylated in isolated adipose tissue stromal cells enriched in preadipocytes, whereas myogenic and endothelial promoters are methylated,Citation46 indicating that these cells have already committed to an adipogenic fate and additionally supporting a role for DNA methylation on lineage selection and cell differentiation. Moreover, increased levels of pparg2 promoter methylation have been described in visceral adipose tissue of several mouse models of diabetesCitation47 indicating that the gene needs to remain unmethylated in order to maintain the mature characteristics of the cells. Surprisingly, DNA demethylation in the exon 1 of the Rho guanine nucleotide exchange factor WEGF takes place during adipogenesis concurrently with its progressive silencing,Citation48 demonstrating that the relationship between DNA methylation and transcription may not be as straightforward as usually assumed.
Higher-order chromatin structure also regulates gene expression and thus cell differentiation. During differentiation of porcine MSCs into adipocytes, adipogenic genes such as those coding for transcription factors PPARγ (peroxisome proliferator activated receptor-γ) and C/EBPα(CCAAT/enhancer binding protein-α) are repositioned from the nuclear periphery to the nuclear interior coinciding with their increased transcription.Citation49 Interestingly, we have previously observed a similar change in the nuclear pattern of H3K4me2 during adipogenesis.Citation50 In 3T3-L1 preadipocytes, H3K4me2, which was observed to be enriched in the promoters of still silent adipogenic genes such as the hormone adiponectin, was found strongly enriched along the nuclear border in inmature cells and dramatically rearranged towards the interior nucleoplasma in differentiated cells.Citation50 The region along the nuclear envelope is occupied by mid-to-late replicating chromatin in 3T3-L1 cells, and mostly includes silent genes.Citation51 Taking together all these results it is tempting to speculate that histone modifications and nuclear location collaborate in regulating transcription of adipogenic genes during adipogenesis. These data are in accordance with the idea that chromatin marks regulate and/or reflect the higher-order chromatin structure.Citation52,Citation53
Factors regulating commitment to the adipose lineage.
Several factors involved in cell commitment and differentiation have been identified and characterized (). Regulators that induce commitment into one lineage often inhibit the differentiation along the other, for example the nuclear receptor PPARγ is a major inducer of adipogenesis whose activation inhibits osteoblastogenesis. Inducers of osteoblastogenesis such as the canonical Wnt/β-catenin pathway suppress the trans-activation and expression of PPARγ in MSCs,Citation54 by activating the histone methyltransferase (HMT) SETDB1.Citation55,Citation56 SETDB1 together with NLK (Nemo-like kinase) and CHD7 (chromodomain helicase DNA binding protein-7) constitutes a corepressor complex that represses PPARγ trans-activation through H3K9 methylation of its target promoters. At the same time, Wnt-5a activates the transcription factor Runx2, a known inductor of ostoeblastogenesis. Citation55,Citation56 Similarly, in vitro studies have shown that attenuation of PPARγ activity by overexpression of the histone deacetylase (HDAC) Sirt1 in MSCs also blocks adipogenesis while favoring osteoblastogenesis.Citation57,Citation58 Sirt1 binds to the promoter of PPARγ target genes together with corepressors NCoR/SMRT,Citation58 resulting in gene silencing.
On the other hand, a family of tension-responsive factors, referred to as tension-induced/inhibited proteins (TIPs), is involved in the determination of the adipogenic versus myogenic lineage from MSCs.Citation59 The TIP family is composed by at least eight members, all of whom possess a SANT (switching-defective protein 3, adaptor 2, nuclear receptor corepresor and transcription factor IIIB) domain,Citation60 characteristic of proteins with chromatin-modifying activities. Therefore, these proteins possibly exert their effects through chromatin remodeling of their target promoters. TIP-1 is induced by stretch and promotes smooth muscle differentiation by binding to the promoter of myogenic transcription factor Serum Response Factor (srf ). TIP-3, on the contrary, is suppressed by stretch and promotes adipogenesis in nonstretched MSCs by binding to the pparg gene promoter.Citation59 A recently identified member of the family, TIP-6 was shown to bind to the pparg promoter in NIH-3T3 fibroblasts and induce adipogenesis by recruiting the HAT p300 through interaction with the SANT domain, resulting in increased histone acetylation and expression of pparg.Citation60
Origins of brown adipose tissue.
The existence of a common adipose precursor cell for brown and white fat cells was supported by the ability of white adipocytes to transdifferentiate into BAT cells under certain conditions.Citation61–Citation64 Several in vitro studies support the differentiation of white preadipocytes into brown adipocytes and aim to identify the factors implicated in this switch in cell fate. Thus, functional inactivation of the retinoblastoma protein (pRb) in mouse embryo fibroblasts (MEFs) and in white preadipocytes results in increased differentiation of adipose cells with a gene expression pattern consistent with that of brown adipocytes.Citation65 On the other hand, receptor-interacting protein 140 (rip140) knockout mice exhibit a lean phenotype due to an increase in BAT-specific genes.Citation66 RIP140 nucleates a repressive complex containing histone deacetylases HDAC1 and HDAC3, the H3K9 methyltransferase G9 and DNA methyltransferases Dnmt1, Dnmt3a/3b, that is recruited to the ucp1 promoter during white adipocyte differentiation to mediate ucp1 gene repression by DNA methylation and histone hypoacetylation, maintaining a white phenotype.Citation67,Citation68
Interestingly, a series of recent works have demonstrated that brown and white preadipocytes arise from different precursor cells, with brown preadipocytes being strikingly related to myoblasts. Citation69–Citation71 Seale et al.Citation70 have shown by means of lineage tracking studies that skeletal muscle progenitor cells can give rise to either muscle or brown fat cells, but not white fat cells. The decision of this precursor cell to differentiate into muscle or brown adipocytes is controlled by the expression of PRMD16, a 140 kD PR (PRD1-BF-1RIZ1 homologous)-domain containing proteinCitation70,Citation72 that activates a number of brown adipocyte genes, such as UCP1 or the cofactor PGC1α, when expressed in cultured white preadipocytes or in white fat depots in vivo. Moreover, PRMD16 selectively represses white fat-specific genes such as the hormone resistin by interacting with the corepressors C-terminal-binding protein-1 and 2 (CtBP1, CtBP2). Recruitment of PGC1γ to the PRMD16 complex displaces CtBP allowing PRMD16 to activate brown adipocyte-specific genes.Citation73 Recently it has been shown that adipogenic transcription factor C/EBPγ is the critical partner in the PRDM16 transcriptional complex,Citation74 although the presence of histone modifying enzymes or chromatin-associated proteins has not been described yet.
However, an alternative origin of brown fat cells in vivo is also documented, as lineage tracking studies show that brown adipocytes in mice adapted to cold have never expressed the myogenic marker myf5 contrary to what happens with adipocytes in the conventional BAT deposits,Citation70 thus giving support to the traditional idea that white adipocytes may differentiate into brown fat cells.
Differentiation of Preadipocytes
Chromatin remodeling in adipogenesis.
Taking the 3T3-L1 cell line as a model of white adipocyte differentiation, the transcriptional cascade consists in a reduced number of transcription factors acting in time-controlled fashion. The increased expression and protein accumulation of C/EBPγ and C/EBPδ in the first hours of differentiation stimulate expression of C/EBPα and PPARγ, which in turn maintain the expression of each other.Citation4,Citation5 C/EBPα and PPARγ are considered the master regulators of adipogenesis and directly control transcription of most adipogenic genes.Citation75,Citation76 However, PPARγ is the only known factor that is both necessary and sufficient to induce adipogenesisCitation77–Citation79 and there are no other factors described to date that promote adipogenesis in its absence. Consistent with that, most pro-adipogenic factors and cofactors seem to act precisely through the induction of PPARγ expression or activity, whereas most inhibitors of adipogenesis may function in part by blocking PPARγ.
In preadipocytes, C/EBPγ and C/EBPδ are already bound to the cepba and pparg promoters,Citation80 while PPARγ is bound to the promoters of its target genes in association with a repressive complex composed of pRb and HDAC3,Citation81 (). When differentiation is induced, recruitment by C/EBPγ of the chromatin remodeling complex SWI/SNF promotes transcription of the pparg gene.Citation82 In the case of the cebpa promoter, C/EBPγ is initially blocked by a repressive complex constituted by mSin3A/HDAC1, but once PPARγ protein accumulates, it is able to target HDAC1 to the 26S proteasome, causing its degradation and allowing activation of cebpa expression.Citation83 Glucocorticoids have also been shown to exert the same effect,Citation84 in this case mediated by the HAT GCN5, which acetylates C/EBPβ within a cluster of lysine residues, resulting in dissociation of the interaction between C/EBPβ and HDAC1. Once C/EBPα accumulates in the nucleus of differentiating cells, it displaces C/EBPβ from the promoters of late adipogenic genes, such as leptin, adiponectin or pparg itself.Citation75,Citation76,Citation80,Citation82 Like C/EBPβ, C/EBPα promotes transcription of its target genes by recruiting the SWI/SNF complex.Citation85 At the same time, C/EBPα promotes cell cycle exit by repressing E2F-dependent promoters also through its interaction with the SWI/SNF complex.Citation86,Citation87 In agreement with its pleiotropic role in adipogenesis, knockdown of the snf5/Ini1 subunits of the SWI/SNF complex blocks adipogenic differentiation of 3T3-L1 and MSCs.Citation88
Histone modifications in adipogenesis.
Given the preponderance of HAT/HDAC interactions with adipogenic regulators, it is easy to recognize the key role that histone acetylation plays in adipogenesis. Histone acetylation increases at the promoters of adipogenic marker genes during adipocyte differentiation, correlating with their increased expression,Citation50 whereas a decrease in HDAC expression takes place throughout this process.Citation89 Furthermore, blocking HDAC activity with general inhibitors such as trichostatin A increases adipogenesis, whereas overexpression of HDAC1 impairs it.Citation81,Citation84,Citation89 Unexpectedly, a negative effect of HDAC inhibitors on adipogenesis has also been described by some works.Citation90,Citation91 Moreover, treatment of mature adipocytes with the HDAC inhibitor apicidin but not other inhibitors such as sodium butyrate, induced dedifferentiation of mature adipocytes. Citation90 This apparent contradiction may be due to the different specificities of the HDAC inhibitors used, as particular inhibitors present different affinity for the different families of HDACs that do exist. Also, these data may suggest that HDACs may play a key role in silencing anti-adipogenic genes during adipogenesis and in mature cells. In this sense, transcription factor KLF6 promotes adipogenesis by silencing the preadipocyte marker gene dlk1 through HDAC3 recruitment to the dlk1 promoter.Citation92 Accordingly, we have observed that the promoter region of dlk1 is acetylated in 3T3-L1 fibroblasts, when the gene is highly expressed, becoming progressively deacetylated throughout differentiation as the gene is gradually silenced.Citation50
On the initial phases of adipogenesis, PPARγ is blocked by its association with a repressive pRb-HDAC3 complex resulting in the recruitment of deacetylase activity to PPARγ target promoters and their consequent repression.Citation81 When pRb is inactivated by phosphorylation upon induction of differentiation, this complex is dissociated and PPARγ is then free to interact with HATs CBP/p300 and activate transcription.Citation81,Citation93,Citation94 Downregulation of CBP/p300 expression significantly reduces adipocyte differentiation. Citation93,Citation95 Similarly, coexpression of HAT SRC1 with PPARγ enhances the transcriptional activity of the factor and adipogenesis, whereas coexpression of corepressor NCoR suppresses it.Citation96 In 3T3-L1 cells, addition of PPARγ ligands breaks the PPARγ/NCoR complex and results in activation of PPARγ transcriptional activity.Citation97,Citation98 At the same time, binding to a ligand also enhances interaction of PPARγ with CBP/p300, although this interaction may also happen in the absence of ligand.Citation94
Cyclin D1 blocks PPARγ activity by recruiting HDAC1 and HDAC3 as well as the H3K9 HMT SUV39H1 to PPARγ target promoters, resulting in decreased expression of adipogenic genes.Citation99 Cyclin D3 and its kinase partner CDK6, on the other hand, have a positive role in differentiation by binding to and phosphorylating PPARγ resulting in transcriptional activation of the factor.Citation100 Thus, a relay of cyclins occurs during adipogenesis. At the beginning of differentiation elevated levels of Cyclin D1 block PPARγ transcriptional activity, whereas after mitotic clonal expansion, the decrease of Cyclin D1 and increase of Cyclin D3 ensure the activation of the factor.
Together with acetylation, histone methylation has been shown to participate actively in the regulation of adipocyte differentiation. Mouse embryos deficient for the histone arginine methyltransferase CARM1 display reduced BAT and silencing of the enzyme in 3T3-L1 cells results in impaired adipogenesis due to decreased PPARγ trans-activation.Citation101 Deletion of the H3K9 demethylase JMJD1A in mice results in obesity and metabolic syndrome.Citation102,Citation103 JMJD1A is recruited to the PPRE of the ucp1 gene, resulting in H3K9 demethylation and increased transcription. Citation103 Decreased UCP1 expression in absence of JMJD1A may contribute to defective thermogenesis and fat burning in the knockout mice. In contrast, targeted inactivation of the H3K4 HMT MLL3 in mouse results in PPARγ-dependent impaired adipogenesis.Citation104 MLL3 or its paralogue MLL4 form the ASCOM complexes that regulate the transcriptional activity of PPARγ by being recruited to PPARγ target promoters, such as that of lipid transporter aP2, and increasing H3K4 methylation and consequently transcription.Citation104
We have observed that the promoters of key adipogenic genes, such as the hormone apm1, present significant levels of H3K4me2 in preadipocytes, when these genes are not expressed. Moreover, this signal coincides with significant loading of RNA Pol II at the same regions, suggesting that this mark is related to developmentally poised chromatin.Citation50 At the same time the apm1 promoter has been shown to present detectable levels of H3K9me2 in preadipocytes,Citation105 thus indicating the existence of a bivalent mark in this promoter in precursor cells. During terminal differentiation, significant increases in both histone H3 acetylation and H3K4me3 coincide with upregulation of the main adipogenic genes, whereas a concomitant decrease in histone acetylation and H3K4 trimethylation is observed in the promoter of the progressively silenced preadipocyte-specific gene dlk1.Citation50 Accordingly with this increase in H3K4me3 during adipogenesis, a recent report has shown that PTIP, a protein that associates with H3K4 HMTs MLL3 and MLL4 and regulates histone methylation, is essential for adipogenesis.Citation106,Citation107 Deletion of PTIP impairs the enrichment of H3K4me3 and the loading of RNA Pol II on pparg and cebpa promoters, consequently impairing induction of PPARγ and C/EBPα expression.
Monomethylation of H4K20 also increases in adipogenic genes during adipogenesis and has been shown to play an important role in this process.Citation108 In a feedback loop, the H4K20 HMT Set7/Setd8 is upregulated by PPARγ during adipogenesis, at the time that this enzyme positively regulates the expression of PPAR? and its target genes trough H4K20 monomethylation.
The role of miRNAs in adipogenesis.
In recent years a number of works have demonstrated a key role of microRNAs in cell differentiation, including adipogenesis. MicroRNAs are single-stranded RNAs of 19–25 nucleotides that originate from endogenous hairpin-shaped transcripts. These miRNAs interact with their targets by base-pairing and result in silencing by either translational repression of cleavage of the target RNA. A wide number of miRNAs, such as miR-103 and miR-143 increase during in vivo and in vitro adipocyte differentiation and their ectopic expression increases adipogenesis.Citation109 Interestingly, these miRNAs were found to be downregulated in mouse models of obesity, thus suggesting that they play a role in phenotypic maintenance and function of mature adipose cells. Another report showed downregulation of miR-31, miR-125b-5p and miR-326 during adipocyte differentiation from adipose-derived stem cells, suggesting a role for these miRNAs in this process.Citation110 miR-27 has been shown to downregulate PPARγ and C/EBPα expression and subsequently plays a key role in adipogenesis.Citation111 The expression of miR-27 decreases during adipogenesis and, interestingly, was found to be increased in adipose tissue of obese mouse. Finally, significant correlation of the levels of differents miRNAs with metabolic parameters such as fasting plasma glucose or circulating leptin and adiponectin has also been reported,Citation112 highlighting the importance of these factors in the regulation of metabolism.
Concluding Remarks
Epigenetics has become an aspect that can not be overlooked when attempting to understand the regulation of gene expression. In this review, we have attempted to put into perspective recent studies highlighting the interplay between the chromatin environment and transcriptional regulation in the context of adipocyte differentiation. A number of works have revealed the importance of epigenetics in the transition from undifferentiated to differentiated cells. Adipogenesis appears to involve the combined regulation of chromatin structure, histone modifications and the regulation by miRNAs. All these mechanisms are employed by sequence-specific transcription factors to coordinately regulate expression of sets of genes that either maintain pluripotency, direct cells towards a particular lineage, or maintain the differentiated state of the mature cells. The continuing study and identification of these epigenetic signals and the factors that are responsible of their establishment and turnover would ensure the discovery of new targets for the treatment of obesity and its associated diseases.
Abbreviations
BAT | = | brown adipose tissue |
BMP | = | bone morphogenetic protein |
C/EBP | = | CCAAT/enhancer binding protein |
CtBP | = | C-terminal-binding protein |
ESCs | = | embryonic stem cells |
HAT | = | histone acetyltransferase |
HDAC | = | histone deacetylase |
HMT | = | histone methyltransferase |
MEFs | = | mouse embryo fibroblasts |
MSCs | = | mesenchymal stem cells |
NCoR | = | nuclear corepressor |
PGC1 | = | PPARγ coactivator 1 |
PPAR | = | peroxisome-proliferator associated receptor |
PPRE | = | PPAR response element |
RIP140 | = | receptor interacting protein 140 |
SANT | = | switching-defective protein 3, adaptor 2, nuclear receptor corepresor, and transcription factor IIIB domain |
SRF | = | serum response factor |
TIP | = | tension-induced/inhibited protein |
UCP1 | = | uncoupling protein 1 |
WAT | = | white adipose tissue |
Figures and Tables
Figure 1 Cell fate decision of MSCs. Commitment of MSCs into the chondro-osteogenic lineage has been shown to rely on the expression of the master transcription factor Runx2.Citation114–Citation116 Sox proteins are the major transcription factors that regulate chondrogenic differentiation, whereas Runx2/Cbfa1 and Osterix/Osx participate in both osteocyte and chondrocyte differentiation. The Wnt canonical pathway activates Runx2 and induces an osteogenic fate,Citation117 at the expense of the adipogenic lineage.Citation55,Citation56 On the other hand, BMP2 induces the expression of Sox9 but represses Runx2, thus driving MSCs into chondrogenesis. Commitment to the myogenic lineage and myogenesis are governed by muscle-specific transcription factors that include Myf5, MyoD, myogenin and MRF4,Citation118,Citation119 as well as the induction of TIP-1 expression, whereas expression of TIP-3 directs the progenitors to the adipogenic lineage.Citation59 The myogenic precursors can give rise to either myocytes or brown adipocytes, depending on the absence or presence of adipogenic PRDM16.Citation70,Citation72 However, white adipocytes can also be transdifferentiated into brown adipocytes under different circumstances.
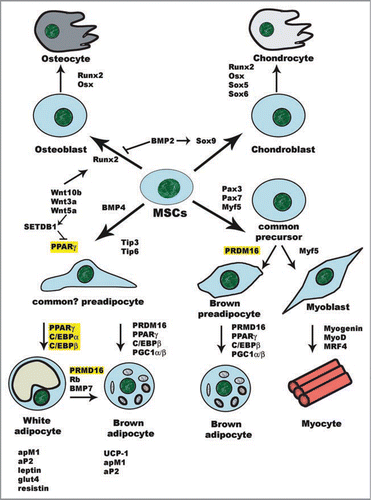
Figure 2 Epigenetic events during adipocyte differentiation. The schematics show the main epigenetic events that take place during adipogenesis represented on a hypothetical promoter incorporating characteristics from different adipogenic genes. In preadipocytes, C/EBPβ is already bound to the promoters of its target genes including pparg and cebpaCitation75,Citation76,Citation80,Citation82 but remains inactive by its interaction with HDAC1/mSin3A.Citation83,Citation84 Similarly, PPARγ is bound to its target promoters in association with a number of repressors including HDACs and histone methyltransferases.Citation57,Citation58,Citation75,Citation76,Citation80–Citation82,Citation97,Citation99 At this stage, most adipogenic genes are characterized by bivalent histone marks, such as methylation of H3K27, H3K9 and H3K4.Citation35–Citation38,Citation50 Methylation of DNA is also foundCitation120 as well as recruitment of a still inactive RNA Pol II.Citation50 Upon induction of differentiation, pRB phosporylation results in its inactivation and HDAC1 is dislodged from PPARγ which is now free to bind CBP/p300 and a number of other coactivators.Citation81,Citation94,Citation95,Citation100 In the early phases of differentiation, increased amounts of PPARγ displace HDAC1 from C/EBPβ allowing the factor to recruit the SWI/SNF remodeling complex and stimulate transcription of cebpa.Citation83 Glucocorticoids have the same effect by stimulating C/EBPβ acetylation by GCN5.Citation84,Citation113 High levels of PPARγ and C/EBPα direct terminal differentiation. Citation75,Citation76 Decrease of H3K27/H3K9 methylation is observed in adipogenic promoters, accompanied by increased histone acetylation and H3K4/H3K20 methylation.Citation38,Citation50,Citation108 Another characteristic feature during terminal differentiation of adipocytes is the spread of di and tri-methylation of H3K4, acetylation of H3 and the Pol II to the coding region of the adipogenic genes, coinciding with high levels of transcription of those genes.Citation38,Citation50
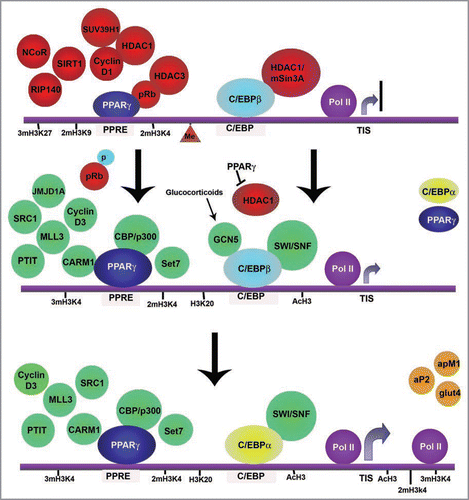
Table 1 Histone modifying enzymes involved in adipogenesis
Acknowledgements
This work was supported by grants BFU2006-14251/BMC and SAF2006-07382 from the Spanish Ministry of Science and Innovation (MICINN) awarded to M.P. and R.G. respectively. We are grateful to Rosa Gasa, Perla Kaliman and Felicia Hanzu for critical reading of the manuscript.
References
- Goldberg AD, Allis CD, Bernstein E. Epigenetics: a landscape takes shape. Cell 2007; 128:635 - 638
- Bernstein BE, Meissner A, Lander ES. The mammalian epigenome. Cell 2007; 128:669 - 681
- Cinti S. The adipose organ. Prostaglandins Leukot Essent Fatty Acids 2005; 73:9 - 15
- Rosen ED, MacDougald OA. Adipocyte differentiation from the inside out. Nat Rev Mol Cell Biol 2006; 7:885 - 896
- Farmer SR. Transcriptional control of adipocyte formation. Cell Metab 2006; 4:263 - 273
- Fuks F. DNA methylation and histone modifications: teaming up to silence genes. Curr Opin Genet Dev 2005; 15:490 - 495
- Jenuwein T, Allis CD. Translating the histone code. Science 2001; 293:1074 - 1080
- Couture JF, Trievel RC. Histone-modifying enzymes: encrypting an enigmatic epigenetic code. Curr Opin Struct Biol 2006; 16:753 - 760
- Kouzarides T. Chromatin modifications and their function. Cell 2007; 128:693 - 705
- Kristjuhan A, Walker J, Suka N, Grunstein M, Roberts D, Cairns BR, et al. Transcriptional inhibition of genes with severe histone H3 hypoacetylation in the coding region. Mol Cell 2002; 10:925 - 933
- Kurdistani SK, Tavazoie S, Grunstein M. Mapping global histone acetylation patterns to gene expression. Cell 2004; 117:721 - 733
- Santos-Rosa H, Schneider R, Bannister AJ, Sherriff J, Bernstein BE, Emre NC, et al. Active genes are tri-methylated at K4 of histone H3. Nature 2002; 419:407 - 411
- Bernstein BE, Humphrey EL, Erlich RL, Schneider R, Bouman P, Liu JS, et al. Methylation of histone H3 Lys 4 in coding regions of active genes. Proc Natl Acad Sci USA 2002; 99:8695 - 8700
- Schneider R, Bannister AJ, Myers FA, Thorne AW, Crane-Robinson C, Kouzarides T. Histone H3 lysine 4 methylation patterns in higher eukaryotic genes. Nat Cell Biol 2004; 6:73 - 77
- Lachner M, O’Carroll D, Rea S, Mechtler K, Jenuwein T. Methylation of histone H3 lysine 9 creates a binding site for HP1 proteins. Nature 2001; 410:116 - 120
- Papp B, Muller J. Histone trimethylation and the maintenance of transcriptional ON and OFF states by trxG and PcG proteins. Genes Dev 2006; 20:2041 - 2054
- Gesta S, Tseng YH, Kahn CR. Developmental origin of fat: tracking obesity to its source. Cell 2007; 131:242 - 256
- Billon N, Monteiro MC, Dani C. Developmental origin of adipocytes: new insights into a pending question. Biol Cell 2008; 100:563 - 575
- Trayhurn P, Wood IS. Adipokines: inflammation and the pleiotropic role of white adipose tissue. Br J Nutr 2004; 92:347 - 355
- Kissebah AH, Peiris AN. Biology of regional body fat distribution: relationship to non-insulin-dependent diabetes mellitus. Diabetes Metab Rev 1989; 5:83 - 109
- Gesta S, Bluher M, Yamamoto Y, Norris AW, Berndt J, Kralisch S, et al. Evidence for a role of developmental genes in the origin of obesity and body fat distribution. Proc Natl Acad Sci USA 2006; 103:6676 - 6681
- Cannon B, Nedergaard J. Brown adipose tissue: function and physiological significance. Physiol Rev 2004; 84:277 - 359
- Farmer SR. Molecular determinants of brown adipocyte formation and function. Genes Dev 2008; 22:1269 - 1275
- Nedergaard J, Bengtsson T, Cannon B. Unexpected evidence for active brown adipose tissue in adult humans. Am J Physiol Endocrinol Metab 2007; 293:444 - 452
- Cypess AM, Lehman S, Williams G, Tal I, Rodman D, Goldfine AB, et al. Identification and importance of brown adipose tissue in adult humans. N Engl J Med 2009; 360:1509 - 1517
- Tiraby C, Langin D. Conversion from white to brown adipocytes: a strategy for the control of fat mass?. Trends Endocrinol Metab 2003; 14:439 - 441
- Billon N, Iannarelli P, Monteiro MC, Glavieux-Pardanaud C, Richardson WD, Kessaris N, et al. The generation of adipocytes by the neural crest. Development 2007; 134:2283 - 2292
- Green H, Kehinde O. An established preadipose cell line and its differentiation in culture II. Factors affecting the adipose conversion. Cell 1975; 5:19 - 27
- Green H, Kehinde O. Spontaneous heritable changes leading to increased adipose conversion in 3T3 cells. Cell 1976; 7:105 - 113
- Pinney DF, Emerson CP Jr. 10T1/2 cells: an in vitro model for molecular genetic analysis of mesodermal determination and differentiation. Environ Health Perspect 1989; 80:221 - 227
- Kassem M. Mesenchymal stem cells: biological characteristics and potential clinical applications. Cloning Stem Cells 2004; 6:369 - 374
- Meshorer E, Yellajoshula D, George E, Scambler PJ, Brown DT, Misteli T. Hyperdynamic plasticity of chromatin proteins in pluripotent embryonic stem cells. Dev Cell 2006; 10:105 - 116
- Bartova E, Krejci J, Harnicarova A, Kozubek S. Differentiation of human embryonic stem cells induces condensation of chromosome territories and formation of HP1 foci. Differentiation 2008; 76:24 - 32
- Gao X, Tate P, Hu P, Tjian R, Skarnes WC, Wang Z. ES cell pluripotency and germ-layer formation require the SWI/SNF chromatin remodeling component BAF250a. Proc Natl Acad Sci USA 2008; 105:6656 - 6661
- Azuara V, Perry P, Sauer S, Spivakov M, Jorgensen HF, John RM, et al. Chromatin signatures of pluripotent cell lines. Nat Cell Biol 2006; 8:532 - 538
- Bernstein BE, Mikkelsen TS, Xie X, Kamal M, Huebert DJ, Cuff J, et al. A bivalent chromatin structure marks key developmental genes in embryonic stem cells. Cell 2006; 125:315 - 326
- Bracken AP, Dietrich N, Pasini D, Hansen KH, Helin K. Genome-wide mapping of Polycomb target genes unravels their roles in cell fate transitions. Genes Dev 2006; 20:1123 - 1136
- Noer A, Lindeman LC, Collas P. Histone H3 modifications associated with differentiation and long-term culture of mesenchymal adipose stem cells. Stem Cells Dev 2009; 18:725 - 736
- Fouse SD, Shen Y, Pellegrini M, Cole S, Meissner A, Van Neste L, et al. Promoter CpG methylation contributes to ES cell gene regulation in parallel with Oct4/Nanog, PcG complex, and histone H3 K4/K27 trimethylation. Cell Stem Cell 2008; 2:160 - 169
- Kim D, Patel SR, Xiao H, Dressler GR. The role of PTIP in maintaining embryonic stem cell pluripotency. Stem Cells 2009; 27:1516 - 1523
- Meissner A, Mikkelsen TS, Gu H, Wernig M, Hanna J, Sivachenko A, et al. Genome-scale DNA methylation maps of pluripotent and differentiated cells. Nature 2008; 454:766 - 770
- Mohn F, Weber M, Rebhan M, Roloff TC, Richter J, Stadler MB, et al. Lineage-specific polycomb targets and de novo DNA methylation define restriction and potential of neuronal progenitors. Mol Cell 2008; 30:755 - 766
- Bowers RR, Kim JW, Otto TC, Lane MD. Stable stem cell commitment to the adipocyte lineage by inhibition of DNA methylation: role of the BMP-4 gene. Proc Natl Acad Sci USA 2006; 103:13022 - 13027
- Tang QQ, Otto TC, Lane MD. Commitment of C3H10T1/2 pluripotent stem cells to the adipocyte lineage. Proc Natl Acad Sci USA 2004; 101:9607 - 9611
- Jones PA, Wolkowicz MJ, Harrington MA, Gonzales F. Methylation and expression of the Myo D1 determination gene. Philos Trans R Soc Lond B Biol Sci 1990; 326:277 - 2784
- Noer A, Sorensen AL, Boquest AC, Collas P. Stable CpG hypomethylation of adipogenic promoters in freshly isolated, cultured, and differentiated mesenchymal stem cells from adipose tissue. Mol Biol Cell 2006; 17:3543 - 3556
- Fujiki K, Kano F, Shiota K, Murata M. Expression of the PPARg gene is repressed by DNA methylation in visceral adipose tissue of mouse models of diabetes. BMC Biol 2009; 7:38
- Horii T, Morita S, Kimura M, Hatada I. Epigenetic regulation of adipocyte differentiation by a Rho guanine nucleotide exchange factor, WGEF. PLoS ONE 2009; 4:5809
- Szczerbal I, Foster HA, Bridger JM. The spatial repositioning of adipogenesis genes is correlated with their expression status in a porcine mesenchymal stem cell adipogenesis model system. Chromosoma 2009; 118:647 - 663
- Musri MM, Corominola H, Casamitjana R, Gomis R, Parrizas M. Histone H3 lysine 4 dimethylation signals the transcriptional competence of the adiponectin promoter in preadipocytes. J Biol Chem 2006; 281:17180 - 17188
- Dimitrova DS, Berezney R. The spatio-temporal organization of DNA replication sites is identical in primary, immortalized and transformed mammalian cells. J Cell Sci 2002; 115:4037 - 4051
- Maison C, Bailly D, Peters AH, Quivy JP, Roche D, Taddei A, et al. Higher-order structure in pericentric heterochromatin involves a distinct pattern of histone modification and an RNA component. Nat Genet 2002; 30:329 - 334
- Rice JC, Briggs SD, Ueberheide B, Barber CM, Shabanowitz J, Hunt DF, et al. Histone methyltransferases direct different degrees of methylation to define distinct chromatin domains. Mol Cell 2003; 12:1591 - 1598
- Bennett CN, Longo KA, Wright WS, Suva LJ, Lane TF, Hankenson KD, MacDougald OA. Regulation of osteoblastogenesis and bone mass by Wnt10b. Proc Natl Acad Sci USA 2005; 102:3324 - 3329
- Takada I, Mihara M, Suzawa M, Ohtake F, Kobayashi S, Igarashi M, et al. A histone lysine methyltransferase activated by non-canonical Wnt signalling suppresses PPARg transactivation. Nat Cell Biol 2007; 9:1273 - 1285
- Takada I, Suzawa M, Matsumoto K, Kato S. Suppression of PPAR transactivation switches cell fate of bone marrow stem cells from adipocytes into osteoblasts. Ann NY Acad Sci 2007; 1116:182 - 195
- Backesjo CM, Li Y, Lindgren U, Haldosen LA. Activation of Sirt1 decreases adipocyte formation during osteoblast differentiation of mesenchymal stem cells. Cells Tissues Organs 2009; 189:93 - 97
- Picard F, Kurtev M, Chung N, Topark-Ngarm A, Senawong T, Machado DO, et al. Sirt1 promotes fat mobilization in white adipocytes by repressing PPARg. Nature 2004; 429:771 - 776
- Jakkaraju S, Zhe X, Pan D, Choudhury R, Schuger L. TIPs are tension-responsive proteins involved in myogenic versus adipogenic differentiation. Dev Cell 2005; 9:39 - 49
- Badri KR, Zhou Y, Dhru U, Aramgam S, Schuger L. Effects of the SANT domain of tension-induced/inhibited proteins (TIPs), novel partners of the histone acetyltransferase p300, on p300 activity and TIP-6-induced adipogenesis. Mol Cell Biol 2008; 28:6358 - 6372
- Plum L, Rother E, Munzberg H, Wunderlich FT, Morgan DA, Hampel B, et al. Enhanced leptinstimulated Pi3k activation in the CNS promotes white adipose tissue transdifferentiation. Cell Metab 2007; 6:431 - 445
- Tiraby C, Tavernier G, Lefort C, Larrouy D, Bouillaud F, Ricquier D, et al. Acquirement of brown fat cell features by human white adipocytes. J Biol Chem 2003; 278:33370 - 33376
- Vogler O, Lopez-Bellan A, Alemany R, Tofe S, Gonzalez M, Quevedo J, et al. Structure-effect relation of C18 long-chain fatty acids in the reduction of body weight in rats. Int J Obes (Lond) 2008; 32:464 - 473
- Tseng YH, Kokkotou E, Schulz TJ, Huang TL, Winnay JN, Taniguchi CM, et al. New role of bone morphogenetic protein 7 in brown adipogenesis and energy expenditure. Nature 2008; 454:1000 - 1004
- Hansen JB, Jorgensen C, Petersen RK, Hallenborg P, De Matteis R, Boye HA, et al. Retinoblastoma protein functions as a molecular switch determining white versus brown adipocyte differentiation. Proc Natl Acad Sci USA 2004; 101:4112 - 4117
- Leonardsson G, Steel JH, Christian M, Pocock V, Milligan S, Bell J, et al. Nuclear receptor corepressor RIP140 regulates fat accumulation. Proc Natl Acad Sci USA 2004; 101:8437 - 8442
- Kiskinis E, Hallberg M, Christian M, Olofsson M, Dilworth SM, White R, et al. RIP140 directs histone and DNA methylation to silence Ucp1 expression in white adipocytes. EMBO J 2007; 26:4831 - 4840
- Christian M, Kiskinis E, Debevec D, Leonardsson G, White R, Parker MG. RIP140-targeted repression of gene expression in adipocytes. Mol Cell Biol 2005; 25:9383 - 9391
- Timmons JA, Wennmalm K, Larsson O, Walden TB, Lassmann T, Petrovic N, et al. Myogenic gene expression signature establishes that brown and white adipocytes originate from distinct cell lineages. Proc Natl Acad Sci USA 2007; 104:4401 - 4406
- Seale P, Bjork B, Yang W, Kajimura S, Chin S, Kuang S, et al. PRDM16 controls a brown fat/skeletal muscle switch. Nature 2008; 454:961 - 967
- Walden TB, Timmons JA, Keller P, Nedergaard J, Cannon B. Distinct expression of muscle-specific MicroRNAs (myomirs) in brown adipocytes. J Cell Physiol 2009; 218:444 - 449
- Seale P, Kajimura S, Yang W, Chin S, Rohas LM, Uldry M, et al. Transcriptional control of brown fat determination by PRDM16. Cell Metab 2007; 6:38 - 54
- Kajimura S, Seale P, Tomaru T, Erdjument-Bromage H, Cooper MP, Ruas JL, et al. Regulation of the brown and white fat gene programs through a PRDM16/CtBP transcriptional complex. Genes Dev 2008; 22:1397 - 1409
- Kajimura S, Seale P, Kubota K, Lunsford E, Frangioni JV, Gygi SP, Spiegelman BM. Initiation of myoblast to brown fat switch by a PRDM16-C/EBPb transcriptional complex. Nature 2009; 460:1154 - 1158
- Lefterova MI, Zhang Y, Steger DJ, Schupp M, Schug J, Cristancho A, et al. PPARg and C/EBP factors orchestrate adipocyte biology via adjacent binding on a genome-wide scale. Genes Dev 2008; 22:2941 - 2952
- Nielsen R, Pedersen TA, Hagenbeek D, Moulos P, Siersbaek R, Megens E, et al. Genome-wide profiling of PPARg:RXR and RNA polymerase II occupancy reveals temporal activation of distinct metabolic pathways and changes in RXR dimer composition during adipogenesis. Genes Dev 2008; 22:2953 - 2967
- Barak Y, Nelson MC, Ong ES, Jones YZ, Ruiz-Lozano P, Chien KR, et al. PPARg is required for placental, cardiac and adipose tissue development. Mol Cell 1999; 4:585 - 595
- Rosen ED, Sarraf P, Troy AE, Bradwin G, Moore K, Milstone DS, et al. PPARg is required for the differentiation of adipose tissue in vivo and in vitro. Mol Cell 1999; 4:611 - 617
- Rosen ED, Hsu CH, Wang X, Sakai S, Freeman MW, Gonzalez FJ, et al. C/EBPa induces adipogenesis through PPARg: a unified pathway. Genes Dev 2002; 16:22 - 26
- Salma N, Xiao H, Imbalzano AN. Temporal recruitment of CCAAT/enhancer-binding proteins to early and late adipogenic promoters in vivo. J Mol Endocrinol 2006; 36:139 - 151
- Fajas L, Egler V, Reiter R, Hansen J, Kristiansen K, Debril MB, et al. The retinoblastoma-histone deacetylase 3 complex inhibits PPARg and adipocyte differentiation. Dev Cell 2002; 3:903 - 910
- Salma N, Xiao H, Mueller E, Imbalzano AN. Temporal recruitment of transcription factors and SWI/SNF chromatin-remodeling enzymes during adipogenic induction of the PPARg nuclear hormone receptor. Mol Cell Biol 2004; 24:4651 - 4663
- Zuo Y, Qiang L, Farmer SR. Activation of CCAAT/enhancer-binding protein alpha (C/EBPa) expression by C/EBPb during adipogenesis requires a PPARgassociated repression of HDAC1 at the C/ebpa gene promoter. J Biol Chem 2006; 281:7960 - 7967
- Wiper-Bergeron N, Wu D, Pope L, Schild-Poulter C, Hache RJ. Stimulation of preadipocyte differentiation by steroid through targeting of an HDAC1 complex. EMBO J 2003; 22:2135 - 2145
- Pedersen TA, Kowenz-Leutz E, Leutz A, Nerlov C. Cooperation between C/EBPa TBP/TFIIB and SWI/SNF recruiting domains is required for adipocyte differentiation. Genes Dev 2001; 15:3208 - 3216
- Muller C, Calkhoven CF, Sha X, Leutz A. C/EBPa requires a SWI/SNF complex for proliferation arrest. J Biol Chem 2004; 279:7353 - 7358
- Wang GL, Shi X, Salisbury E, Sun Y, Albrecht JH, Smith RG, et al. Cyclin D3 maintains growth-inhibitory activity of C/EBPa by stabilizing C/EBPa-cdk2 and C/EBPa-Brm complexes. Mol Cell Biol 2006; 26:2570 - 2582
- Caramel J, Medjkane S, Quignon F, Delattre O. The requirement for SNF5/INI1 in adipocyte differentiation highlights new features of malignant rhabdoid tumors. Oncogene 2008; 27:2035 - 2044
- Yoo EJ, Chung JJ, Choe SS, Kim KH, Kim JB. Downregulation of histone deacetylases stimulates adipocyte differentiation. J Biol Chem 2006; 281:6608 - 6615
- Kim SN, Choi HY, Kim YK. Regulation of adipocyte differentiation by histone deacetylase inhibitors. Arch Pharm Res 2009; 32:535 - 541
- Lagace DC, Nachtigal MW. Inhibition of histone deacetylase activity by valproic acid blocks adipogenesis. J Biol Chem 2004; 279:18851 - 18860
- Li D, Yea S, Li S, Chen Z, Narla G, Banck M, et al. Kruppel-like factor-6 promotes preadipocyte differentiation through HDAC3-dependent repression of DLK1. J Biol Chem 2005; 280:26941 - 26952
- Chen S, Johnson BA, Li Y, Aster S, McKeever B, Mosley R, et al. Both coactivator LXXLL motifdependent and —independent interactions are required for PPARg function. J Biol Chem 2000; 275:3733 - 3736
- Gelman L, Zhou G, Fajas L, Raspe E, Fruchart JC, Auwerx J. p300 interacts with the N- and C-terminal part of PPARg2 in a ligand-independent and -dependent manner, respectively. J Biol Chem 1999; 274:7681 - 7988
- Takahashi N, Kawada T, Yamamoto T, Goto T, Taimatsu A, Aoki N, et al. Overexpression and ribozyme-mediated targeting of transcriptional coactivators CREBbinding protein and p300 revealed their indispensable roles in adipocyte differentiation through the regulation of PPARg. J Biol Chem 2002; 277:16906 - 16912
- Nolte RT, Wisely GB, Westin S, Cobb JE, Lambert MH, Kurokawa R, et al. Ligand binding and co-activator assembly of the PPARg. Nature 1998; 395:137 - 143
- Yu C, Markan K, Temple KA, Deplewski D, Brady MJ, Cohen RN. The nuclear receptor corepressors NCoR and SMRT decrease PPARg transcriptional activity and repress 3T3-L1 adipogenesis. J Biol Chem 2005; 280:13600 - 13605
- Guan HP, Ishizuka T, Chui PC, Lehrke M, Lazar MA. Corepressors selectively control the transcriptional activity of PPARg in adipocytes. Genes Dev 2005; 19:453 - 461
- Fu M, Rao M, Bouras T, Wang C, Wu K, Zhang X, et al. Cyclin D1 inhibits PPARg-mediated adipogenesis through histone deacetylase recruitment. J Biol Chem 2005; 280:16934 - 16941
- Sarruf DA, Iankova I, Abella A, Assou S, Miard S, Fajas L. Cyclin D3 promotes adipogenesis through activation of PPARg. Mol Cell Biol 2005; 25:9985 - 9995
- Yadav N, Cheng D, Richard S, Morel M, Iyer VR, Aldaz CM, et al. CARM1 promotes adipocyte differentiation by coactivating PPARg. EMBO Rep 2008; 9:193 - 198
- Inagaki T, Tachibana M, Magoori K, Kudo H, Tanaka T, Okamura M, et al. Obesity and metabolic syndrome in histone demethylase JHDM2a-deficient mice. Genes Cells 2009; 14:991 - 1001
- Tateishi K, Okada Y, Kallin EM, Zhang Y. Role of Jhdm2a in regulating metabolic gene expression and obesity resistance. Nature 2009; 458:757 - 761
- Lee J, Saha PK, Yang QH, Lee S, Park JY, Suh Y, et al. Targeted inactivation of MLL3 histone H3-Lys-4 methyltransferase activity in the mouse reveals vital roles for MLL3 in adipogenesis. Proc Natl Acad Sci USA 2008; 105:19229 - 19234
- Sakurai N, Mochizuki K, Goda T. Modifications of histone H3 at lysine 9 on the adiponectin gene in 3T3-L1 adipocytes. J Nutr Sci Vitaminol (Tokyo) 2009; 55:131 - 138
- Cho YW, Hong T, Hong S, Guo H, Yu H, Kim D, et al. PTIP associates with MLL3- and MLL4-containing histone H3 lysine 4 methyltransferase complex. J Biol Chem 2007; 282:20395 - 20406
- Cho YW, Hong S, Jin Q, Wang L, Lee JE, Gavrilova O, Ge K. Histone methylation regulator PTIP is required for PPARg and C/EBPa expression and adipogenesis. Cell Metab 2009; 10:27 - 39
- Wakabayashi K, Okamura M, Tsutsumi S, Nishikawa NS, Tanaka T, Sakakibara I, et al. The PPARg/RXRa heterodimer targets the histone modification enzyme PR-Set7/Setd8 gene and regulates adipogenesis through a positive feedback loop. Mol Cell Biol 2009; 29:3544 - 3555
- Xie H, Lim B, Lodish HF. MicroRNAs induced during adipogenesis that accelerate fat cell development are downregulated in obesity. Diabetes 2009; 58:1050 - 1057
- Tang YF, Zhang Y, Li XY, Li C, Tian W, Liu L. Expression of miR-31, miR-125b-5p and miR-326 in the Adipogenic Differentiation Process of Adipose-Derived Stem Cells. OMICS 2009; 13:331 - 336
- Lin Q, Gao Z, Alarcon RM, Ye J, Yun Z. A role of miR-27 in the regulation of adipogenesis. FEBS J 2009; 276:2348 - 2358
- Kloting N, Berthold S, Kovacs P, Schon MR, Fasshauer M, Ruschke K, et al. MicroRNA expression in human omental and subcutaneous adipose tissue. PLoS ONE 2009; 4:4699
- Wiper-Bergeron N, Salem HA, Tomlinson JJ, Wu D, Hache RJ. Glucocorticoid-stimulated preadipocyte differentiation is mediated through acetylation of C/EBPb by GCN5. Proc Natl Acad Sci USA 2007; 104:2703 - 2708
- Komori T, Yagi H, Nomura S, Yamaguchi A, Sasaki K, Deguchi K, et al. Targeted disruption of Cbfa1 results in a complete lack of bone formation owing to maturational arrest of osteoblasts. Cell 1997; 89:755 - 764
- Otto F, Thornell AP, Crompton T, Denzel A, Gilmour KC, Rosewell IR, et al. Cbfa1, a candidate gene for cleidocranial dysplasia syndrome, is essential for osteoblast differentiation and bone development. Cell 1997; 89:765 - 771
- Ducy P, Zhang R, Geoffroy V, Ridall AL, Karsenty G. Osf2/Cbfa1: a transcriptional activator of osteoblast differentiation. Cell 1997; 89:747 - 754
- Krishnan V, Bryant HU, MacDougald OA. Regulation of bone mass by Wnt signaling. J Clin Invest 2006; 116:1202 - 1209
- Molkentin JD, Olson EN. Defining the regulatory networks for muscle development. Curr Opin Genet Dev 1996; 6:445 - 453
- Molkentin JD, Olson EN. Combinatorial control of muscle development by basic helix-loop-helix and MADS-box transcription factors. Proc Natl Acad Sci USA 1996; 93:9366 - 9373
- Sakamoto H, Kogo Y, Ohgane J, Hattori N, Yagi S, Tanaka S, et al. Sequential changes in genome-wide DNA methylation status during adipocyte differentiation. Biochem Biophys Res Commun 2008; 366:360 - 366