Abstract
As the world of critical care medicine advances, extracorporeal therapies (ECC) have become commonplace in the management of the high risk intensive care patient. ECC encompasses a wide variety of technologies from hemodialysis, continuous renal replacement therapy (CRRT) and plasmapheresis, to cardiopulmonary bypass (CPB), extracorporeal life support (ECLS) and hepatic support. The development of internal man made organs is the next step with ventricular assist devices and artificial lungs. As we advance the technologies with smaller devices, and more intricate circuitry, we lack the keystone necessary to control the blood-biomaterial interface. For the last 50 years much has been learned about surface induced thrombosis and attempts have been made to prevent it with alternative systemic anticoagulation, circuitry surface modifications, or a combination of both. Despite these efforts, systemic or regional anticoagulation remain necessary for both laboratory and clinical application of ECC. As such, the development of an endothelial-like, biomimetic surface to reduce or perhaps even eliminate the blood activation/thrombus formation events that occur upon exposure to artificial surfaces is paramount.
Introduction
As the world of critical care medicine advances, extracorporeal therapies (ECC) have become commonplace in the management of the high-risk intensive care patient. ECC encompasses a wide variety of technologies, from hemodialysis, continuous renal replacement therapy (CRRT) and plasmapheresis, to cardiopulmonary bypass (CPB), extracorporeal life support (ECLS) and hepatic support. The development of internal, man-made organs is the next step, with ventricular assist devices and artificial lungs. As we advance the technologies with smaller devices and more intricate circuitry, we lack the keystone necessary to control the blood-biomaterial interface. For the last 50 years, much has been learned about surface-induced thrombosis and attempts have been made to prevent it with alternative systemic anticoagulation, circuitry surface modifications or a combination of both. Despite these efforts, systemic or regional anticoagulation remain necessary for both laboratory and clinical application of ECC. As such, the development of an endothelial-like, biomimetic surface to reduce or perhaps even eliminate the blood activation/thrombus formation events that occur upon exposure to artificial surfaces is paramount.
The blood surface interaction that evolves into thrombus formation has been well recognized since the advent of extracorporeal circuit therapies. Heparin was initially discovered in 1916 by Howell and McLean during a time of unprecedented learning and discovery in all areas of science. Once identified it required purification and extraction, which was perfected by Charles and Scott in 1933, and in 1937 Murray and Best were able to demonstrate successful thrombus prevention without toxic side effects in a dog model of vascular injury.Citation1 Simultaneously, Brinkhous and his teamCitation2 discovered that heparin required a plasma cofactor (antithrombin III) to anticoagulate blood for prevention of clotting inside and outside the body, and by 1939, Chargaff and Olson were able to show in vivo reversal of heparin anticoagulation with the use of protamine.Citation3 As this understanding came to light, Dr. John Gibbon and his wife were working on the first cardiopulmonary bypass machine, and by 1953 a perfected version, along with heparin anticoagulation, was used to support an 18 year-old female with a large ASD for 26 minutes of intracardiac surgery.
The Challenges with Present Day Management of the Blood-Biomaterial Interface
The problems of thrombosis and anticoagulation that were of concern during these times of discovery remain concerns in the present day as we advance our extracorporeal support systems. It is well known that the moment blood interacts with artificial surfaces during ECC, protein adsorption is near immediate (see ). Circulating fibrinogen and albumin are two of the main proteins that selectively adhere to the surface. As this occurs, platelets flowing near the surface end up adhering and activating, creating a cascade of events that results in thrombus formation. Platelets do not act in isolation, however, so that as this process is underway so is the activation of the coagulation cascade by the intrinsic pathway resulting in the release of inflammatory mediators and the production of thrombin. In addition, as the time on ECC increases, these interactions are escalated and feedback to further accelerate thrombus formation and inflammation mimicking an acute condition similar to disseminated intravascular coagulation and SIRS occurs.
The best example of prolonged ECC would be extracorporeal life support (ECLS) for profound cardiac or respiratory failure. It has been applied to more than 40,000 moribund patients worldwide, with survival ranging from 40% in cardiac arrest to 90% in neonatal respiratory failure (new ELSO registry data). These patients have all required systemic anticoagulation, and the gold standard is systemic heparinization. Certainly, heparin has proved useful in these therapies, as it is inexpensive, easily titrated, can be monitored at bedside for minute to minute changes and can be easily reversed by protamine. Although this makes heparin appear as the ideal anticoagulant, there are many problems with its use that can result in clinically destabilizing side effects. Thrombocytopenia is an expected side effect after the initiation of ECLS due to activation and adherence such that platelet counts can drop as low as 40% from baseline within the first 4 hours of ECLS.Citation4 Thereafter, the only means by which to maintain adequate platelet function is supportive, with platelet transfusions to maintain counts around 100,000 per microliter. Heparin does not prevent the platelet surface interactions that occur, and, in fact, heparin itself can indirectly cause further platelet activation, dysfunction and consumption.Citation5 It is therefore not surprising that bleeding complications account for >30% of all complications (patient and mechanical) on prolonged ECLS regardless of age, and the most devastating of these bleeding complications is intracranial hemorrhage as shown in .Citation6
Another more recent form of prolonged ECC is continuous renal replacement therapy (CRRT), but unlike ECLS, it only runs for 3 days before circuitry and dialysis filters require changing. In addition, the artificial surface area when compared to ECLS is smaller, and the blood flow rates are 10 times less than that during ECLS. Regardless of these differences, the blood surface interactions remain, and anticoagulation of these circuits is necessary. Initial anticoagulation was with heparin and protamine reversal, with only the circuitry receiving the heparin, not the patient, although patients essentially became systemically heparinized over time. Present day management has since changed so that most centers now regionally anticoagulate the circuit with citrate. This type of anticoagulation addresses the issue of platelet consumption, adhesion and activation during ECC by creating a calcium-poor environment within which platelets cannot function and are thus inhibited from adhesion to the circuit and its components. It obviates the need for systemic anticoagulation and theoretically allows for normal platelet function within the patient. This form of anticoagulation for ECC is not without it problems, however: (1) meticulous management of calcium levels within the patient is necessary so that already critically ill patients do not suffer further decompensation due to hypocalcemia; (2) in the smaller patient population, at least two central venous access catheters are necessary to ensure a dedicated line for calcium infusion and (3) at high blood flow rates, as with ECLS, citration of the blood and then replacement of calcium is not possible, as the transit time of the blood through the system is too short to allow this form of anticoagulation to take place without jeopardizing patient stability (citrate toxicity or hypocalcemia).
Previous and Current Coating Modifications to Prevent Thrombosis during ECC
Several companies as well as research groups have pursued the development of surface coatings to obviate the need for systemic or regional anticoagulation in extracorporeal circulation, most specifically in the therapies of CPB and ECLS. Perhaps the most well known of these are the heparin bonded surfaces, which in some centers have become the standard circuitry composition but have not yet obviated the use of systemic anticoagulation. Wendel and Ziemer as well as Mangosh reviewed the use of these commercial heparin surface treatments including the Carmedia Bioactive Surface®, Duraflo II®, BioLine®, AOThel® and Corline® coatings, as applied to extracorporeal circulation.Citation7 These surfaces have been shown to reduce the activation of the alternative complement pathway and thus the inflammatory response associated with the initiation of CPB or ECLS; the results have only shown marginal differences when compared to non-coated surfaces.Citation8 Prolonged ECC without anticoagulation in sheep has been reported using a covalently bound heparin coating. However, covalently bound heparin, which seemed so promising in the laboratory,Citation2 still requires systemic anticoagulation when used clinically,Citation9 as it has no effect on the ongoing platelet activation and production of microaggregates that no longer adhere to the circuitry surface, which instead are swept downstream into the patient. The use of heparin-bound systems has unmasked the primary problem of the blood-biomaterial interface, which is platelet adherence to the artificial surfaces followed by platelet activation, aggregation, consumption and all the other cascade of events that follow, as mentioned above. These platelet events occur in the face of systemic anticoagulation, and the systemic anticoagulation itself can lead to further platelet consumption and dysfunction, which is ultimately detrimental to the patient.
Other surface modification techniques to prevent cellular adhesion investigated for either ECLS or CPB have included using pure, very smooth silicone rubber or polyurethane, pre-exposure of the surfaces to albumin and other coating proteins as well as immobilizing synthetic functional groups such as polyethylene oxide (PEO), polyethylene glycol (MPEG), phosphorylcholine, sulfonate/sulfate groups,Citation10 L-lactide, poly (2-methylethylacrylate (PMEA) and other sugars.Citation11,Citation12 Despite extensive research to develop a non-thrombogenic surface that mimics the endothelium, platelet activation still occurs, thus these modifications have not completely solved the clinical challenges of platelet passivation.
Normal Endothelial Function
The question to be asked then is how does normal endothelium prevent thrombus formation yet encourage hemostasis when it is needed within the body. Normal endothelium is truly amazing. The endothelial cells produce, secrete, and/or express over 12 different inhibitors and activators combined that affect platelet function, the coagulation cascade or both. is a simplistic representation of many of these substances and how integral the endothelium is to normal hemostasis. As above, it is apparent that thrombin inhibition does not calm the blood-biomaterial interface and that controlling the platelet interaction at the surface may be the key to the successful creation of a compatible artificial surface. The most notable inhibitors of platelet function are nitric oxide (NO), prostacyclin and matrix metalloproteinases. In the presence of all three inhibitors, platelets are completely inhibited such that they cannot be activated through any of the multiple pathways of activation. What is unique to these inhibitors, as opposed to those used clinically for platelet inhibition, such as asprin or dipyridamol, is that this inhibition is not permanent for the life of the platelet but rather is similar to “anesthesia” of the platelet, so that once the platelet is no longer exposed to these inhibitors, it resumes normal function.
Of these three potent inhibitors, NO has by far been the most common agent studied for incorporation into polymers, likely due to its ability to be present in both liquid and gas states. If the biocompatible surface cannot been applied to the surface of gas exchange devices without jeopardizing their function, NO can be incorporated into the sweep gas of these devices. The mechanisms by which NO is able to temporarily inhibit or anesthetize platelets are both direct and indirect, and in the early 1990s, Radomski and Moncada were able to detail the exact mechanisms by which this is achieved.Citation13 The effect lasts no longer than milliseconds in a flowing system, as NO has a high binding affinity for heme iron and therefore is scavenged by several different hemoproteins within the blood. The specific effect that NO has on platelets is a result of NO binding with the hemoprotein, soluble guanylate cyclase (SGC). The result of this coupling causes the increased production of cGMP, which, in turn, directly affects platelet function by inhibiting Ca2+ influx into the platelet, inhibiting GPIIb/IIIa expression and binding with fibrinogen, inhibiting phosphorylation of myosin light chains, inhibiting phosphorylation of protein kinase C and modulating phospholipase A2- and C-mediated responses. The binding of NO to SGC also causes a decrease in the local Ca2+ concentration. Aside from binding to hemoproteins, NO also reacts with thiols in the blood, which results in the formation of S-nitrosothiols. This formation prolongs the half-life and biologic activity of NO and allows for further platelet inhibition. Consequently, those platelets that are exposed to endothelium are inhibited temporarily, while those not near the surfaces remain functional. This is one of the major mechanisms by which the endothelium prevents thrombosis in normally circulating blood, even in those areas that are stagnant, for minutes. Radomski and colleagues imparted the knowledge base necessary for the creation of artificial surfaces that mimic the endothelium, and, as such, research in this area has been under study since the early 1990s, with a now fast-approaching successful entry into the clinical arena.
Mimicking the Endothelium—The Use of Nitric Oxide (NO) to Prevent Thrombosis during ECC
There are two possible methods to deliver NO to inhibit platelet activation during ECLS:
Infuse NO into the ventilating gas of the membrane lungs in the extracorporeal life-support circuit and
Incorporate molecules that release NO in a controlled fashion into the plastic components of the circuit, creating a surface that simulates normal endothelium.
Mellgren et al. were the first to study the use of NO gas in the sweep flow of the artificial oxygenators used during ECLS. This was done using an in vitro model of ECLS with human whole blood, both control and study groups running simultaneously and the endpoints being a reduction in platelet consumption, adhesion and activation in the study groups. As expected, both activation and consumption were reduced, likely by cGMP-mediated inhibition of platelet adhesion and aggregation. Other investigators have since corroborated this finding by performing similar in vitro experiments.Citation15 This phenomenon demonstrates the non-thrombogenic effect of NO administered through an active membrane, but as of present this has not been applied clinically because the rest of the ECLS circuitry remains thrombogenic.
At the same time Radomski demonstrated the potency of NO as a reversible platelet inhibitor, Keefer et al.Citation16 performed some of the first studies using complexes of nitric oxide (NO donors) for the controlled biologic release of nitric oxide to demonstrate NO's antiplatelet effect. These NO donors were embedded into polymer matrices to create NO-releasing surfaces via terminal cleavage of NO from the donor structure under biological conditions. illustrates the basic ideology for the creation of these nonthrombogenic materials, whereby the NO donor complex is blended into the polymer materials and remains stable within the coating until exposed to physiological conditions. By 1996, Smith et al.Citation17 used several NO donor compounds in arteriovenous grafts in baboons and demonstrated reduced thrombogenicity and vascular smooth muscle proliferation in the NO-doped grafts. In 1997, in vitro studies, Espadas-Torre et al.Citation18 doped an NO compound (MAHMA/NO) into the membranes of electrochemical sensors and demonstrated reduced platelet adhesion while maintaining a functional sensor.
Simultaneously, as Smith et al. and Espandas-Torre et al. were studying NO-releasing plastics, our research laboratory began the work of applying NO-releasing materials as coatings for extracorporeal circuits. With the promise of eliminating the need for systemic anti-coagulation with the use of NO release, we formed an interdisciplinary group of leading experts in the area of NO materials in order to fabricate extracorporeal circuits that could function without systemic anticoagulation by releasing low levels of continuous NO from the circuit surface. Herein, we review our research efforts to create thromboresistant NO-releasing extracorporeal circuits.
First Generation NO-Releasing ECC
In the first studies, MAHMA/NO was incorporated into a thin layer of plasticized PVC such that the NO surface flux [the amount of NO released as a function of surface area at a given time point (mol·cm−2·min−1)] was linear throughout the entire duration of the study.Citation19 This 4 h model of ECC (see ) studied four groups of animals that included a systemically heparinized control group, a systemically heparinized NO-doped-surface group, a nonheparinized control group and a nonheparinized NO-doped-surface group. These studies demonstrated reduced platelet consumption and adhesion in the NO-doped-surface groups compared to controls, with no benefit of concomitant systemic heparinization. Scanning electron micrographs (SEM) of the NO-releasing surfaces compared to the controls (which did not possess an NO donor) clearly showed a reduction in adhered platelet number and activation. In addition, SEM confirmed findings by Salzman and colleagues that systemic heparinization has a detrimental effect on platelets, as these surfaces regardless of NO release or not, were covered by adhered dysmorphic platelets that had undergone various stages of activation and degranulation (see ). Inhibition of thrombosis in the absence of systemic heparinization using the NO-releasing circuits in our rabbit model of extracorporeal circulation (ECC) was unprecedented and these findings set the stage for the next five years of our research.
Although these MAHMA/NO-doped polymer circuits were effective in preventing thrombosis during a 4 h run of extracorporeal circulation, Mowery et al.Citation20 later determined that the entire NO donor compound leached from the polymer coating into the circulation. As a result, NO was released systemically instead of only at the blood-biomaterial interface. More concerning, however, was that the byproducts were carcinogenic nitrosamines. This led to the further development of more lipophilic NO donor compounds that remain within the polymer or NO donor moieties, which are covalently attached to the structural back-bone of the polymer itself. The successive generations of materials described below all released NO exclusively from the surface of the coating without any other potentially toxic byproducts.
Second Generation ECC
As a next step, two types of silica-based, NO-releasing materials were evaluated in our 4 h non-heparinized rabbit model of ECC. First, a NO-releasing silicone rubber (DACA-SR/NO) was tested (see structure in and ref. Citation21). This NO-releasing surface supported the previous work in that platelet consumption was reduced and no active thrombus was formation was identified on the surface. In addition, this was the first study to demonstrate NO release from a synthetic surface without leaching of other compounds.
The difficulty with using DACA-SR/NO as an NO-releasing material for circuit coatings was two-fold. First, at the desired coating thickness, DACA-SR/NO had limited time release of NO. Second, in order to achieve an adequate NO surface flux over a more prolonged time period, the coating had to be thickened significantly, resulting in technical limitations of use. This was especially limiting with the ¼” ECC circuits and 8 Fr cannulas used in the rabbit studies. These technical challenges were perceived as significant limitations to long-term applications such as ECLS in neonates.
Simultaneous with the DACA-SR/NO evaluations, a second approach was developed whereby diazeniumdiolate groups were tethered to the surface of tiny fumed silica particles (silicone dioxide, 0.2–0.3 mm) and then blended into polymeric materials.Citation22 Silica particles are routinely used as reinforcement fillers and to adjust the rheology in a wide variety of polymers. In addition to preventing leaching, this particular surface also allowed us the ability to vary and fine-tune the rate of NO release from the surface (NO surface flux) by altering the amount of polymer to Sil/NO within the matrix. It has been well described that NO flux from the endothelial surface of cultured endothelial cells is 0.5 × 10−10 mol·cm−2·min−1 and upon stimulation ranges from 1.6 to 4.1 × 10−10 mol·cm−2·min−1.Citation23,Citation24 With this understanding and the ability to vary the amount of NO flux from the surface of these extracorporeal circuits, three different NO flux groups were tested against controls in the rabbit model. As was seen with all of the other NO-releasing polymers, there was a significant reduction in platelet consumption and activation when compared to controls. In addition, when looking solely at the NO-doped groups, as NO flux dropped below 4.0 × 10−10 mol·cm−2·min−1, platelet consumption increased.
Using this information, a swine model of ECC was used to track the threshold flux at which platelet consumption would occur without systemic heparinization in a 24 h ECC run. demonstrates the threshold flux at which platelet consumption occurs (4.0 × 10−10 mol·cm−2·min−1) in this model. After 12 hours of continuous ECC and upon reaching the threshold flux, platelet counts dropped dramatically. Although these results were encouraging and aided in determining the NO flux threshold beyond which platelets are no longer preserved, the raceway of the circuit was easily delaminated when the circuits ran for prolonged periods of time. This required a return to the polymer lab for further development of a surface robust enough to perform beyond 24 hours.
Third Generation ECC
Due to the thickness and delamination difficulties observed with the silicone-based materials, coatings were fabricated by embedding discrete lipophilic NO donor complexes into plasticized PVC. The advantage to this approach is two-fold: (1) the NO flux is easily controlled without having to increase the thickness of the coating and (2) leaching is eliminated. As a result, a discrete, lipophilic analog of MAHAMA/NO was synthesized (DBHD/NO).Citation25 This compound was found to be stable within the organic phase, did not leach from the polymer coating and had the versatility to be incorporated into a variety of polymer matrices while maintaining durability. Moreover, the amount of NO release from the polymer could be exquisitely controlled by blending various amounts of the NO donor into the polymer matrix, which allowed us to prepare coatings with precisely controlled NO-release profiles. This allowed us to determine the critical NO flux necessary to prevent platelet consumption and activation at the surface of the circuitry during ECC.
We fabricated circuits that released NO at a steady state surface flux, 0, 4, 10 and 25 × 10−10 mol·cm−2·min−1 as shown in . We found, in all cases, that platelet function was preserved, but that platelet consumption was proportional to NO release in the absence of systemic anticoagulation. Furthermore, the optimal level of NO needed to preserve platelet consumption was 13.7 × 10−10 mol·cm−2·min−1. Beyond this flux, there was no additive effect of higher levels of NO release. Finally, regardless of high levels of NO release from the surfaces, potential toxic side-effects of NO, specifically, methemoglobin (metHb) production, was not observed (all levels remained less than 2%).Citation26
Summary of ECC NO-Releasing Surfaces
Taken together, we have clearly demonstrated that platelets are conserved in ECC regardless of the identity of the NO donor source. Further, as shown in , platelet consumption is decreased without compromising platelet function. At the same time, toxic indicators of NO, such as the formation of met-Hb are not observed. Artificial surfaces that mimic the endothelial surface by releasing NO are causing a localized effect on platelet activation, and the results are not systemic. This allows for normal platelet function within the critically ill patient who cannot afford to suffer hemorrhage. The positive attributes of the third generation coating make it close to ideal for conducting the definitive studies of ECC without anticoagulation in larger animals for extended time points. These studies are currently underway in our laboratory. The completion of these studies should provide valuable information regarding the true possibility of being able to perform ECC clinically without the adverse side effects of systemic drug therapies.
Future Generation ECC
Although platelet conservation has been demonstrated in these models, the use of NO donor compounds within plastics has some limitations that may need to be overcome for clinical use. First, the current coatings contain only a finite reservoir of NO donor that can be released, which limits the duration of activity, especially in the coating of small cannulae, due to the thickness necessary to have an effective surface. Secondly, extrusion is used to currently manufacture the circuitry for ECC, which requires temperatures >180°C. This temperature range is not compatible with the heat stability of the NO donor molecules. One alternative to preparing NO-producing coatings that can potentially overcome these challenges is to utilize the substantial reservoir of NO precursors already present within normal blood in the form of nitrite and S-nitrosothiols, including S-nitrosocysteine and S-nitrosoglutathione (GSNO), which are formed from reactions with oxidized NO intermediates. The Meyerhoff lab has demonstrated that thin polymers prepared with copper particles, copper(II) ligands or selenium-moeities can generate NO from S-nitrosothiols in vitro,Citation27–Citation31 although long term activity has not yet been demonstrated.
Another alternative for the preparation of non-thromogenetic coatings that mimic the endothelium is to consider other functionalities that contribute to the non-thrombogenic nature of the natural endothelium and create combination coatings. For example, the combination of NO, which acts to passivate platelets, in concert with a thrombin inhibitor may work to prevent clotting on the surfaces of ECC. Indeed, the combination of heparin-bonded surfaces with NO-releasing surfaces may, in fact, prevent both platelet activation and adhesion to surfaces while at the same time preventing the formation of a clot. In 1998, Hayashi and co-workers first demonstrated that NO gas infusion into the oxygenator off a circuit enhanced the biocompatibility of the oxygenation in heparin-coated bypass circuits.Citation32 More recently, Zhou demonstrated that a NO-releasing surface can be combined with a heparin-bonded surface to optimize both NO surface flux as well as maintain heparin activity.Citation33 This work was remarkable in that it demonstrated that the entire extracorporeal circuit can release NO as well as possess an active thrombin inhibitor, simultaneously. Finally, in a similar fashion, active thrombomodulin was immobilized onto a polymer containing an NO donor,Citation34 demonstrating again that that NO-release coatings can be fabricated in concert with NO-release strategies to get one step closer to a truly non-thrombogenic surface that mimics the natural endothelium.
The evolution of the non-thrombogenic surface has been challenging and exciting. Its realization is near, and as we work through all of the road blocks, we continue to develop more robust, reliable and endothelial-like surfaces. There is no doubt that they will be brought into the clinical arena within the next 2–5 years. This will change the course of critical care medicine and the risks to those patients who require extracorporeal therapies the most.
Figures and Tables
Figure 3 Illustration of the surface bound and released factors of the endothelium responsible for its thromboresistivity
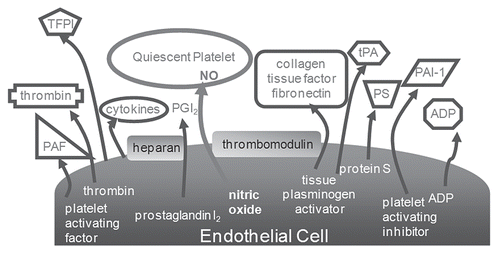
Figure 4 Schematic of experimental design used to evaluate the effect of NO-releasing coatings (materials shown beneath the model) on extracorporeal circuits.
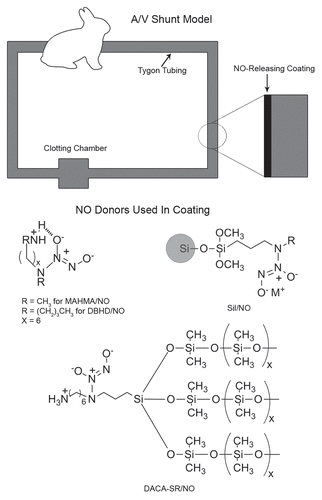
Figure 5 SEM pictures of first generation NO-releasing ECC with magnification of the outlined area adjacent. (1a and b) represent the MAHMA/NO doped circuits with and without systemic heparinization, while (2a and b) represent the controls with and without systemic heparinization. Note the dysmorphic platelets seen when systemic heparinization is present and the scarcity of platelet adherence and activation in the presence of an NO-releasing ECC.
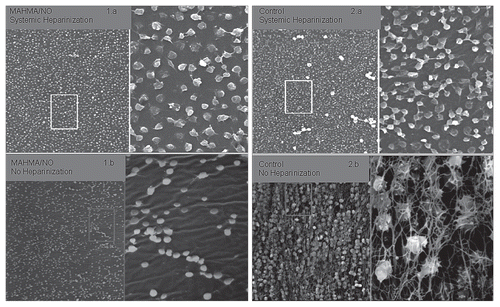
Table 1 NO surface flux as a function of weight percentage of NO donor
Table 2 Hemostatic responses to plastic materials
References
- Murray DWG, Jaques LB, Perrett TS, Best CH. Heparin and the thrombosis of veins following injury. Surgery 1937; 2:163 - 187
- Edmunds LH Jr. Hastings lecture: Breaking the blood-biomaterial barrier. ASAIO J 1995; 41:824 - 830
- Edmunds LH Jr. The evolution of cardiopulmonary bypass: Lessons to be learned. Perfusion 2002; 17:243 - 251
- Plotz FB, Wildevuur WR, Wildevuur CRH, Delius RE, Bartlett RH. Platelet consumption during neonatal extracorporeal life support (ECLS). Perfusion 1992; 7:27 - 33
- Salzman EW, Rosenberg RD, Smith MH, Lindon JN, Favreau L. Effect of heparin and heparin fractions on platelet aggregation. J Clin Invest 1980; 65:64 - 73
- ECMO Registry of the Extracorporeal Life Support Organization (ELSO) 2009; July Ann Arbor, Michigan
- Wendel HP, Ziemer G. Coating techniques to improve the hemocompatibility of artificial devices used for extracorporeal circulation. Eur J Cardiothorac Surg 1999; 16:342 - 350
- Mangoush O, Purkayastha S, Haj-Yahia S, Kinross J, Hayward M, Bartolozzi F, et al. Heparin-bonded circuits versus nonheparin-bonded circuits: an evaluation of their effect on clinical outcomes. Eur J Cardiothorac Surg 2007; 31:1058 - 1069
- Gorman RC, Ziats N, Rao AK, Gikakis N, Sun L, Khan MM, et al. Surface-bound heparin fails to reduce thrombin formation during clinical cardiopulmonary bypass. J Thorac Cardiovasc Surg 1996; 112:557 - 558
- Eynden FV, Carrier M, Ouellet S, Demers P, Forcillo J, Perrault LP, et al. AVECOR oxygenator: a randomized study. J Card Surg 2008; 23:288 - 293
- Kidane AG, Salacinski H, Tiwari A, Bruckdorfer R, Seifalian AM. Anticoagulant and antiplatelet agents: their clinical and device application(s) together with usages to engineer surfaces. Biomacromolecules 2004; 5:798 - 813
- Ranucci M, Balduini A, Ditta A, Boncilli A, Brozzi S. A systematic review of biocompatible cardiopulmonary bypass circuits and clinical outcome. Ann Thorac Surg 2009; 87:1311 - 1319
- Radomski MW, Moncada S. The biological and pharmacological role of nitric oxide in platelet function. Adv Exp Med Biol 1993; 344:251 - 265
- Mellgren K, Friberg LG, Mellgren G, Hedner T, Wennmalm A, Wadenvik H. Nitric oxide in the oxygenator sweep gas reduces platelet activation during experimental perfusion. Ann Thorac Surg 1996; 61:1194 - 1198
- Keh D, Gerlach M, Kurer I, Spielmann S, Kerner T, Busch T, et al. Nitric oxide diffusion across membrane lungs protects platelets during simulated extracorporeal circulation. Euro J Clin Inv 1999; 29:344 - 350
- Maragos CM, Morley D, Wink DA, Dunams TM, Saavedra JE, Hoffman A, et al. Complexes of NO with nucleophiles as agents for the controlled biological release of nitric oxide vasorelaxant effects. J Med Chem 1991; 34:3242 - 3247
- Smith DJ, Chakravarthy D, Pulfer S, Simmons ML, Hrabie JA, Citro ML, et al. Nitric oxide releasing polymers containing the [N(O)NO]− group. J Med Chem 1996; 39:1148 - 1156
- Espadas-Torre C, Oklejas V, Mowery K, Meyerhoff ME. Thromboresistant chemical sensors using combined nitric oxide release ion sensing polymeric films. J Am Chem Soc 2007; 119:2321 - 2322
- Annich GM, Meinhardt JP, Mowery KA, Ashton BA, Merz SI, Hirschl RB, et al. Reduced platelet activation and thrombosis in extracorporeal circuits coated with nitric oxide release polymers. Crit Care Med 2000; 28:915 - 920
- Mowery KA, Schoenfisch MH, Saavedra JE, Keefer LK, Meyerhoff ME. Preparation and characterization of hydrophobic polymeric films that are thromboresistant via nitric oxide release. Biomaterials 2000; 21:9 - 21
- Zhang H, Annich GM, Miskulin J, Osterholzer K, Merz SI, Bartlett RH, et al. Nitric oxide releasing silicone rubbers with improved blood compatibility: preparation, vharacterization and in vivo evaluation. Biomaterials 2002; 23:1485 - 1494
- Zhang H, Annich GM, Miskulin J, Stankiewicz K, Osterholzer K, Merz SI, et al. Nitric oxide-releasing fumed silica particles: Synthesis, characterization and biomedical application. J Am Chem Soc 2003; 125:5015 - 5024
- Vaughn MW, Kuo L, Liao JC. Estimation of nitric oxide production and reaction rates in tissue by use of a mathematical model. Am J Physiol 1998; 274:2163 - 2176
- Radomski MK, Palmer RM, Moncada S. The role of nitric oxide and cgmp in platelet adhesion to vascular endothelium. Biochem Biophys Res Commun 1987; 148:1482
- Batchelor MM, Reoma SL, Fleser PS, Nuthakki VK, Callahan RE, Shanley CJ, et al. More lipophilic dialkyldiamine-based diazeniumdiolates: Synthesis, characterization and application in preparing thromboresistant nitric oxide release polymeric coatings. J Med Chem 2003; 46:5153 - 5161
- Skrzypchak AM, Lafayette NG, Bartlett RH, Zhou Z, Frost MC, Meyerhoff ME, et al. Effect of varying nitric oxide release to prevent platelet consumption and preserve platelet function in an in vivo model of extracorporeal circulation. Perfusion 2007; 22:193 - 200
- Oh BK, Meyerhoff ME. Spontaneous catalytic generation of nitric oxide from S-nitrosothiols at the surface of polymer films doped with a lipophilic copper(II) complex. J Am Chem Soc 2003; 125:9552 - 9553
- Oh BK, Meyerhoff ME. Catalytic generation of nitric oxide from nitrite at the interface of polymeric films doped with lipophilic Cu(II)-complex: A potential Route to the preparation of thromboresistant coatings. Biomaterials 2004; 25:283 - 293
- Hwang S, Cha W, Meyerhoff ME. Polymethacrylates with a covalently linked Cu(II-)cyclen complex for the in situ generation of nitric oxide from nitrsothiols in blood. Angew Chem Inter Ed 2006; 45:2745 - 2748
- Hwang S, Meyerhoff ME. Polyurethane with tethered copper(II)-cyclen complex: Preparation, characterization and catalytic generation of nitric oxide from S-nitrosothiols. Biomaterials 2008; 29:2443 - 2452
- Wu YD, Rojas AP, Griffith GW, Skrzypchak AM, Lafayette N, Bartlett RH, et al. Improving blood compatibility of intravascular oxygen sensors via catalytic decomposition of s-nitrosothiols to generate nitric oxide in situ. Sensors Actuators B 2007; 1:36 - 46
- Hayashi Y, Sawa Y, Nishimura M, Chang JC, Amemiya A, Kagisaki K, et al. NO gas infusion to the oxygenator enhances the biocompatibility of heparin coated extracorporeal by pass circuits. ASAIO 1998; 44:456
- Zhou ZR, Meyerhoff ME. Preparation and characterization of polymeric coatings with combined nitric oxide release and immobilized active heparin. Biomaterials 2005; 26:6506 - 6517
- Wu B, Gerlitz B, Grinnell BW, Meyerhoff ME. Polymeric coatings that mimic the endothelium: combining nitric oxide release with surface-bound active thrombomodulin and heparin. Biomaterials 2007; 28:4047 - 4055